-
PDF
- Split View
-
Views
-
Cite
Cite
Krishnav Biyani, Durgesh Kumar Tripathi, Jeong Hyun Lee, Sowbiya Muneer, Dynamic role of iron supply in amelioration of cadmium stress by modulating antioxidative pathways and peroxidase enzymes in mungbean, AoB PLANTS, Volume 11, Issue 2, April 2019, plz005, https://doi.org/10.1093/aobpla/plz005
- Share Icon Share
Abstract
Iron deficiency and cadmium (Cd) stress causes a rapid change in plant physiology. The aim of this work was to characterize the effects of Fe supplementation on redox reactions in leguminous plants under Cd toxicity. The experiments were performed in hydroponic nutrient media, using mungbean grown under 300 µM cadmium chloride with or without Fe for 10 days. The Fe concentration in plants grown under Fe deficiency was reduced by the presence of Cd; however, it recovered to control levels after Fe was supplied. Similarly, a very high Cd concentration was observed in plants grown under Fe deficiency in the presence of Cd toxicity but when Fe was supplied the Cd concentration decreased. After 5 days of Fe deficiency, the activities of antioxidant enzymes (superoxide dismutase, catalase, and glutathione reductase and ascorbate peroxidase) were significantly higher than in plants supplied with Fe in the absence or presence of Cd. However, after 10 days of Fe deficiency, these enzyme activities were severely decreased in the presence of Cd toxicity but increased when Fe was present. The activities of other peroxidase enzymes such as guaiacol peroxidase, syringaldazine peroxidase, polyphenol oxidase and benzidine peroxidase decreased after 10 days under Cd toxicity and were further reduced in the absence of Fe. Furthermore, the level of reduced glutathione and oxidized glutathione increased for the first 5 days under Cd toxicity under Fe deficiency but was reduced after Fe was supplied to Cd-treated plants. The data indicate that supply of Fe contributes to the alleviation of Cd toxicity in redox reaction pathways in mungbean plants.
Introduction
Although iron is abundant in the earth’s crust, it is largely unavailable for plants, especially those growing in alkaline and calcareous soils. Higher plants such as graminaceous plants and dicots acquire iron in the form of phytosiderophores, which solubilize ferric Fe in the rhizosphere (Romheld and Marschner 1996; Cohen et al. 1998; Kobayashi et al. 2006; Meda et al. 2007). Unlike graminaceous plants, legumes often experience Fe deficiency, which primarily affects their seed development (Santos et al. 2013). Moreover, plants under Fe deficiency express alterations in several physiological parameters such as in photosynthesis, respiration, nitrogen fixation, DNA synthesis and chlorophyll formation (Vasconcelos et al. 2006; Eva et al. 2011; Muneer et al. 2012).
When Fe uptake is very low in plants, they often accumulate toxic metals in their roots (Kobayashi et al. 2006; Eva et al. 2011). Cadmium is a common toxic metal that affects plant growth and development. Cadmium enters plants via roots and hinders basic metabolic reactions such as photosynthesis (Hasan et al. 2009). Cadmium induces oxidative stress by generating reactive oxygen species (ROS) (Romero et al. 2007) in the form of thiobarbituric acid (TBARS), hydrogen peroxide (H2O2) and singlet oxygen (O2−1). Reactive oxygen species are considered an unavoidable by-product of normal aerobic metabolism that can disrupt cellular homeostasis (Mittler 2002). Reactive oxygen species can also impact the expression of several genes and can also influence signal transduction pathways in many biological processes (Pitzschke et al. 2006). Reactive oxygen species react with several other biomolecules such as lipids, proteins and nucleic acids, causing lipid peroxidation (Muneer et al. 2014). However, plants use a diverse group of redox reactions involving superoxide dismutase (SOD), ascorbate peroxidase (APX), catalase (CAT), glutathione reductase (GR) and low molecular weight antioxidants such as reduced glutathione (GSH) and oxidized glutathione (GSSG) to scavenge different types of ROS and thereby protect cells against injury and potential damage (Mittler 2002).
The non-specific enzyme guaiacol peroxidase (GPOX) can metabolize guaiacol as a substrate and can function as an effective quencher of ROS in cells (Van Assche and Clijsters 1990). Several other peroxidase enzymes such as syringaldazine peroxidase (SPOX), polyphenol oxidase (PPO) and benzidine peroxidase (BPOX) are also known as stress ameliorating enzymes as well as being involved in lignin biosynthesis (Lee et al. 2007). In conjunction with these enzymes SOD, CAT, APX, DHAR (dehydroascrobate reductase), GR, GSH and GSSG play an essential role in the scavenging process. The improved and amended expression of these redox enzymes in response to biotic or abiotic stress is already well known (Bowler et al. 1992; Eide et al. 1996; Lee et al. 2009).
