-
PDF
- Split View
-
Views
-
Cite
Cite
Peng Xu, Katerina Kourentzi, Richard Willson, Honghua Hu, Anand Deva, Patricia McGuire, Caroline Glicksman, Marshall Kadin, IL-9 Is a Biomarker of BIA-ALCL Detected Rapidly by Lateral Flow Assay, Aesthetic Surgery Journal, Volume 44, Issue 12, December 2024, Pages 1286–1292, https://doi.org/10.1093/asj/sjae137
- Share Icon Share
Abstract
A delayed seroma around breast implants is the most common clinical presentation of breast implant–associated anaplastic large cell lymphoma (BIA-ALCL). Interleukin-9 (IL-9), IL-10, and IL-13 concentrations are significantly higher in BIA-ALCL than in benign seromas, offering a means to distinguish between these conditions.
The aim of this research was to test the ability of a lateral flow assay (LFA) to detect high concentrations of IL-9 rapidly. In addition, the authors compared CD30 and IL-9 LFAs for distinguishing BIA-ALCL from benign seromas.
Samples of 26 seromas (15 benign, 11 malignant) were tested on in-house-prepared LFA strips for IL-9 and CD30. Nanoparticle-conjugated antibodies specific to IL-9 and CD30 were used for detection. The intensity of both the test line (TL) and a control line (CL) were analyzed and the TL/CL ratio was calculated. IL-9 protein and IL-9 transcription factor PU.1 were stained in BIA-ALCL lines and clinical samples.
The IL-9 LFA could reliably distinguish BIA-ALCL from benign seromas when the IL-9 concentration was >10 ng/ml. The CD30 LFA was positive in all 11 malignant cases. In 1 case with only faint CD30 and IL-10 TLs, the IL-9 LFA was clearly positive. Immunohistochemistry showed that IL-9 and PU.1 were present in tumor cells in BIA-ALCL lines and clinical samples.
Concentrations of IL-9 >10 ng/ml reliably distinguished BIA-ALCL from benign seromas. Moreover, the IL-9 LFA could detect BIA-ALCL when both the CD30 and IL-10 LFAs were not definitive, suggesting a multiplex LFA measuring IL-9, CD30, and IL-10 might be more effective in detecting BIA-ALCL in selected cases.
摘要
乳房植入物周围迟发性血清肿是乳房植入物相关间变性大细胞淋巴瘤 (BIA-ALCL) 最常见的临床表现。BIA-ALCL 中的白细胞介素 9 (IL-9)、IL-10 和 IL-13 浓度明显高于良性血清肿,这为区分这些病症提供了一种方法。
本研究旨在测试横向流动试验 (LFA) 快速检测高浓度 IL-9 的能力。此外,作者还比较了 CD30 和 IL-9 LFA 以区分 BIA-ALCL 和良性血清肿。
在内部制备的 LFA 条上对 26 个血清肿(15 例为良性,11 例为恶性)样本进行了 IL-9 和 CD30 测试。使用针对 IL-9 和 CD30 的纳米颗粒偶联抗体进行了检测。对测试系 (TL) 和对照系 (CL) 进行了强度分析,并计算了 TL/CL 比值。在 BIA-ALCL 系和临床样本中对 IL-9 蛋白和 IL-9 转录因子 PU.1 进行了染色。
当 IL-9 浓度 >10 ng/ml 时,IL-9 LFA 可以可靠地区分 BIA-ALCL 与良性血清肿。11 例恶性病例中 CD30 LFA 均为阳性。在 1 例仅有微弱 CD30 和 IL-10 TL 的病例中,IL-9 LFA 明显阳性。免疫组化结果显示,在 BIA-ALCL 系和临床样本的肿瘤细胞中均存在 IL-9 和 PU.1。
IL-9 浓度 >10 ng/ml 可以可靠地区分 BIA-ALCL 与良性血清肿。此外,当 CD30 和 IL-10 LFA 都不确定时,IL-9 LFA 可以检测出 BIA-ALCL,这表明在选定病例中,测量 IL-9、CD30 和 IL-10 的多重 LFA 可能更有效地检测 BIA-ALCL。
Approximately 30 million women and trans adults have received breast implants following reconstructive surgery for breast cancer because of a high genetic risk for breast cancer or for other anatomic considerations.1,2 In one prospective cohort study of patients with textured implants, the overall risk of breast implant–associated anaplastic large cell lymphoma (BIA-ALCL) was 1 in 355 women or 0.311 cases per 1000 person-years (95% CI, 0.118-0.503).3 If detected early, BIA-ALCL can be cured by surgery alone.4,5 However, if local invasion or metastases occurs, death is possible and radiation, chemotherapy, and/or immunotherapy are required.
