-
PDF
- Split View
-
Views
-
Cite
Cite
David Scieszka, Russell Hunter, Jessica Begay, Marsha Bitsui, Yan Lin, Joseph Galewsky, Masako Morishita, Zachary Klaver, James Wagner, Jack R Harkema, Guy Herbert, Selita Lucas, Charlotte McVeigh, Alicia Bolt, Barry Bleske, Christopher G Canal, Ekaterina Mostovenko, Andrew K Ottens, Haiwei Gu, Matthew J Campen, Shahani Noor, Neuroinflammatory and Neurometabolomic Consequences From Inhaled Wildfire Smoke-Derived Particulate Matter in the Western United States, Toxicological Sciences, Volume 186, Issue 1, March 2022, Pages 149–162, https://doi.org/10.1093/toxsci/kfab147
- Share Icon Share
Abstract
Utilizing a mobile laboratory located >300 km away from wildfire smoke (WFS) sources, this study examined the systemic immune response profile, with a focus on neuroinflammatory and neurometabolomic consequences, resulting from inhalation exposure to naturally occurring wildfires in California, Arizona, and Washington in 2020. After a 20-day (4 h/day) exposure period in a mobile laboratory stationed in New Mexico, WFS-derived particulate matter (WFPM) inhalation resulted in significant neuroinflammation while immune activity in the peripheral (lung, bone marrow) appeared to be resolved in C57BL/6 mice. Importantly, WFPM exposure increased cerebrovascular endothelial cell activation and expression of adhesion molecules (VCAM-1 and ICAM-1) in addition to increased glial activation and peripheral immune cell infiltration into the brain. Flow cytometry analysis revealed proinflammatory phenotypes of microglia and peripheral immune subsets in the brain of WFPM-exposed mice. Interestingly, endothelial cell neuroimmune activity was differentially associated with levels of PECAM-1 expression, suggesting that subsets of cerebrovascular endothelial cells were transitioning to resolution of inflammation following the 20-day exposure. Neurometabolites related to protection against aging, such as NAD+ and taurine, were decreased by WFPM exposure. Additionally, increased pathological amyloid-beta protein accumulation, a hallmark of neurodegeneration, was observed. Neuroinflammation, together with decreased levels of key neurometabolites, reflect a cluster of outcomes with important implications in priming inflammaging and aging-related neurodegenerative phenotypes.
Wildfire smoke (WFS) exposure poses an increasing threat to a growing and aging global population. A demonstrable increase in the extent of wildfires and resultant damage has been documented in the United States over the past 15 years, an effect which correlates well with changing global temperatures (Reid et al., 2016a). In 2020, wildfires from the west coast generated smoke that engulfed much of the United States in high concentrations of particulate matter (PM), potentially impacting the health and well-being of 100s of millions of citizens for several months.
Wildfires release high concentrations of airborne particulates and gases derived from varying biomass and anthropogenic fuels (eg, buildings, vehicles, etc.) that create unfamiliar and toxic exposures to populations far away from the source. Both PM and carbon monoxide are stable components of WFS that can travel thousands of miles. Even at a distance, WFS exposures increase acute respiratory hospital admissions (Rappold et al., 2012; Reid et al., 2016b; Tinling et al., 2016; Wettstein et al., 2018). Additionally, underserved minority populations, including Native American communities, are disproportionately vulnerable to wildfire events (Davies et al., 2018).
Wildfires produce complex mixtures of inhaled toxicants that can not only damage the lungs but also promote systemic health effects. Although most gaseous products remain localized, PM from WFPM is carried far distances across the continent by prevailing winds. Short- and long-term pulmonary, cardiovascular, and neurological outcomes from inhaled ambient PM are well studied; however, relatively little is known about the impact of WFS-derived PM on neurological outcomes—including implications for priming age-related disease sequelae. Ambient PM2.5 (PM less than 2.5 microns in aerodynamic diameter) has been associated with increased incidence of Alzheimer’s disease and related disorders (ADRD), suicide, depression, psychosis, and other adverse neurological outcomes (Braithwaite et al., 2019; Cacciottolo et al., 2017; Iaccarino et al., 2021; Younan et al., 2020). Impairment of blood brain barrier (BBB) and neuroinflammation have been implicated in pathophysiology of these neurological disorders (Cai et al., 2018; Castano Barrios et al., 2021). More recently, neurometabolite alterations in relation to neuroinflammation are being recognized as key drivers underlying cellular senescence and neurodegenerative disorders (Jakaria et al., 2019; Lautrup et al., 2019; Oh et al., 2020).
Prior studies suggest that inhaled PM induces proteolytic activity in the lung, leading to shedding of endogenous peptide fragments into the circulation that are bioactive and promote systemic endothelial inflammatory responses (Aragon et al., 2017; Mostovenko et al., 2019, 2021). The BBB consists of tightly connected brain endothelial cells contouring the vasculature of the central nervous system (CNS), with astrocytic end feet surrounding this vasculature, and nearby microglia capable of monitoring local events. Trafficking of leukocytes is heavily regulated by the BBB (Engelhardt et al., 2017). The serum-borne, endogenously released peptides from inhaled toxicants are thought to act through ligand-receptor endothelial interactions to upregulate adhesion molecules such as intercellular adhesion molecule (ICAM)-1 and the leukocyte chemoattractant CCL2 that may facilitate peripheral leukocyte transmigration into the CNS. CCL2 signaling activates the transmembrane adhesion molecule, leukocyte function-associated antigen-1 (LFA-1) on peripheral immune cells allowing them to interact with endothelial ICAM-1. Similarly, endothelial vascular cell adhesion molecule (VCAM)-1 interactions may play critical roles in regulating peripheral immune access to the CNS, and also mediating neuroinflammatory consequences of aging-related proteins in blood (Yousef et al., 2019). Endothelial cell activation and interaction with peripheral leukocytes may therefore initiate the neuroinflammatory events following environmental exposures, such as WFS, leading to reactive astrogliosis, inflammation, and neurodegenerative phenotypes.
The present study exploited naturally occurring wildfires in California, Arizona, and Washington in October of 2020 to examine the neuroinflammatory consequences of WFS-derived PM at a site >300 km away from the source. PM concentrations used in this study, due to the distant site and dilution factor, reflect a level lower than that experienced by tens of millions of people living in California, Oregon, and Washington during these wildfire events in 2020. Hallmarks of aging- and ADRD-related neurodegeneration, such as metabolite alterations, microglial activation, and pathological β-amyloid accumulation, were examined to gather supportive evidence as to the in vivo relevance of WFPM exposure as an unrecognized contributor to neurological aging and degenerative sequelae.