Although several studies have reported the effect of Fe levels on Cd toxicity in Strategy I plants (all plants except graminaceous monocots) based on the response to iron shortage (Eide et al. 1996; Korshunova et al. 1999; Vert et al. 2002; Yoshihara et al. 2006), little is known about the response to Cd toxicity in Fe-deficient leguminous plants (Fabaceae family) and particularly in mungbean plants, which are consumed in high quantities throughout the world (according to FAO statistics 2011). In a previous study, we analysed leguminous plants under low levels of Cd toxicity (Muneer et al. 2012, 2013), but due to several anthropogenic activities, Cd levels in the soil have been increasing (López-Millán et al. 2009; Hashem 2013; Sánchez-Pardo et al. 2013), resulting in severe loss of crop productivity. Thus, the main aim of this study was to determine whether mungbean seedlings are able to modulate the uptake of Cd under supplementation of Fe for two different time intervals.
Materials and Methods
Growing conditions and Fe/Cd combined treatments
Surface-sterilized seeds of mungbean (Vigna radiata var. radiata, Korea) were germinated as described previously (Muneer et al. 2012). Experiments were conducted in 300 mL magenta boxes in a controlled plant growth chamber with a 16 h photoperiod (under 100 µmol m−2 s−1 light). A day/night temperature and relative humidity regimes of 25 ± 2 °C and 70 % were implemented, respectively. Seeds were washed with 1 % sodium hypochlorite for 10 min followed by three washings in double-distilled water. Sterilized seeds were germinated in the dark on wet filter paper in covered petri dishes for 2 days. The germinated seeds were then transferred to quarter-strength Hoagland nutrient solution for an additional 14 days containing (at mM concentrations for the macro elements): 1.0 NH4NO3; 0.4 KH2PO4; 3.0 CaCl2; 1.5 MgSO4; 0.15 K2HPO4; 0.2 Fe(III)-EDTA; and (µM for the micro elements): 14 H3BO3; 5.0 MnSO4·H2O; 3.0 ZnSO4·7H2O; 0.7 CuSO4·5H2O; 0.7 (NH4)6MO7O24; 0.1 COCl2. The nutrient solution was continuously aerated and renewed every 3 days. On the 15th day, young mungbean plants were divided into four treatment groups receiving different treatments of Fe and Cd (CdCl2): adequate Fe (+Fe/−Cd, control), adequate Fe plus 300 µM Cd (+Fe/+Cd), Fe-deprived without Cd (−Fe/−Cd) and Fe-deprived in presence of Cd (300 µM) (−Fe/+Cd), with four replicates. A high concentration of CdCl2 (300 µM) was used in this study, in contrast to our previous studies, in which plants were treated with a moderate concentration of 50 µM CdCl2 (Muneer et al. 2012, 2013). Fe deficiency was achieved by removing Fe sources (Fe(III)-EDTA) from the nutrient medium. The light conditions were supplemented using fluorescent light at a canopy height of 200 µmol m−2 s−1 for 16 h per day. After 5 and 10 days of treatment, plant samples were taken and frozen in liquid nitrogen and stored in a freezer (−80 °C) for further analysis. To analyse samples for chemical parameters, samples were dried in an oven for 24–48 h. For experiments 10–15 magenta boxes (containing four seedlings) for each treatment were used (Fig. 1 is a representation of the four treatments).
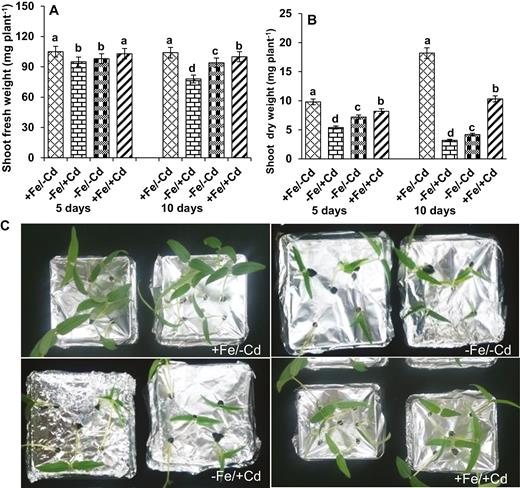
Changes in biomass (A) fresh weight, (B) dry weight and (C) morphological differences in leaves as affected by four Fe/Cd combined treatments: adequate Fe with −Cd (+Fe/−Cd, control), Fe-deficient with Cd stress (−Fe/+Cd), Fe-deficient –in absence of Cd (−Fe/−Cd) and adequate Fe with Cd stress (+Fe/+Cd) for 10 days. Vertical bars indicate ±SE of the means for n = 4. Means denoted by a different letter are significantly different at P < 0.05 according to the Tukey’s studentized range test.
Assessment of biomass and chemical analysis
The assessment of shoot biomass and chemical analysis of Fe and Cd concentrations were performed as described previously (Muneer et al. 2012).
Determination of oxidative stress (TBARS and H2O2)
Thiobarbituric acid reactive substances (TBARS) were determined using the method of Heath and Packer (1968). Hydrogen peroxide was determined as described by Lin and Kao (2001a).
Determination of non-protein thiol content and pigment analysis
Non-protein thiol contents were determined as described by Del Longo et al. (1993), while pigments like chlorophyll and carotenoid were determined using the dimethyl sulfoxide (DMSO) method, as previously described by Hiscox and Israclstam (1979), and calculated using formulae given by Arnon (1949).
