-
PDF
- Split View
-
Views
-
Cite
Cite
Xiaoyan Qi, Qiong Liu, Zuxing Wei, Xuyang Hou, Yuhong Jiang, Yin Sun, Shu Xu, Leping Yang, Jun He, Kuijie Liu, Chronic exposure to BDE-47 aggravates acute pancreatitis and chronic pancreatitis by promoting acinar cell apoptosis and inflammation, Toxicological Sciences, Volume 199, Issue 1, May 2024, Pages 120–131, https://doi.org/10.1093/toxsci/kfae024
- Share Icon Share
Abstract
The effect of 2,2′,4,4′-tetrabromodiphenyl ether (BDE-47), a persistent environmental pollutant commonly used as a flame retardant in various consumer products, on pancreatitis has not been clearly elucidated, although it has been reported to be toxic to the liver, nervous system, and reproductive system. Acute pancreatitis (AP) and chronic pancreatitis (CP) models were induced in this study by intraperitoneal injection of caerulein. The aim was to investigate the impact of BDE-47 on pancreatitis by exposing the animals to acute (1 week) or chronic (8 weeks) doses of BDE-47 (30 mg/kg in the low-concentration group and 100 mg/kg in the high-concentration group). Additionally, BDE-47 was utilized to stimulate mouse bone marrow-derived macrophages, pancreatic primary stellate cells, and acinar cells in order to investigate the impact of BDE-47 on pancreatitis. In vivo experiments conducted on mice revealed that chronic exposure to BDE-47, rather than acute exposure, exacerbated the histopathological damage of AP and CP, leading to elevated fibrosis in pancreatic tissue and increased infiltration of inflammatory cells in the pancreas. In vitro experiments showed that BDE-47 can promote the expression of the inflammatory cytokines Tnf-α and Il-6 in M1 macrophages, as well as promote acinar cell apoptosis through the activation of the PERK and JNK pathways via endoplasmic reticulum stress. The findings of this study imply chronic exposure to BDE-47 may exacerbate the progression of both AP and CP by inducing acinar cell apoptosis and dysregulating inflammatory responses.
In recent years, there has been extensive research on the impact of environmental pollutants on the development of diseases in organisms. Polybrominated diphenyl ethers (PBDEs) are a group of organic persistent pollutants that include more than 200 congeners and have been widely used as flame retardants in commercial and household products to prevent fire accidents (Karandrea et al., 2017; Ríos et al., 2021; Yost et al., 2016). PBDEs are characterized by their persistence and ability to accumulate in the environment (Marquès et al., 2022). They are commonly found in air, water, and soil, and tend to accumulate in lipid-rich tissues including adipose tissue, placenta, blood, and milk. The rise of PBDEs and their derivatives in the environment has resulted in their accumulation in human tissues, posing significant risks to human health (Montalbano et al., 2020). BDE-47, also known as 2,2′,4,4′-Tetrabromodiphenyl ether, is the most frequently detected PBDE congener in both environmental samples and human tissues. Its persistent toxicity has garnered considerable attention in relation to human health (Chang et al., 2020; Liu et al., 2019).
BDE-47 exhibits a wide range of toxic effects, such as hepatotoxicity, reproductive and developmental toxicity, neurotoxicity, and endocrine toxicity (Ji et al., 2019; Xue et al., 2022; You et al., 2018; Zhang et al., 2015). For instance, Yang et al. (2019) demonstrated that exposure to BDE-47 aggravated liver steatosis and liver fibrosis in diet-induced obese mice. Park et al. (2014) reported that abnormal activation of inflammatory cytokines, induced by BDE-47, could destroy the functions necessary for placental development and successful pregnancy. In a study conducted by Liu et al. (2022), it was found that BDE-47 induced PC-12 cell differentiation through the downstream TrkA pathway, leading to the loss of hippocampal neurons in BALB/c mice. Although toxicity studies of BDE-47 have made progress in a number of tissue and metabolic diseases, there is currently a limited understanding of its impact on the pancreas and we found that the effect of BDE-47 on pancreatitis has not been reported.
PBDEs, due to their persistent nature and high lipophilicity, have the ability to accumulate in the adipose tissue and pancreas of exposed organisms (Darnerud and Risberg, 2006; Liu et al., 2023; Lundgren et al., 2007). Pancreatitis, an inflammatory disease of the pancreas, is a commonly observed condition (Leung, 2010). Pancreatitis is the leading cause of gastrointestinal disease-related hospitalizations and is associated with considerable morbidity, mortality, and socioeconomic burden (Mayerle et al., 2019). Pancreatitis can be divided into acute pancreatitis (AP) and chronic pancreatitis (CP) based on the severity and progression of the disease. AP is characterized by local and systemic inflammatory reactions. Although most patients experience mild AP, about 20% will progress to moderate or severe AP, which can involve pancreatic necrosis, tissue damage, organ failure, or a combination of these factors, resulting in a mortality rate of 20%–40% (Boxhoorn et al., 2020; Gukovskaya et al., 2017). Patients who experience episodes of AP have a 20%–30% likelihood of relapse, and approximately 10% of recurrent cases eventually develop CP. Once CP is diagnosed, the risk of pancreatic cancer increases significantly (Beyer et al., 2020; Boxhoorn et al., 2020; Cai et al., 2022). Studies have revealed that systemic complications associated with AP are caused by excessive acinar cell death and uncontrolled or relaxed control of immune system activation (Gukovskaya et al., 2017; Habtezion, 2015; Phillip et al., 2014).
Interestingly, previous studies have revealed a close relationship between PBDE toxicity and the promotion of cell death and inflammation in the body. For example, the pentaBDE mixture DE-71 can enhance the proinflammatory response of monocytes and macrophages (Mynster Kronborg et al., 2016), and BDE-47 aggravates liver injury and promotes apoptosis of bronchial epithelial cells by promoting inflammation (Montalbano et al., 2020; Yang et al., 2019). However, the impact of PBDEs on the progression of pancreatitis has not been investigated. Thus, this study focuses on examining the effects of BDE-47 on AP and CP, as well as elucidating its underlying mechanism. These findings will contribute to a novel understanding of the pancreatic toxicity associated with BDE-47 exposure during pancreatitis.