MATERIALS AND METHODS
Animals and exposures
Male C57BL/6 mice (Jackson Labs) at 2 months of age were housed in quarantine for 1 week prior to transport to the mobile laboratory located in Paguate, New Mexico (35°08′07.7″N 107°22′34.4″ W; Figure 1A). Mice were housed in AAALAC-approved facilities, on a 12-h light: dark cycle and provided a standard chow diet and water ad libitum. Mice were transported to the mobile laboratory 3 days prior to exposure initiation so that they could be acclimated to the chamber conditions. A total of 24 mice were used, randomly and evenly divided into filtered air (FA) control and concentrated PM2.5 exposed groups; 6 mice per reusable plastic animal case system (EF9614G, 2154F400SU). All procedures were conducted with approval by the University of New Mexico Animal Care and Use Committee.
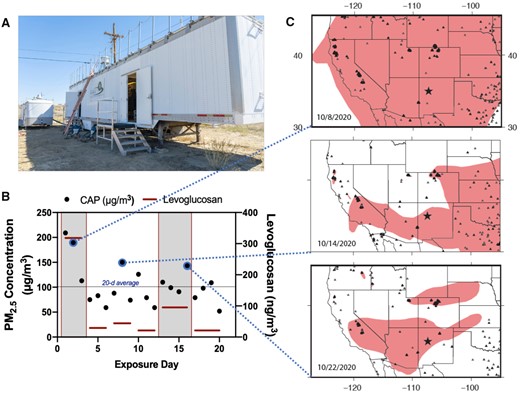
Exposure to concentrated WFPM particulates in Paguate, New Mexico. A, Mobile laboratory containing rodent exposure chambers and a Harvard-type particle concentrator. B, Daily PM2.5 gravimetric mass concentrations for the 20 days of exposure (black dots), along with levoglucosan measures (red bars) that span 3–4 days, as filters were pooled to provide sufficient material. C, Smoke and wildfire locations for 3 representative days in October 2020 derived from the National Oceanic and Atmospheric Administration Hazard Mapping System Fire and Smoke Product (Ruminski et al., 2006, 2007; Ruminski and Kondragunta, 2006), with dashed lines indicating the link to exposure days in (B). The black star indicates the location of the mobile laboratory in Paguate, New Mexico. Smoke plumes shown in pink were derived manually from geostationary and polar-orbiting satellites and represent a minimum smoke PM2.5 concentration of 5 µg/m3. Fire locations shown by triangles were obtained with automated fire detection algorithms; the nearest fire (on the border of Arizona and New Mexico) was over 300 km away from the mobile laboratory (Ruminski et al., 2006).
Exposures in the mobile laboratory were conducted in custom Hinners chambers with wire-bottom mouse cages; water was available to mice throughout the exposures, but food was withheld (4 h/day). PM2.5 concentration was facilitated by a Harvard-type concentrator and mice were exposed whole body for 4 h per day for 20 consecutive days (Sun et al., 2013). Exposures began on October 6, 2020, and the final day was October 25, 2020. After the last round of exposures, mice were transported back to the UNM laboratories, randomized, and euthanized (blinded) the following day for tissue collection.
Particulate matter characterization
Exposure concentrations were measured in real-time from a tube that leads directly into the exposure chamber with a DustTrak II (TSI, Inc; Shoreview, Minnesota), and 47 mm quartz filter weights collected for the duration of each daily exposure were used to gravimetrically confirm final daily averages. Filter samples were processed and analyzed in Class 100 clean rooms at the Michigan State University Exposure Science Laboratory. The samples were analyzed gravimetrically for particle mass using a microbalance (XPR6UD5, Mettler Toledo) in a temperature- and humidity-controlled clean laboratory as described in the Federal Reference Method (Agency USEP, 1997). PM samples collected on quartz filters were maintained at −40°C after sampling and were analyzed for carbonaceous aerosols (organic carbon and elemental carbon) by a thermal-optical analyzer (Sunset Laboratory) using the NIOSH 5040 method.
Particle samples collected on Teflon filters were wetted with ethanol and extracted in 1% nitric acid solution. The extraction solution was sonicated for 48 h in an ultrasonic bath, and then allowed to passively acid digest for 2 weeks. Extracts were then analyzed for 20 trace elements using high-resolution inductively coupled plasma-mass spectrometry (ELEMENT2, Thermo Finnigan). This analysis method incorporated daily quality assurance and quality control measures including field blanks, Type I water blanks, replicate analyses, and external standards as described (Harkema et al., 2009).
Quantification of levoglucosan was performed in the MSU Mass Spectrometry and Metabolomics Core. The details of sample extraction and analysis are described elsewhere (Simpson et al., 2004). In brief, for each sample, PM from approximately 100 mg of filter punches was extracted with 3 ml methanol (MeOH) containing 2 µg/ml sedoheptulose anhydride as an internal standard (Millipore Sigma). One milliliter of the methanolic extract was dried down and derivatized with 100 µl of N-trimethylsilylimidazole (Millipore Sigma). One milliliter of the derivatized samples in splitless mode was analyzed using an Agilent 7890A GC/single quadrupole mass spectrometer with 5975C inert XL MSD. The mass spectrometer was operated using 70 eV electron ionization in selected ion monitoring mode for m/z 204 and 333. Quantification of levoglucosan, 3 TMS derivative were based on a levoglucosan (Millipore Sigma) standard curve.
Lung lavage, bone marrow, cytokines, and histopathology
Following euthanasia, lungs were lavaged with phosphate-buffered saline (PBS) as described previously (Tyler et al., 2018). Total cell counts and differentials were conducted in a blinded manner. For bone marrow extraction, bone marrow was flushed from hind limb femur and tibia bones as described previously (Bolt et al., 2016). Briefly, hind legs were dissected at the hip and femur, and tibia bones were cleaned to remove residual fur and tissue. Subsequently, both ends of each bone were clipped off before being placed into the same 0.5-ml microcentrifuge tube with a hole poked in the bottom with an 18G needle. The 0.5-ml tube was nested into a 1.5-ml microcentrifuge tube and bone marrow was centrifuged out of the bones into the 1.5-ml Eppendorf tube at 10 000 × g for 15 s. Cell pellets were resuspended in cell freezing media (FBS, 5% DMSO) for long-term storage before subsequent thawing, cell lysis, and cytokine measurements. Cytokines were measured in BALF and bone marrow using an electrochemiluminescent assay (MesoScale Discovery, V-PLEX Proinflammatory Panel 1 and Cytokine Panel 1 Mouse Kits), according to the manufacturer’s instructions. Lungs were either perfusion fixed at 25 cmH2O in 4% paraformaldehyde for histology (N = 6/group) or frozen in liquid nitrogen. Transverse-oriented serial sections, 2–3 mm, were excised from the fixed left lung lobe, embedded in paraffin, and 5–6 μm thick sections cut from the anterior surface. Lung sections were stained with hematoxylin and eosin (H&E) for light microscopic examination by a board-certified veterinary pathologist (JRH) to determine exposure-related changes.
