-
PDF
- Split View
-
Views
-
Cite
Cite
Ida Holásková, Meenal Elliott, Kathleen Brundage, Ewa Lukomska, Rosana Schafer, John B Barnett, Long-term Immunotoxic Effects of Oral Prenatal and Neonatal Atrazine Exposure, Toxicological Sciences, Volume 168, Issue 2, April 2019, Pages 497–507, https://doi.org/10.1093/toxsci/kfz005
- Share Icon Share
Abstract
Atrazine and its metabolites are present at high concentrations in many water supplies in agro-intensive areas. Because residents in these areas drink water from sources fed from these contaminated supplies, we investigated the long-term immunotoxicity of combined prenatal and neonatal (perinatal) exposure to atrazine via drinking water, on the immune system in mice. At 6 months of age, upon immunization with heat-killed Streptococcus pneumoniae, the serum IgG antibody response against the T independent antigen phosphorylcholine was significantly higher in male, but not female, atrazine-exposed mice as compared with that in untreated controls. No alterations were present in all offspring in the serum antibody response against the T-dependent antigen pneumococcal surface protein A (PspA). ELISpot analysis showed only a small, insignificant reduction in PspA-specific IgG producing splenocytes in atrazine-treated male offspring. Interestingly, upon ex vivo stimulation with anti-CD3 and anti-CD28 antibodies, significant decreases in interleukin (IL)-2, tumor necrosis factor-α, interferon-γ, and IL-17A and a decreasing trend in IL-10 were observed in splenocytes from atrazine-exposed male, but not female mice. Analysis of thymic and splenic cell populations showed no effects of atrazine exposure in either sex. This is the first time that long-term changes in the immune response were observed after a perinatal exposure to atrazine and it demonstrates that these early life exposures can result in permanent changes to the immune system as well as a male bias in these effects.
Atrazine [2-chloro-4-ethylamino-6-isopropylamino-1,3,5-triazine, CAS no. 1912-24-9) is a triazine herbicide used extensively in the United States to control weeds in corn, sugar cane and golf courses grass. Contamination of pond and ditch water in areas treated with atrazine often exceeds 500 μg per liter (Frank et al., 1987; Kolpin et al., 2000). Triazine herbicides including atrazine and its metabolite, deethylatrazine (DEEA) are among the most frequently detected pesticides in streams and ground water regardless of whether the area is agricultural, urban, undeveloped, or a major aquifer (Gilliom et al., 2007) with seasonal increase during April–July (Larson et al., 1999; Winchester et al., 2009). Atrazine continues to be approved for agricultural use in many developed countries, for example, the United States (EPA, 2006), Canada (HCPMRA, 2007), and Australia (https://apvma.gov.au/node/12371; last accessed January 23, 2019). Atrazine is not used in the European Union because of concerns that ground water concentrations would exceed their general maximum established for pesticides (https://ec.europa.eu/food/plant/pesticides_en; last accessed January 23, 2019).
Most published studies on the in vivo immunotoxicity of atrazine have involved exposure of adult animals to the compound. Not surprising, the effects vary depending on species, sex and exposure paradigm, ie, dose, length of exposure, time from exposure to assay, and route of exposure. This is illustrated when comparing the results reported by Chen et al. (2013), Zhao et al. (2013), and Filipov et al. (2005). Chen et al. (2013) investigated the effects of atrazine at three dose levels with daily exposure by gavage for 28 days in male Balb/c mice. At their highest dose (175 mg/kg bw/day), there was a suppression of cell-mediated, humoral, and innate immune functions including ex vivo interferon-γ (IFN-γ) and IL-4 production in response to ConA stimulation (Chen et al., 2013). At an intermediate dose (87.5 mg/kg bw/day), the spleen cell proliferation index to ConA, and IFN-γ (but not IL-4) production was decreased, whereas at the lowest dose (43.75 mg/kg bw/day), only the proliferative response was decreased (Chen et al., 2013). The apoptotic index of thymocytes was increased at the high and intermediate dose (Chen et al., 2013). Zhao et al. (2013) used a similar dosing paradigm with female C57Bl/6 mice but with different dose levels. There was decreased lymphocyte transformation and NK cell activity at both the high (125 mg/kg bw/day) and intermediate (25 mg/kg bw/day) doses (Zhao et al., 2013). In addition, the production of IL-4 but not IL-2, IFN-γ or tumor necrosis factor-α (TNF-α) was reduced at both high and intermediate doses (Zhao et al., 2013). Splenocyte phenotyping demonstrated significant decrease in percentages of CD3+ and CD4+ cells in both the high and intermediate dose groups (Zhao et al., 2013). The only effect noted with low dose (5 mg/kg bw/day) group was a reduction in spleen weight (Zhao et al., 2013). Filipov et al. (2005) used 4 week old C57Bl/6 mice at 2 of the same doses as Zhao et al. (2013), 25 and 125 mg/kg, and saw similar effects after dosing for only 14 days. Given that dosing time was shorter, the dose required to evoke an effect was higher for some responses, eg, CD4+ levels in peripheral blood (Filipov et al., 2005).
