-
PDF
- Split View
-
Views
-
Cite
Cite
Margit Rosner, Katharina Schipany, Markus Hengstschläger, The Decision on the “Optimal” Human Pluripotent Stem Cell, Stem Cells Translational Medicine, Volume 3, Issue 5, May 2014, Pages 553–559, https://doi.org/10.5966/sctm.2013-0194
- Share Icon Share
Summary
Because of recent advances, the array of human pluripotent stem cells now contains embryonic stem cells, derived from “surplus” in vitro fertilization embryos or from cloned embryos; induced pluripotent stem cells; and amniotic fluid stem cells. Here, we compare these stem cell types regarding ethical and legal concerns, cultivation conditions, genomic stability, tumor developing potentials, and applicability for disease modeling and human therapy. This overview highlights that in the future appropriate methodological management must include a decision on the “optimal” stem cell to use before the specific application
Introduction
Human stem cells provide very important models for functional studies of genes involved in the molecular development of human genetic diseases. This holds true because, given fundamental biological differences, many human pathological phenotypes fail to be successfully replicated in mice [1]. In addition, human stem cells are considered to be a very powerful therapeutic tool. Bone marrow-derived stem cells have been used in regenerative therapies for more than 50 years. Multipotent mesenchymal stromal/stem cells from almost all adult tissues, hematopoietic stem cells, and fetal cord blood stem cells are currently investigated for their therapeutic applicability in numerous clinical and preclinical settings. The major drawbacks of these cells are often their limited differentiation potential as well as the enormous effort needed to keep them growing in culture [1–4]. Consequently, the group of pluripotent stem cells, including human embryonic stem cells (hESCs), human induced pluripotent stem cells (hiPSCs), and human amniotic fluid stem cells (hAFSCs), are of special interest in this context [1, 3, 4]. Very recently, methodological optimization has allowed the derivation of human embryonic stem cells from embryos obtained via somatic cell nuclear transfer (SCNT-hESCs) [5]. All of these pluripotent human stem cell types differ regarding their cellular origins, the protocols used for their isolation or derivation, the required cell-culturing techniques, or their genomic stability. Of special relevance for their putative clinical applications are their different tendencies to be tumorigenic in vivo [6, 7].
Loose and Stringent Pluripotency Criteria
In parallel with the latest progress in reprogramming somatic cells into induced pluripotent stem cells, a discussion was initiated with regard to defining standards for the use of the term “pluripotency” for stem cells. It was highlighted that in the loosest sense a pluripotent stem cell must be able to proliferate indefinitely while maintaining cellular identity (self-renewal), must express pluripotent stem cell markers, and must be able to differentiate into cells of all three embryonic germ layers. An international consortium of stem cell researchers came to the conclusion that besides hESCs and hiPSCs, hAFSCs also fulfill these loose pluripotency criteria [8]. In the strictest sense, pluripotency describes cells whose progeny can reconstitute an entire organism. In the case of murine pluripotent stem cells, testing their transmission through the germ line of chimeric animals to yield live pups is the routinely used standard. Because of practical and ethical limitations, functional pluripotency of human stem cells is often confirmed by their capacity to generate teratomas when injected into immunodeficient mice [9]. Still, assaying teratoma formation does not always allow a final conclusion. For example, hiPSCs derived from different human sources and established with different protocols have been found to harbor varying teratoma formation potencies [10]. In addition, detailed investigation of the literature revealed that the test for teratoma formation potency has been used for only half of the novel hESC and hiPSC lines reported in the scientific literature [11].
Human Embryonic Stem Cells
Due to the recent advances, hESCs can now be derived from the inner cell mass of the blastocyst of either “surplus” in vitro fertilization (IVF) embryos or of embryos upon somatic cell nuclear transfer (cloning). For any human cell type, ethical and legal concerns exist, for instance about ownership or about sequencing and publishing their entire genome. Both the naturally existing hESCs [12] and the artificially generated SCNT-hESCs [5] are associated with specific additional ethical and legal concerns that deal with the question of when human life begins and with the moral status of the embryo. SCNT-hESCs are obtained via somatic cell nuclear transfer and might therefore raise additional ethical and legal concerns associated with the issue of “human cloning.” Despite the need for sophisticated cell culture conditions, including the requirement of matrices-supported cell attachment, supplementation of specific growth factors, and particular cell passaging protocols, hESCs can be cultured efficiently and can fulfill the stringent pluripotency criteria [5, 8–10, 12] (Table 1; Fig. 1).
