-
PDF
- Split View
-
Views
-
Cite
Cite
Adriana Aranda-Rickert, Carolina Rothen, Patricia Diez, Ana María González, Brigitte Marazzi, Sugary secretions of wasp galls: a want-to-be extrafloral nectar?, Annals of Botany, Volume 120, Issue 5, November 2017, Pages 765–774, https://doi.org/10.1093/aob/mcx075
- Share Icon Share
Abstract
Background and Aims The most widespread form of protective mutualisms is represented by plants bearing extrafloral nectaries (EFNs) that attract ants and other arthropods for indirect defence. Another, but less common, form of sugary secretion for indirect defence occurs in galls induced by cynipid wasps. Until now, such galls have been reported only for cynipid wasps that infest oak trees in the northern hemisphere. This study provides the first evidence of galls that exude sugary secretions in the southern hemisphere and asks whether they can be considered as analogues of plants’ EFNs.
Methods The ecology and anatomy of galls and the chemical composition of the secretion were investigated in north-western Argentina, in natural populations of the host trees Prosopis chilensis and P. flexuosa. To examine whether ants protect the galls from natural enemies, ant exclusion experiments were conducted in the field.
Key Results The galls produce large amounts of sucrose-rich, nectar-like secretions. No typical nectary and sub-nectary parenchymatic tissues or secretory trichomes can be observed; instead there is a dense vascularization with phloem elements reaching the gall periphery. At least six species of ants, but also vespid wasps, Diptera and Coleoptera, consumed the gall secretions. The ant exclusion experiment showed that when ants tended galls, no differences were found in the rate of successful emergence of gall wasps or in the rate of parasitism and inquiline infestation compared with ant-excluded galls.
Conclusions The gall sugary secretion is not analogous to extrafloral nectar because no nectar-producing structure is associated with it, but is functionally equivalent to arthropod honeydew because it provides indirect defence to the plant parasite. As in other facultative mutualisms mediated by sugary secretions, the gall secretion triggers a complex multispecies interaction, in which the outcome of individual pair-wise interactions depends on the ecological context in which they take place.
INTRODUCTION
Most protective mutualisms are based on interactions mediated by carbohydrate-rich food supplies in the form of nectar, honeydew and sugary secretions (Rico-Gray and Oliveira, 2007). Nectar is secreted by extrafloral nectaries (hereafter EFNs) in many plants (Koptur, 1992; Marazzi et al., 2013b), while honeydew and sugary secretions are excreted by hemipteran insects and lepidopteran larvae (Way, 1963; Pierce et al., 2002), respectively. In both cases, these sources of high-energy food attract aggressive arthropods for indirect defence against natural enemies (see Rico-Gray and Oliveira, 2007, and references therein). Omnivorous ants are the most common partners involved in this kind of interaction, because of their aggression and abundance (Heil and McKey, 2003). The independent evolution of more or less sophisticated structures producing nectar and honeydew for ant guarding has occurred in a vast number of lineages and even kingdoms, and represents a widespread phenomenon in nature (e.g. Wäckers, 2005; Weber and Keeler, 2012).
Another, but less common, form of sugary secretion that attracts aggressive partners occurs in galls induced by cynipid wasps (Hymenoptera: Cynipidae; genera Andricus, Disholcaspis and Dryocosmus) from the temperate regions in the northern hemisphere that use oaks (Quercus, Fagaceae) as host trees (e.g. Bequaert, 1924; Washburn, 1984; Seibert, 1993; Stone and Cook, 1998; Fernandes et al., 1999). Galls consist of modified host plant tissues that provide food and shelter for the developing larvae inside, which, in turn, control the development and morphology of the gall (Cornell, 1983; Price et al., 1987; Stone and Schönrogge, 2003) and have a negative impact on the host plant’s growth and/or fitness (Weis and Kapelinski, 1984). Each species of cynipid wasp, also known as oak gall wasps, attacks one or several closely related oak species (Stone and Cook, 1998; Nieves-Aldrey, 2001). Oak trees lack floral and extrafloral nectar, and several studies have demonstrated that ants consuming the sugary gall secretion reduce mortality of the gall wasp larvae due to parasitoid wasp attack (Washburn, 1984; Fernandes et al., 1999; Stone et al., 2002; Inouye and Agrawal, 2004) and to inquiline intrusion (Seibert, 1993). Inquilines are obligate or facultative inhabitants of galls, and because they feed partially or entirely on the gall tissues, they negatively affect cynipid wasp survival by competing for the same microenvironment and resources meant for development of the cynipid larvae (Seibert, 1993). Parasitoid wasps can also attack these inquilines (Washburn and Cornell, 1981; Wiebes-Rijks and Shorthouse, 1992).