Pregnant women exposed to atrazine-contaminated water expose themselves as well as their unborn children (Winchester et al., 2009). Rooney et al. (2003) observed a decrease in the magnitude of the delayed-type hypersensitivity response and the IgM antibody response to sheep red blood antigen in 2-week-old rats exposed prenatally and postnatally (via lactation) to atrazine. In our previous studies, mice were perinatally exposed via a subcutaneous time-release atrazine pellet from 10 days’ post coitus to 11 days of age, to approximate cutaneous exposure reliably, similar to exposure by bathing or swimming in contaminated water. In these studies, male offspring showed an alteration of cell-mediated immune responses at 3 months of age. There was a significant increase in the cytotoxic activity of T-cells of males, with a trend toward the opposite in females (Rowe et al., 2006). Male offspring also showed a significant increase in T independent antibody production to a bacterial antigen. Females had a trend toward a decrease in both functions (Rowe et al., 2006). Similar increases in cytotoxic T lymphocyte and mixed lymphocyte responses (MLR) were seen in male offspring at 3 months of age (Rowe et al., 2008). Limited analysis of 6-month-old female offspring showed significant reduction in the number of antibody secreting cells (ASC) and the MLR levels (Rowe et al., 2008). Collectively, the evidence indicates that pre- and neonatal exposure to atrazine results in lasting effects on the immune response of the offspring and that the noted changes may differ depending on the age of the offspring.
Municipal water treatment plants are not routinely equipped to remove contaminants, such as atrazine and metabolites, thus, humans in atrazine-affected areas drink water from these contaminated sources. Therefore, we investigated the long-term effects of prenatal and postnatal (perinatal) exposure to atrazine delivered via drinking water to the dam on the immune system of offspring at 6 months of age. The major findings of the current study were alterations in specific antibody production and cytokine production in male offspring.
MATERIALS AND METHODS
Animals and treatment groups
Balb/c mice (Harlan Laboratories, Indianapolis, Indiana) were bred using the breeding trio arrangement, placing two females with one proved breeder male for 3 days. The weights of the female mice were recorded at the start of the breeding and on day 10 after coitus (dpc). Females that gained at least 15% of their initial body weight by 10 dpc were considered pregnant (Seaborn et al., 2011) and were assigned at random to either atrazine in drinking water (1250 ppb in 0.01% ethanol) or control water (tap water containing 0.01% ethanol as vehicle), beginning on 10 dpc for a total of 21 days of prenatal/lactational exposure. After weaning, one-half of each offspring cohort were separated by cage and sex and aged to 6 months under standard conditions for the analyses reported herein. Fewer dams were exposed to the vehicle than to atrazine, therefore, strain, sex, and age-matched controls (Harlan) were purchased shortly after weaning and were housed in the same standard conditions. Statistical analysis of the data obtained from the offspring from WVU-raised (dam exposed to vehicle) versus strain, sex, and age-matched weanling controls from Harlan revealed that there was no statistical difference in 25 tested variables (spleen and thymus), justifying combining the data from these two cohorts for comparison with the atrazine-exposed offspring. Mice were immunized with heat-killed Streptococcus pneumoniae (HKSP) as described below.
Preparation of heat-killed S. pneumoniae (HKSP) and immunization
Functional analysis of the immune system was performed by immunizing the offspring with a heat-killed S. pneumoniae strain R36A (HKSP). S. pneumoniae strain R36A is an avirulent, nonencapsulated strain. The kinetics of the serum antibody response and the predominant types of antibody isotypes in the immune response against phosphorylcholine (PC) and pneumococcal surface protein A (PspA) have been well characterized (Wu et al., 1999, 2000). The vaccine was prepared as described previously (Hanson et al., 2012; Salazar et al., 2005). Briefly, S. pneumoniae strain R36A was grown to mid-log phase in Todd–Hewitt broth supplemented with 2% yeast extract (BD Biosciences) at 37°C. The culture was grown to OD600 of 0.4, and immediately heat killed at 60°C for 1 h, washed twice in 0.85% saline, and re-suspended in saline at 2 × 109 CFU/ml. Sterility and the number of CFU were confirmed by culture on blood agar plates prior to killing and the heat-killed stock was stored at −80°C. Mice were immunized by intraperitoneal route with 2 × 108 CFU HKSP in 100 μl saline. Blood, spleens, and thymi were collected 10 days following immunization.
Primary cell culture and CD3/CD28 stimulation
Spleens and thymi were dissected immediately after euthanasia and submerged into 5 ml of ice-cold cell-wash buffer, consisting of RPMI-1640 medium (Mediatech, Inc. Manassas, Virginia) supplemented with 2% heat inactivated fetal bovine serum (HyClone Laboratories, Logan, Utah), 1.4 mM l-glutamine (ThermoFisher, Pittsburgh, Pennsylvania), 5 mM HEPES (HyClone Laboratories, Logan, Utah) 100 units/ml penicillin and 100 μg/ml streptomycin (BioWhittaker, Walkersville, Maryland), and 1 mM 2-mercaptoethanol (ThermoFisher). Spleens and thymi were smashed between sterile-frosted sections of microscope slides and passed through a 23G needle 3–4 times to prepare single cell suspensions. Cells were pelleted and red blood cells were removed using RBC lysis buffer (Sigma Chemical Co., St. Louis, Missouri). Cells were then washed with cell-wash buffer and viable cells were enumerated using a hemocytometer using the trypan blue dye exclusion method. For growth and overnight stimulation, splenocytes from each mouse were seeded in triplicates at 1 × 105 cells per well in 96-well plates in complete medium (cell-wash buffer above with 10% FBS) at 37°C in 5% CO2 and stimulated with anti-mouse CD3 (10 μg/ml; BD Biosciences, San Jose, California) and anti-mouse CD28 (2 μg/ml; BD Biosciences) or without stimulation. After a 24-h incubation, plates were centrifuged and supernatants were collected and stored at −20C° until assay. Cell preparations from each mouse were processed individually.