The etiology of BIA-ALCL is controversial although its pathology commonly shows chronic inflammation, with fibrosis, plasma cells, lymphoid follicles, mast cells, and eosinophils. Chronic inflammation could be triggered by multiple, possibly combined, etiologic agents such as bacteria in a biofilm, shell shedding of particulates, and shell surface characteristics leading to friction, or by implant-associated reactive compounds.6 To better understand the pathogenesis and clinical presentation of BIA-ALCL we analyzed the cytokines in seromas and capsule surrounding breast implants. In our first study comparing cytokines of tumor cell lines, malignant seromas, and benign seromas around breast implants, 7 of 8 malignant seromas had higher levels of interleukin-9 (IL-9) and 5 of 8 had higher levels of IL-10 than benign seromas. In a second study, IL-10, IL-13, eotaxin, and IL-9 were found to have significant differences compared to all reactive seromas.7 In a third study of a larger number of different seromas, mean concentrations of IL-9, IL-10, and IL-13 were significantly higher in BIA-ALCL than in benign seromas.8 Because IL-10 had the highest predictive value for BIA-ALCL, a lateral flow assay (LFA) was developed to detect this cytokine. However, as the concentrations of IL-9 were greater than those of IL-10 in 40% of patients with BIA-ALCL in the larger study, we decided to develop an LFA for IL-9 to detect BIA-ALCL in those patients.
METHODS
Patients
Unused portions of 26 seromas (15 benign, 11 malignant) were obtained with informed consent from patients of plastic surgeons in the United States (n = 14) and Australia (n = 12). Specimens were deidentified and their use approved by IRBs of Macquarie University (reference number 5201600427) and Lifespan (reference number 1325270-6). Collection of specimens for the study began in January 2017 and was completed in August 2023. Immunohistochemical stains were done between January 2020 and September 2023. The LFAs were carried out between December 1, 2023 and March 15, 2024.
Lateral Flow Assay (LFA)—Conjugation of Latex Particles With Anti-IL-9 and Anti-CD30 Antibodies
LFAs were conducted according to a previously published protocol.8 LFA strips were prepared by striping mouse anti-IL-9 antibody (Biolegend, catalog number 507602) on nitrocellulose membrane using a BioDot dispenser (catalog number XYZ30600124). Black latex detection particles (ThermoFisher, catalog number DBK1040CA were conjugated with mouse anti-IL-9 antibody (Biolegend, catalog number 512005). After the 100 µL 0.5% solid latex particles had been rinsed three times in 50 mM MES buffer (pH 5.8), 1.2 µL of freshly prepared 50 mg/mL (N-hydroxysuccinimide, Millipore Sigma, catalog number 130672) and 1.2 µL of freshly prepared 10 mg/mL (N-[3-Dimethylaminopropyl]-N′-ethylcarbodiimide hydrochloride, Millipore Sigma, catalog number E7750-100) were sequentially added to the particles in MES buffer with sonication. After a 30-minute incubation, particles were rinsed twice with 1× PBS and then incubated with 25 µg of IL-9 antibody for 2 to 3 hours. Finally, the particles were blocked with 4% BSA in 1× PBS overnight at 4°C.