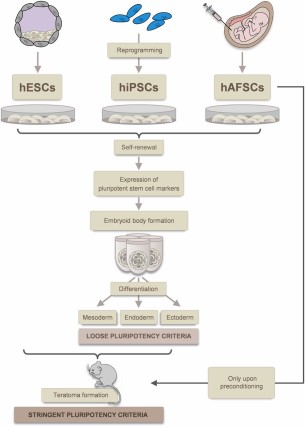
Tumorigenicity versus pluripotency: hESCs, hiPSCs, and hAFSCs harbor the potential for self-renewal, express pluripotent stem cell markers, form embryoid bodies, and can be differentiated into cells of all three germ layers. Whereas hESCs and hiPSCs form teratomas when injected into nude mice, hAFSCs are not tumorigenic per se and only form teratomas upon preconditioning with epigenetic regulators. Abbreviations: hAFSCs, human amniotic fluid stem cells; hESCs, human embryonic stem cells; hiPSCs, human induced pluripotent stem cells.
Comparison of human embryonic stem cells, induced pluripotent stem cells, and amniotic fluid stem cells
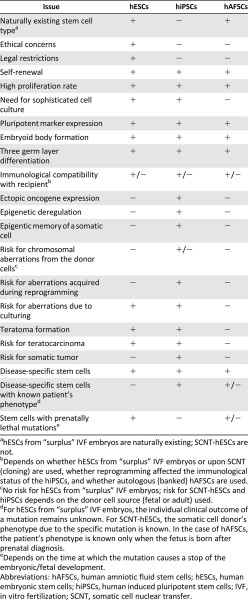
Comparison of human embryonic stem cells, induced pluripotent stem cells, and amniotic fluid stem cells
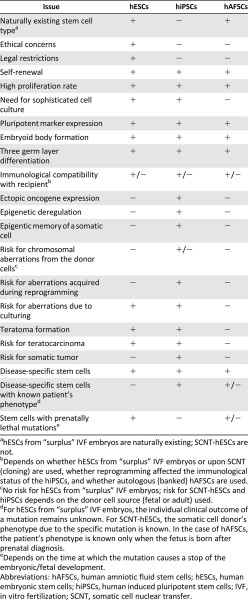
Human Induced Pluripotent Stem Cells
Human induced pluripotent stem cells originally were generated by the induction of a reprogramming process in an adult fibroblast via the ectopic expression of oncogenic transcription factors and stem cell marker genes [13, 14]. In between, various different methods for their derivation have been described [6, 8, 9]. These stem cells are not associated with the ethical and legal concerns of hESCs, can be grown efficiently under sophisticated culture conditions, and fulfill the stringent pluripotency criteria [8–10, 13, 14] (Table 1; Fig. 1).
Human Amniotic Fluid Stem Cells
First evidence for the existence of hAFSCs was provided in 2003, when a highly proliferative cell type expressing the pluripotent stem cell marker Oct4 was reported to exist in human amniotic fluid [15]. Human amniotic fluid stem cells represent a natural occurring stem cell entity that can be grown under standard cell culture conditions without the ethical concerns or legal restrictions of hESCs. These stem cells harbor the potential for self-renewal, express pluripotent stem cell markers, can form embryoid bodies, and can be differentiated into cells of all three germ layers [4, 15–18]. However, it was shown that injection of 3–8 × 106 cells of four independent human AFSC lines into rear leg muscles of 4-week-old male severe combined immunodeficient/Beige mice did not induce teratoma development. Still, because hAFSCs fulfill the loose pluripotency criteria, De Coppi et al. stated, “We conclude that AFS cells are pluripotent stem cells capable of giving rise to multiple lineages including representatives of all three embryonic germ layers” [16]. Another independent study confirmed that hAFSCs do not form teratomas. Moschidou et al. subcutaneously injected hAFSCs in the dorsal flank of 8- to 12-week-old common y chain−/− RAG2−/− C5−/− immunodeficient mice (n = 5, positive control hESCs) and did not detect teratoma development for up to 12 weeks after injection. Interestingly, preconditioning with the histone deacetylase inhibitor valproic acid, which is a U.S. Food and Drug Administration (FDA)-approved drug for the treatment of epilepsy, induced hAFSCs to form teratomas [19]. In summary, hAFSCs fulfill the loose pluripotency criteria and can be driven to also match the stringent criteria via preconditioning (Table 1; Fig. 1).