Cell staining and flow cytometry
Single cell suspensions of splenocytes and thymocytes were prepared as described above. Splenocytes were stained to detect B-cells (CD45R+), αβ T-cells (TCR β chain+), CD4+ T cells, CD8+ T cells, macrophages (CD11b), granulocytes (Ly-6G+ and Ly6C+), NK cells (CD314+), regulatory T cells (Treg; CD4+CD25+Foxp3+), and tolerogenic T cells (CD8+CD28+CD223+). Thymocytes were stained to detect various subsets of developing T cells using appropriate markers. Briefly, single-positive (SP) and double-positive (DP) cell subpopulations were identified using anti-CD4 and anti-CD8 antibodies. To identify the different double-negative (DN) subpopulations, CD44 and CD25 expression was determined on the CD4-CD8- population. A detailed list of antibody conjugates, source, and name of the antibody clones is provided in Supplementary Table 1. Supplementary Table 2 provides a list of the phenotypic identity of each measured cell type.
Surface and intracellular staining was performed as described previously (Hanson et al., 2012; Holásková et al., 2012). Cells (1 × 106 per aliquot) were stained using the following procedure: cells were washed with PBSAz (phosphate-buffered saline containing 2% FBS and 0.1% sodium azide) and then incubated with whole rat and mouse IgG (Jackson ImmunoResearch, West Grove, Pennsylvania) for 30 min on ice to block Fc receptors, followed by a PBSAz wash. The cells were incubated for 30 min on ice with fluorochrome-labeled antibodies, washed three times with PBSAz, and fixed overnight at 4°C with 0.4% paraformaldehyde. The paraformaldehyde was removed by washing with PBSAz and cells resuspended in PBSAz. CD4+ regulatory T (Treg) cells expressing the transcription factor forkhead box P3 were detected by staining for surface CD4 and CD25 followed by fixation/permeabilization for 30 min, washing, and stained for 30 min for intracellular Foxp3. Cells were washed twice, resuspended in PBSAz, and subjected to flow cytometry. Data were acquired using a FACSAria employing Diva 6.1 software (BD Biosciences) and analyzed using FCS Express software version 4 (DeNovo Software). A total of 10 000 events were collected and analyzed for each sample.
Th1/Th2/Th17 cytokine measurements
Cytometric bead array (CBA, Th1/Th2/Th17, BD Biosciences) was used to assess IL-2, IL-4, IL-6, IFN-γ, TNFα, IL-17a, and IL-10 in cell culture supernatants from both anti-CD3/CD28-stimulated and nonstimulated splenocytes according to manufacturer’s protocol. Data were acquired using FACSCaliber flow cytometer (BD Biosciences). Limits of detection for the cytokines are in pg/ml (0.1 for IL-2, 0.003 for IL-4, 1.4 for IL-6, 0.5 for IFN-γ, 0.9 for TNFα, 0.8 for IL-17a, and 16.8 for IL-10). In addition to the CBA assay, transforming growth factor Beta1 (TGF-β1) was measured using the Flex set designed for this cytokine (BD Biosciences). Gating and analysis were performed employing software for CBA array.
PspA and PC ELISA
PspA for use in the ELISA was prepared as described in Hanson et al. (2012). Plasmid UAB055, which contains the truncated PspA gene attached to a 6-His tag (gift of Dr Susan Hollingshead, Department of Microbiology, UAB, Birmingham, Alabama), was transformed into BL21 (DE3) pLysS (EMD Biosciences, San Diego, California). A selected transformant colony was grown in Luria Bertani broth (Becton Dickinson Company) supplemented with ampicillin (100 μg/ml; Sigma-Aldrich) to an OD600 of 0.5, induced with 1 mM IPTG for 1 h and harvested. His-tagged PspA protein was purified from periplasmic extract using BugBuster HisBind purification kit (EMD Biosciences), dialyzed extensively against PBS, adjusted to 0.25 mg/ml and stored at 4°C.
Blood samples were collected from immunized mice and sera obtained by standardized methods and stored at −20°C until assayed as described in Hanson et al. (2012). For ELISA assays, Immulon 2 plates (ThermoFisher) were coated overnight at 4°C with 2 μg/ml PC-BSA or 5 μg/ml PspA. Plates were washed (PBS + 0.05% Tween-20) and blocked with 1% BSA in PBS at 37°C for 1 h. Plates were washed and 100 μl/well serum diluted in PBS was added starting at 1/400 for PC and 1/50 for PspA with two-fold dilutions thereafter, and allowed to bind overnight at 4°C. Plates were then washed and incubated with alkaline phosphatase (AP)-conjugated antibodies (Southern Biotechnology Associates, Birmingham, Alabama) for 3 h at 37°C. After washing, 100 μl/well phosphatase substrate (Sigma-Aldrich) dissolved in diethanolamine buffer, pH 9.8 was added. Absorbance values were read at 405 nm (A405) at timed intervals using a μQuant spectrophotometer (Bio-Tek Instruments, Winooski, Vermont) using KCJunior software (Bio-Tek Instruments). To determine the titer, a standard of pooled sera were diluted and titrated on each ELISA plate. The titer for each sample was determined by comparison to this standard when the A405 for the standard was 0.4 at a 1:3200 dilution to measure all anti-PC antibody isotypes and 0.2 for a dilution of 400 for anti-PspA IgM and IgG isotypes. These dilutions were chosen because they were in the linear part of the curve for the respective isotypes.