Echocardiography
Before transport to the mobile exposure laboratory and after exposures just prior to euthanasia, mice were briefly anesthetized with isoflurane for Doppler ultrasound assessment of cardiac function (Vevo LAZR-X, FUJIFILM VisualSonics, ref: 52886-15). Anesthesia was maintained at approximately 1%, and fur was removed by Nair. We targeted heart rate values above 400 beats per minute and acquired for 1 min per mouse under blinded conditions while in supine position. Short-axis M-mode images were collected on all mice for assessment of cardiac output, ejection fraction, end-systolic dimensions, and end-diastolic dimensions. Values were obtained from 3 tracings of 3–5 consecutive beats (between breaths) per mouse. All analysis was blinded.
Brain tissue digestion for flow cytometry
Under isoflurane anesthesia, all 24 mice underwent transcardial ice-cold 0.1 M PBS (pH = 7.4) perfusion. Brains were dissected and preserved in liquid nitrogen for various assays; distribution of samples is shown in Supplementary Table 1. One half-brain hemispheres (right side) from 6 mice per group were harvested in ice-cold HBSS buffer and processed immediately according to Miltenyi gentleMACS adult neural tissue digestion protocol, as described (Tyler et al., 2018). Briefly, brain tissues were minced with fine tip scissors and processed with enzymatic and mechanical digestion steps with gentleMACS Octo dissociator with heaters (Miltenyi Biotec, California). The other dissected hemisphere was flash frozen in liquid N2. Dissections included hippocampus, prefrontal cortex, cerebellum, and “remaining brain material.” Twelve remaining brain material tubes were used for subsequent amyloid beta ELISA (n = 6 per group). Five hippocampus and neocortex from these mice were lost in shipping due to winter ice storms. Following digestion steps on original half-brains, cell suspensions were passed through 70 μm cell strainers. Myelin debris were removed with Debris removal solution, used according to the manufacturer’s protocol. Cells were resuspended in PBS (without calcium and magnesium, Sigma-Aldrich, St Louis, Missouri) and kept on ice until proceeding to viability dye staining.
Cell staining for surface and intracellular antigens for flow cytometry
For flow cytometry analysis, live cells were counted on a hemocytometer using trypan blue staining exclusion criteria. Between 0.2 and 1 × 106 cells were transferred in flow-assisted cell sorting (FACs) tubes, stained with Viability dye eFlour 450 (eBioscience, San Diego, California) for 30 min. After a wash with FACs buffer (1× PBS containing 0.5% bovine serum albumin and 1 mM EDTA) and incubation with a saturating solution of Fc block (BD Biosciences, San Jose, California), cells were stained for fluorochrome-conjugated antibodies for surface antigens for 25 min in the dark on ice. Antibodies against mouse CD11b, CD45, MHC-II, and CD31 were all purchased from Themo Fisher Scientific, Massachusetts and were used as 0.125–0.5 µg/106 cells, as recommended by the manufacturer. Cells were examined for intracellular levels of various proinflammatory factors: tumor necrosis factor, TNFα, CCL2, and inducible nitric oxide synthase, iNOS. For intracellular staining, cells were fixed with Fixation buffer and then permeabilized using intracelullar fixation and permeabilization buffer set (eBioscience). Cells were then stained with fluorochrome-conjugated antibodies for the intracellular immune factors for another 1 h at room temperature in the dark. After another wash with 1× permeabilization buffer, cells were resuspended in 250–300 µl FACs buffer and immediately proceeded for data acquisition. At least 50 000 live cell events were collected for each sample. Single-stained controls and isotype controls were used for laser compensation and data analysis. Data were acquired using the BD LSR Fortessa cell analyzer (BD Biosciences, San Jose, California) and analyzed using Flow Jo software v10.7.1.
Flow cytometry gating strategy
The gating strategy for determining different cell subsets in the brain tissues is similar described in our prior report (Tyler et al., 2018). Briefly, doublets (cell clumps) were excluded, and the live cells were identified based on their size, granularity (FSC vs SSC) and negative viability dye staining. Cerebrovascular endothelial cells were identified based on negative expression of the common leukocyte marker, CD45, and positive staining for CD31 (PECAM-1, platelet endothelial cell adhesion molecule-1). All CD45+ cells were first gated for PMN or neutrophil marker, Ly6G (1A8) staining, which were also verified by their positive CD11b staining. The population of CD45+1A8– (leukocytes that are not neutrophils) were further analyzed to identify microglia with low or medium expression of CD45 (CD45low/med, CD11b+) as distinguished from infiltrating macrophages/monocytes with CD45high expression (CD45high CD11b+). Additionally, infiltrating monocytes/macrophages were further analyzed for Ly6C expression to identify inflammatory monocytes (1A8–CD11b+CD45high Ly6C+). Median or geometric mean fluorescent intensities were plotted for activation markers or cytokine expression on these different immune and endothelial cell subsets.
Metabolomics tissue preparation
Briefly, each cerebellum sample (∼20 mg, n = 6) was homogenized in 200 µl MeOH: PBS (4:1, v:v, containing 1810.5 μM 13C3-lactate and 142 μM 13C5-glutamic acid) in an Eppendorf tube using a Bullet Blender homogenizer (Next Advance, Averill Park, New York). Then 800 µl MeOH:PBS (4:1, v:v, containing 1810.5 μM 13C3-lactate and 142 μM 13C5-glutamic acid) was added and, after vortexing for 10 s, the samples were stored at −20°C for 30 min. The samples were then sonicated in an ice bath for 30 min. The samples were centrifuged at 14 000 RPM for 10 min (4°C), and 800 µl of supernatant was transferred to a new Eppendorf tube. The samples were then dried under vacuum using a CentriVap Concentrator (Labconco, Fort Scott, Kansas). Prior to mass spectrometry analysis, the obtained residue was reconstituted in 150 μl 40% PBS/60% ACN. A quality control sample was pooled from all the study samples.