Materials and methods
Animal model
Male C57BL/6J mice aged 6–8 weeks were purchased from Hunan SJA Laboratory Animal Co., Ltd, with 10 mice in each group. The mice were housed in a specific pathogen-free barrier facility at the Medical Laboratory Animal Center of the Second Xiangya Hospital of Central South University. The experimental procedures conformed to the standards of the Guide for the Care and Use of Laboratory Animals and were approved by the Institutional Animal Care and Use Committee of the Second Xiangya Hospital of Central South University. The study methods did not involve the principles of randomization and blinding.
AP molds were prepared by intraperitoneal injection of caerulein (50 μg/kg) at 1 h intervals, for a total of 8 injections. The mice were sacrificed at 24 h after the first injection of caerulein. The CP model was constructed by administering caerulein injections 3 days per week for up to 4 weeks. The body weights of the mice were measured and recorded, and each injection of caerulein was prepared at a dose of 50 μg/kg. The injections were administered 6 times a day, with a 1-h interval, 3 days a week for 4 weeks.
Western blotting
The cells were collected, washed with cold PBS and dissolved in appropriate amounts of protein lysis solution containing protease inhibitor, phosphatase inhibitor, and RIPA (Radio immunoprecipitation Assay) (the dilution ratio of the 3 was 1:1:100). After being placed on ice for 30 min to allow for lysis, the mixture was centrifuged at 4°C and 12 000 rpm for 15 min. The resulting supernatant was collected and recorded. The protein concentration was measured using the BCA (Bicinchoninic Acid) method, and the loading volume was calculated accordingly. The prepared protein samples were denatured by incubating them in a water bath at 100°C for 10 min. The denatured protein samples were added to the lanes of the electrophoresis tank, followed by electrophoresis conducted under appropriate voltage and current conditions. Subsequently, the proteins were transferred onto methanol-activated PVDF membranes using the wet transfer method. The membrane was then blocked with 5% skimmed milk for 1.5 h at room temperature. The primary antibodies were incubated separately overnight at 4°C in a shaker. The next day, the primary antibody was washed off with TBST, and the then secondary antibody was incubated for 45 min at room temperature. Finally, the development and fixation were performed on a chemiluminescence imager, and the molecular weight and net optical density values of the target bands were analyzed by a gel image processing system.
Quantitative real-time PCR
The target tissues and cells were collected, rinsed with cold PBS, and treated with an appropriate amount of TRIZOL reagent. Next, 0.2 times the volume of chloroform was added to the mixture, which was then vortexed, thoroughly mixed, and subsequently subjected to centrifugation at 4°C for 15 min at 12 000 g. The supernatant was collected, and the RNA was extracted by adding an equal volume of isopropanol and then centrifuged at 4°C for 10 min at 12 000 g. The supernatant was discarded, leaving behind a visible white precipitate. Subsequently, 75% anhydrous ethanol was added and centrifuged at 4°C and 8000 g for 5 min. The supernatant was discarded, and DEPC (Diethylpyrocarbonate) water was added to obtain the RNA. The cDNA was synthesized using a high-capacity cDNA reverse transcription kit. Primers were designed with the following primer sequences: Il-1β (forward, TCGCAGCAGCACATCAACAAGAG, reverse, TGCTCATGTCCTCATCCTGGAAGG), Il-6 (forward, CTCCCAACAGACCTGTCTATAC, reverse, CCATTGCACAACTCTTTTCTCA), Il-10 (forward, GTAGAAGTGATGCCCCAGGC, reverse, CACCTTGGTCTTGGAGCTTATT), Il-4 (forward, CCCCCAGCTAGTTGTCATCC, reverse, AGGACGTTTGGCACATCCAT), Cd206 (forward, GACTGCTGCTGAGTCCAGTT, reverse, AGGGATCGCCTGTTTTCCAG), Tnf-α (forward, ATGTCTCAGCCTCTTCTCATTC, reverse, GCTTGTCACTCGAATTTTGAGA), Col1a1 (forward, TGAACGTGGTGTACAAGGTC, reverse, CCATCTTTACCAGGAGAACCAT), Fn1 (forward, CCGAGTCTGGCAGCATTGTGATC, reverse, CAAGTTGGTTGGAGGAGCGACAG), αSMA (forward, CGTGGCTATTCCTTCGTGACTACTG, reverse, CGTCAGGCAGTTCGTAGCTCTTC), and β-actin (forward, GAGGTATCCTGACCCTGAAGTA, reverse, CACACGCAGCTCATTGTAGA). PCR was performed using 2× Universal SYBR Green Fast qPCR mix, and the results were divided using a LightCycler 96 system.
Amylase and lipase assays
Plasma was obtained from blood by centrifuging at 3000 rpm for 10 min with 20 μl of 0.5 M EDTA. The trypsin and lipase activities in plasma were quantified by a clinical analysis system. Amylase activity was assessed using an EPS substrate-based kit, and lipase activity was determined using a methyltriazine substrate-based kit, both measured by a clinical assay AU1000 system (Beckman).
Masson trichrome staining
Paraffin sections were dewaxed and washed sequentially with tap water and distilled water. Nuclei staining was then performed using either Regaud hematoxylin staining solution or Weigert iron hematoxylin staining solution for 5–10 min. This was followed by alcoholic fractionation with 1% hydrochloric acid for 5–15 s and a thorough water wash with the addition of 0.1%–1% lithium carbonate drop by drop for 5 min to enhance the anti-blue effect. The sections were stained with Masson Lichon red acidic reddening solution for 5–10 min and then washed with a 2% glacial acetic acid aqueous solution for 1 min. Masson Lichon red acidic reddening solution was added for 5–10 min. Subsequently, the sections were washed successively with a 2% glacial acetic acid aqueous solution for 1 min, a 1% phosphomolybdic acid aqueous solution for 3–5 min, and again with a 2% glacial acetic acid aqueous solution for 1 min. They were then stained directly with aniline blue aqueous solution or 1% photo green aqueous solution for 1–2 min without prior washing. Afterward, the sections were washed with a 0.2% glacial acetic acid aqueous solution for 1 min, dehydrated briefly with 95% ethanol for 2–3 s, and dehydrated 3 times with anhydrous ethanol for 5 min each time. The films were sealed with neutral gum, examined under a microscope, and images were collected and analyzed.