PspA and PC ELISpot
The number of splenic ASC producing anti-PspA and anti-PC antibodies were enumerated by ELISPOT as described previously (Holásková et al., 2012). Briefly, Acrowell 96-well filter plates (Pall Life Sciences, Ann Arbor, Michigan) were coated with 50 μl of PspA or PC-BSA (10 μg/ml) overnight at 4°C. Subsequent wash steps used PBS + 0.01% Tween-20. Plates were blocked for 2 h at 37°C by adding 200 μl/well RPMI medium containing 25% FBS. Plates were washed and splenocytes (100 μl/well) were plated in triplicates at 5 × 106 cells/ml. Plates were incubated for 4–6 h at 37°C in a 5% CO2 incubator. After washing, goat anti-mouse AP-conjugated IgM or IgG antibodies (Southern Biotechnology Associates), diluted 1:2000 in PBS + 1% BSA + 0.05% Tween-20, were added to the appropriate wells (100 μl/well). Plates were incubated overnight at 4°C and washed. SIGMAFAST 5-bromo-4-chloro-3-indolyl phosphate/nitro blue tetrazolium tablets (Sigma-Aldrich) were dissolved in distilled water and 100 μl added to each well. Color development was stopped by washing with distilled water. The number of spots/well was counted using a dissection microscope (Olympus Optical Co., Melville, New York). The number of specific ASC was calculated by using the mean number of spots from triplicate wells. The number of specific ASC was normalized to 1 × 106 splenic B cells, which were determined as the number of CD45R+ cells for each mouse employing flow cytometric analysis. Comparable fold increases were noted when normalized to whole spleen (Salazar et al., 2005).
Design of experiment and statistics
Exposure to atrazine (two groups: control and atrazine) was assigned to pregnant dams in a completely randomized design. For logistical reasons, collections of blood and tissues from aged mice representing both treatments and sex of offspring were done on two separate days, designated as a random replicate in the statistical analyses. Data from male and female offspring were separated for statistical analyses. Multiple blocking randomizations took place due to sex, age, and collection replications, thus the litter was not accounted for in the final statistical analyses as it would be preferred and used as the litter identifier in the model for statistical analyses. However, it was ensured each group was composed of multiple litters to prevent any confounding. Numbers of offspring utilized for each statistical test, with respective number of litter dams are listed in Supplementary Tables 3–7.
Goodness of fit for normal distribution on all continuous data (antibody titers, numbers of ASC, concentrations of cytokines, percentage and numbers of specific splenocytes and thymocytes, and ASC counts) were examined using Shapiro-Wilk W test and over-dispersion test, as recommended by Dittrich and Lehmann (2012), in addition to the Levene test of homogeneity of variance. Based on these tests, the effect of treatment on anti-PC and anti-PspA antibodies (ELISA), and the number of IgG and IgM ASC (ELISpot) from 6-month-old mice were analyzed by ANOVA with fixed effect of treatment and random effect of replicate, for males and females separately using Log10(data + 1)-transformed data. T-test or Welch test (where appropriate) were used for statistical analyses of cell populations and cytokine levels using natural Log-transformed data. All data were analyzed using JMP and SAS software (JMP, Version Pro 12.2, SAS Institute Inc., Cary, North Carolina; SAS, Version 9.4, SAS Institute Inc., Cary, North Carolina). Significance criterion alpha for all tests was 0.05.
RESULTS
Antibody Responses to PC and PspA Are Altered in Perinatally Atrazine-Exposed Male Offspring
A critical measurement of the function of the immune system is antibody production. The PC component of the HKSP vaccine is a type-2 T independent antigen that induces IgG2b and IgG3 in addition to IgM antibodies (Wu et al., 1999). Male offspring that were perinatally exposed to atrazine produced significantly more total IgG, specifically, the IgG2b and IgG3 subtypes (Figure 1A;Supplementary Table 3). Anti-PC IgM levels were not different between the atrazine-exposed and control male offspring (Figure 1A;Supplementary Table 3). In contrast, female offspring showed no difference in anti-PC IgM or IgG (total and subtypes) (Figure 1B;Supplementary Table 3). There were no significant differences in the PC-specific ASC in male or female offspring (Figs. 2A and 2B, Supplementary Table 4).
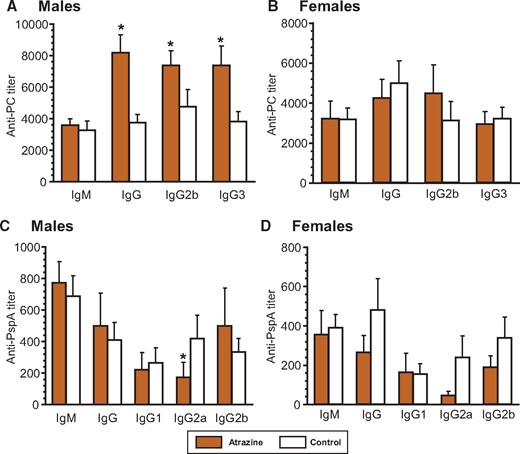
Serum anti-Streptococcus pneumoniae antibody levels 10 days after immunization with heat-killed S. pneumoniae (HKSP) vaccine. Offspring were immunized with HKSP as described and subtype-specific anti-HKSP antibody assays were performed using serum harvested 10 days after immunization. Anti-PC (A and B) and anti-PspA (C and D) antibody was quantified using an ELISA technique (see Materials and Methods), which measured HKSP-specific subtype antibodies. The averages (bars) and SEM for each antibody subtype are shown and the numbers of mice (and the number of litters they were derived from) appear in columns in Supplementary Table 3. Values significantly different between the control animals and the atrazine-exposed animals at p ≤ .05 are designated by an asterisk.