Galls secreting a sugary liquid have so far been reported only for those induced on oaks by cynipid (and rarely cecidomyid) wasps of the northern hemisphere (notably by Bequaert, 1924). In the southern hemisphere, three cynipid gall wasp species of the genus Eschatocerus, all endemic to southern South America, have been described as gall inducers on species of the legume genera Prosopis and Vachellia (formerly Acacia) (Ronquist et al., 2015), known to bear EFNs visited by nectar-consuming ants (Aranda-Rickert et al., 2014). In particular, several species of these genera have recently been documented as host plants of E. acaciae, but the induced galls lack sugary secretions and the ants as well (Nieves-Aldrey and San Blas, 2015).
Unlike the ecology of the cynipid wasp–host plant interaction, the structure of these galls is less well understood. Cynipid galls generally develop on terminal branches of the host plant, are sub-spherical or ovoidal, up to 35 mm large, spongy when developing and hardening when mature (Diaz and de Santis, 1975). Each gall is plurilocular, enclosing many larval ellipsoidal cells disposed radially. However, no study has identified – to our knowledge – the secretory structure producing such nectar-like liquid on the gall. In fact, studies mentioning these secreting galls (Bequaert, 1924; Washburn, 1984; Seibert, 1993, and citations therein) refer to them interchangeably as ‘nectar-secreting’ and ‘honeydew-secreting’, but provide no histological analysis confirming the presence of any nectary tissues.
In this study, we ask whether nectar-secreting galls can be considered as analogues of plants’ EFNs by investigating the anatomy and ecology of the first galls found to exude a sugary secretion in the southern hemisphere. That is, we focused on the study system involving the South American cynipid wasp E. acaciae, the legume hosts P. chilensis (Mol.) Stuntz and P. flexuosa DC, and several ant and non-ant consumers of the gall nectar-like secretion. Specifically, first, we determined the volume and chemical composition of the nectar-like gall secretion and identified and anatomically characterized the structure producing this secretion. Secondly, we followed the entire gall life cycle and described the interaction between nectar consumers and gall wasps by surveying consumers and their behaviour in their natural habitat. Finally, we carried out an exclusion experiment to assess whether the ants and/or other nectar consumers protect gall wasps against their natural enemies, the inquilines and the parasitoids.
MATERIALS AND METHODS
Study system
We conducted this study between August 2015 and April 2016 in undisturbed natural sites around Anillaco, La Rioja, Northwest Argentina (28º48′S, 66º56′W, altitude around 1400 m a.s.l.). Climate is semi-arid to arid (mean annual temperature of 16·6 °C; mean annual precipitation of 272 mm, Anillaco Meteorological Station, series from 1999 to 2012) and is markedly seasonal, with a relatively long dry season from May to November (winter–spring) and a wet season from December to April (summer–autumn). The sites are within the Monte Desert biome, a shrubby steppe in which Prosopis species are important vegetation elements (Abraham et al., 2009). At our study sites, P. chilensis is the most common tree, followed by P. flexuosa (Aranda-Rickert, 2014). They are 3–10 m tall and characterize the xeroriparian forests along desert washes.
Prosopis chilensis and P. flexuosa bear EFNs on their petiole and rachis between leaflet pairs (Vilela and Palacios, 1997) that are visited by nectar-consuming ants (Aranda-Rickert et al., 2014). They are also well documented as host plants of the gall-inducing wasp E. acaciae, along with Vachellia aroma (Gillies ex Hook. & Arn.) Seigler & Ebinger, V. caven (Molina) Seigler & Ebinger, P. alba Griseb. and P. nigra Hieron. (Nieves-Aldrey and San Blas, 2015).
Field work and data collection
Field observations and gall collection were done from early spring (September) 2015 to early autumn (March) 2016 on different populations of wild P. chilensis and P. flexuosa trees. Active secreting galls at different developmental stages were collected and fixed in formaldehyde–acetic acid–alcohol (FAA) 50 % for 48 h, and then processed for anatomical studies. A voucher of each species was deposited at the herbarium Carmen L. Cristóbal [CTES], Corrientes, Argentina. Gall presence was also verified in other potential host legume species. We noted the presence and number of galls per tree during mid spring (October), which is when galls were completely developed and actively secreting the nectar-like secretion. Because we noted no differences in gall infestation between the two Prosopis species, we focused our next studies on individuals of the more abundant P. chilensis.
Secretion quantification and sugar composition
Secretion volume and chemical composition were determined on ten healthy, active secreting galls from five trees (two galls per individual). The galls were first cleaned with distilled water and a cotton swab and isolated from nectar-consumer insects by a cover mesh to allow accumulation of secretion. After 24 h, the bagged galls were harvested by cutting the branch. One of each pair was used to determine the secretion solute concentration immediately after collection, and the other to determine the sugar composition. Due to its high viscosity, it was not possible to collect and measure secretion volumes using graduated capillaries. The total secretion volume per gall was thus first inferred as the sum of the volume of each secretion drop estimated using the radius measured under binocular lens and a classic formula for the volume of a sphere or hemi-sphere. Afterwards, we removed all the secretion from the gall surface using the tip of sterilized needles and used it for chemical analyses. Finally, we measured the gall size of the collected galls as the diameter of the longest axis with a digital calliper.