Similarly, CD30 LFA strips were made by striping mouse anti-CD30 antibody (R&D Systems, catalog number MAB2291). Detection particles were made by conjugating blue latex particles (ThermoFisher, catalog number DB1040CB) with affinity-purified goat polyclonal anti-CD30 (R&D Systems, catalog number AF229).
Seroma fluid (30 µL) was diluted to specified concentrations in 1× PBS with 0.2% Triton (PBST) mixed with 1 µL (0.45% solids) of detection particles, and pipeted onto the sample pad of the LFA strip. After 5 minutes, each strip was washed with 20 to 60 µL PBST. A positive result was a black (IL-9) or blue (CD30) test line (TL), the intensity of which corresponded to the concentration of IL-9 or CD30. A control line (CL) ensured the flow of detection particles and confirmed a negative result.
Recombinant IL-9 (R&D Systems, catalog number 209-ILB/CF) or CD30 (R&D Systems, catalog number 844155) proteins ranging from 0 to 50 ng/mL were diluted in the benign seroma and also tested on the strips as standards. The relative density of TL to CL was determined by photographing LFA strips in uniform light followed by image analysis with NIH Image J. Images were inverted to black background for intensity measurement. The TL/CL ratio was calculated and compared.
Immunohistochemistry
Following antigen retrieval by heat (90°C), 5 µm paraffin sections were stained with Biolegend MH9A4 anti-human IL-9 mouse antibody or mouse IgG2b, k isotype matched control, and 25 µg/mL PU.1 rat monoclonal IgG2A clone number 823123. Antibody staining was detected with kits from R&D Systems. Primary antibody was omitted in negative controls.
RESULTS
LFA for IL-9
A serial dilution of IL-9 protein ranging from 0 to 50 ng/mL spiked into a benign seroma was tested on IL-9 strips. The intensities of the TL and CL were measured by image analysis. The TL/CL ratio was highly correlated with IL-9 protein concentration (Figure 1; r2 = 0.93, y = 0.23x + 0.68, P < .01), indicating that the TL/CL ratio can be used to estimate IL-9 concentrations. However, it should be noted that even without added IL-9 protein a faint TL could still be detected.
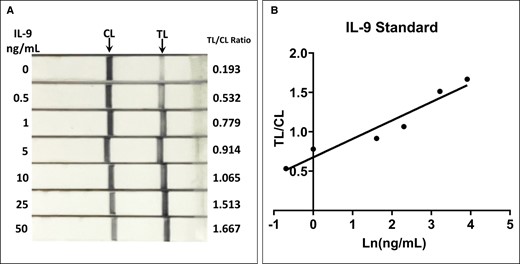
(A) IL-9 standard test on lateral flow assay strips. IL-9 protein was diluted in a benign seroma to concentrations ranging from 0 to 50 ng/mL. (B) The TL/CL, calculated by image analysis, correlated well with the measured seroma concentrations (r2 = 0.93, y = 0.23x + 0.68, P < .01). IL-9, interleukin-9; CL, control line; TL, test line.
A total of 26 seromas, 15 benign and 11 malignant, were tested with the IL-9 LFA. The TL/CL ratio for malignant seromas was significantly higher than that for benign seromas (Figure 2A; benign, 0.272 ± 0.029 [n = 15] vs malignant, 0.579 ± 0.078 [n = 11]; P < .001, t-test). The IL-9 concentration was measured in 10 benign and 8 malignant samples. Faint TLs were detected in both benign and malignant seromas with IL-9 concentrations of <10 ng/mL. However, the TL/CL ratio was significantly higher in the malignant samples with IL-9 concentrations >10 ng/mL (Figure 2B,C; benign, 0.298 ± 0.013 [n = 10]; ALCL with low IL-9, 0.372 ± 0.021 [n = 4]; ALCL with high IL-9, 0.802 ± 0.094 [n = 4]; P < .0001, one-way ANOVA). In each of those cases, the concentration of IL-9 was greater than that for IL-10 measured by flow cytometry.8 The correlation between IL-9 concentration and TL/CL ratio was also analyzed (Figure 3D; r2 = 0.58, y = 0.11x + 0.15, P = .0002).