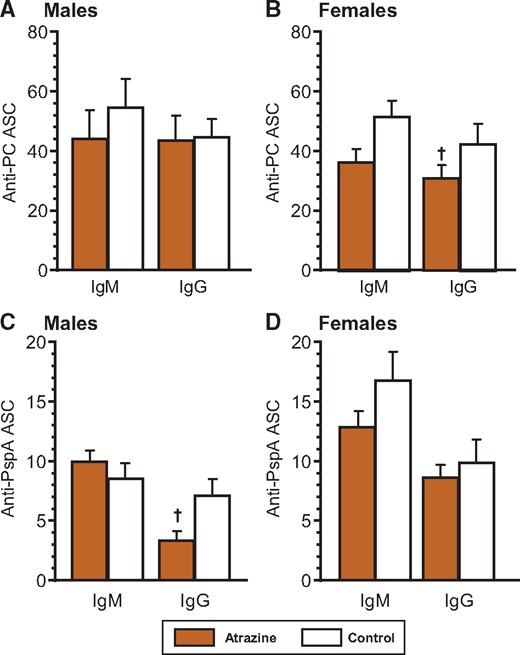
Splenic anti-Streptococcus pneumoniae ELIspot levels in 6-month-old animals after immunization with heat-killed S. pneumoniae (HKSP) vaccine. Offspring were immunized with HKSP as described and ELIspot assays were performed using spleen cells harvested 10 days after immunization. Anti-PC (A and B) and anti-PspA (C and D) were quantified using an ELIspot technique (see Materials and Methods), which measured number of individual spleen cells producing HKSP-specific IgM or IgG antibody. The averages (bars) and SEM are shown and the numbers of mice (and the number of litters they were derived from) appear in columns in Supplementary Table 4. Values significantly different between the control animals and the atrazine-exposed animals at p ≤ .09 are designated by a dagger.
The dominant T-dependent antigen contained in the HKSP vaccine is PspA. Aside from decreased IgG2a in male offspring, neither male nor female mice treated with atrazine had any significant difference in serum anti-PspA IgM and total IgG, or individual IgG1, and IgG2b subtypes from control mice (Figs. 1C and 1D;Supplementary Table 3). No significant differences were noted in the number of PspA-specific IgM or IgG ASC in the male or the female atrazine-treated mice from those in control mice (Figs. 2B and 2C;Supplementary Table 4).
Production of Several Cytokines Is Altered in Perinatally Atrazine-Exposed Male Offspring
Cytokine production was determined in splenocytes stimulated ex vivo with anti-CD3 and anti-CD28 antibodies. Production of IL-2, IFN-γ, TNF-α, and IL17a in splenocytes from male atrazine-exposed offspring was significantly lower than control offspring (Figure 3; Supplementary Table 5). There was a trend for a decrease in IL-10 production in male atrazine offspring (Figure 3; Supplementary Table 5). The production of IL-6 and IL4 was similar in the atrazine-exposed and control male offspring (Figure 3; Supplementary Table 5). Atrazine-exposed female offspring showed no significant difference in cytokine levels from those in control female mice (Figure 3; Supplementary Table 5).
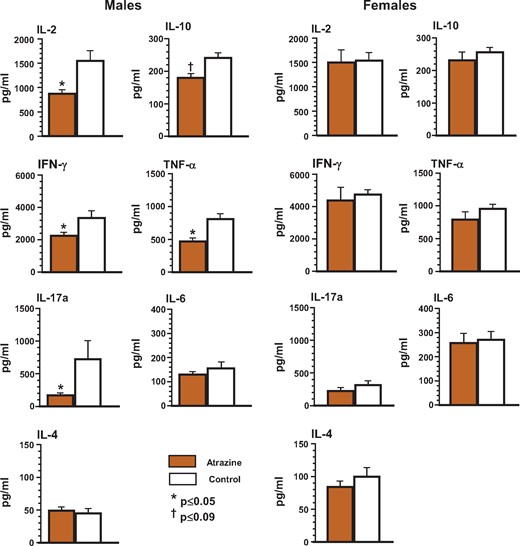
Ex vivo cytokine production by splenocytes isolated from the offspring after pre/postnatal atrazine exposure at 6 months of age. Single cell suspensions of splenocytes were prepared using standard techniques as described. The cells were stimulated in vitro with anti-CD3/anti-CD28 antibody to induce cytokine production. Culture supernatants were collected at the appropriate time to measure peak cytokine production using a cytometric bead array as described in the Materials and Methods. The averages (bars) and SEM are shown and the numbers of mice (and the number of litters they were derived from) appear in columns in Supplementary Table 5. Values significantly different between the control animals and the atrazine-exposed animals at p ≤ .05 are designated by an asterisk and at p ≤ .09 are designated by a dagger.
Thymus and Spleen Cell Populations Are Not Affected by Perinatal Exposure to Atrazine
We next sought to determine whether there were any differences in the frequencies of the phenotypes of various immune cells within the thymus and the spleen of atrazine-treated mice that would explain the differences in the immune response we had observed. The markers used to distinguish each of the phenotypes are listed in Supplementary Table 2. No significant differences were observed between atrazine-exposed and control offspring of either sex in the subsets of developing T cells in the thymus (Figure 4, Supplementary Table 6) or Treg populations in the thymus and spleen (Figure 5, Supplementary Table 6). Similarly, there were no significant differences in the splenic phenotypes (Figure 6, Supplementary Table 7). Separate analysis of the splenic B cell subpopulations (B1 [CD45R+CD11b+] versus B2 [CD45R+CD11b-]) was also performed (data not shown). B1 cells represent a small percentage (∼1%) of the total B cells in the spleen (Dexter and Corley, 1987), and there were no significant differences in the number of B1 cells between experimental groups of either sex.