Total sugar concentration was determined by immediately expelling the droplets onto the prismatic surface of a temperature-compensating refractometer (0–95 %; Leica ABBE Mark II) with sugar scales based on percentage (w/w) sucrose in water. The sugar composition of the second set of secretion samples was analysed separately for each gall using high-performance liquid chromatography (HPLC). The samples were vacuum centrifuged, diluted in ultra-pure water, filtered (0·22 μm pores) and degassed (TestLab Ultrasonic Cleaner). Sugar detection was performed on a high-pressure liquid chromatograph (Shimadzu Co., Japan) equipped with LC-20AT liquid chromatograph pumps; CTO-10AVP column thermostat, RID-20A refractometer, MetaCarb 87C, 300 × 7·8 mm chromatograph column (Agilent Technologies) and LabSolutions v. 5.71 software. The amount of the sample injected onto the column was 20 μL. The separation was conducted at 85 °C with the mobile phase at a flow rate of 0·5 mL min–1. Individual sugars in the nectar were identified by comparing the retention times with those of standards. The resulting peaks were used as an approach to estimate the relative content of each sugar component. The mean ± s.e. of five samples was calculated.
Anatomical studies
For anatomical studies, FAA-fixed gall material was dehydrated in an ethanol solution series, embedded in paraffin (Johansen, 1940) and sectioned transversally and longitudinally (12 μm thick) with a rotary microtome (Leica RM 2165). Sections were stained following two separate procedures: either with brilliant cresyl blue or with a safranin–fast green combination (Johansen, 1940), mounted using synthetic Canada balsam and, finally, examined with a Leica DMLB light microscope, and a digital camera LEICA ICC50HD. For gall surface analysis with scanning electron microscopy (SEM), the FAA-fixed material was dehydrated in an ascending acetone series, dried at critical point with CO2 (Denton Vacuum LLC, DCP-1) and sputtered with palladium–gold (Denton Vacuum, Desk II). Samples were examined using a Jeol LV5800 SEM of the SEM-Service of the Universidad Nacional del Nordeste (Corrientes, Argentina).
Survey of gall secretion consumers
For surveys, a total of 20 trees and 69 galls were selected. We counted the number of ants and non-ant nectar consumers on each gall during a 1 min interval (thus avoiding counting the same individual twice) and noted their behaviour. Surveys were carried out between 10·00 and 16·00 h, which correspond to the highest ant activity and diversity during this time of the year in our study region (Aranda-Rickert et al., 2014). When necessary, insects were collected and fixed in alcohol for taxonomic identification.
Exclusion experiments
In late winter (August) 2015, before galls appeared on trees, we selected 15 P. chilensis trees, separated by at least 10 m, for the exclusion experiment. Each experimental tree harboured between 40 and 60 galls, thus avoiding those trees that were heavily infected (>100 galls per tree) as well as those with only a handful of galls. At the beginning of spring (September), the marked trees were examined every 2 d for the eruption of new galls through the epidermis of young twigs. Once the galls were visible, for each tree we tagged two galls in different twigs with a band of masking tape that was wrapped around the supporting branches and we randomly assigned one twig as control (ant-access) and the other as treatment (ant-exclusion) (n = 15 galls per treatment). To prevent access by ants to the treatment galls, we applied on the tape a 4 cm ring of a sticky and non-toxic resin (Hormigel®). Foliar bridges to adjacent, untreated branches were clipped throughout the experiment. Except for marking, controls were left undisturbed. Potential parasitoids, inquilines and nectar consumers were collected by suspending two yellow-painted sticky traps in the foliage of each experimental tree. The traps were collected and replaced weekly, from gall appearance until wasp emergence.
The galls on each experimental branch were examined for the presence of ants and non-ant nectar consumers every week, between 10·00 and 16·00 h, for a total of eight censuses (120 observations on 15 galls per treatment from September to November). Each gall observation lasted 2 min, where we noted species, number of individuals and their behaviour. This procedure continued until we noted that galls hardened and nectar secretion stopped (early summer). By this time, the galls were harvested and maintained individually in plastic boxes, covered with a mesh with a base of regularly humidified plaster, and kept indoors at room temperature. Emergence was recorded once a week, and all emerged arthropod fauna were counted and taxonomically identified. When no more emergences were registered (August 2016), the galls were cut open and examined under a binocular lens to verify that no individual was still inside.
To test for any differences in ant and non-ant gall nectar consumer densities (number of individuals per gall census) between ant-access and ant-exclusion treatments we used a one-way analysis of vaiance (ANOVA). The differences in the emergence success of gall wasps and the rates of parasitism and inquiline attack were analysed using paired t-test, in which we test the null hypothesis that the frequencies (the number of galls in which at least one individual of each group emerged) are equal between the matched pairs of experimental stems (ant-exclusion and ant-access). Variables were square root transformed to meet assumptions of the analyses when the data did not satisfy the normality and homogeneity of variances assumptions. All analyses were done with Statistica (StatSoft, Tulsa, OK, USA).