Hematoxylin and eosin staining
The tissues samples were embedded, sectioned, and then baked at 60°C for 45 min. Subsequently, the sections were immersed in xylene I for 20 min, xylene II for 20 min, anhydrous ethanol I for 5 min, anhydrous ethanol II for 5 min, and 75% alcohol for 5 min, followed by a 3-min rinse with tap water. The sections were stained with hematoxylin staining solution for 3–5 min. They were then differentiated using differentiation solution, washed with tap water again, returned to blue with blue return solution, and finally washed with running water. The sections were dehydrated in 85% and 95% gradient alcohol for 5 min each, and then stained with eosin staining solution for 5 min. The sections were immersed in anhydrous ethanol for 5 min, followed by a 5-min immersion in dimethyl, and finally 5 min in xylene II. After that, they were sealed with neutral gum. Finally, the sections were sent for microscopic examination, image acquisition, and analysis.
Immunohistochemistry
The pancreatic tissues were embedded, sectioned, and then baked at 60°C for 45 min. They were subsequently dewaxed in xylene and anhydrous ethanol with varying concentration gradients. After a 3-min rinse in tap water, the tissues were placed in boiled TRIS-EDTA repair solution for 20 min, followed by a 30-min cooling period. They were then rinsed in PBS for 3 min. Once the excess PBS was removed, the tissues were placed on wet boxes and incubated overnight at 4°C with the primary antibody added dropwise to each piece. The next day, the primary antibody was washed off 3 times with PBS. Subsequently, the sections were incubated with peroxidase blocker for 20 min, washed with PBS, and then incubated for 20 min at room temperature with a drop of secondary antibody. Afterward, the sections were rinsed with water and a drop of DAB was added for 5 min to facilitate color development. Hematoxylin staining was applied for 1 min. Subsequently, the sections were immersed in tap water, followed by the dropwise addition of a differentiation solution for 1 s. After another tap water immersion, the sections were treated with hot water anti-blue for 7 min. They were then removed, dehydrated sequentially in a concentration gradient of anhydrous ethanol and xylene, and sealed with neutral gum. Finally, the results were observed under the microscope.
Immunofluorescence staining
The cell medium was discarded, and the cells were washed with PBS for 3 min. Then, the cells were fixed with paraformaldehyde for 10 min, followed by another wash with PBS. Next, Triton was added to permeabilize the cells for 10 min. After that, the cells were incubated with the blocking solution at room temperature for 30 min. Subsequently, the cells were placed in a humidified chamber, and the primary antibody was added dropwise and incubated overnight at 4°C. On the following day, the primary antibody was washed away using PBS, and then the cells were incubated with the secondary antibody at room temperature for 1 h. The secondary antibody was washed off with PBS, a new slide was taken, DAPI was added dropwise to stain the nuclei, and images were obtained under a fluorescence microscope or confocal microscope.
Primary pancreatic stellate cells and primary acinar cell culture
C57 mice were decannulated and sacrificed, and mouse pancreatic tissues were extracted under aseptic conditions. The pancreatic tissues were cut to the size of beans, collagenase IA and trypsin inhibitor were added, and the tissues were digested at 37°C in a 5% CO2 incubator for 15 min. Digestion was terminated by adding complete medium; the tissues were centrifuged at 300 rpm for 2 min and gently washed 3 times with PBS, and centrifugation was repeated 3 times. The tissue was resuspended in DMEM/F12 complete medium and returned to the incubator. During this period, careful observation was conducted, and the medium was regularly replaced. Generally, after 7 days of culture, the emergence of radiolucent pancreatic fibroblasts was observed.
The protocol for isolation of acinar cells from mice was based on a mechanical and enzymatic dissociation technique, as described in our previous report (He et al., 2021). Briefly, 6- to 8-week-old mice were sacrificed by CO2 asphyxiation, and the pancreas was then dissected and immersed in Waymouth’s medium. The pancreas was cut into approximately 1 cm pieces and incubated in HBSS 1× buffer containing 200 U/ml collagenase IA, 0.25 mg/ml trypsin inhibitor, and 0.2% FBS on a shaker (80 rpm/min) at 37°C for 20 min. Next, the pancreas was triturated slightly by passing through a 1-ml pipette tip 10 times and filtering through a 100-µm filter. After 2 washes with PBS, the acinar cells were resuspended in complete medium (Waymouth’s medium supplemented with 2.5% FBS, 1% penicillin/streptomycin, 0.25 mg/ml trypsin inhibitor, and 25 ng/ml recombinant human epidermal growth factor) and cultured at 37°C in a humidified incubator containing 5% CO2.
Flow cytometry
The apoptosis rate of cultured cells was determined using flow cytometry after staining with annexin V and PI. The annexin V/PI apoptosis detection kit was purchased from Beyotime, China, and utilized following the manufacturer’s guidelines. Briefly, cells from various groups were harvested, washed 3 times with PBS, resuspended in 1× binding buffer, and then stained with 5 μl of annexin V and 10 μl of PI solution for 15 min at 37°C. Next, the cells were analyzed using BD FACSARIA II flow cytometry (Becton Dickinson).
The expression of CD206 and CD80 in marrow-derived macrophages (BMDMs) was determined using flow cytometry after staining with anti-CD80 antibody (conjugated with PE-CF594) and anti-CD206 antibody (conjugated with Alexa Fluor 647). The cells were analyzed using BD FACSARIA II flow cytometry (Becton Dickinson).
Antibodies
The antibodies were purchased as follows: anti-Ly6G (1:50 dilution, no. 14-5931-82, Thermo), anti-CD68 (1:500 dilution, no. sc-20060, Santa), anti-CD80 (1:100 dilution, no. 562504, BD), anti-CD206 (1:100 dilution, no. 565250, BD), anti-aSMA (1:500 dilution, no. sc-53142, Santa), anti-COL1Α1 (1:800 dilution, no. 67288-1-Ig, Proteintech), anti-Bcl-2 (1:1000 dilution, no. A0208, Abclonal), anti-Bak (1:1000 dilution, no. 12105, CST), anti-Cleaved Casp3 (1:1000 dilution, no. abs132005, Absin), anti-Casp3 (1:1000 dilution, no. A0214, Abclonal), anti-Cleaved Casp9 (1:1000 dilution, no. 10380-1-AP, Proteintech), anti-Casp9 (1:1000 dilution, no. sc-56076, Santa), anti-actin (1:5000 dilution, no. 81115-1-RR, Proteintech), anti-p-PERK (1:1000 dilution, no. AP0886, Abclonal), anti-PERK (1:1000 dilution, no. 3192S, CST), anti-ATF4 (1:1000 dilution, no. A0201, Abclonal), anti-CHOP (1:1000 dilution, no. 2895S, CST), anti-p-JNK (1:1000 dilution, no. 4668S, CST), anti-JNK (1:1000 dilution, no. 9252S, CST).