![IL-9 lateral flow assay. (A) Comparison of 15 benign and 11 ALCL seromas (benign, 0.272 ± 0.029 [n = 15] vs ALCL, 0.579 ±0.078 [n = 11]; P < .001, t-test). Error bars indicate standard deviation. (B) Representative image of samples tested on IL-9 strips. (C) Statistical analysis among benign seromas, ALCL with low IL-9 and high IL-9 concentration samples (benign, 0.298 ± 0.013 [n = 10]; ALCL with low IL-9, 0.372 ± 0.021 [n = 4]; ALCL with high IL-9, 0.802 ± 0.094 [n = 4]; P < .0001, one-way ANOVA). (D) Correlation between measured IL-9 seroma concentrations and TL/CL ratios (r2 = 0.58, y = 0.11x + 0.15, P = .0002). ALCL, anaplastic large cell lymphoma; IL-9, interleukin-9; CL, control line; TL, test line.](https://oup.silverchair-cdn.com/oup/backfile/Content_public/Journal/asj/44/12/10.1093_asj_sjae137/1/m_sjae137f2.jpeg?Expires=1747916759&Signature=v8n87qCZeImCS-Xkrez1pMmaWadnveGqN7TtazdZ63XswUZkloS8~eTzd7NbOcuAqpAGmH9ipqFU9b7io1o605~v4~KAU0rJ3hv-UT4WIOXf0GynXQo6Ru7KgqqOfNL~lqjo6JfC7rsX6IIL7MvwVqshtcpiy9tdv1ZRo4R6yxV3FMrTXZ7cmEf~Rq-UZ6Z036daX0Gg52fSfoPH8DWyZX0FhkU-h7KN2b8etCwfnMe8a-jcSy5nPHYdylVPagF4B7Acc8eLU4ffI8ecW19nqgkMOaBpOSXtsbdLXqaSv9ihBF0O8RsCSrg0zl3cXey4QPm8TNxk2R7o52XAc7oZBg__&Key-Pair-Id=APKAIE5G5CRDK6RD3PGA)
IL-9 lateral flow assay. (A) Comparison of 15 benign and 11 ALCL seromas (benign, 0.272 ± 0.029 [n = 15] vs ALCL, 0.579 ±0.078 [n = 11]; P < .001, t-test). Error bars indicate standard deviation. (B) Representative image of samples tested on IL-9 strips. (C) Statistical analysis among benign seromas, ALCL with low IL-9 and high IL-9 concentration samples (benign, 0.298 ± 0.013 [n = 10]; ALCL with low IL-9, 0.372 ± 0.021 [n = 4]; ALCL with high IL-9, 0.802 ± 0.094 [n = 4]; P < .0001, one-way ANOVA). (D) Correlation between measured IL-9 seroma concentrations and TL/CL ratios (r2 = 0.58, y = 0.11x + 0.15, P = .0002). ALCL, anaplastic large cell lymphoma; IL-9, interleukin-9; CL, control line; TL, test line.