RESULTS
Gall presence and life cycle
Galls were found exclusively on mature individuals (i.e. > 3 m in height) of P. chilensis and P. flexuosa trees. They were not present on the other Prosopis species (P. torquata) or on the acacias [Vachellia aroma, V. caven and Senegalia gilliesii (Steud.) Seigler & Ebinger], also found in the study area. In general, the pattern of geographical distribution of Prosopis trees with galls was highly patchy. In some localities, trees lacked galls, while in others almost all contiguous trees were bearing them, with numbers ranging from few galls per tree (i.e. up to ten) to overinfestation (i.e. >100).
Galls of E. acaciae began to erupt in early spring (September) and always on the distal portion of the twigs of the host trees. Gall development followed leaf and flower development. The galls were mostly found solitary and sometimes in clusters of two to three galls. Nectar-like secretion was produced from gall eruption until the gall wasps emerged and galls started to lignify (early summer), for a total of 3 months (September, October and November) (see Fig. 1 for a description of the gall life cycle).
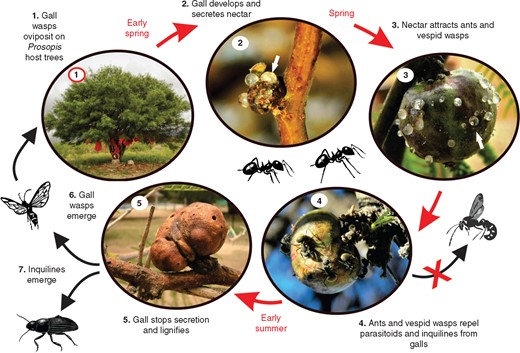
Life cycle of Eschatocerus acaciae galls on Prosopis sp. trees and their interaction with nectar consumers and natural enemies in La Rioja, Argentina. White arrows show the sugary secretions of the galls.
Secretion quantification and sugar composition
The secretion accumulated in the form of droplets at 21–34 points randomly dispersed across the gall surface (mean diameter of the examined galls ± s.e. = 26·9 ± 2·1 mm, n = 10). Gall produced individually 15·3–61·9 μL of extremely viscous secretion in 24 h. Sugar concentration in the secretion was 73·1 ± 1·48 % (mean ± s.e., n = 5). It was composed mainly of sucrose (94·14 ± 2·74 %) whereas there were only small quantities of glucose (2·01 ± 0·85 %), fructose (0·9 ± 0·8 %) and other oligosaccharides (2·96 ± 1·19 %) (mean ± s.e.).
Anatomical studies
The gall’s general anatomical structure is relatively simple, consisting of an epidermis lacking stomata and trichomes and an inner, highly vascularized ground matrix. The epidermis is unistratified, covered by a slightly thickened cuticle (Fig. 2A). In fully developed galls, the epidermis cells are elongated, parallel to the surface, possibly because their cell division does not accompany the fast development of galls (i.e. as size compensation of the increasing gall) (Fig. 2B).
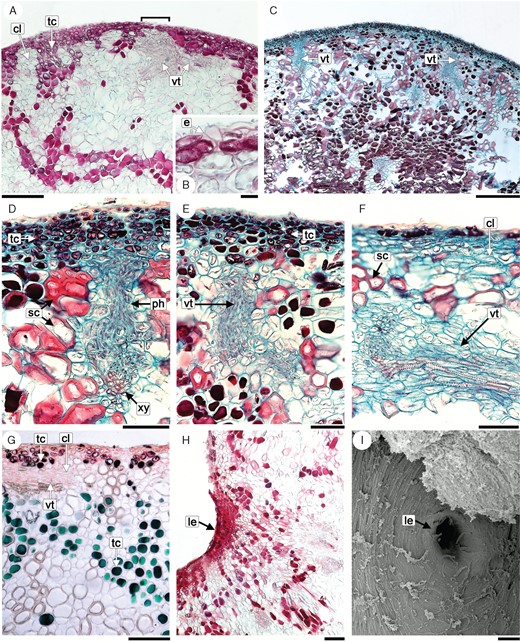
Anatomical studies of Eschatocerus acaciae galls. (A) Transverse section of young gall, note the vascular bundle near the epidermis. (B) Details of zone marked in (A), showing interruptions of epidermis. (C) Transverse section of adult gall with several vascular tissues besides sclereids and tanniferous cells. (D–F) Detail of several vascular bundles reaching the sub-dermal tanniferous tissues; (A–F, H) safranin–fast green. (G) Transverse section of young gall stained with cresyl blue; (I, H) transverse section thought a lenticel, (I) superficial view of lenticel (SEM). Abbreviations: cl, chlorenchyma cell; e, epidermis; le, lenticels; ph, phloem; sc, sclereids; tc, tannifeous cells; vt, vascular tissues; xy, xylem. Scale bars = A, C: 200 μm, B: 20 μm, D–I: 100 μm.
The ground matrix of young galls consists of parenchyma cells with patches of tanniferous cells (Fig. 2A). Tanniferous cells are characterized by the intense staining which was dark red (safranin) and green (cresyl blue). Below the epidermis, a few layers of chlorenchyma alternate with these patches. In fully developed galls, an increase of tannin content in the parenchyma is observed. In addition, numerous scattered parenchyma cells become differentiated into sclereids (46 × 116 μm). Secondary walls of macrosclereids are 7·9–9·0 μm thick, composed of distinctive layers and numerous broad simple pits (Fig 2D–F).