Future Perspectives on the “Optimal” Stem Cell for Disease Modeling
The tools of choice for disease modeling are human pluripotent stem cells harboring naturally occurring disease-causing mutations. Human embryonic stem cells with specific mutations can be generated from IVF embryos, which have been excluded from transfer into the uterus upon preimplantation genetic diagnosis. Over 2 decades, this genetic testing is offered to couples, whose potential offspring are at risk of single-gene disorders or structural and numerical chromosome aberrations. In countries where it is legal to destroy human embryos for research purposes, registries for hESC lines carrying specific mutations have been created [20]. The very recent methodological success in generating SCNT-hESCs might allow the derivation of SCNT-hESCs harboring specific mutations of the somatic cell donors in the future [5]. Many hiPSC lines from patients with single-gene disorders, chromosome syndromes, and complex diseases have already been generated [1, 21]. Since the 1970s, amniocentesis is a widespread standard procedure of prenatal care. Indications for amniocentesis are always situations with increased risk for fetal mutations. A variety of hAFSC banks have already been initiated to provide hAFSCs with specific naturally occurring genetic aberrations, which are of relevance for certain human pathologies [20] (Fig. 2).
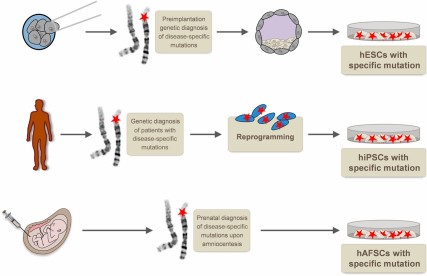
Pluripotent stem cells for disease modeling: hESCs with naturally occurring disease-causing mutations can be established from selected embryos after preimplantation genetic diagnosis. Reprogramming of somatic cells from patients with specific mutations allows disease-modeling attempts with hiPSCs. hAFSCs with specific mutations can be obtained upon prenatal diagnosis. Abbreviations: hAFSCs, human amniotic fluid stem cells; hESCs, human embryonic stem cells; hiPSCs, human induced pluripotent stem cells.
We argue here that the choice of a specific stem cell type depends on the question one wants to study with regard to disease modeling. First, it must be taken into account that, whereas hESCs and hAFSCs are naturally existing stem cell entities, hiPSCs (and also SCNT-hESCs) are artificially generated. The latter can harbor mutations accumulated during the life span of the somatic donor cell. This issue might probably be addressed by using fetal donor cells, which do not allow correlation of the in vitro stem cell features with the individual adult clinical phenotypes. That has not been studied in detail for SCNT-hESCs so far, but hiPSCs are known to accumulate chromosomal aberrations and gene mutations during the process of reprogramming. Furthermore, different hiPSCs exhibit varying differentiation propensities, what might at least partly result from the fact that during reprogramming hiPSCs do not perfectly erase the epigenetic pattern of the original differentiated cells from which they are derived. A third source of genetic aberrations is the adaption to cell culture. Mutated cells might have selective advantages upon extended culturing on matrices, under specific growth factor supplementation with the need for sophisticated passaging techniques [6, 9, 21]. Naturally existing hAFSCs are of fetal origin and can easily be cultured under nonsusceptible standard cell culture conditions without the need for surface coatings or specific growth factor supplementation. Importantly, hAFSCs can be routinely passaged via standard techniques involving single-cell dissociation. When compared with hESC and hiPSC culture, which is generally highly prone to cell death because of single-cell dissociation, passaging of hAFSCs is associated with good survival rates that strongly reduce the selective pressure during culture. Although the culture methods for hESCs and hiPSCs have been streamlined in recent years and different laboratories currently investigate whether hAFSCs could also be grown/differentiated if the culture conditions include particular factors/matrices, the fact that hAFSC culture is generally more straightforward is an obvious advantage of these cells (Table 1; Fig. 3). In contrast to hiPSCs (and likely also SCNT-hESCs), hESCs generally do not allow establishment of a disease-modeling tool for which the individual clinical outcome of a patient is known. In case of hAFSCs, the patient's clinical phenotype is only known when the fetus is born after prenatal diagnosis. Importantly, in contrast to hESCs and hAFSCs, hiPSCs normally allow disease-modeling approaches only for mutations that are not prenatally lethal (Table 1).