Liquid chromatography-tandem mass spectrometry
Targeted liquid chromatography-tandem mass spectrometry (LC-MS/MS) techniques were similar to several recent reports (Carroll et al., 2015; Eghlimi et al., 2020; Gu et al., 2015, 2016; Jasbi et al., 2019; Shi et al., 2019). Briefly, LC-MS/MS experiments were performed on an Agilent 1290 UPLC-6490 QQQ-MS (Santa Clara, California) system. Each cerebellum sample was injected twice: first a 10-µl volume for analysis using negative ionization mode and second a 4-µl volume for analysis using positive ionization mode. Both chromatographic separations were performed in hydrophilic interaction chromatography (HILIC) mode on a Waters XBridge BEH Amide column (150 × 2.1 mm, 2.5 µm particle size, Waters Corporation, Milford, Massachusetts). The flow rate was 0.3 ml/min, autosampler temperature was kept at 4°C, and the column compartment was set at 40°C. The mobile phase was composed of Solvents A (10 mM ammonium acetate, 10 mM ammonium hydroxide in 95% H2O/5% acetonitrile [ACN]) and B (10 mM ammonium acetate, 10 mM ammonium hydroxide in 95% ACN/5% H2O). After the initial 1 min isocratic elution of 90% B, the percentage of Solvent B decreased to 40% at t = 11 min. The composition of Solvent B maintained at 40% for 4 min (t = 15 min), and then the percentage of B gradually went back to 90%, to prepare for the next injection.
The mass spectrometer is equipped with an electrospray ionization (ESI) source. Targeted data acquisition was performed in multiple reaction monitoring (MRM) mode. The whole LC-MS system was controlled by Agilent Masshunter Workstation software (Santa Clara, California). The extracted MRM peaks were integrated using Agilent MassHunter Quantitative Data Analysis (Santa Clara, California). ACN, MeOH, ammonium acetate, and acetic acid, all LC-MS grade, were purchased from Fisher Scientific (Pittsburgh, Pennsylvania). Ammonium hydroxide was bought from Sigma-Aldrich (St Louis, Missouri). Standard compounds corresponding to the measured metabolites were purchased from Sigma-Aldrich and Fisher Scientific.
Immunofluorescence microscopy
The right hemisphere of each fresh-frozen mouse brain was cryosectioned (10 µm thick, n = 4) along the sagittal plan starting 1.5 mm from the lateral aspect. Brains from APP/PS1 mice (Jackson Labs) with mutant human amyloid precursor protein and presenilin 1 on a C57BL/6 background were used as a positive control for amyloid pathology. Sections were collected on charged slides, air dried and then fixed with 4% paraformaldehyde. Sections were blocked and permeabilized in 5% normal goat serum and 0.1% Triton X-100 for 1 h at room temperature. Sections were then probed at 4°C overnight with primary antibodies in blocking buffer: vascular marker anti-rat zonula occludens 1 (ZO1, Developmental Studies Hybridoma Bank, Iowa City, Iowa; R26.4C, 1:5), astrocytic marker anti-chicken glial fibrillary acidic protein (GFAP, Abcam, Cambridge, Massachusetts; ab4674, 1:2500), amyloid pathology marker anti-mouse Aβ-42 (Thermo Scientific, MA5-36246, 1:500), early neurodegenerative disease marker anti-rabbit Sorcin (Thermo Scientific, PA5-95611, 1:500), microglial marker anti-guinea pig ionizing calcium binding adaptor molecule 1 (IBA1, Synaptic Systems, Goettingen, Germany; 234-004, 1:2000), and BBB permeation marker anti-rabbit albumin (Bioss, bs-2256R, 1:300). Sections were then probed with corresponding AlexaFluor 488/568/680/750-conjugated goat secondary antibodies at room temperature for 2 h. Mounted sections were cover slipped in ProLong Diamond Antifade Mountant (Thermo Scientific). Slides were imaged using constant exposure and illumination settings optimized per channel via multichannel immunofluorescence with a Zeiss AxioImager M2 microscope with structured illumination and optical sectioning (0.5-μm stack thickness). Image acquisition and visualization were performed with Zeiss Zen 2.1 software with constant gray level settings per channel across all sections.
Amyloid beta 42 ELISA
Soluble toxic Aβ-42 was assessed in remaining brain material (Supplementary Table 1) tissue homogenate samples via ELISA with the Human/rat β-amyloid (Aβ-42) ELISA kit (Wako Pure Chemical Industries, 290-62601, Richmond, Virginia), according to the manufacturer’s instructions. Total sample protein was acquired by performing a BCA with the Pierce BCA Protein Assay Kit (Thermo Scientific, 23225). Resultant Aβ peptide content was normalized to total sample protein content, tested for normal distribution via Shapiro-Wilk before log2 correcting for right-tailed distribution.
Brain tissue preparation for cytokine/chemokine measurements
Left hippocampi and prefrontal cortices (n = 5, nonoverlapping with brains from flow cytometry) were thawed and processed for protein content using a common RIPA buffer and extraction protocol. Specifically, 500 µl RIPA lysis buffer (150 mM NaCl, 50 mM Tris, 1% Triton X-100, 0.5% sodium deoxycholate, 0.1% sodium dodecyl sulfate, protease inhibitor added directly before assaying) was added to homogenization tubes with 20 homogenizing beads (1.4 mm zirconium ceramic oxide beads, Omni International, cat. no. 196453). Brain tissue was homogenized (Roche MagNA Lyser) at 6000 rpm for 60 s. Cell content was briefly centrifuged to reduce bubbling, 500 µl RIPA was added, and homogenization was repeated. Tubes were agitated at 4°C for 2 h before centrifugation at 16 000 × g for 20 min at 4°C. Resultant protein-rich supernatant was used for chemokine and cytokine measurements.
Statistics
Normality was determined via Shapiro-Wilk testing. In the even or normally distributed data, student’s t test was used. For nonnormally distributed data, t tests were employed on log2() transformed data based on right-tailed distribution. Pearson’s correlation was performed on PM elemental composition. Tests were either conducted in GraphPad Prism v9.1.1 or Rstudio v1.4.1564.
RESULTS
Exposure Characterization
The mobile laboratory-based concentration of PM2.5 led to 4-h exposure levels averaging 104 µg/m3 across the 20-day period (Figure 1B). During the peak of WFS transported to this region, high concentrations of 209 and 191 µg/m3 were seen (first 2 days of exposure). We also measured the overall content of levoglucosan, a specific marker for woodsmoke, in pooled PM filter samples, which revealed that the first 3 days of exposure had a high contribution of wood burning in the PM2.5. Increased levoglucosan levels were also evident on exposure days 13–16. Potassium levels in the WFPM appeared elevated on days with greater wildfire contributions (Supplementary Table 2) and correlations among K, Mg, and Mn were noted, consistent with previous studies of wildfire PM composition (Supplementary Figure 1) (Verma et al., 2009). Modeling of wind trajectories for the initial period of the exposure identified a strong likelihood that woodburning-derived PM originated in southern California (Figure 1C). Geospatial analysis of the western United States revealed extensive smoke distribution during the exposure period. Additional contributing wildfires were present in Colorado, Washington, and Arizona. Importantly, mice were only exposed for 4 h a day, so that the 24 h average PM2.5 exposure could be estimated as much lower, potentially <20 µg/m3 (Supplementary Table 2).