Mixed within the ground matrix is a dense, irregular network of vascular bundles, not organized in a eustele. Vascular bundles are collateral, with procambium as a meristem. The xylem vessels are annularly or helically thickened. The phloem is associated with abundant parenchyma. The vascularization is found throughout the gall, but vascular bundles are especially abundant in the periphery. The smaller vascular traces reach up to 4–6 cell layers below the epidermis, where they mainly consist of phloem, and where the epidermis cells are smaller and the sub-epidermis consists of fewer cell layers (Fig. 2D–F).
No secretory tissue or secretory cells are present at any structural level of the gall (i.e. no secretory stomata, glandular trichomes, nectary parenchyma or sub-nectary parenchyma). The hole-shaped depressions observed on the galls’ surface are not secretory; the tissue below consists of compact layers of small and packed cells rich in tanniferous content (Fig. 2H, I).
Gall secretion consumers
The vast majority of inspected galls (92·7 % of 69) were tended by a guild of nectar consumer arthropods composed of ants (three subfamilies and six species), predatory wasps (three species), Diptera (three species) and Coleoptera (one species) (Table 1). On average, we observed 1·87 ± 0·15 (± s.e., n = 69) arthropods on each gall. Ants were by far the most frequent and abundant gall nectar consumers. They were on approx. 84 % of the galls and represented 86 % of the total arthropod individuals. Predatory wasps and Diptera flies were observed with almost equal low frequency (wasps, 13·04 %; Diptera, 10·14 % of the inspected galls) and number (wasps, 7·75 %; Diptera, 6·2 % of total arthropod individuals); the Coleoptera (a ladybird beetle) was seen only once.
Order, Family . | Species . | Frequency . | Abundance . |
---|---|---|---|
Hymenoptera, Formicidae | Subfamily Formicinae | ||
Camponotus blandus Smith | 2 | 8 | |
Camponotus mus Roger | 51 | 84 | |
Subfamily Myrmicinae | |||
Cephalotes liogaster Santschi | 2 | 6 | |
Crematogaster rochai Forel | 1 | 5 | |
Solenopsis sp. | 1 | 6 | |
Subfamily Pseudomyrmicinae | |||
Pseudomyrmex denticollis Emery | 1 | 1 | |
Hymenoptera, Vespidae | Polybia scutellaris White | 6 | 7 |
Polistes cavapyta Saussure | 2 | 2 | |
Hymenoptera, Sphecidae | Prionyx sp. | 1 | 1 |
Diptera, Muscidae | Musca domestica Linnaeus | 3 | 3 |
Morellia sp. | 4 | 4 | |
Dolichophaonia sp. | 3 | 1 | |
Coleoptera, Coccinelidae | Zagreus sp. | 1 | 1 |
Order, Family . | Species . | Frequency . | Abundance . |
---|---|---|---|
Hymenoptera, Formicidae | Subfamily Formicinae | ||
Camponotus blandus Smith | 2 | 8 | |
Camponotus mus Roger | 51 | 84 | |
Subfamily Myrmicinae | |||
Cephalotes liogaster Santschi | 2 | 6 | |
Crematogaster rochai Forel | 1 | 5 | |
Solenopsis sp. | 1 | 6 | |
Subfamily Pseudomyrmicinae | |||
Pseudomyrmex denticollis Emery | 1 | 1 | |
Hymenoptera, Vespidae | Polybia scutellaris White | 6 | 7 |
Polistes cavapyta Saussure | 2 | 2 | |
Hymenoptera, Sphecidae | Prionyx sp. | 1 | 1 |
Diptera, Muscidae | Musca domestica Linnaeus | 3 | 3 |
Morellia sp. | 4 | 4 | |
Dolichophaonia sp. | 3 | 1 | |
Coleoptera, Coccinelidae | Zagreus sp. | 1 | 1 |
Frequencies are the number of spatially independent occurrences (number of galls in which the interaction was observed), and abundances are the total number of individuals of each taxon.
Data correspond to 69 galls on 20 Prosopis chilensis trees surveyed once during October 2016 in La Rioja, Argentina.