Statistical analysis
All data are expressed as the mean ± standard error using GraphPad Prism 8. The significance between 2 groups was analyzed by unpaired Student’s t test (using a parametric test when the data had a normal distribution and homoscedasticity otherwise using a nonparametric test), and 1-way analysis of variance was used to determine the differences among multiple groups. A p value of < .05 was considered statistically significant.
Results
Effects of acute exposure to BDE-47 on AP and CP in mice
To explore the effects of PBDEs on pancreatitis, we chose BDE-47, the most frequently detected PBDE congener, to administer to mice with AP and CP models. Initially, we examined the effects of acute exposure to BDE-47 on AP. The low-concentration group (30 mg/kg) and high-concentration group (100 mg/kg) of mice were orally administered BDE-47 for 1 week. Then, the AP model was induced by caerulein, and the mice were sacrificed the next day for testing (Figure 1A). The results of hematoxylin and eosin (H&E) staining revealed no significant difference in pancreatic histology between the BDE-47-treated groups and the control group (Figs. 1B and 1C). Furthermore, there was no significant difference in the activity of amylase and lipase in plasma among the BDE-47 acute exposure groups and the control group (Figure 1D). Neutrophils were marked by Ly6G. Therefore, we analyzed the infiltration of neutrophils in pancreatic tissues using immunofluorescence, and there was no obvious difference observed among the groups exposed to BDE-47 acutely and the control group (Figure 1E). Subsequently, we investigated the impact of acute BDE-47 exposure on CP. After intragastrical administration of low (30 mg/kg) and high (100 mg/kg) BDE-47 for 1 week, the mice were intraperitoneally injected with caerulein for 4 weeks (6 times/days, 3 days/week) (Figure 1F). Pancreatic tissues from the CP model were subjected to H&E and Masson trichrome staining after acute exposure, and no obvious difference was found compared with the control group (Figure 1G). Thus, these findings suggested that acute exposure to BDE-47 did not affect the development of AP and CP in mice.
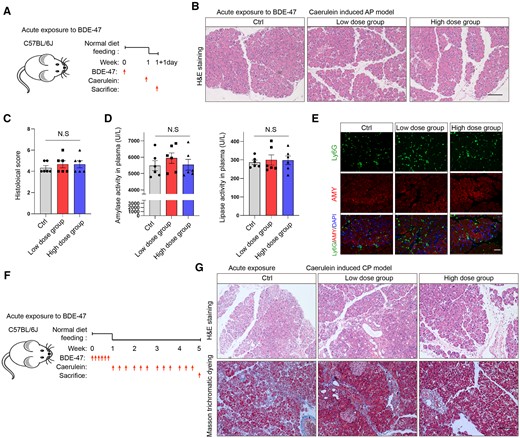
Effects of acute exposure to 2,2′,4,4′-tetrabromodiphenyl ether (BDE-47) on acute pancreatitis (AP) and chronic pancreatitis (CP). A, Schematic diagram of acute exposure to BDE-47 and induction of AP: Mice were orally administered BDE-47 for 1 week (low-concentration group: 30 mg/kg, high-concentration group: 100 mg/kg), and AP was induced by caerulein. The mice were sacrificed on the following day. B, hematoxylin and eosin (H&E) staining was used to analyze the pathological changes in pancreatic tissue in the AP model after acute exposure. The scale was 100 µm. C, Pancreatic histological scores in AP models after acute exposure. D, Plasma amylase and lipase activity in each group. E, Neutrophils were labeled with Ly6G, and the number of neutrophils infiltrated in pancreatic tissue was analyzed by immunofluorescence. F, Experimental schematic diagram of acute exposure to 2,2′,4,4′-tetrabromodiphenyl ether (BDE-47) and induction of CP: Low (30 mg/kg) and high (100 mg/kg) concentrations of BDE-47 were administered by gavage for 1 week, followed by an intraperitoneal injection of caerulein for 4 weeks (6 times/days, 3 days/week). G, H&E staining was used to analyze the pathological damage of pancreatic tissue in the CP model after acute exposure (above), and Masson trichrome staining was used to analyze the fibrosis of the pancreas (below). The scale was 100 µm. Data in graphs are presented as the mean ± SD of 3 independent experiments. Ctrl, control; NS, no significance.
Chronic exposure to BDE-47 aggravates AP and CP in mice
PBDEs exhibit persistence and bioaccumulation in vivo. Therefore, we prolonged the exposure time of BDE-47 to mice to further explore its effect on pancreatitis. The mice were orally administered BDE-47 for a duration of 8 weeks, with a low-concentration group receiving 30 mg/kg and a high-concentration group receiving 100 mg/kg, and then AP was induced by caerulein injection. On the second day, the experimental mice were sacrificed to examine the impact of chronic BDE-47 exposure on AP (Figure 2A). H&E staining of the pancreas revealed a noticeable increase in pathological injury and infiltration of inflammatory cells caused by BDE-47 when compared with the control group (Figs. 2B and 2C), and the plasma levels of amylase and lipase exhibited a significant elevation (Figure 2D). However, no significant difference was observed between the low-concentration and high-concentration groups. Next, we examined the effect of chronic exposure to BDE-47 on CP. Chronic exposure of BDE-47 to CP was induced through gavage of BDE-47 at low (30 mg/kg) and high (100 mg/kg) concentrations for 4 weeks, followed by coadministration of caerulein and BDE-47 from week 5 to week 8 (Figure 2E). H&E and Masson’s trichrome staining revealed that the BDE-47-treated group exhibited more severe pancreatic tissue damage and fibrosis compared with the unexposed group (Figure 2F). Furthermore, CD68 was utilized as a marker for macrophages, which play a pivotal role in the progression of CP. Immunohistochemical staining analysis demonstrated a significant increase in the number of macrophages infiltrating pancreatic tissue following exposure to BDE-47 (Figure 2G). To investigate whether the effect is determined by BDE-47 alone or a combination of BDE-47 and caerulein, we chose to analyze the influence of BDE-47 over a lengthy period of 8 weeks. We found that the sole administration of BDE-47 did not induce noticeable histological changes in the pancreas or show evidence of pancreatitis at any point during this period (Supplementary Figs. 1A and 1B). Therefore, these findings indicate that chronic exposure to BDE-47 exacerbates both AP and CP in mice.