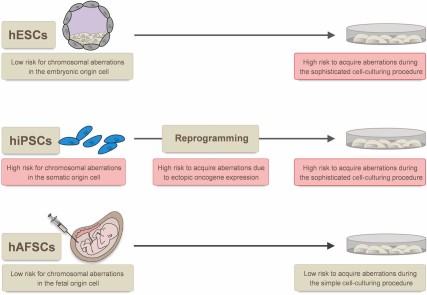
Sources of genetic aberrations in stem cells. The risk for chromosomal aberrations in the cell of origin is higher for hiPSCs (adult cells) than for hAFSCs (fetal cells) and for hESCs (embryonic cells). The stress induced during reprogramming (hiPSCs) and the need for more sophisticated cell culture conditions, including the requirement of matrices-supported cell attachment, supplementation of specific growth factors, and particular cell passaging techniques (hiPSCs and hESCs), are associated with a higher risk to induce genetic aberrations. Abbreviations: hAFSCs, human amniotic fluid stem cells; hESCs, human embryonic stem cells; hiPSCs, human induced pluripotent stem cells.
Future Perspectives on the “Optimal” Stem Cell for Therapy
With regard to the use of stem cells in human patients, a major hurdle is the immunological rejection after transplantation into the patient's body [3, 9]. Intensive future investigations will reveal whether the recent success in generating SCNT-hESCs can indeed provide a solution for this problem when it comes to the use of embryonic stem cells. Comparable to SCNT-hESCs, one can argue that using hiPSCs generated from cells of the patient who would receive the cell transplantation therapy could be a strategy to avoid the immune reactivity against the transplanted cells. However, currently, discussion concerns the extent to which the artificial reprogramming process might alter the immunological status of hiPSCs and what consequences for the immunorejection response of the recipient one must expect. These processes must be understood in detail to allow the use of hiPSCs in human therapies. In case of hAFSCs, the immunological aspect could be addressed by banking and autologous use of the patient's “own” hAFSCs in the future [3, 9, 20–23] (Table 1).
Whereas hAFSCs are not tumorigenic per se, hESCs and hiPSCs fulfill the stringent pluripotency criteria [6–8] (Fig. 1). Here, it is important to mention that no study exists that has investigated in detail whether any of the pluripotent stem cell types discussed here would induce tumor formation when injected into a human body. This tumorigenicity is considered the key obstacle to the safe use of hESCs (including SCNT-hESCs) and hiPSCs in regenerative medicine. It has been reported that some pluripotent hESCs even form teratocarcinomas, the malignant cousins of teratomas, when injected into immunodeficient mice. Thus, it is theoretically possible that under certain circumstances therapies using hESCs could result in teratocarcinoma formation in the patient. Regardless of the risk of malignant tumor development, the production of benign teratomas as a side effect in humans would be extremely alarming. In fact, it would be unacceptable given that benign teratomas could be numerous and highly destructive to surrounding normal or regenerating tissue in the patient. The formation of teratomas in sensitive body regions, such as the central nervous system or the myocardium, would trigger considerable safety concerns. In case of hiPSCs, it has been shown that, depending on the type of their chromosomal aberrations (from the donor cell, acquired upon reprogramming, or induced during cell culture), they can even trigger the development of somatic tumors. For their putative use in human therapies, it is important to realize that different hiPSCs vary with regard to their tumorigenicity [6, 21–23] (Table 1; Fig. 4).
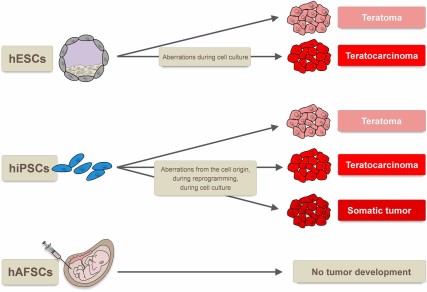
Tumor types formed by human pluripotent stem cells. hESCs form benign teratomas when injected into nude mice, and genetic aberrations during cell culture can induce the potential to form malignant teratocarcinomas. Whereas for hiPSCs the formation of teratomas, teratocarcinomas, and even somatic tumors has been reported, hAFSCs are not tumorigenic. Abbreviations: hAFSCs, human amniotic fluid stem cells; hESCs, human embryonic stem cells; hiPSCs, human induced pluripotent stem cells.