Pulmonary and Systemic Responses
Histological assessment of lungs revealed a modest increase in the macrophage population in WFPM-exposed mice compared with FA (Figs. 2A and 2B). This was confirmed quantitatively with cells recovered in the BALF increased 4-fold in WFPM- versus FA-exposed mice, principally due to macrophage elevations (Figure 2C). The numbers of neutrophils were not elevated by WFPM exposure (Supplementary Table 3). However, neutrophil recruitment cytokines IL-17, MIP1, and MIP2 were statistically elevated (Figure 2D), whereas TNFα and other conventional inflammatory mediators were unaltered (Supplementary Table 3). The absence of neutrophils suggests that any acute pulmonary inflammatory phase had subsided, with effector responses trending downward toward resolution. Additionally, the inflammatory cytokine IP10 was significantly elevated, indicating a persistent response in cellular subtypes of monocytes, T cells, NK cells, or eosinophils (Ahmadi et al., 2013). We questioned whether this inflammation was reflected systemically through bone marrow examinations. Interestingly, MIP2 and IP10 were elevated in the bone marrow of WFPM-exposed mice (Figure 2E). However, many other BALF, whole lung, regional brain tissue, and bone marrow inflammatory protein markers were unaltered by the 20-day WFPM exposure (Supplementary Tables 3–6), including KC/Gro, TNFα, and IL-6, confirming that the overall pulmonary inflammatory response to this exposure was expectedly modest, or resolving from the earlier higher exposure concentrations. Additionally, echocardiography was performed to assess cardiac function; however, no significant changes were noted between FA and WFPM mice after the 20-day exposure (Supplementary Table 7).

Significant pulmonary and bone marrow cytokine responses to 20-day exposure to wildfire smoke (WFPM). Light photomicrographs of hematoxylin and eosin-stained lung tissue from the left lung lobe of mice exposed to (A) filtered air (FA) or (B) concentrated fine ambient particles. Slightly more alveolar macrophages (arrows) were present in WFPM-exposed mice than FA control mice. Stippled arrow, particle laden-alveolar macrophage (black cytoplasmic material); solid arrow, alveolar macrophage without cytoplasmic particles; a, alveolus; ad, alveolar duct. (C) Airway macrophages in bronchoalveolar lavage fluid (n = 10) were significantly elevated after WFPM exposure. (D) Whole lung cytokine IL-17 (n = 6), and bronchoalveolar lavage cytokines (n = 10), MIP-1a, MIP-2, and IP-10 were significantly elevated by WFPM. (E) Bone marrow concentrations of MIP-2 and IP-10 were elevated by WFPM. Independent samples; two-tailed t test; mean and SEM shown, *p < .05, **p < .01, ***p < .005, ****p < .001. All measured cytokines are provided in Supplementary Tables 2–5.
Neuroinflammaging
We assayed prefrontal cortices and hippocampal regions for inflammatory proteins and cerebelli for aqueous metabolites including specific assessments for NAD and related metabolites. Cytokines from the hippocampus showed no statistical differences (Supplementary Table 5). However, prefrontal cortices showed a decrease in KC/Gro (Supplementary Table 5). WFPM-exposed mice displayed reduced NAD+, NADH, succinate, and taurine compared with FA-exposed mice (Figure 3A), all of which have important implications for aging (Jakaria et al., 2019; McReynolds et al., 2020; Oh et al., 2020). These aging-related metabolites prompted a direct examination of Aβ-42 levels, the more pathogenic peptide variant that aggregates faster, which was significantly increased in WFPM-exposed mouse half-brain homogenates (Figure 3A). Immunofluorescence of brain sections revealed the localization of increased Aβ-42 appeared proximal to cerebrovasculature together with greater staining for the early neurodegenerative disease marker sorcin (Genovese et al., 2020) as also found in matched staining of APP/PS1 model mouse brain (Figure 3C). Taken together, WFPM exposure—from a source >300 km away—gives rise to numerous markers of an accelerated inflammaging response with increased markers consistent with a neurodegenerative phenotype.
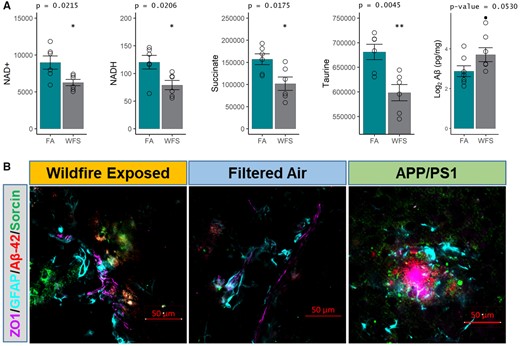
Markers related to aging and neurodegenerative disease pathogenesis. A, NAD+, NADH, succinate, and taurine were all downregulated (n = 6), and amyloid beta (Aβ, n = 5) levels were upregulated in WFPM-exposed mice (n = 6, cerebellum; n = 5, rest of brain material, log2() correction for normality; independent samples; 2-tailed t test; mean and SEM shown). B, Representative images of neurodegenerative pathogenic markers found at the neocortical neurovascular unit on the sagittal plane starting 1.5 mm from the lateral aspect in WFPM-exposed mice. Brain Aβ-42 (red) increases in WFPM-exposed mice were observed only proximal to the neurovascular unit (endothelial ZO1 and astrocyte GFAP). Additionally, the early pathogenic marker Sorcin increased (green), indicating ER stress/unfolded protein responses proximal to areas of Aβ-42 staining, which was also observed in unexposed APP/PS1 mice of the same age and background as a positive control, though they exhibited higher density Aβ-42 aggregation.
Given the proximity of these markers to the cerebrovasculature, we further wanted to assess indications of a neuroinflammatory response comparable with outcomes we have seen with other inhaled toxicant exposures (Aragon et al., 2017; Mostovenko et al., 2021; Tyler et al., 2018). Phenotypically, the neurovascular unit appeared similar to that previously seen after pulmonary particulate exposure, with extravascular albumin staining, increased GFAP process volume and greater IBA1 localization to indicate a reactive, neuroinflammatory state in WFPM-exposed mice (Figure 4A). A neuroinflammatory state was affirmed with cytometric quantitation of microglia and CNS infiltrating peripheral immune cells (Supplementary Figure 2: gating strategy). An increase of microglial frequency along with their surface expression of CD45 were significantly elevated in WFPM-exposed brains compared with FA controls (Figure 4B). Moreover, intracellular levels of proinflammatory proteins CCL2 and iNOS were increased in microglia after WFPM exposure (Figure 4B). We validated microglial activation by measuring elevated surface expression of ICAM-1 and iNOS, though the increasing trend in TNFα expression did not reach significance (Figure 4B).