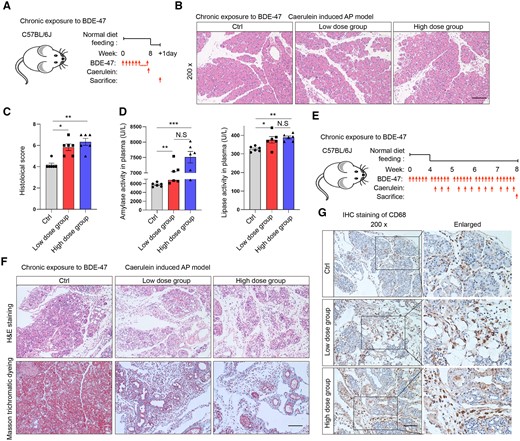
Chronic exposure to BDE-47 aggravates AP and CP. A, Schematic diagram of chronic exposure to BDE-47 and induction of acute pancreatitis: The mice were orally administered BDE-47 for a duration of 8 weeks, with a low-concentration group receiving 30 mg/kg and a high-concentration group receiving 100 mg/kg, and then AP was induced by caerulein injection. B, H&E staining was used to analyze the pathological changes in pancreatic tissue in the AP model after chronic exposure. The scale was 100 µm. C, Pancreatic histochemical scores in AP models after chronic exposure. D, Plasma amylase and lipase levels. E, Diagram depicting the process of animal intervention: Chronic exposure of BDE-47 to CP was induced through gavage of BDE-47 at low (30 mg/kg) and high (100 mg/kg) concentrations for 4 weeks, followed by coadministration of caerulein and BDE-47 from week 5 to week 8. F, H&E staining was used to analyze the pathological damage changes in pancreatic tissue in the CP model after chronic exposure (above), and Masson trichrome staining was used to analyze pancreatic fibrosis (below). The scale was 100 µm. G, CD68-labeled macrophages and immunohistochemistry (IHC) staining were used to analyze the number of infiltrated macrophages in pancreatic tissue. Data in graphs are presented as the mean ± SD of 3 independent experiments. Ctrl, control; NS, no significance, *p < .05, **p < .01 and ***p < .001.
BDE-47-promoted M1 macrophage activation but did not influence M2 macrophage activation
In order to investigate the mechanism by which BDE-47 exacerbates AP and CP, we initially evaluated the impact of BDE-47 on macrophage biology. BMDMs were cultured and induced as in our previous report (He et al., 2021). Then, the cells were exposed to different concentrations of BDE-47, as determined according to a prior study (Liu et al., 2022). The CCK8 assay showed that BDE-47 could directly damage macrophages, resulting in a concentration-dependent decrease in cell activity (Figure 3A). Flow cytometry analysis revealed that 8.83% of macrophages underwent apoptosis following 48-h treatment with 20 µM BDE-47, whereas 20.55% of macrophages underwent apoptosis after exposure to 40 µM BDE-47 (Figure 3B). BMDMs were pretreated with BDE-47 for 2 h and subsequently stimulated with 100 ng/ml lipopolysaccharide (LPS) for 24 h. Treatment with BDE-47 alone led to an upregulation of Il-6 and Tnf-α mRNA expression, which might be explained by its proapoptotic effect, but it did not influence the expression of CD206 or Il-10 mRNA (Supplementary Figs. 2A and 2B). In addition, 20 µM BDE-47 did not affect Il-1β mRNA expression and 40 µM BDE-47 reduced Il-1β expression, BDE-47 upregulated the expression of Il-6 and Tnf-α mRNA in a concentration-dependent manner (Figs. 3C and 3D), which was consistent with a previous report (Gao et al., 2023). Subsequently, the effect of BDE-47 on M2 macrophages was examined. M2 macrophages were induced by IL-4 (20 ng/ml) for 24 h. After cotreatment with BDE-47, the expression of the activation markers of M2 macrophages, including Cd206, Il-4 and Il-10, was determined by quantitative real-time PCR (q-PCR). The results showed that 20 or 40 µM BDE-47 could not affect the expression of these genes (Figure 3E). To definitively ascertain the impact of BDE-47 on macrophage activation, we employed flow cytometry to examine the phenotypic switch in BMDMs treated with BDE-47. The results showed that BDE-47 increased the proportion of M1 macrophages stimulated by LPS, as indicated by CD80 expression, but did not influence the proportion of M2 macrophages stimulated by IL-4, as marked by CD206 expression (Figs. 3F and 3G). In summary, whereas BDE-47 could promote apoptosis, it also significantly enhanced the activation of M1 macrophages, potentially contributing to the exacerbation of AP.
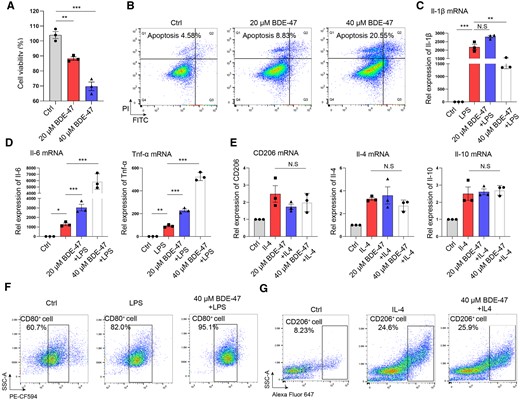
BDE-47 promoted M1 macrophage activation but did not change M2 macrophage activation. A, BDE-47 (20 and 40 µM) directly acted on bone marrow-derived macrophages, and CCK8 was used to detect cell activity. B, Macrophage apoptosis was detected by flow cytometry. C, BMDMs were pretreated with BDE-47 for 2 h, 100 ng/ml LPS was then added to stimulate them for 24 h, and Il-1β mRNA expression was detected by quantitative real-time PCR (q-PCR). D, The expression levels of Il-6 and Tnf-α in M1 macrophages were detected by q-PCR. E, The expression levels of the M2 macrophage markers CD206, Il-4 and Il-10 were detected by q-PCR. F, The ratio of CD80-positive cells in BMDMs treated by 40 μM BDE-47. G, The ratio of CD206-positive cells in BMDMs treated by 40 μM BDE-47. Data in graphs are presented as the mean ± SD of 3 independent experiments. Ctrl, control; NS, no significance, *p < .05, **p < .01 and ***p < .001.