![CD30 lateral flow assay. (A) CD30 standards were diluted from 0 to 10 ng/mL in a benign seroma. (B) Correlation of CD30 concentration with TL/CL ratio (r2 = 0.72, y = 0.05x + 0.7, P = .02). (C) Comparison of CD30 in benign and ALCL seromas (benign, 0.198 ± 0.036 [n = 15] vs ALCL, 0.779 ± 0.083 [n = 11]; P < .0001, t-test). CL, control line; TL, test line.](https://oup.silverchair-cdn.com/oup/backfile/Content_public/Journal/asj/44/12/10.1093_asj_sjae137/1/m_sjae137f3.jpeg?Expires=1747916759&Signature=ZMM1fweegPSRK2HpGFv4wVbXOvAY0N4jOIAQ8ZvHK7pR6OdT6aiCyjMIYJsZzRyvCa8h63D7xxgs9WxeSUUBBmgHZwjZutnQjFky~nia9XsK0yJCiqfPY-gPEn6qV8CfjklD~uVAb7nsfI-240u-EhHDBhjnY7w-CpfaFXuVEB6E1vCb4R7SvDWdmMTo7DOfrnvqWg20KLNX2Su0q8ef72MuytAa7sO~eCM4DHRMYliWRIuHtqCEyMc0T9cSNSWc5g5SCjdI3LG5b-oVlE9KDim6tNzpFauc-MnqQd4nZRMaprkVYgsxCki3owgYlsrluEClK67T2ep2aPHfRWkD~g__&Key-Pair-Id=APKAIE5G5CRDK6RD3PGA)
CD30 lateral flow assay. (A) CD30 standards were diluted from 0 to 10 ng/mL in a benign seroma. (B) Correlation of CD30 concentration with TL/CL ratio (r2 = 0.72, y = 0.05x + 0.7, P = .02). (C) Comparison of CD30 in benign and ALCL seromas (benign, 0.198 ± 0.036 [n = 15] vs ALCL, 0.779 ± 0.083 [n = 11]; P < .0001, t-test). CL, control line; TL, test line.
LFA for CD30
A CD30 LFA was tested on the same seromas and the results of the 2 assays were compared. Figure 3A,B shows the correlation between the TL/CL ratio and the concentration of CD30 standards (r2 = 0.72, y = 0.05x + 0.7, P = .02). The TL/CL ratio for CD30 was >0.6 in 6 of 9 cases and significantly higher in ALCL than in benign samples (Figure 3C; benign, 0.198 ± 0.036 [n = 15] vs ALCL, 0.779 ± 0.083 [n = 11]; P < .0001, t-test). Error bars indicate standard deviation. In 1 case (1817) in which the CD30 TL/CL ratio was <0.6, the IL-9 LFA was clearly positive for BIA-ALCL (Figure 4). IL-9 LFAs for S1817 in Figure 4 and Figure 2B were prepared on separate strips with different sample aliquots explaining the different TL/CL ratios.
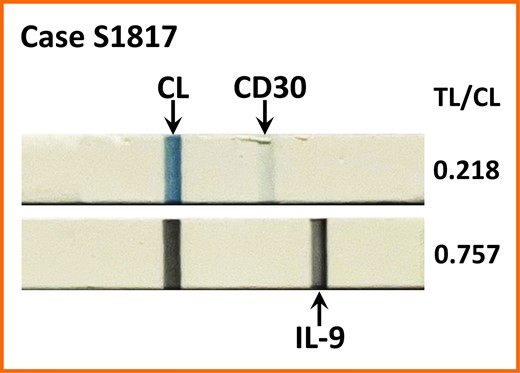
IL-9 lateral flow assay yields a strong TL in case S1817 with only a faint TL for CD30. CL, control line; TL, test line.
All patients with BIA-ALCL had textured implants. The manufacturer and implant type when known is shown in Supplemental Table 1, available online at www.aestheticsurgeryjournal.com. All patients with BIA-ALCL presented with Stage IA disease except patient 1610 who had Stage IIA disease with the highest level of CD30 but <10 ng/mL IL-9. Despite this interesting difference, there are too few patients to determine a significant correlation of CD30 levels with disease stage.