Enzyme assays
Ascorbate peroxidase activity was estimated according to Nokano et al. (2006), while SOD activity was determined using the method of Dhindsa et al. (1981), and CAT activity was determined using the method of Aebi (1984). Glutathione reductase activity was determined as described by Rao (1992).
Determination of GSH and GSSG
Reduced GSH and oxidized GSSG were determined using the glutathione recycling method described by Anderson (1985).
Peroxidase enzyme assays
Peroxidase enzymes were extracted as described by Lee et al. (2007) and the activity of each enzyme was measured using appropriate substrates. Guaiacol peroxidase activity was detected according to Lee and Lin (1995). Benzidine peroxidase activity was estimated according to Cuypers et al. (2002), while SPOX activity was determined as described by Quiroga et al. (2000). Polyphenol oxidase activity was determined according to the method of Siriphanich and Kader (1985).
Active staining of peroxidase-related enzymes
The peroxidase active staining of GPOX, SPOX, BPOX and PPO was determined as described by Lee et al. (2007).
Statistical analysis
The results were analysed using statistical analysis software (SAS), with four replicates for each treatment. The conclusions were based on differences between means, with a significance level set at P < 0.05.
Results
Shoot biomass analysis under Fe and Cd treatments
Cd stress caused a significant decrease in shoot fresh and dry weight (Fig. 1A and B). The fresh and dry biomass accumulation of Cd-treated plants was significantly lower than in plants that were adequately supplemented with Fe (+Fe/+Cd). The greatest reduction was observed in −Fe/+Cd plants after 10 days of treatment (Fig. 1C, visual differences), whereas this effect was reversed after the addition of Fe to the plants (+Fe/+Cd), even in the presence of Cd treatment.
Fe and Cd concentration
The concentration of Fe provides a sensitive marker with which to evaluate the response of normal or Fe-deficient plants to Cd exposure. Plants responded to Fe deprivation by displaying a 54 % reduction in Fe concentration for the first 5 days (Fig. 2A). This reduction was more severe in Cd-treated plants (−Fe/+Cd) after 10 days of treatment (76 %), whereas Fe supplementation counteracted the negative effect of Cd toxicity. A high concentration of Cd was observed in Cd-treated plants (Fig. 2B), but the concentration decreased slowly in Cd-treated plants supplemented with Fe.
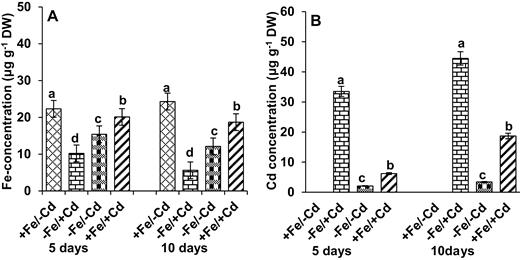
Changes in the concentration of (A) Fe and (B) Cd in leaves as affected by four Fe/Cd combined treatments: adequate Fe with −Cd (+Fe/−Cd, control), Fe-deficient with Cd stress (−Fe/+Cd), Fe-deficient –in absence of Cd (−Fe/−Cd) and adequate Fe with Cd stress (+Fe/+Cd) for 10 days. Vertical bars indicate ±SE of the means for n = 4. Means denoted by a different letter are significantly different at P < 0.05 according to the Tukey’s studentized range test.
Production of ROS in response to Cd toxicity and its alleviation by Fe supplementation
Cd-treated young mungbean leaves exhibited ROS accumulation in the form of TBARS and H2O2. Thiobarbituric acid reactive substance content increased significantly in Fe-deficient plants (Fig. 3A), and further increased following the addition of Cd (−Fe/+Cd). However, the concentration of TBARS decreased when Cd-treated plants were supplied with Fe (+Fe/+Cd).
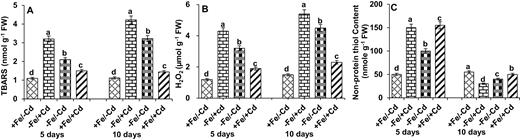
Reactive oxygen species production in the form of (A) TBARS and (B) H2O2 and oxidative marker in the form of (C) non-protein thiol content in leaves as affected by four Fe/Cd combined treatments: adequate Fe with −Cd (+Fe/−Cd, control), Fe-deficient with Cd stress (−Fe/+Cd), Fe-deficient –in absence of Cd (−Fe/−Cd) and adequate Fe with Cd stress (+Fe/+Cd) for 10 days. Vertical bars indicate ±SE of the means for n = 4. Means denoted by a different letter are significantly different at P < 0.05 according to the Tukey’s studentized range test.
Similarly, H2O2 concentration increased by 166 % in the first 5 days in the presence of Fe and Cd (+Fe/+Cd) and increased even more (by 258 %) in the absence of Fe (−Fe/+Cd) (Fig. 3B). The H2O2 concentration increased by 260 % after 10 days of Fe deprivation in Cd-treated plants (−Fe/+Cd). The H2O2 levels recovered when Fe was supplied to Cd-treated plants.