BDE-47 did not affect the activation of pancreatic stellate cells
In order to investigate the underlying cause for the exacerbation of fibrosis in CP resulting from chronic exposure to BDE-47, pancreatic stellate cells were extracted for in vitro experiments. The stellate cells were treated with different concentrations of BDE-47 for 48 h, and their cell viability was assessed using the CCK-8 assay. Intriguingly, in line with the aforementioned findings, exposure to BDE-47 led to a concentration-dependent decrease in stellate cell viability (Figure 4A). Primary pancreatic stellate cells were stimulated with TGF-β for 24 h, and q-PCR assays revealed that BDE-47 had no impact on the mRNA expression of fibrosis-associated markers (αSMA, Col1α1, and Fn1) in these cells (Figs. 4B–D). Furthermore, immunofluorescence assays confirmed that BDE-47 had no influence on the protein expression of profibrotic markers (αSMA and COL1Α1) (Figure 4E). Thus, the activation of pancreatic stellate cells did not contribute to the exacerbation of fibrosis in CP resulting from chronic exposure to BDE-47.
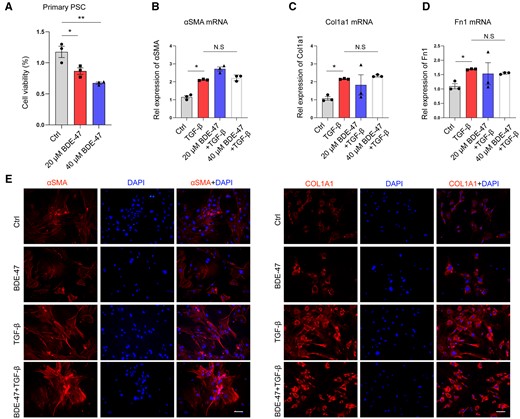
BDE-47 did not affect the activation of pancreatic stellate cells. A, Mouse pancreatic primary stellate cells were extracted and treated with different concentrations of BDE-47 for 48 h. Cell viability was detected by CCK8 assay. B–D, TGF-β (10 ng/ml) was used to stimulate primary pancreatic stele cells, and q-PCR was used to detect the effect of BDE-47 on the expression of profibrotic marker RNA (aSMA, Col1a1, and Fn1) in pancreatic stele cells. E, The protein expression of aSMA and COL1Α1 was detected by immunofluorescence assay. The scale is 20 μm. Data in graphs are presented as the mean ± SD of 3 independent experiments. Ctrl, control; NS, no significance, *p < .05, **p < .01.
BDE-47-promoted apoptosis of pancreatic acinar cells
In order to investigate the mechanism by which BDE-47 exacerbates pancreatic tissue damage, primary pancreatic acinar cells were isolated and incubated with BDE-47 and caerulein for 6 h (Figure 5A). The release of pancreatic enzymes is indicative of acinar cell injury, which can be assessed by measuring the levels of amylase and lipase in the cell supernatant. Therefore, the cell supernatant from each group was collected to measure amylase and lipase activity. The results demonstrated that BDE-47 alone increased the levels of lipase and amylase, and this effect was significantly enhanced when BDE-47 was co-incubated with caerulein (Figs. 5B and 5C). Because quantifying the apoptosis rate was not feasible with primary acinar cells, we instead investigated the effect of BDE-47 on the acinar cell line, 266-6 cells. The apoptosis rate of 266-6 cells was detected by flow cytometry. Similar to primary acinar cells, treatment with 40 μM BDE-47 alone resulted in an increased apoptosis rate (12.89% vs 5.35%), and when coincubation of BDE-47 with taurocholate acid further elevated the apoptosis rate compared with taurocholate acid treatment alone (28.76% vs 18.93%) (Figure 5D). Previous studies have suggested that the generation of ROS induced by BDE-47 may contribute to cell death (Zhao et al., 2020). Because mitochondria are the primary source of ROS and to gain further insight into the molecular mechanism underlying BDE-47-induced apoptosis in acinar cells, we examined the expression of apoptosis effectors or activators associated with the mitochondrial pathway. In acinar cells, BDE-47 upregulated the protein expression of Bak, Cleaved Caspase 3, and Cleaved Caspase 9, whereas downregulating the expression of the anti-apoptotic factor Bcl-2 (Figure 5E). Furthermore, the immunohistochemistry (IHC) assays revealed that chronic exposure to BDE-47 obviously increased the expression of cleaved Caspase 3 in the pancreas in both the AP and CP models (Figure 5F). Collectively, these findings provide evidence that BDE-47 promotes apoptosis in acinar cells during both AP and CP.
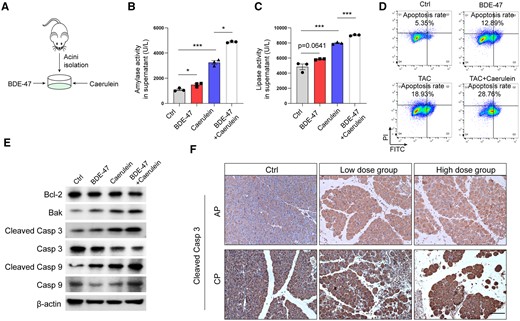
BDE-47 increased the apoptosis of pancreatic acinar cells. A, Schematic diagram of simulated pancreatitis in vitro: primary mouse acinus cells were extracted and incubated with BDE-47 and caerulein. B–C, The levels of amylase and lipase in the supernatant were detected. D, Pancreatic acinar cell Line 266-6 cells were selected, and apoptosis was detected by flow cytometry. E, The expression of Bcl-2, Bak, cleaved Caspase 3, and cleaved Caspase 9 was detected by Western blotting (WB). F, IHC measured pancreatic cleaved Caspase 3 expression in AP and CP models after chronic exposure. The scale is 100 μm. Data in graphs are presented as the mean ± SD of 3 independent experiments. Ctrl, control, *p < .05, and ***p < .001.
Activation of endoplasmic reticulum stress is the key mechanism by which BDE-47 aggravates acinar cell injury
Acinar cells equipped with a high protein synthesis ability were thus easily influenced by endoplasmic reticulum disorder. Endoplasmic reticulum stress is often triggered by pancreatic toxins and plays a crucial role in inducing apoptosis. The expression levels of key endoplasmic reticulum stress players, including p-JNK, p-PERK, ATF4, and CHOP, were detected by Western blotting (WB), and cotreatment of BDE-47 with caerulein obviously increased the expression of these proteins in acinar cells, suggesting that PERK and JNK pathway-mediated endoplasmic reticulum stress was enhanced by BDE-47 (Figure 6A). Furthermore, immunohistochemical analysis was used to detect CHOP expression in AP and CP models, providing in vivo confirmation that BDE-47 increased endoplasmic reticulum stress in acinar cells (Figure 6B). Subsequently, acinar cells were incubated with the PERK inhibitor GSK2606414, which was found to enhance the viability of acinar cells cotreated with BDE-47 and caerulein (Figure 6C). Moreover, the JNK inhibitor SP600125 was able to reverse the detrimental effect of BDE-47 and caerulein on cell viability (Figure 6D).