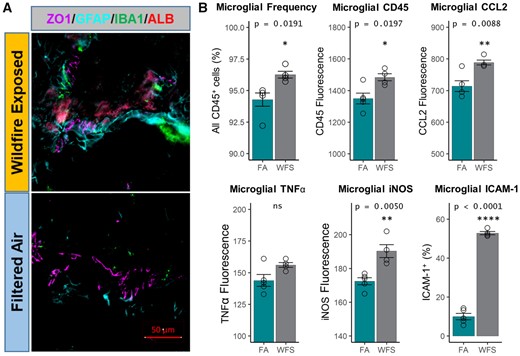
Microglial activation following WFPM. A, Imaging of the neurovascular unit on the sagittal plane starting 1.5 mm from the lateral aspect reflected similar pathology as reported with other pollutants previously with more pronounced GFAP (cyan) staining and a greater presence of IBA1+ (green) microglia/macrophages adjacent to large-diameter (>20 µm) cerebrovasculature (ZO1+, purple), along with an increased staining in parenchymal albumin (red) in WFPM-exposed relative to FA control (right hemisphere). B, Percentage of microglia and their surface expression levels of CD45, adhesion molecule ICAM-1 and intracellular levels of proinflammatory factors, iNOS, TNFα, and CCL2 were analyzed, n = 3–5 independent samples, left hemisphere homogenate; 2-tailed t test; mean and SEM shown.
Given peripheral immune reactivity (Figure 2C), infiltration of peripheral immune cells into the CNS was also examined by cytometric analysis of the perfused brains. Contrary to the BALF, an increased influx of mature (1A8high) neutrophils was detected in WFPM-exposed brains, displaying significantly higher levels of CD11b and MHC-II expression (Figure 5A). The marker CD45high includes various subsets of peripheral leukocytes, and no changes were observed on this macro level. However, further analysis of a CD45highCd11b+ subset revealed significant increases in CNS-infiltrating Ly6C+ inflammatory monocytes in the brain, suggesting an influx of these cells due to WFPM exposure (Figure 5B). Furthermore, there were significant increases in CD45highLFA-1+ and CD45highICAM-1+ peripheral macrophages that exhibited a greater expression of MHC-II in WFPM-exposed brains (Figure 5C).
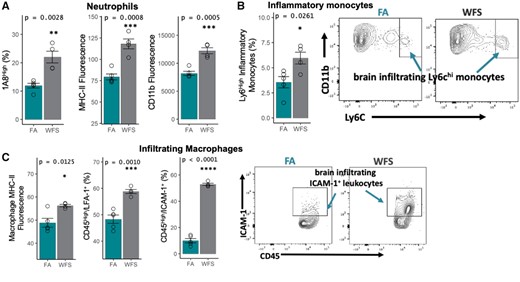
Infiltrating immune cells in the brain following WFPM exposure. A, Increased frequency of mature neutrophils (with high expression of 1A8), along with overall increase in MHC-II and CD11b surface expression of all CD45+1A8+ neutrophils in the brain due to WFPM exposure. B, Inflammatory monocytes in the brain were increased in frequency with WFPM exposure. C, CNS infiltrating CD45highCD11b+ macrophage/monocyte population shows increased MHC-II expression and frequency of LFA-1+ and ICAM-1+; peripheral leukocytes were also increased due to WFPM exposure, n = 4–5 independent samples, left hemisphere homogenate; 2-tailed t test; mean and SEM are shown.
Flow cytometry also revealed a complex phenotype among brain endothelial cells (CD31+/CD45−) following 20-day WFPM exposure, with subpopulations divided by their level of CD31 expression (Figure 6A). WFPM shifted CD31+/CD45− endothelial cells overall to a greater expression of CD31 (Figure 6) consistent with vascular repair and restoration of the BBB (Wimmer et al., 2019), with these prorepair CD31high/CD45− cells exhibiting reduced levels in most inflammatory markers (Figure 6C). At the same time, CD31med/CD45− cells retained their inflammatory profile after WFPM exposure with increases in CCL2, TNFα, iNOS, LFA-1, and VCAM-1 (Figure 6B).
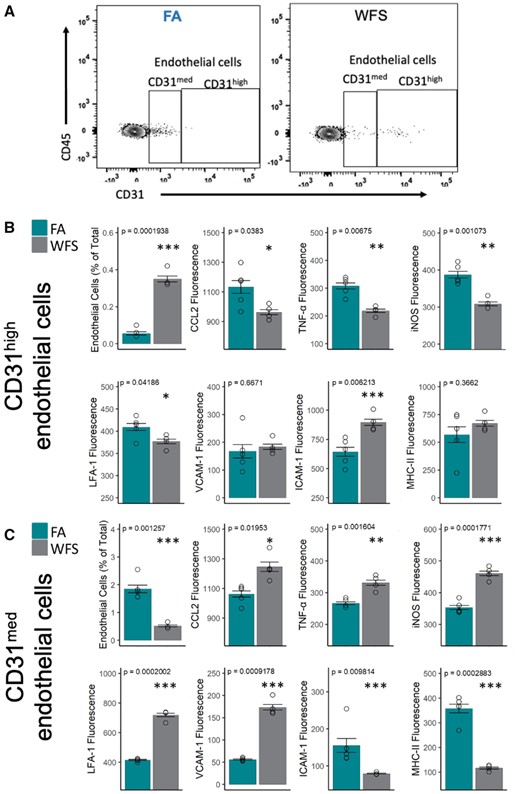
Cerebrovascular endothelial cells exhibit a heterogeneous inflammatory response to WFPM. A, Representative flow cytometry plots of endothelial cells (with medium and high expression of CD31/PECAM-1 on CD45− cells) in the brain in FA or WFPM exposure groups. These 2 subsets of endothelial cells displayed comparatively different neuroimmune activity profile, as detailed in (B) and (C). B, Frequency of endothelial cells with high expression of CD31 was increased following WFPM exposure; however, these endothelial cells displayed reduced levels of these proinflammatory factors. Increased frequency of ICAM-1 expressing endothelial cells was observed due to WFPM exposure in this endothelial subset despite no changes in MHC-II. C, Frequency of CD31med endothelial cells were reduced following WFPM exposure, however, these cells displayed increased proinflammatory phenotype such as increased levels of CCL2, TNFα, and iNOS, although reduced levels of ICAM-1 and MHC-II were observed, n = 4–5 independent samples, left hemisphere homogenate; 2-tailed t test; mean and SEM are shown.