Non-protein thiol content under Fe and Cd treatment
Non-protein thiol contents were used as an oxidative marker for Cd treatments (Fig. 3C). Fe-deficient plants increased their content of non-protein thiols during the first 5 days under Cd treatment (−Fe/+Cd). However, after 10 days of Fe deficiency, non-protein thiols decreased significantly by 45 % in Cd-treated plants, but when Fe was resupplied (+Fe/+Cd) to Cd-treated plants, the thiol content increased by 9 % compared to the control.
Degradation of photosynthetic pigments due to Cd toxicity and its improvement in response to Fe supplementation
After 10 days of Fe/Cd combined treatment the total chlorophyll and carotenoid content in Fe-deprived plants decreased significantly relative to the control (−Fe/−Cd) (Fig. 4A and B). Levels decreased more severely (by 74 and 54 %, respectively) in Cd-treated plants (−Fe/+Cd), but when Cd-treated plants were supplemented with Fe, the total chlorophyll and carotenoid content increased.
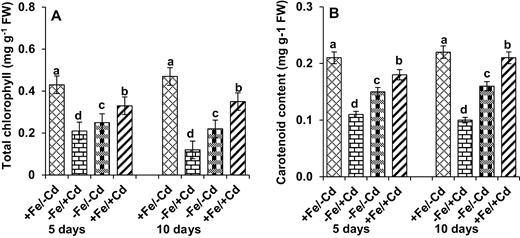
Changes in (A) total chlorophyll and (B) carotenoid content in leaves as affected by four Fe/Cd combined treatments: adequate Fe with −Cd (+Fe/−Cd, control), Fe-deficient with Cd stress (−Fe/+Cd), Fe-deficient –in absence of Cd (−Fe/−Cd) and adequate Fe with Cd stress (+Fe/+Cd) for 10 days. Vertical bars indicate ±SE of the means for n = 4. Means denoted by a different letter are significantly different at P < 0.05 according to the Tukey’s studentized range test.
Changes in enzyme activities in response to Cd toxicity and their improvement in response to Fe supplementation
The antioxidant enzymes were induced as follows under Fe deprivation: SOD by 23 %, CAT by 38 %, GR by 94 % and APX by 65 %. The activity of these enzymes was most affected under Cd toxicity in the absence of Fe (−Fe/+Cd). After 10 days of Fe deprivation, the activity of these enzymes was reduced by >90 % on average compared to control young mungbean seedlings and decreased by 116 % for APX relative to the control (Fig. 5). The activity of these enzymes recovered when Fe was supplied.
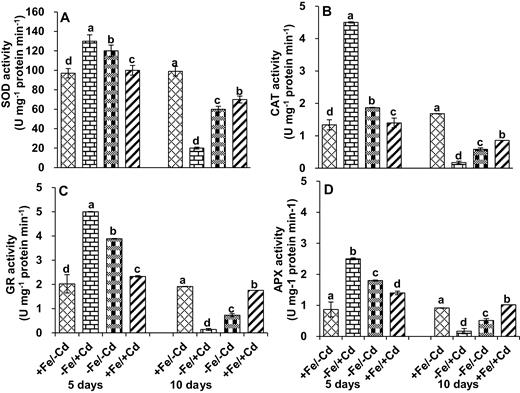
Antioxidant enzyme activity: (A) superoxide dismutase (SOD), (B) catalase activity (CAT), (C) glutathione reductase activity (GR) and (D) ascorbate peroxidase activity (APX) in leaves as affected by four Fe/Cd combined treatments: adequate Fe with −Cd (+Fe/−Cd, control), Fe-deficient with Cd stress (−Fe/+Cd), Fe-deficient –in absence of Cd (−Fe/−Cd) and adequate with Cd stress (+Fe/+Cd) for 10 days. Vertical bars indicate ±SE of the means for n = 4. Means denoted by a different letter are significantly different at P < 0.05 according to the Tukey’s studentized range test.
The levels of GSH and GSSG in mungbean seedlings under Fe deprivation (−Fe/−Cd) increased by 116 and 50 %, respectively, in the first 5 days (Fig. 6A and B). After 10 days of Cd toxicity under Fe deprivation the level of GSH and GSSG was reduced by 82 and 78 %, respectively (−Fe/+Cd), whereas the negative effects of Cd treatment in mungbean plants on levels of GSH and GSSG were recovered by Fe supplementation.
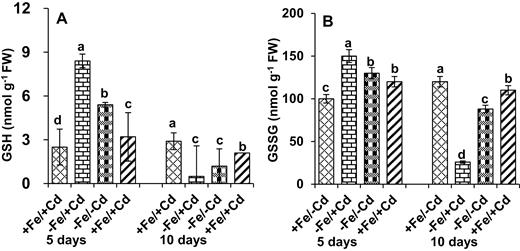
Non-enzymatic antioxidant activity: (A) GSH and (B) GSSG in leaves as affected by four Fe/Cd combined treatments: adequate Fe with −Cd (+Fe/−Cd, control), Fe-deficient with Cd stress (−Fe/+Cd), Fe-deficient –in absence of Cd (−Fe/−Cd) and adequate Fe with Cd stress (+Fe/+Cd) for 10 days. Vertical bars indicate ±SE of the means for n = 4. Means denoted by a different letter are significantly different at P < 0.05 according to the Tukey’s studentized range test.