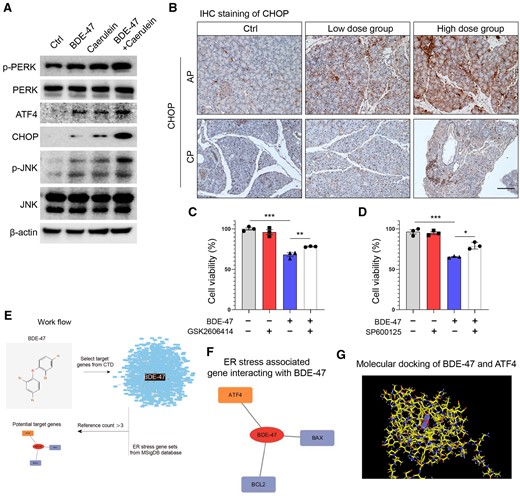
Activation of endoplasmic reticulum stress is the key mechanism by which BDE-47 aggravates acinar cell injury. A, Primary acinus cells were extracted and cotreated with BDE-47 and caerulein, and the expression of the key endoplasmic reticulum stress proteins p-PERK, p-JNK, ATF4, and CHOP was detected by WB. B, CHOP expression in AP and CP models was determined by IHC. C, The effects of the PERK inhibitor GSK2606414 (0.2 μM) on the activity of acinar cells induced by BDE-47 (40 μM) and caerulein were detected by CCK8. D, The effects of the JNK inhibitor SP600125 (2.5 μM) on the activity of acinar cells induced by BDE-47 and caerulein were detected by CCK8. E, Validation workflow for potential target proteins in BDE-47-induced endoplasmic reticulum stress. F, Comparative Toxicogenomics Database and MSigDB database were used to verify the genes targeted by BDE-47 and regulate endoplasmic reticulum stress. Using an evidence threshold greater than 3, 3 target genes were identified: ATF4, BAX, and BCL2. G, The 3-dimensional representation of the binding mode of BDE-47 with ATF4 proteins. The residues in the binding site are shown by color stick and BDE-47 is shown in blue sticks. Data in graphs are presented as the mean ± SD of 3 independent experiments. Ctrl, control, *p < .05, **p < .01, and ***p < .001.
To verify the potential targeting protein by BDE-47 to induce endoplasmic reticulum stress, we conducted molecular docking prediction analysis. The Comparative Toxicogenomics Database is a publicly available and reliable resource that can be accessed at https://ctdbase.org/about. It provides curated information about interactions between chemicals and genes/proteins. We identified the genes that are targeted by BDE-47. Subsequently, we retrieved the gene sets associated with endoplasmic reticulum stress from the MSigDB database, which is available at https://www.gsea-msigdb.org (Figure 6E). By identifying the overlapping genes, our team successfully identified the specific genes within the gene sets associated with endoplasmic reticulum stress that are targeted by BDE-47. The results of docking simulation showed 3 genes using a threshold of evidence greater than 3 (ATF4, BAX, and BCL2) associated with endoplasmic reticulum stress (Figure 6F). Figure 6G illustrates the 3-dimensional representation of the binding mode between BDE-47 and ATF4 proteins. In conclusion, our findings suggest that BDE-47 promotes acinar cell death by inducing endoplasmic reticulum stress through the PERK/JNK pathway.
Discussion
The adverse health effects caused by exposure to persistent pollutants known as PBDEs have gained increasing attention in the field of public health. These effects include reproductive toxicity, hepatotoxicity, neurotoxicity, and endocrine disorders (Ji et al., 2019; Karandrea et al., 2017; Park et al., 2014; Zhang et al., 2015). A growing body of research has confirmed the close relationship between PBDEs and inflammatory infiltration and oxidative stress (Crespo et al., 2017; Yang et al., 2019; Zhang et al., 2015). Once PBDEs enter the human body, they are specifically targeted and deposited in adipose tissue (Darnerud and Risberg, 2006). The pancreas, being a common site for adipose deposition, is also a target organ for PBDEs (Kim et al., 2014; Lundgren et al., 2007). However, there have been limited studies on the toxicity of PBDEs specifically targeting the pancreas. Pancreatitis is a prevalent gastrointestinal disorder, and its severity depends on the modulation of the inflammatory response (Gukovskaya et al., 2017). It is speculated that the accumulation of PBDEs in the body may exacerbate the severity of pancreatitis through proinflammatory mechanisms, similar to the toxicity observed in other organs. This study was conducted to investigate the hypothesis. Our results, which support our scientific hypothesis, indicate that exposure to BDE-47 exacerbates pancreatitis by upregulating the expression of inflammatory cytokines and promoting apoptosis of acinar cells, thereby aggravating pancreatic tissue damage.
It takes time for BDE-47 to aggravate the development of AP and CP. PBDEs are associated with low-dose and continuous exposure in real-world environments. Consequently, in this study, we found that exposing AP and CP model mice to BDE-47 for a short period of time did not result in a difference in disease severity compared with the unexposed group. As reported in another study, subacute exposure to decabromodiphenyl ether (28 days) did not affect spleen or blood immune cells in Wistar rats (Van der Ven et al., 2008). Exposure of mice to BDE-47 for up to 8 weeks resulted in increased severity of both AP and CP, aggravated pathological injury and fibrosis in pancreatic tissue, and increased infiltration of acute and chronic immune cells in the tissues. BDE-47 had a similar effect on the chronic damage of pancreatic tissue as it did on other tissues. For instance, exposure of mice to BDE-47 for 16 weeks resulted in damaged genome integrity and innate immunity in breast tissue (Lamkin et al., 2022). Obese mouse models exposed to BDE-47 for 15 weeks exhibited significant liver toxicity (Yang et al., 2019). However, in real-life scenarios, organisms are exposed to PBDEs for extended periods due to their ubiquity, persistence, and accumulation. Speculatively, as animal exposure experiments are extended, tissue damage may become more severe, emphasizing the need for increased attention to the adverse health effects of PBDEs on organisms and environmental pollution.