Order, Family . | Species . | Frequency . | Abundance . |
---|---|---|---|
Hymenoptera, Formicidae | Subfamily Formicinae | ||
Camponotus blandus Smith | 2 | 8 | |
Camponotus mus Roger | 51 | 84 | |
Subfamily Myrmicinae | |||
Cephalotes liogaster Santschi | 2 | 6 | |
Crematogaster rochai Forel | 1 | 5 | |
Solenopsis sp. | 1 | 6 | |
Subfamily Pseudomyrmicinae | |||
Pseudomyrmex denticollis Emery | 1 | 1 | |
Hymenoptera, Vespidae | Polybia scutellaris White | 6 | 7 |
Polistes cavapyta Saussure | 2 | 2 | |
Hymenoptera, Sphecidae | Prionyx sp. | 1 | 1 |
Diptera, Muscidae | Musca domestica Linnaeus | 3 | 3 |
Morellia sp. | 4 | 4 | |
Dolichophaonia sp. | 3 | 1 | |
Coleoptera, Coccinelidae | Zagreus sp. | 1 | 1 |
Order, Family . | Species . | Frequency . | Abundance . |
---|---|---|---|
Hymenoptera, Formicidae | Subfamily Formicinae | ||
Camponotus blandus Smith | 2 | 8 | |
Camponotus mus Roger | 51 | 84 | |
Subfamily Myrmicinae | |||
Cephalotes liogaster Santschi | 2 | 6 | |
Crematogaster rochai Forel | 1 | 5 | |
Solenopsis sp. | 1 | 6 | |
Subfamily Pseudomyrmicinae | |||
Pseudomyrmex denticollis Emery | 1 | 1 | |
Hymenoptera, Vespidae | Polybia scutellaris White | 6 | 7 |
Polistes cavapyta Saussure | 2 | 2 | |
Hymenoptera, Sphecidae | Prionyx sp. | 1 | 1 |
Diptera, Muscidae | Musca domestica Linnaeus | 3 | 3 |
Morellia sp. | 4 | 4 | |
Dolichophaonia sp. | 3 | 1 | |
Coleoptera, Coccinelidae | Zagreus sp. | 1 | 1 |
Frequencies are the number of spatially independent occurrences (number of galls in which the interaction was observed), and abundances are the total number of individuals of each taxon.
Data correspond to 69 galls on 20 Prosopis chilensis trees surveyed once during October 2016 in La Rioja, Argentina.
Ants and predatory, nectar-consuming wasps were mutually exclusive, and ants showed the most aggressive behaviour, repelling all other arthropods that tried to feed on the secretion (Supplementary Data Video S1). The most common ant species was Camponotus mus Roger, found on 73·9 % of the examined galls and with 75·67 % of the total individual ants counted. We found that this ant species nested inside the trunk of some of the host trees (and inside old and dried galls; see later). The cessation of nectar production and ant and other arthropod attendance coincided with the complete development of the gall wasp larvae and their subsequent emergence.
Exclusion experiment
A total of 249 ant individuals, all belonging to C. mus, were counted overall on ant-access galls, against only three individuals on ant-excluded treatments (ANOVA F1,238 = 191·58, P < 0·0001). However, on branches from which ants were excluded, two species of vespid wasps (Polybia scutellaris White and Polistes cavapyta Saussure) completely replaced the ants and dominated the nectar resource. In total, 107 vespid wasps (both species combined) were counted on ant-excluded galls, against 13 on ant-access treatments (ANOVA F1,239 = 107·93, P < 0·0001). Ant and wasp attendance on the ant-access and ant-excluded galls, respectively, was constant, and confrontations were observed whenever both taxa happened to meet on the same gall; usually the ants deterred the wasp. A third group of nectar consumers consisted of three species of Diptera, though they were comparatively less numerous (ant-access = 25 vs. ant-excluded = 21 individuals) and only sporadically present, with no significant differences in their densities between treatments (ANOVA F1,239 = 0·22, P = 0·63). Indeed, these flies were fast enough to avoid ant and wasp attacks, but they could stay on the galls and consume nectar only for short periods of time.
At the end of the experiment, two galls from one of the experimental trees were eaten by goats; therefore, our final sample size was lowered to 14 galls per treatment. The galls hosted four parasitoid wasp species (Hymenoptera, Superfamily Chalcidoidea) of four families (Torymidae Eulophidae, Eurytomidae and Eupelmidae). They all emerged together with both male and female E. acaciae individuals (early summer). Additional species of at least four orders (Hymenoptera, Coleoptera, Lepidoptera and Diptera) were reared from the galls as long as 8 months after E. acaciae emergence. These late emerged Hymenopteran species included Tanaostigmodes coeruleus Kieffer & Joergensen (Chalcidoidea, Tanaostigmatidae), one species of Bethylidae (Superfamily Chrysidoideae) and one species of Ichneunomidae (Superfamily Ichneumonoidea), and were always found together with the Coleoptera, Diptera and Lepidoptera larvae. By this time, the galls were completely lignified and dry, with their internal tissues almost entirely fed out and full with the inquilines’ larval excrements. We also found C. mus ants inside some of the dried galls, apparently using them as some kind of satellite nests consisting of only workers.
The emergence success of the gall wasp E. acaciae showed no significant differences when ants tended galls compared with when they were excluded (paired t-test, t = –2·09, n = 14, P = 0·057). Similarly, the exclusion protocol did not significantly affect the rate of parasitism (t = –0·43, P = 0·47) or the rate of inquiline attack (t = 1·3, P = 0·21; n = 14). Also, when each family of parasitoid wasps was analysed separately, no significant differences between treatments were found (Torymidae, t 0·81, P = 0·43; Eulophydae, t = –0·43, P = 0·67; Eurytomidae, t = 0·37, P = 0·72; Eupelmidae, t = –1·47, P = 0·16, n = 14).