Guaiacol peroxidase activity (Fig. 7A) was significantly induced for the first 5 days of Cd toxicity under Fe deprivation in mungbean seedlings; however, 10 days after Fe deprivation GPOX was significantly reduced by 63 % (−Fe/−Cd), but increased by 75 % under Cd treatment (−Fe/+Cd). Conversely, the activity of GPOX was maintained at control levels in Cd-treated plants when Fe was supplied. In mungbean plants a rapid 2.5-fold increase in BPOX was observed under Fe deprivation (−Fe/−Cd), with a 4-fold increase under Cd treatment with Fe deprivation (−Fe/+Cd) (Fig. 7B). Reduced activity of BPOX was observed after 10 days of Fe deprivation under Cd treatment, whereas its activity returned to control levels when Fe was supplied to Cd-treated plants. Similarly, SPOX increased significantly by 30 % under Fe deprivation (Fig. 7C) and a 50 % induction of SPOX was observed in Cd-treated, Fe-deprived plants. Conversely, the activity of SPOX in Fe-deprived plants was reduced by >58 % under Cd treatment, and returned to control levels in mungbean plants under Cd treatment with Fe supplementation. A significant induction of PPO (Fig. 7D) was observed in mungbean plants under Cd treatment with Fe deprivation 5 days after treatment, and a significant reduction 10 days after treatment. The negative effect of Cd toxicity on PPO activity was reversed by Fe supplementation.
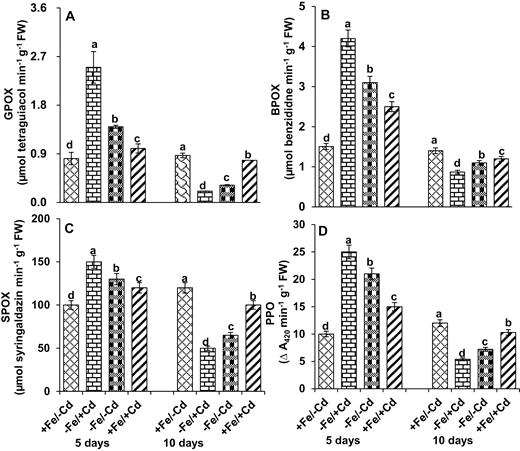
Peroxidase-related enzyme activities: (A) guaiacol peroxidase (GPOX), (B) benzidine peroxidase (BPOX), (C) syringaldazine peroxidase (SPOX) and (D) polyphenol oxidase (PPO) in leaves as affected by four Fe/Cd combined treatments: adequate Fe with −Cd (+Fe/−Cd, control), Fe-deficient with Cd stress (−Fe/+Cd), Fe-deficient in absence of Cd (−Fe/−Cd) and adequate Fe with Cd stress (+Fe/+Cd) for 10 days. Vertical bars indicate ±SE of the means for n = 4. Means denoted by a different letter are significantly different at P < 0.05 according to the Tukey’s studentized range test.
Changes in peroxidase isozymes in response to Cd toxicity and impact of Fe supplementation
After NATIVE-PAGE, the active staining of major peroxidase enzymes was used to identify the expression of isozymes in mungbean leaves. The GPOX isozymes (at 64.7 kDa) were substantially induced in Fe-deprived plants under Cd treatment (Fig. 8) for the first 5 days, whereas expression was reduced after 10 days of Cd treatment under Fe deprivation. The expression level of GPOX was maintained at control levels when Fe was supplied to Cd-treated plants. Syringaldazine peroxidase isozymes are commonly detected at 51.4 kDa and were detected in all plants, with a higher expression level after 5 days in Cd-treated plants under Fe deprivation and a low expression level after 10 days (−Fe/+Cd). The expression of SPOX was maintained at control levels by supplying Fe to Cd-treated mungbean plants (+Fe/+Cd). Like GPOX and SPOX, the expression level of BPOX (at 48.9 kDa) under Cd treatment and Fe deprivation (−Fe/+Cd) was higher after 5 days and lower after 10 days compared to the control (+Fe/−Cd), whereas under Fe supplementation expression was maintained at control levels (+Fe/−Cd) in Cd-treated plants. The level of PPO expression was similarly induced after 5 days and reduced after 10 days in Cd-treated plants under Fe deprivation, whereas the expression level recovered after Fe supplementation in Cd-treated plants.
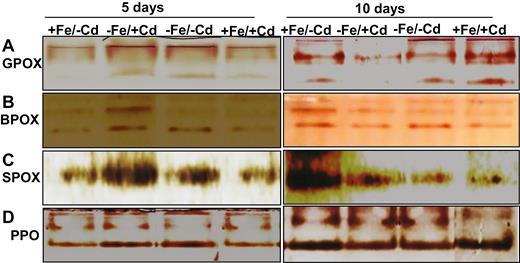
Active staining of peroxidase-associated enzymes: (A) guaiacol peroxidase (GPOX), (B) benzidine peroxidase (BPOX), (C) syringaldazine peroxidase (SPOX) and (D) polyphenol oxidase (PPO) in leaves as affected by four Fe/Cd combined treatments: adequate Fe with −Cd (+Fe/−Cd, control), Fe-deficient with Cd stress (−Fe/+Cd), Fe-deficient –in absence of Cd (−Fe/−Cd) and adequate Fe with Cd stress (+Fe/+Cd) for 10 days.