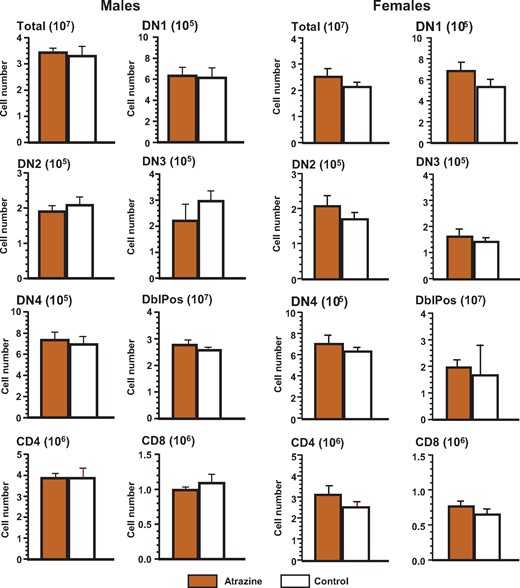
Phenotype of the thymocytes of the offspring after pre/postnatal atrazine exposure at 6 months of age. Single cell suspensions of thymocytes were made using standard techniques as described and stained with the appropriate fluorochrome-conjugated antibodies to quantify the cell type indicated. The fluorochrome-antibody combinations are listed in Supplementary Table 1. The averages (bars) and SEM are shown and the numbers of mice (and the number of litters they were derived from) appear in columns in Supplementary Table 6. The figure ordinate provides the number of cells of the listed phenotype and the scale multiplier is provided in parenthesis with the figure title.
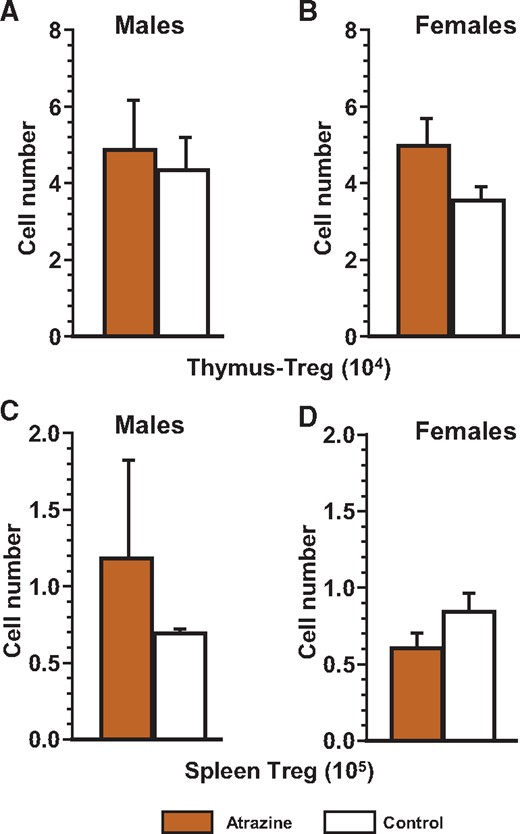
Number of regulatory T thymocytes (A and B) and regulatory T splenocytes (C and D) of the offspring after pre/postnatal atrazine exposure at 6 months of age. Single cell suspensions of thymocytes and splenocytes were made using standard techniques as described. These cells were stained with the appropriate fluorochrome-conjugated antibody (Supplementary Table 1) to quantify the cell type indicated as listed in Supplementary Table 2. The averages (bars) and SEM are shown and the numbers of mice (and the number of litters they were derived from) appear in columns in Supplementary Table 7. The figure ordinate provides the number of cells of the listed phenotype and the scale multiplier is provided in parenthesis with the figure title.
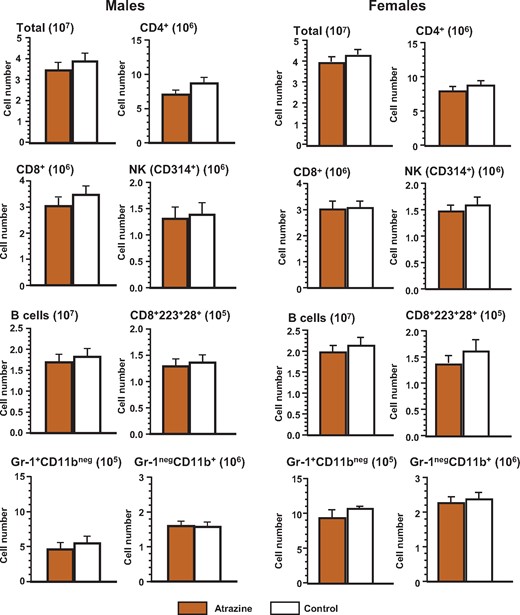
Phenotype of the splenocytes of the offspring after pre/postnatal atrazine exposure at 6 months of age. Single cell suspensions of splenocytes were made using standard techniques as described. These cells were stained with the appropriate fluorochrome-conjugated antibody (Supplementary Table 1) to quantify the cell type indicated (Supplementary Table 2). The averages (bars) and SEM are shown and the numbers of mice (and the number of litters they were derived from) appear in columns in Supplementary Table 7. The figure ordinate provides the number of cells of the listed phenotype and the scale multiplier is provided in parentheses.
DISCUSSION
The herbicide atrazine is an endocrine-disrupting chemical (EDC) used extensively in corn and sugarcane cultivation in the United States (EPA, 2006). A number of studies exploring potential adverse effects of this chemical as an occupational hazard (Curwin et al., 2005a,b; Lozier, 2010) for farmers, and for the general public that may have consumed potentially tainted waters in the area have been described. Although there is significant interest in the effects of atrazine as an endocrine disruptor via pre- and/or postnatal route (Cooper et al., 2000; Rayner et al., 2007; Winchester et al., 2009), very few studies address potential immunotoxic effects of this compound in mammals (Chen et al., 2013; Karrow et al., 2005; Rooney et al., 2003; Rowe et al., 2006, 2008). Another EDC bisphenol A (BPA), a compound known to leach from products used for infant care, such as feeding bottles, has also garnered extensive scrutiny as a possible health hazard (reviewed by Acconcia et al., 2015). Given the similar, estrogenic, mode of action between BPA (binding to nuclear and extra-nuclear estrogen receptors [Hong et al., 2006; Thomas and Dong, 2006]) and atrazine (inducing aromatase, thus aiding conversion of androgens to estrogens via second messenger system [Kucka et al., 2012; Roberge et al., 2004]), we will discuss our findings in light of what is already known about BPA.