DISCUSSION
‘The oak bears still another hairy gall, moreover without use, which secretes in the spring a juice resembling honey in taste as well as in touch’ (Theophrastus, c. 300 BC). Washburn (1984) cites this ancient phrase in his publication to present the first empirical demonstration of a mutualism between cynipid gall wasps and ants mediated by a nectar-like gall secretion in the northern hemisphere. No other similar mutualism has been reported until now. Over 30 years after Washburn’s first work, we report a new case of galls secreting a nectar-like reward for indirect defence. It is a system from the southern hemisphere that involves a different host tree species and cynipid wasp genus. Furthermore, this is the first study that anatomically explores such galls and identifies the possible source of the nectar-like secretion, discussing the implications for our understanding of such systems.
Gall anatomy and nectar-like secretion
The general anatomy of cynipid galls on Prosopis corresponds to that of other galls. This similarity can be summarized in five aspects: (1) the proliferation of the parenchyma (i.e. hyperplasia) forming the ground matrix of the gall (e.g. Krishnamurthy et al., 1977; Raman, 2011); (2) the presence of tanniferous substances in cells (e.g. Taper and Case, 1987; Gonzalez and Solis, 2015); (3) the presence of sclereids (Meyer and Maresquelle, 1983; Stone and Schönrogge, 2003); (4) a dense and almost excessive vascularization (Isaias, 1998); and (5) the lenticel-like structures (i.e. the hole-shaped depressions) on the gall surface, also described from leaf intumescences of some Eucalyptus species (Pinkard et al., 2006) and the epidermis of mature edible fleshy fruits (Sterling, 1953).
Bequaert (1924) is the first to compile a list of what he interchangeably calls the ‘honey’- and ‘honeydew-secreting’ galls, using the word ‘nectaries [found at the surface of galls]’ (p. 102, p. 107 and p. 109, respectively) but admitting that their anatomy and physiology had not been studied. Unfortunately, subsequent ecological work on these galls (e.g. Felt, 1940; Cornell, 1983; Washburn, 1984; Seibert, 1993) continued to cite Bequaert but without providing anatomical analyses. Because our study shows that there is no nectary structure in Prosopis cynipid galls, we use the term nectar-like, whereas nectar should be used exclusively for nectaries (see Nicolson et al., 2007; Escalante-Pérez and Heil, 2012).
We hypothesize that this liquid is a mix of water from xylem and sugars from the phloem deriving from the many vascular traces reaching the epidermal area (Fig. 2), similar to those usually described in true nectaries (see Escalante-Pérez and Heil, 2012). Another way in which plants can secrete nectar-like liquids are wounds of plant tissues by herbivores (Koptur, 1984; Lortzing et al., 2016). Among (floral and extrafloral) nectaries, the most similar to the gall secretory system are those nectaries that lack any externally visible structure, but their secreting tissue (embedded within the bearing organ) can be identified with anatomical studies (e.g. non-structural nectaries, e.g. Diaz-Castelazo et al., 2005; Nepi, 2007; non-individualized EFNs, Marazzi et al., 2013a).
Gall ecology
Galls create a microhabitat for the gall wasp progeny that is also exploited by other arthropods (Price et al., 1987). In our study, the diversity of such gall inquilines and other intruders included four Chalcidoidea species that have been reported elsewhere as common and obligatory parasitoids of cynipid gall wasps (Grissell and Schauff, 1990; Inouye and Agrawal, 2004; Medianero and Nieves-Aldrey, 2011; Nieves-Aldrey and San Blas, 2015). The other hymenopteran species that were reared from the galls and emerged later, after the gall wasp emergence, can be regarded as casual or accidental members of the gall community, probably finding their hosts among the insects that use cynipid galls facultatively as shelters for their larvae (Lepidoptera, Coleoptera and Diptera). The Chalcidoidea genera Tanaostigmodes and some Ichneumonidae species have also been reported as inquilines in cynipid galls (La Salle, 2005; Medianero and Nieves-Aldrey, 2011).
Because of the high diversity of inquilines and other intruders, the avoidance of attack by natural enemies is generally considered as a stronger selection pressure acting on the evolution of galls than any environmental stress (reviewed by Stone et al., 2002). However, only the presence of a nectar-like secretion has been experimentally demonstrated to act as a defensive strategy against inquilines and parasitoids of galls (Washburn, 1984; Seibert, 1993; Fernandes et al., 1999; Inouye and Agrawal, 2004). Our study shows that the secretion of the galls induced by E. acaciae on Prosopis is produced consistently in considerable amounts during the whole development of the gall wasp larvae, and is indeed nectar like. Its composition is similar to that of EFNs (e.g. Ruffner and Clark, 1986; Smith et al., 1990; Koptur, 1994; Heil et al., 2000), and it is particularly rich in sucrose, a strong attractant for ants (Bixenmann et al., 2011; Heil, 2011).