IL-9 and PU.1 Expression in Tumor Cells and Tissue
To determine if IL-9 is produced by tumor cells, we stained BIA-ALCL cell lines and clinical cases for IL-9. Figure 5A,B shows intracellular staining of IL-9 in the BIA-ALCL line TLBR1 and a representative clinical sample. Because PU.1 is a transcription factor necessary for IL-9 production9 we stained clinical cases for PU.1. Figure 5C shows nuclear staining of tumor cells for PU.1.
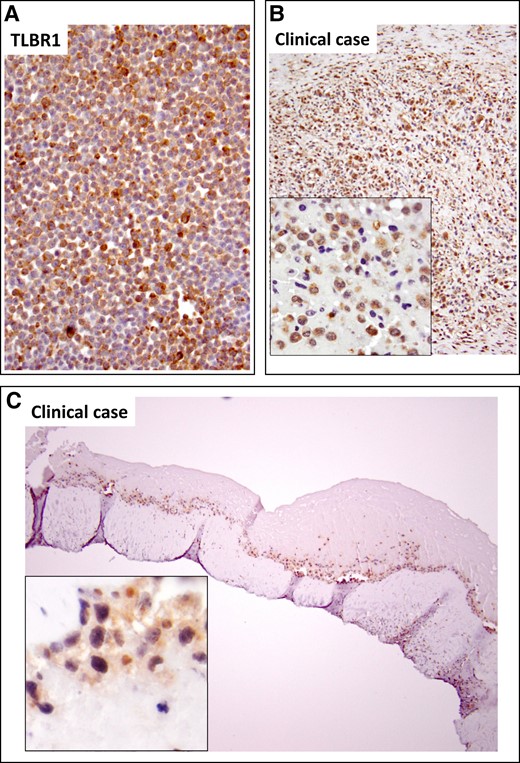
IL-9 and PU.1 immunochemistry. IL-9 was localized to tumor cells in the breast implant–associated anaplastic large cell lymphoma TLBR1 cell line (A) and clinical sample (B). (C) The transcription factor PU.1 necessary for IL-9 production was detected in nuclei of tumor cells. IL-9, interleukin-9.
DISCUSSION
This study shows that an LFA for IL-9 can distinguish BIA-ALCL from benign seromas when the concentration of IL-9 is >10 ng/mL. In cases when the IL-9 concentration is low (<10,000 pg/mL), CD30 is diagnostic of BIA-ALCL. Interestingly, in 1 case with an IL-9 concentration of >10 ng/ml and a positive IL-9 LFA, the CD30 LFA TL/CL ratio was low, producing a faint TL; in this case the concentration of IL-10, another marker of BIA-ALCL, was only 6 pg/mL, and an IL-10 LFA produced a faint TL. Hence a multiplex of IL-9, IL-10, and CD30 would be desirable for future screening of patients suspected of having BIA-ALCL.
IL-9 is a pleotropic cytokine produced by a variety of immune cells in different amounts. IL-9 is considered to be a product of CD4+ T cells, termed Th9 cells. Ramming et al found that differentiation of Th9 cells is controlled by unique and dynamic epigenetic modifications in the promoter region of its main transcription factor, PU.1.10 Naive T cells required more intense stimulation to switch the chromatin pattern in the PU.1 promoter from a repressive to a permissive state, and therefore to produce IL-9, than did memory T cells. This appears to be consistent with the hypothesis that ALCL cells are derived from immature thymocytes.11 We detected IL-9 and PU.1 in tumor cells in cell lines and clinical samples. The source of IL-9 from tumor cells is consistent with our finding of IL-9 in supernatants of 3 of 4 TLBR cell lines, proportional to the number of tumor cells.12
Accumulating evidence from transgenic mice reveals that systemic or lung-specific overexpression of IL-9 causes an asthmatic phenotype, including eosinophilic and lymphocyte inflammation, increased immunoglobulin E production, subepithelial collagen deposition, and mast cell hyperplasia.13,14 We described similar pathologic features in capsules surrounding implants of patients with BIA-ALCL.15
IL-9 plays a role in the pathogenesis of other lymphomas. Lv et al reported elevated serum levels of IL-9 in diffuse large B-cell lymphoma patients (24/30) compared with healthy controls (0/15); positive expression of IL-9 was correlated with adverse prognosis indicators.16 Kumar et al showed that malignant T cells in cutaneous T-cell lymphoma express IL-9 and IL-9R, suggesting an autocrine feedback loop.17 Serum levels of up to 3350 pg/mL were found in patients with nodular sclerosing Hodgkin lymphoma and were associated with negative prognostic factors including systemic B symptoms.18 We have previously described IL-9 activity in neoplastic cells of systemic ALCL.19 A recent report indicates