Currently, pluripotent stem cell-based clinical strategies are still in their infancy. For example, the biopharmaceutical company Geron was granted permission by the clinical advisory board of the FDA for a trial using hESC-derived oligodendrocytes for patients with spinal cord injury. This was not achieved until Geron was able to show that the cysts found in mice transplanted with these cells did not represent manifestations of teratomas [22]. Novocell is working on trials using hESC-derived pancreatic progenitor cells for the treatment of Type 1 diabetes. Advanced Cell Technology recently received approval by the FDA for a phase I/II clinical trial for the treatment of Stargardt disease and age-related macular degeneration with hESC-derived retinal pigment epithelial cells [21]. In general, detailed preclinical testing is required before stem cell derivatives can be used for cell transplantation in humans. Only comprehensive investigation and characterization of stem cell-based products before their clinical use allow achievement of safety assurance. The FDA argues for multiparametric testing, including morphological evaluation, detection of phenotype-specific cell surface antigens, assessment of unique biochemical markers, and genomic/proteomic analyses. Furthermore, it must be taken into account that a cell probe, which is considered to be safe, can spontaneously transform during extended ex vivo culture. It is therefore of highest relevance to establish routine test approaches to monitor, for example, cell growth kinetics or chromosome anomalies [23]. For therapeutic use, the aim is to use pluripotent stem cells to generate cells of the desired lineage and transplant only lineage-specific cells after sorting. However, it is well know that cell sorting is never perfect and a few hundred pluripotent stem cells are sufficient to generate teratomas. Different strategies have been considered to circumvent this problem. One could transfect the cells with suicide genes that are specifically expressed in the pluripotent status. It would also be possible to use pluripotent stem cell killing agents to treat the stem cell-derived cell mixture before transplantation. However, both strategies will mediate serious stress to the entire cell product with unknown consequences for the therapeutic potential. Another strategy to cope with the tumorigenicity risk of pluripotent stem cells is to transplant and afterward monitor the patient with the aim of early stage tumor detection and elimination on formation in the patient's body (Fig. 5). All of these strategies have obvious serious limitations and risks, which stress the importance of critically evaluating the specific stem cell sources for their susceptibility to these different approaches before the decision about which stem cell type to use is made [3, 6, 20–23].
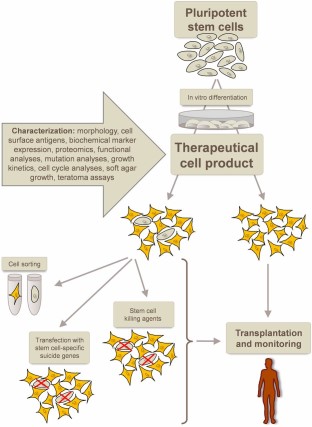
Development of safe cell therapeutics from pluripotent stem cells. After in vitro differentiation into the cell type of interest, the resulting preclinical cell product must thoroughly be characterized. Remaining undifferentiated, tumorigenic stem cells are suggested to be eliminated via cell sorting, transfection with stem cell-specific suicide genes, or via stem cell killing agents. Upon transplantation, monitoring of the patients is required to allow early detection and elimination of tumors.
Conclusion
The major goals of current human stem cell research are genetic disease modeling and stem cell-based human therapies. Because different types of pluripotent human stem cells are available, investigators must accurately define the “optimal” stem cell for the investigation of the specific scientific question or for the most promising treatment (with the lowest risk of side effects) of the specific disease of interest. For disease modeling, the choice of the stem cell with a specific mutation depends on the phenotypical questions that one wants to investigate. For stem cell-based therapies, circumstances under which an optimized strategy would involve those pluripotent stem cells must be assessed precisely to determine which can be differentiated into curative cell types in vitro but are expected not to trigger tumor development in vivo, such as hAFSCs.
Author Contributions
M.R. and K.S.: conception and design, final approval of manuscript; M.H.: conception and design, preparation of artwork, manuscript writing, final approval of manuscript.
Disclosure of Potential Conflicts of Interest
The authors indicate no potential conflicts of interest.