Relationship between Fe concentration and other descriptive parameters related to antioxidative pathways and peroxidases
Linear correlations between Fe concentration and other descriptive parameters related to antioxidative pathways and peroxidases were assessed using the values obtained from the four treatment groups (Table 1). The Fe concentration in leaves was closely correlated with GSH, GSSG, SPOX and PPO (P < 0.001) after 10 days of treatment. The correlation between Fe concentration and total chlorophyll and carotenoid was significant, with P < 0.01 at both 5 and 10 days of Fe/Cd treatment.
Linear correlations between Fe concentration and other descriptive parameters. The correlation analysis was done at 5 and 10 days affected by four Fe/Cd combined treatments: sufficient in Fe with −Cd (+Fe/−Cd, control), Fe-deficient with Cd stress (−Fe/+Cd), Fe-deficient but −Cd (−Fe/−Cd) and sufficient Fe with Cd stress (+Fe/+Cd). The correlation coefficient (r) and significant differences are given; n = 16. *P ≤ 0.05; **P ≤ 0.01; ***P ≤ 0.001.
Fe | Fresh biomass | Dry biomass | Chlorophyll | Carotenoid | ||||
5 days | Fe | – | r = 0.981*** | r = 0.960*** | r = 0.881** | r = 0.985*** | ||
10 days | Fe | – | r = 0.911** | r = 0.899** | r = 0.996*** | r = 0.943** | ||
Fe | SOD | CAT | APX | GR | GSH | GSSG | ||
5 days | Fe | – | r = 0.430* | r = 0.807** | r = 0.753** | r = 0.990*** | r = 0.831** | r = 0.356* |
10 days | Fe | – | r = 0.442* | r = 0.791** | r = 0.810** | r = 0.922** | r = 0.969*** | r = 0.975*** |
Fe | GPOX | BPOX | SPOX | PPO | ||||
5 days | Fe | – | r = 0.803** | r = 0.843** | r = 0.945*** | r = 0.956*** | ||
10 days | Fe | – | r = 0.731** | r = 0.783** | r = 0.978*** | r = 0.989*** |
Fe | Fresh biomass | Dry biomass | Chlorophyll | Carotenoid | ||||
5 days | Fe | – | r = 0.981*** | r = 0.960*** | r = 0.881** | r = 0.985*** | ||
10 days | Fe | – | r = 0.911** | r = 0.899** | r = 0.996*** | r = 0.943** | ||
Fe | SOD | CAT | APX | GR | GSH | GSSG | ||
5 days | Fe | – | r = 0.430* | r = 0.807** | r = 0.753** | r = 0.990*** | r = 0.831** | r = 0.356* |
10 days | Fe | – | r = 0.442* | r = 0.791** | r = 0.810** | r = 0.922** | r = 0.969*** | r = 0.975*** |
Fe | GPOX | BPOX | SPOX | PPO | ||||
5 days | Fe | – | r = 0.803** | r = 0.843** | r = 0.945*** | r = 0.956*** | ||
10 days | Fe | – | r = 0.731** | r = 0.783** | r = 0.978*** | r = 0.989*** |
Linear correlations between Fe concentration and other descriptive parameters. The correlation analysis was done at 5 and 10 days affected by four Fe/Cd combined treatments: sufficient in Fe with −Cd (+Fe/−Cd, control), Fe-deficient with Cd stress (−Fe/+Cd), Fe-deficient but −Cd (−Fe/−Cd) and sufficient Fe with Cd stress (+Fe/+Cd). The correlation coefficient (r) and significant differences are given; n = 16. *P ≤ 0.05; **P ≤ 0.01; ***P ≤ 0.001.