that IL-9 is significantly elevated in pretreatment sera of pediatric patients with anaplastic lymphoma kinase (ALK)+ ALCL.20 Qiu et al detected IL-9 in serum-free culture medium harvested from ALK+ ALCL cell lines.21 Treatment of these cell lines with an anti-IL-9-neutralizing antibody decreased phosphorylated JAK3 (pJAK3) and its kinase activity, along with pStat3 and ALK activity. These effects were associated with decreased cell proliferation and colony formation in soft agar and cell-cycle arrest. Thus, detection of IL-9 by LFA could have applications to other lymphomas including systemic ALCL.
This study was limited by the low number of malignant samples from this rare disease available for testing. We plan a larger prospective study to test the reproducibility of our LFAs at different sites. We also plan to obtain more patients with staging information and implant type to predict outcome as well as to detect BIA-ALCL. Development of a multiplex LFA is technically challenging because of the difficulty having the different detection reagents run together. We are working to address these issues.
CONCLUSIONS
IL-9 is a tumor cell biomarker of BIA-ALCL that can be detected by immunohistochemistry and LFA. Concentrations of IL-9 >10 ng/mL reliably distinguished BIA-ALCL from benign seromas. Moreover, IL-9 LFA can detect BIA-ALCL when CD30 LFA is not definitive and IL-10 is of low concentration with a faint or absent LFA TL, suggesting that a multiplex LFA for IL-9, CD30, and IL-10 might be more effective in detecting BIA-ALCL in selected cases. We recommend that LFAs be used as a rapid screening test with final diagnosis by morphology together with immunohistochemistry or flow cytometry.
Supplemental Material
This article contains Supplemental material located online at www.aestheticsurgeryjournal.com.
Disclosures
Members of The Aesthetic Society (Garden Grove, CA) contributed research samples. The authors declared no potential conflicts of interest with respect to the research, authorship, and publication of this article.
Funding
This study was supported by a research grant from The Aesthetic Foundation.
REFERENCES
Author notes
Dr Xu is a senior research scientist, Department of Pathology, University of Virginia, Charlottesville, VA, USA.
Dr Kourentzi is an associate professor, William A. Brookshire Department of Chemical and Biomolecular Engineering, University of Houston, Houston, TX, USA.
Dr Wilson is the chair, William A. Brookshire Department of Chemical and Biomolecular Engineering, University of Houston, Houston, TX, USA.
Dr Hu is a research fellow, Macquarie Medical School, Faculty of Medicine, Health and Human Sciences, Macquarie University, Sydney, Australia.
Dr Deva is a professor, Macquarie Medical School, Faculty of Medicine, Health and Human Sciences, Macquarie University, Sydney, Australia. Dr Deva is an international editor for Aesthetic Surgery Journal, MO, USA
Dr McGuire is a plastic surgeon in private practice in St Louis, MO, USA; and is the Aesthetic Breast Surgery section co-editor for Aesthetic Surgery Journal.
Dr Glicksman is a clinical assistant professor, Hackensack Meridian School of Medicine, Nutley, NJ, USA; and is the Aesthetic Breast Surgery section co-editor for Aesthetic Surgery Journal.
Dr Kadin is a professor, Department of Pathology, University of Virginia, Charlottesville, VA, USA.