DISCUSSION
The recent spate of wildfires around the globe have provoked concern that smoke exposures may promote vascular and, by extension, neurological consequences (Farugia et al., 2021; Schuller and Montrose, 2020). Although clear relationships between ambient air pollutants, such as PM2.5 and carbon monoxide, and increased incidence of ADRD have been reported (Cacciottolo et al., 2017; Iaccarino et al., 2021; Younan et al., 2020), there have been no specific assessments of WFS PM impacts on neuroinflammation or markers of ADRD. Findings of the present study confirm that the WFPM-derived PM2.5 traveling from distant wildfires can promote numerous outcomes consistent with ADRD pathogenesis and neurological aging.
Neuroinflammation and key reduced metabolites occurred in otherwise healthy mice exposed for 80 total hours of WFPM-derived PM2.5, including microglial activation and peripheral leukocyte invasion. The concentrations in our study averaged 104 µg/m3 for only 4 h per day of exposure. Few comparable studies have been published for comparison, but glial activation has been reported in several studies of diesel particulate exposure at much higher levels (200–1000 µg/m3) (Chen et al., 2021). Fresh diesel emissions at 500–2000 µg/m3 for 4 weeks induced clear signs of neuroinflammation in rats, in terms of Iba1 concentrations and other cytokine levels in brain homogenates (Levesque, Taetzsch et al., 2011). A parallel study of 6-month exposures found minimal signs of neuroinflammation at lower concentrations of diesel emissions (35, 100 µg/m3), but greater evidence of such responses at 300–1000µg/m3 (Levesque, Surace et al., 2011). Ambient urban nanoparticle (median diameter = 55 nm) exposure of 330 µg/m3 for 150 h led to inflammatory responses in the corpus callosum including microglial activation (Iba1 staining) and increased levels of complement C5 (Babadjouni et al., 2018). Thus, although neuroinflammation has previously been reported for other particulates, the robust nature of responses in the present study at such low PM concentrations implies a potent role for the wildfire PM contribution.
Findings of a 13-day wildfire episode in Washington state found 24 h average PM2.5 to be 96 µg/m3 (up from a baseline of 4.3 µg/m3) with 2 counties experiencing peak concentrations near 400 µg/m3 (Liu et al., 2020, 2021). During this period, we estimate that over 1.6 million residents of Washington State experienced 24 h exposures well in excess of 100 µg/m3 on average, and all 7 million people in the state were exposed to levels higher than our 24 h exposure average (Supplementary Figure 3). Although those people also spend a lot of time indoors, our exposures in mice were only for 4 h per day, possibly modeling that behavior. Similar exposures were seen for populations in Oregon and California. In addition to PM, carbon monoxide and potentially other gaseous pollutants can further contribute to neurological morbidity (Chang et al., 2014). Notably, the long distance from the source and method of PM2.5 fractionation used in our study would lead to disproportionately low gaseous pollutants; thus, this study does not address potential additivity of the total pollutant atmosphere.
The pathways leading from inhaled pollutants to neurological outcomes are being discerned through mechanistic toxicological studies. We have suggested that circulating bioactive peptide fragments arise from pulmonary matrix metalloproteinase activity following inhalation of a diverse array of pollutants, including PM and ozone (Aragon et al., 2016a, 2017; ,Robertson et al., 2013; Tyler et al., 2018). Endothelial VCAM-1 drives leukocyte invasion, which reduces BBB integrity and reactive consequences at the neurovascular unit; specific aging-related circulating factors may augment VCAM-1 signaling and promote BBB impairment (Yousef et al., 2019). Controlled exposures to woodsmoke caused a circulating bioactivity that induced endothelial VCAM-1 in ex vivo incubations, notably more potently than other emissions including diesel/gasoline emissions and ozone (Aragon et al., 2016b). The present findings from flow cytometric assessment of VCAM-1 expression in CD31med/CD45− cerebrovascular endothelial cells confirms the in vivo relevance of this effect, but notably at a remarkably low concentration of PM2.5. Identification of evoked circulating factors after WFS exposure and the endothelial receptor interactions is essential to understanding pathways that confer susceptibility among individuals. Furthermore, the apparent initial steps of BBB repair and inflammation resolution, seen as an increase in CD31high/CD45− cells with reduced iNOS, CCL2, and TNFα expression after 20 days of exposure, highlight another potential contributor to susceptibility, with genetic and lifestyle factors in the general population adding variability to the efficiency and quality of inflammatory resolution.
Endothelial cell responses are complex, with control and PM-exposed mice exhibiting relatively different levels of CD31, which drove the segregated analysis of CD31med versus CD31high. CD31 is not a passive, constitutive marker of endothelial cells in the brain, and it has been noted as a key player for maintaining BBB integrity (Wimmer et al., 2019) and neutrophil recruitment in inflamed brains (Winneberger et al., 2021). Using a bifurcated analysis strategy for the endothelial cell analysis, we illustrate a potentially dynamic system that is crucial to acclimation to environmental stressors. Cells highly expressing CD31 were also downregulating other canonical inflammatory pathways, including CCL2 and iNOS. Thus, we postulate that subchronic exposure to inhaled PM from WFPM likely leads to repair of the cerebrovascular endothelium, but little can be inferred from the present study design as to the timeframe of this resolution.
Despite the worst concentrations of the exposure occurring on the first 2 days of the 20-day exposure, there was little indication of resolution of microglial activation or the invading peripheral leukocytes. Peripheral immune cells were not only in greater numbers in the brains of WFPM-exposed mice, but they also expressed greater MHC-II protein on the surface, which is associated with the inflammatory response in neurodegenerative diseases (Schetters et al., 2017; Wyss-Coray and Mucke, 2002). Furthermore, peripheral immune cells expressed higher levels of inflammatory markers ICAM-1, iNOS, and CCL2 in WFPM-exposed mice. These proinflammatory immune cells may interact with microglia and astrocytes and further propagate neuroinflammation. Eventually these immune cells may help resolve inflammation or injury in the brain, but the resolution of this infiltration may require months (Schwartz and Baruch, 2014). Repeated insults may therefore confound beneficial outcomes or escalated other pathologies such as Alzheimer’s disease or multiple sclerosis (Schwartz and Baruch, 2014). Understanding the molecular and cellular pathways regulating the resolution, as well as the range of environmental and pathological stressors that promote inflammation is, again, a priority for understanding how susceptibility to neurodegenerative disease arises.