We previously reported the effects of atrazine exposure in offspring of females implanted with subcutaneous pellets containing this pesticide from 10 days post coitus to 11 days after birth of pups (Rowe et al., 2006). In this study, exposure to atrazine occurred in utero as well as through lactation (Rowe et al., 2006). Exposure was delayed until after implantation (day 10 post coitus) because atrazine has been reported to interfere with implantation in some strains of rats (Cummings et al., 2000; Narotsky et al., 2001). When the offspring were assayed at 3 months of age, the most consistent atrazine-induced effects were seen in the male offspring. There was a significant increase in the cytotoxic T-cell function in atrazine-exposed males, although a trend toward decreased cytotoxic T-cell activity was observed in females (Rowe et al., 2006). Male offspring also had a significant increase in T independent antibody production against PC following immunization with HKSP although females only showed a trend toward a decreased antibody response (Rowe et al., 2006). A similar increase in the MLR was found in male offspring at 3 months of age (Rowe et al., 2008). In identically exposed 6-month-old female offspring, a significant reduction in the number of ASC and MLR response was noted, however, the serum antibody levels were unchanged (Rowe et al., 2008). No males were assayed at this time point (Rowe et al., 2008).
The current study was designed to investigate long-term alterations of immune system function following exposure to atrazine via drinking water to mimic exposure in humans via one common route. It is well-established that the developing immune system in the neonate is quite plastic and disturbances at this stage leave permanent changes and/or deficits in the humoral arm of the immune system (Vakil et al., 1986, 1991). We, therefore, chose to study whether prenatal and neonatal exposure to atrazine induced potentially harmful and long-term effects on the immune response of the offspring following immunization with HKSP. Atrazine was administered to pregnant mice at 10 days post coitus and continued up to 10 days after birth of the offspring. The immune response was determined in the offspring at 6 moa. The atrazine dose was similar to that previously tested by subcutaneous implantation in the form of a pellet (Rowe et al., 2006) and provided a comparison to the effects observed in that study. In some instances, the data from the studies reported herein, differ from those previously reported by our group on animals of the same age (Rowe et al., 2008). In those previous subcutaneous dosing studies (Rowe et al., 2008), the number of ASC were significantly decreased in the female atrazine-exposed offspring whereas no changes were noted in the female or male offspring exposed to atrazine via the drinking water reported herein. We speculate that these differences are due to the different routes of exposure, however, it should also be noted that humans could conceivably be exposed by drinking as well as bathing in contaminated water. Thus, the lack of a change in ASC in offspring exposed solely via their mother’s drinking water should not be taken as a “lack of risk” to humans living in areas with a contaminated water supply.
We focused our attention on the antibody response to a common human pathogen, Streptococcus pneumoniae, the causative agent of pneumonia in infants and the elderly. Immunization with a heat-killed preparation of the organism Streptococcus pneumoniae (HKSP) induces a protective antibody response in most inbred strains of mice (Briles et al., 1982; Yother et al., 1982). Significant changes were noted in male offspring perinatally exposed to atrazine and immunized with HKSP at 6 moa. The serum antibody response to the T independent antigen PC showed a consistent increase in the IgG subtypes but not IgM in males (Figure 1A;Supplementary Table 3). Because PC is a type-2 T independent antigen, IgG2b and IgG3 antibody subtypes are induced by this antigen in addition to IgM (Wu et al., 1999). No significant changes were noted in female siblings of these mice. This suggests that there are potential sex-based determinants that differentially influence the humoral immune response in male mice. These data corroborate our findings with the subcutaneous dosing method, where male offspring showed a significant increase in anti-PC antibody production at 3 months of age (Rowe et al., 2006). Rooney et al. (2003) also reported changes in the anti-sheep erythrocyte IgM responses in male but not female rat offspring at 8 weeks of age in rats exposed perinatally to atrazine via the dam.
In the current study, no significant differences were observed in either sex in the anti-PC-specific ASC between atrazine-exposed and control mice. The reason for the lack of a difference between the ASC response and the serum titers to PC may be due to different reasons. It is possible that the serum antibody levels represent an accumulation of antibody over time, whereas, the ASC response represents a particular snapshot of time within the spleen. We have previously noted that the number of specific ASC in the bone marrow more closely correlates with serum levels than splenic ASC (Salazar et al., 2005). One speculates that the levels of IgG antibodies in the serum are regulated outside the spleen and may involve expansion of ASC within the bone marrow or another site and may be influenced by cytokines present within their milieu. No differences were noted in the T-dependent anti-PspA antibody levels of any subtype in either sex (Figs. 1C and 1D;Supplementary Table 3), with the notable exception of the small but consistent decrease in IgG producing PspA-specific ASC in atrazine-exposed male mice (Figure 2C). The significance of this one small change is unknown.