The gall secretion actually triggers a multispecies interaction that is more complex than just involving the ants as defensive partners of the cynipid wasps. Indeed, the gall nectar also attracted many vespid wasps and some Diptera flies. While the latter are probably nectar thieves, ants and vespid wasps can be considered as functionally equivalent. Vespid wasps are important predators (Raveret Richter, 2000) and have been reported as effective plant guards in mutualisms mediated by EFNs (e.g. Koptur, 1985; Cuautle and Rico-Gray, 2003; Alves-Silva et al., 2013). Among ants, Camponotus species were the most frequent and abundant collecting the gall nectar. Species of this genus are indeed typical EFN feeders in the Americas (e.g. Chavarro-Rodriguez et al., 2013; Aranda-Rickert et al., 2014). Interestingly, some of the dried galls were used by C. mus as some kind of satellite nests consisting of only workers.
The interaction involving the gall wasps and their ant/predatory wasp partners is, however, not a text-book example of a mutualism. As often observed in extrafloral nectar-mediated, facultative mutualisms, the benefits gained by the nectar producer are lower than those gained by the nectar consumers or are even almost absent so that it is impossible to quantify them (e.g. Chamberlain and Holland, 2008; Miller et al., 2010; Alves-Silva and Del Claro, 2014). In this system, at least four sets of pair-wise interactions involving the same interacting partners can be identified: plant host–gall wasp, wasp gall secretion–ants or predatory wasps, ants or predatory wasps–parasitoid wasps, ants or predatory wasps–host plant herbivores (this latter interaction and those involving gall inquilines and intruders were not studied here). Each of these interactions depends on several conditions that influence the outcomes of interactions and make them context dependent (see Aranda-Rickert et al., 2017).
In our case, the benefits should be: protection against natural enemies for the cynipid wasp and high-energy food (the nectar-like gall secretion) for the defenders. However, in our experiments, ant exclusion was not related to a lower rate of emergence of E. acaciae wasps or a higher rate of attacks by parasitoids and inquilines. In our experimental setting, it was not possible to exclude at once all kinds of guarding arthropods (excluding flying arthropods with a mesh implies excluding not only predatory but also potential parasitoids and inquilines); therefore, we suppose that excluded ants were replaced by the predatory wasps. Yet, it is to be noted that none of the previous similar experimental studies (i.e. excluding ants with sticky barriers; Washburn, 1984; Seibert, 1993; Fernandes et al., 1999; Inouye and Agrawal, 2004) observed vespid wasps and reported them as gall defenders. Although we suggest a possible defensive role of ants and vespid wasps, our results could also indicate no defensive effect. Such a defensive role could in fact remain latent (i.e. with no apparent and measurable guarding effect) as long as parasitoid and inquiline attacks still allow cynipid wasp survival, and become ecologically relevant (and measurable) in extreme circumstances with an overabundance of natural enemies seriously disrupting the cynipid wasp survival.
Gall secretions were more attractive than plant EFNs to both the ants and the vespid wasps and flies. Prosopis EFNs occur on leaves and usually secrete only small amounts of nectar during much shorter periods of time than galls (Aranda-Rickert et al., 2014). Although we have no quantitative data, Prosopis EFNs were rarely observed to be visited by ants; therefore, they did not affect the interaction between ants and gall wasps. Nevertheless, although the EFNs seem not to facilitate a plant–ant protective mutualism, it is possible that the host plant ultimately benefits from the presence of ants attracted by the galls.
Conclusions
Nectar-like secretions of cynipid galls in South American Prosopis can mediate a complex multispecies web of interactions, in which their role in attracting ants for protection of the cynipid progeny makes galls apparently equivalent to EFNs. Yet, gall secretions are only analogous to EFNs because they too represent a food resource to ants. In fact, they are not functionally analogous to them, because the indirect defence that they mediate is not directed to the plant but to the gall-inducing wasp. This means that the galls’ sugary secretions are functionally equivalent to arthropod’s honeydew and that Bequaert (1924) was not entirely wrong. The secretions are a highly valuable food resource able to attract not one but two aggressive interacting partners, ants and predatory wasps, that appear to function interchangeably as gall defenders. Whether both interacting parts (and the host plant as well) benefit from the interaction might depend on the ecological context in which this interaction takes place, in particular on the outcomes of the several other interactions that revolve around this system.
SUPPLEMENTARY DATA
Supplementary data are available online at https://dbpia.nl.go.kr/aob and consist of Video S1: the aggressive behaviour of the ant Camponotus mus when defending a gall on Prosopis chilensis from vespid wasps and flies.
ACKNOWLEDGEMENTS
We thank J. Torrens and P. Fidalgo for taxonomic identifications of parasitoid and inquiline species, J. L. Nieves-Aldrey for information on E. acaciae life history, Johana Barros for technical help with anatomical studies, and two anonymous reviewers for comments that improved earlier versions of this manuscript. This work was supported by the Consejo Nacional de Investigaciones Científicas y Técnicas (CONICET) of Argentina (PIP2014-2016 to A.A.R.).