Environmental stressors, including air pollution, have been associated with neurodegenerative diseases (Chang et al., 2014; Oudin et al., 2018; Younan et al., 2020), and the present study details pathways that may be points of vulnerability for individuals with genetic predisposition for ADRD. Genetic heritability is actually quite variable across different syndromes of dementia. Frontotemporal dementia only has clear heritability evidence in up to 30% of cases, most of which can be explained by single gene mutations (eg, microtubule-associated protein tau and progranulin) (Greaves and Rohrer, 2019). Alzheimer’s disease has a much higher heritable basis, between 60% and 80% (Jansen et al., 2019). Thus, environmental factors and other pathologies may account for a substantial portion of the remaining incidence. Furthermore, even with the highly heritable Alzheimer’s disease, the age of onset may be influenced by environmental factors interactions (Gatz et al., 2006). The elevation of Aβ-42 seen after WFPM in mice is reminiscent of previous studies of acute ozone inhalation (Tyler et al., 2018). Aβ-42 is an early pathogenic indicator with aggregating potential in humans, but the existence of rodent Aβ-42 in response to air pollution is unclear as mice do not naturally form plaques (Patten et al., 2021). Aβ-42 may be elevated due to increased production or reduced clearance, possibly a repercussion from microglial diversion to the neurovascular unit. Regardless of the mechanism, impairing BBB, driving neuroinflammation and reducing key metabolites may be central to environmental contributions to ADRD incidence; the sensitivity of individuals to these effects and the efficiency of resolution may influence susceptibility among individuals.
Compromised metabolism is a major hallmark of cellular aging. Neurons and neuro-supportive cells undergo numerous changes with aging including reductions of protein quality control and lysosomal dysfunction, along with DNA damage and epigenetic modifications. Genetic variants that influence neural longevity include FOXO3, IL-6, and TOMM40-APOE-APOC1 (Newman and Murabito, 2013; Shadyab and LaCroix, 2015; Zeng et al., 2016). However, it has been estimated that environmental contributions may account for as much as 70% of premature aging (Wyss-Coray, 2016). The aging neuron is vulnerable to reduced metabolic capacity, highlighted as a loss of nicotinamide adenine dinucleotide (NAD+) concentrations (Ivanisevic et al., 2016). This reduction in bioenergetics negatively impacts DNA repair, which appears central to a downward spiral of cellular functionality. NAD+ levels can be augmented with precursor supplementation (nicotinamide mononucleotide) that reduces DNA damage and extends healthspan in mouse models (Fang et al., 2016; Hou et al., 2018). Following WFPM exposure, however, we see significant reductions in NAD+ levels, along with NADH, succinate, and taurine. Although this trend does not indicate aging, per se, it at least suggests that WFPM exposure may add to the burden of aging-related metabolic impairments in the brain. More importantly, neurometabolite alterations are associated with oxidative damage and chronic neuroinflammation (Kane and Sinclair, 2018; Lautrup et al., 2019; McReynolds et al., 2020). NAD+ and taurine boost anti-inflammatory activity. Reduced levels of NAD+ and taurine are associated with chronic neuroinflammation and drives neuroinflammaging, a low-grade inflammation state that drives aging process (Bhat et al., 2020; Verdin, 2015). NAD+ is involved in stress resistance and synaptic plasticity and is downregulated in aged animals, whereas the conversion of NAD+ to NADH is important for ATP generation (Lautrup et al., 2019). Succinate is important for mitochondrial function and oxidative metabolism (Giorgi-Coll et al., 2017). Taurine has been shown to be neuroprotective during aging (El Idrissi et al., 2013).
In summary, WFPM exposure activated microglia, drove infiltration of inflammatory monocytes and MHC-II+ cells into the brain parenchyma, decreased neuroprotective metabolites, and increased Aβ-42 levels. Overall enhanced neuroinflammation from WFPM exposure potentially contributes to neurological aging and pathology, and the effects demonstrated at environmentally relevant concentrations warrant further exploration into long-term neurological sequelae and vulnerability for specific subpopulations, especially in the young and those of advanced age. Beyond simple metrics of plaque buildup, we identified key components of ADRD promotion from systemic inflammation to cerebrovascular endothelial activation and glial proinflammatory activation to downregulation of key energetic metabolites. Based on our novel findings neuroimmune activity and neurometabolism, these data point to fundamental effects of exposure to WFPM leading to wide ranging pathology that may contribute to aging process.
DATA AVAILABILITY
Data are available upon request to the corresponding authors.
SUPPLEMENTARY DATA
Supplementary data are available at Toxicological Sciences online.
ACKNOWLEDGMENTS
The authors are grateful to the support from the Paguate Village and Pueblo of Laguna communities, especially Greg Jojola, Pat Pruitt, Steven Etter, and Kyle Swimmer. The authors thank Dong Sun for access to APP/PS1 mouse brains. The antibody to ZO1 (R26.4C) was developed by D.A. Goodenough at Harvard Medical School, obtained from the Developmental Studies Hybridoma Bank, created by the Eunice Kennedy Shriver National Institute of Child Health and Human Development (NICHD) and maintained at the University of Iowa. Chris Shuey of the Southwest Research and Information Center was essential to engaging the community and siting the mobile laboratory.
AUTHOR CONTRIBUTIONS
Study was designed by M.J.C., J.H., J.W., M.M., D.S., S.N., A.B., A.K.O., and B.B. Exposures and particulate collection were implemented by M.J.C., S.L., J.G.B., M.B., D.S., B.B., and R.H. Particle measurement and characterization were implemented by M.M. and Z.K. Data compilation and analysis were conducted by M.J.C., D.S., J.G.B., and M.M. Lung histopathology was conducted by J.W. and J.H. Brain histopathology was conducted by A.K.O., E.M., and C.G.C. Metabolomics were conducted by H.G. Flow cytometry was conducted by D.S. and S.N. Cytokine and protein measurements were conducted by G.H., D.S., C.M., and A.B. Geospatial and meteorological analysis were conducted by J.R.G. and Y.L. Principal writing of the manuscript was conducted by D.S., M.J.C., and S.N., with major sections contributed by H.G., M.M., Z.K., J.G., A.K.O., and Y.L. All authors read and take responsibility for the entire manuscript.
FUNDING
The National Institute of Environmental Health Sciences (R01 ES026673, R01 ES014639, P42 ES025589).
DECLARATION OF CONFLICTING INTERESTS
The authors declared no potential conflicts of interest with respect to the research, authorship, and/or publication of this article.
REFERENCES
Agency USEP. (
- aging
- cytokine
- phenotype
- inflammation
- cell adhesion molecules
- endothelial cells
- lung
- inspiration
- flow cytometry
- amyloid beta-protein
- cd31 antigens
- intercellular adhesion molecule 1
- mice, inbred c57bl
- microglia
- new mexico
- smoke
- taurine
- vascular cell adhesion molecule-1
- arizona
- bone marrow
- brain
- mice
- particulate matter
- wildfires
Comments