Chronic exposure to BDE-47 exacerbates the dysregulation of the inflammatory network in pancreatitis. Inflammation is an important feature of exocrine pancreatic diseases (Gukovsky et al., 2013). The deterioration or restoration of inflammatory responses and tissue homeostasis relies on coordinated interactions between macrophages, neutrophils, and other types of immune cells, which are controlled by secretory mediators including cytokines and chemokines (Gukovskaya et al., 2017). M1 macrophages, which are proinflammatory cells, can be polarized by LPS and produce proinflammatory cytokines such as IL-6, IL-1β, and TNF-α, and the LPS-induced immune response is mediated through the NF-κB signaling pathway (Islam et al., 2018; Shapouri-Moghaddam et al., 2018). NF-κB signals can be involved in inflammatory responses by activating stimulators (tumor necrosis factor, virus, lymphoid, etc.) to promote the expression of multiple genes and increase cytokine secretion (Hayden et al., 2006). In our study, BDE-47 caused a significant increase in the release of IL-6 and TNF-α from BMDMs when exposed to LPS. This result is consistent with the findings of Mynster Kronborg et al. (2016), who demonstrated that DE-71 increased the secretion of TNF-α, IL-6, IL-1β, and IL-8, thereby enhancing the proinflammatory response of macrophages upon LPS stimulation. The progression of pancreatitis is related to further increases in TNF-α, IL-6, and other inflammatory cytokines and chemokines. These cells recruit other inflammatory cells and amplify signals to promote local and extrapancreatic multi-organ damage (Gukovsky et al., 2013, 2017). These findings suggest that BDE-47 can exacerbate pancreatitis by inducing the secretion of inflammatory cytokines in macrophages, leading to an imbalance in inflammatory networks.
BDE-47 did not promote the transformation of pancreatic stellate cells into myoblasts in vitro. In animal models of pancreatitis, macrophages are commonly detected in the vicinity of fibrotic regions (Xue et al., 2015). LPS-activated macrophages produce cytokines that activate pancreatic stellate cells and promote collagen and fibrin synthesis in cultured pancreatic stellate cells (Gukovskaya et al., 2017; Schmid-Kotsas et al., 1999). Our in vivo experiments showed that chronic exposure to BDE-47 aggravated pancreatic fibrosis. However, when TGF-β was coadministered with BDE-47 in vitro, it resulted in decreased cell viability of fibroblasts and did not exacerbate the transformation of stellate cells into myoblasts. Furthermore, when cell death or inflammation occurs in an organ in the body, macrophages first exhibit the M1 phenotype and release TNF-α, IL-1β, IL-12, and other countermeasures. However, if the M1 phase continues, it can lead to tissue damage. Therefore, M2 macrophages secrete substantial quantities of TGF-β and IL-10 to suppress inflammation and facilitate tissue repair and remodeling (Shapouri-Moghaddam et al., 2018). Our results confirmed that BDE-47 can enhance the proinflammatory response of M1 macrophages, but it has no obvious effect on M2 macrophages. We speculate that BDE-47 exposure aggravates pancreatitis by augmenting the M1 macrophage-mediated inflammatory response.
Chronic exposure to BDE-47 promotes apoptosis of pancreatic acinar cells and aggravates tissue damage in pancreatitis. Pancreatic acinar cell injury, parenchymal necrosis and inflammation are the main pathological features of pancreatitis (Gukovskaya et al., 2017). Oxidative stress is one of the main pathogenic factors of AP (Abdelzaher et al., 2021). Oxygen free radicals produced during AP cause not only acinar cell damage but also pancreatic damage (Affourtit et al., 2011). In our study, BDE-47 promoted apoptosis of pancreatic acinar cells and expression of p-PERK, ATF4, CHOP, and p-JNK. ATF4 controls the expression of a wide range of adaptive genes that allow cells to withstand periods of stress, such as hypoxia or amino acid restriction. Under continuous stress, ATF4 promotes the induction of apoptosis (Wortel et al., 2017). Meanwhile, CHOP is also a key factor in the conversion of prosurvival signals to prodeath signals in the PERK signaling pathway, and the induction of CHOP promotes cell apoptosis (Li et al., 2020). In addition, JNK signaling plays a controlling role in a variety of cellular processes, including proliferation, development, and apoptosis, and can be activated by endoplasmic reticulum stress (Zhao et al., 2021). When we treated with the cells with the PERK inhibitor GSK2606414 and JNK inhibitor SP600125, we found that they both mitigated BDE-47 and cearulein-induced acinar cell death. Our findings resemble the mechanism of placental toxicity of PBDEs found by Zhao et al. (2022) in which BDE-209 can induce placental damage through the PERK/ATF4/CHOP signaling pathway mediated by endoplasmic reticulum stress. Li et al. discovered that exposure to BDE-47 during perinatal pregnancy can induce apoptosis through endoplasmic reticulum stress and lead to maternal thyroid toxicity. In addition, our molecular docking prediction analysis also identified ATF4, BAX, and BCL2 as potential targets of BDE-47-induced endoplasmic reticulum stress. These results suggest that BDE-47 can aggravate the course of pancreatitis through endoplasmic reticulum stress, promoting the apoptotic pathway.
In conclusion, we demonstrated that chronic exposure to BDE-47 aggravates the development of AP and CP by promoting acinar cell apoptosis and the inflammatory response of macrophages. The findings of this study suggest that health risks, including pancreatic toxicity, should be thoroughly considered for some occupational populations working in workshops with high levels of BDE-47 over a long period of time.
Supplementary data
Supplementary data are available at Toxicological Sciences online.
Author contributions
K.L. and J.H. designed the research and performed writing—review & editing. X.Q. and Q.L. performed the methodology, data curation, and writing—original draft. S.X. and L.Y. proposed the conceptualization and was responsible for supervision and project administration. Z.W., X.H., Y.J., and Y.S. were responsible for assistant experiments and resources.
Declaration of conflicting interests
The authors declared no potential conflicts of interest with respect to the research, authorship, and/or publication of this article.
Funding
Hunan Provincial Health Commission (202204014332); Hunan Provincial Natural Science Foundation (2022JJ40721).
References
Author notes
Xiaoyan Qi and Qiong Liu contributed equally to this study.
Comments