Fe | Fresh biomass | Dry biomass | Chlorophyll | Carotenoid | ||||
5 days | Fe | – | r = 0.981*** | r = 0.960*** | r = 0.881** | r = 0.985*** | ||
10 days | Fe | – | r = 0.911** | r = 0.899** | r = 0.996*** | r = 0.943** | ||
Fe | SOD | CAT | APX | GR | GSH | GSSG | ||
5 days | Fe | – | r = 0.430* | r = 0.807** | r = 0.753** | r = 0.990*** | r = 0.831** | r = 0.356* |
10 days | Fe | – | r = 0.442* | r = 0.791** | r = 0.810** | r = 0.922** | r = 0.969*** | r = 0.975*** |
Fe | GPOX | BPOX | SPOX | PPO | ||||
5 days | Fe | – | r = 0.803** | r = 0.843** | r = 0.945*** | r = 0.956*** | ||
10 days | Fe | – | r = 0.731** | r = 0.783** | r = 0.978*** | r = 0.989*** |
Fe | Fresh biomass | Dry biomass | Chlorophyll | Carotenoid | ||||
5 days | Fe | – | r = 0.981*** | r = 0.960*** | r = 0.881** | r = 0.985*** | ||
10 days | Fe | – | r = 0.911** | r = 0.899** | r = 0.996*** | r = 0.943** | ||
Fe | SOD | CAT | APX | GR | GSH | GSSG | ||
5 days | Fe | – | r = 0.430* | r = 0.807** | r = 0.753** | r = 0.990*** | r = 0.831** | r = 0.356* |
10 days | Fe | – | r = 0.442* | r = 0.791** | r = 0.810** | r = 0.922** | r = 0.969*** | r = 0.975*** |
Fe | GPOX | BPOX | SPOX | PPO | ||||
5 days | Fe | – | r = 0.803** | r = 0.843** | r = 0.945*** | r = 0.956*** | ||
10 days | Fe | – | r = 0.731** | r = 0.783** | r = 0.978*** | r = 0.989*** |
Discussion
Mungbean plants are leguminous plants that are a good model for studying Fe-nutritional strategies under metal toxicity, since they are the most sensitive legumes in response to Fe (Muneer et al. 2012, 2013). Several physiological responses to Fe deficiency (Mahmoudi et al. 2005; Andaluz et al. 2009; Jeong and Connolly 2009) like those of photosynthetic pigments (PSI and PSII) (Graziano and Lamittna 2005) in leguminous plants have been studied. However, little is known about the Fe–Cd interaction in leguminous plants, particularly in mungbean plants, one of the most commonly consumed foods worldwide (according to the FAO 2011). The present study was performed to understand the effect of Fe supplementation on redox reactions in Cd-treated plants. Initially, we observed the biomass of plants under four combined treatments and observed that shoot biomass decreased in Cd-treated plants. These results indicate that interactions between Cd and shoots lead to physiological modifications that in turn can affect the water balance, as already demonstrated in other studies (Muneer et al. 2012). We also observed that photosynthetic pigments (total chlorophyll and carotenoid content) were decreased in Cd-treated plants, whereas these negative effects were counteracted by the addition of Fe, suggesting that Fe supply could greatly enhance the growth of plants under Cd stress. A further observation was that the concentration of Fe decreased while Cd concentration increased to extreme levels in Fe-deficient and Cd-toxic plants (−Fe/+Cd) compared to the control, whereas, after Fe supplementation, the concentrations returned to control levels. Enhanced Fe accumulation is particularly important in relation to the need to sustain the PS biosynthetic pathway in Strategy II plants (Astolfi et al. 2010) and the reduction in ethylene production and almost total abolishment of expression of the nicotianamine synthetase gene (LeNAS) in the absence of Fe (Zuchi et al. 2009).
Cadmium is a reactive toxic metal that has a high affinity for functional groups of molecules such as phosphate, carboxyl, amino acid and thiol groups (Zheng et al. 2009). Our study showed that the non-protein thiol content in plants decreased over a prolonged period of Cd stress, whereas it increased in plants supplied with Fe. This indicates that Fe can counteract the negative effect of Cd stress. In our present study, we observed that mungbean leaves produced ROS in the form of TBARS and H2O2 under Fe deprivation in the presence or absence of Cd treatment (Fig. 2), as do many other plant species under other stress conditions (Lee et al. 2007; Muneer et al. 2014). However, in the presence of Fe in Cd-treated mungbean plants, TBARS and H2O2 levels were lower than in control plants. Oxidative damage has been considered one of the phytotoxic effects of ROS generation. Superoxide dismutase is one of the main enzymes in the ascorbate glutathione pathway, which dismutases the superoxide anion to H2O2 (Molassiotis et al. 2005). Our results show that SOD decreased 10 days after Fe deprivation with Cd treatment (Fig. 5A), which indicates that a lack of Fe fails to quench ROS production, possibly due to the low availability of cysteine and the failure or limitation of Fe chelates (Astolfi et al. 2010). However, supplementation of Fe (+Fe/+Cd) under Cd treatment increased SOD activity, which is consistent with previous findings (Muneer et al. 2012) showing that Fe is incorporated into the negative feedback of Cd treatment to maintain redox status.
Catalase is another important enzyme in the redox cycle and as a heme-containing compound, is likely to be affected by Fe deficiency (Ranieri et al. 2001; Shigeoka et al. 2002); thus, Fe deprivation with Cd toxicity (−Fe/+Cd) significantly decreased CAT (Fig. 3B). The reduction of CAT has been observed in many plants under various environmental stressors. Lee et al. (2009) observed that CAT increased under drought conditions in white clover leaves for the first 14 days, which suggests that CAT helps plants extinguish oxidative damage produced by ROS. The reduction of CAT might be due to the reduced expression of genes encoding for CAT (Qadir et al. 2004; Qureshi et al. 2007). However, in our study, CAT activity was increased by Fe supplementation in Cd-treated plants, which was consistent with our previous findings on the combined effect of Fe/Cd for 72 h on root nodules of mungbean plants (Muneer et al. 2012).