To further investigate the mechanism behind the changes in antibody response against HKSP in male atrazine-exposed mice, we measured the capacity of spleen cells for cytokine production ex vivo following nonspecific stimulation with anti-CD3 and anti-CD28 antibodies. Several cytokines were decreased in the male atrazine-exposed offspring. Most notably, there was a reduction in IL-2, TNF-α, and IFN-γ, which are associated with TH1 cell function. TH1 cytokines function in the control of numerous types of infectious agents. IFN-γ is also a key cytokine in the function of T follicular helper (TFH) cells. Thus, the reduction of these cytokines suggests a compromise of the capacity for TH1 cytokine driven immune response and may be responsible for the observed decrease in PspA-specific IgG ASC in the spleen of atrazine-exposed males (Figure 2C). In addition, IL-10 production was less in atrazine-exposed offspring and this cytokine has a role in the differentiation and function of Treg cells. Although the numbers of Treg cells were not decreased, the decrease of two important cytokines that function with Treg cells, ie, IL-2 and IL-10, may speculatively provide an explanation for the increased antibody production to HKSP.
Of the two inflammatory cytokines, IL-17a was significantly reduced in splenocytes from atrazine-exposed mice although IL-6 was not. Both of these cytokines have multiple roles. IL-17a stimulates stromal cells to produce growth factors (G-CSF) as well as chemokines. IL-6 plays a role in antibody production. Therefore, the changes in the levels of IgG anti-PC antibodies between control and atrazine-exposed male mice appear to be independent of IL-6. We did not specifically measure any inflammatory responses that would directly assess changes resulting from alterations in the levels of IL-17. However, IL-17 is known to promote the production of IgG2a and IgG2b antibody subtypes in a T-dependent fashion and neither of these antibody subclasses were significantly changed for the T-dependent portion of the antibody response to HKSP. However, two caveats need mentioning. First, given the highly interactive relationship between cells and cytokines of the immune system, it is not surprising that our findings do not clearly correlate with functions heretofore ascribed to the various cells and cytokines of the immune system. Second, cytokine production was measured after in vitro stimulation with anti-CD3 and anti-CD28, which is a very strong nonantigen-specific stimulus, and thus, may not be representative of the result that may have been obtained with restimulation with HKSP or PspA.
The T independent antibody response has been shown to derive from the B1 subset of B cells that develop early during ontogeny (Masmoudi et al., 1990; Whitmore et al., 1996). Thus, it is likely that the mechanisms for homeostasis of this response are different from those that govern T-dependent and/or non-B1-cell-derived immune responses. The elevated levels of IgG antibodies against PC in atrazine-exposed male mice do not appear to result from a change in the capacity to respond to this antigen. Rather, it suggests a disruption of the process of homeostasis for this immune response, and may involve Treg and/or regulatory B cells. In this light, we note that we only measured the frequencies of Treg cells within the spleen and the thymus and although we failed to observe any numeric differences that atrazine treatment may have induced, it is possible that there were functional changes.
It is appropriate to compare our results with another EDC, BPA. In an experimental autoimmune encephalomyelitis model using the C57Bl/6 and SJL/J mouse strains, gestational exposure to BPA did not alter IL-17 production (Krementsov et al., 2013). The effects of prenatal exposure to BPA on cytokine production (Roy et al., 2012) showed some similarities with the results presented here. Although the experimental design was substantially different, Roy et al. (2012) measured cytokine gene expression in offspring prenatally exposed to BPA and then infected with influenza A. Per this study, early in the infection, at 3 days, no changes in the transcript levels of cytokines were observed for any of the measured cytokines. This may be because no differences exist during the initiation of infection or because they did not segregate their analysis by sex (Roy et al., 2012). Therefore, there is a possibility that sex-determined effect(s), particularly those that bring about delayed and long-term changes, might have been overlooked. This same study also reported significant long-term reductions in TNF-α and IFN-γ in the BPA-treated offspring (Roy et al., 2012). These latter findings are in agreement with our current observations. An extensive multisite study of BPA showed few immune system changes due to BPA exposure and interestingly, those changes reported were not dose dependent (Li et al., 2018a,b).
The reason for this increased sensitivity for immunomodulation in the male offspring is unknown and to our knowledge, this has not been tested or reported for humans. However, the extensive studies by Hayes et al. on the effects of atrazine on feminization of male frogs demonstrated that males are particularly sensitive to atrazine (Hayes et al., 2010).
We conclude that perinatal atrazine exposure alters the T independent, but not the T-dependent, antibody response to a bacterial antigen in male offspring. Although we were unable to attribute our findings to specific cell types or cytokines, the current study demonstrated that atrazine-induced alterations were predominantly associated with TH1 and/or Treg function in a sex-dependent manner. These findings further indicate that the mechanisms behind such alterations are complex. In considering the significance of our data on the possible risk of pre- and neonatal atrazine exposure to humans, a discussion on the relationship of the dose used herein versus the likely level of exposure of a human should be considered. The EPA has published the “margins of exposure” (MOE) (EPA, 2006) for many groups, which includes children, however, there was no MOE listed for immunotoxicity endpoints of individuals exposed during gestation. Given this apparent omission due to lack of data or consideration of existing animal data, this makes the significance of the studies reported herein, highly significant. Future studies will be designed to further dissect the immune response and its regulation and include a thorough assessment of the various subsets of B cells (B1, B2, Breg) and regulatory T cells. Our studies contribute significantly to future guidelines for safe practices in agriculture in order to minimize exposure of children during gestation and the neonatal period of development.
DECLARATION OF CONFLICTING INTERESTS
The author(s) declared no potential conflicts of interest with respect to the research, authorship, and/or publication of this article.
SUPPLEMENTARY DATA
Supplementary data are available at Toxicological Sciences online.
FUNDING
National Institutes of Health (ES014698 to J.B.B.).
REFERENCES
Author notes
These authors contributed equally to this study.
Comments