Another important aspect of this work concerns GR, which exists either in reduced or oxidized form. In this study, Cd treatment reduced GR activity (Fig. 5C) but Fe supplementation counteracted this effect. The reduction of GR activity might be due to the failure of the appearance of NADPH, which is required to reduce the Flavin nucleotides (NagaLakshmi and Prasad 2000), which then transfer their electrons to a disulphide bridge (-S-S-) in the enzyme. Meanwhile, the induction of GR activity in Fe-supplemented plants results in two sulfhydryl groups (-SH) that interact with GSSG and reduce it to GSH (Qureshi et al. 2007). Ascorbate peroxidase activity was reduced under Fe deprivation (Fig. 5D) and was further reduced by Cd treatment (−Fe/+Cd). Ascorbate peroxidase activity has been found to be reduced in the apoplastic fluid as well as at the intracellular level. The reduced APX activity may result from the high demand for iron from the APX molecule as it contains a no-heme iron atom in addition to the heme group (Grill et al. 2001). However, the presence of Fe (+Fe/+Cd) under Cd treatment increased APX activity, which might be attributed to the limited heme-catalysed damage to the APX protein.
Glutathione acts as an essential non-enzymatic antioxidant that plays multiple roles in plant metabolism (Grill et al. 2001). The reduced (GSH) and oxidized (GSSG) forms of glutathione were reduced in the absence of Fe under Cd treatment (Fig. 6), but were increased by Fe supplementation (+Fe/+Cd). The oxidized and reduced forms of glutathione (GSH + GSSG) have been well documented in different species under various environmental conditions, and it has been reported that glutathione acts as a marker for oxidative damage (Anjum et al. 2013). An increment in GSH content was also observed in Scendesmus bijugatus in response to copper stress (NagaLakshmi and Prasad 2000), which indicated that glutathione acts as an antioxidative defence that plays a key role in acclimatizing the plant to environmental stress. The production of ROS is a universal redox-sensing mechanism operating at the cellular level (Noctor et al. 2002) that is detoxified by ascorbate-glutathione cycle enzymes; however, our results showed that in the absence of Fe, glutathione enzyme did not control the pathway in Cd-treated plants. This implies that supply of Fe could help mitigate Cd toxicity by keeping ROS under control and redox reactions active.
Peroxidase isozymes contribute to cell wall properties by catalysing covalent cross-links after oxidation of ester and phenolic compounds (Liyma et al. 1994). These isozymes were induced for the first 7 days and were highly expressed after 14 days of drought stress in white clover leaves (Lee et al. 2007). Guaiacol peroxidase, BPOX, SPOX and PPO are lignified enzymes that play a role in the antioxidative cycle. These enzymes mainly catalyse coumaryl, coniferyl and sinapyl (Boudet 1992). After 5 days of Fe deprivation with Cd toxicity (−Fe/+Cd), GPOX, BPOX, SPOX and PPO activity increased (Fig. 7) with a concomitant increase in peroxide staining (Fig. 8). The increased peroxidase activity could alter the structure of cell wall, which in turn could be correlated with adaptation to Fe deficiency (Cervilla et al. 2009). But 10 days after Fe deprivation in Cd-treated plants, the levels of these peroxidases were reduced and there was a lower level of active staining, whereas Fe supplementation reversed the negative effect by increasing the activity and expression of peroxidases. The observed reduction in activity of these isozymes could reflect the modification of cell wall and phenolic compounds present in the cell and strongly suggests that the presence of Fe reduces the induction of these peroxidases and thus reduces oxidative damage. From these studies we conclude that mungbean plants decrease the redox reactions under Cd treatment in Fe-deficient plants (for overall conclusions, please see Fig. 9), whereas supply of Fe ameliorates these negative effects by increasing redox reactions even in the presence of Cd.
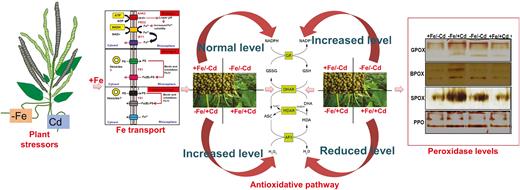
Diagrammatic representation of the present study for the transport of iron, and mungbean affected by four Fe/Cd combined treatments: adequate Fe with −Cd (+Fe/−Cd, control), Fe-deficient with Cd stress (−Fe/+Cd), Fe-deficient but −Cd (−Fe/−Cd) and adequate Fe with Cd stress (+Fe/+Cd) days. The diagram represents how iron is transported to plants and how combined treatments of iron and cadmium interact with each other. Overall, the representation is about how iron helps mungbean to mitigate/alleviate ROS produced in response to cadmium stress.
Source of Funding
Funded by VIT SEED GRANT.
Contribution of Authors
S.M. designed the work and experiments, S.M. and K.B. performed the experiments, D.K.T. helped in statistical analysis of the data and helpful discussions. J.H.L. helped in the isozyme analysis. K.B. and S.M. wrote the paper. S.M. edited and finalized the manuscript.
Conflict of interest
None declared.
Acknowledgments
The authors are thankful to VIT for providing seed grant to corresponding author to carry out the work.
Comments