Visible light exposure induces a broad spectrum of physiologic responses, ranging from changes in gene expression to overt behavior [1–4], which can impact health and well-being. While light has been used therapeutically since antiquity, the advent of modern solid-state lighting technology presents new possibilities to optimize lighting conditions in the built environment for better health.
The light–dark cycle is the primary synchronizer of the mammalian central circadian pacemaker [5–8]. Aberrant light exposure can misalign circadian rhythms, which are ~24-hour endogenously generated physiologic oscillations, relative to each other and relative to external social and work schedules. Circadian misalignment is pervasive in clinical (e.g. circadian rhythm sleep–wake disorders) and general populations (e.g. shiftworkers, jet lag). A majority of individuals working nonstandard hours, including shiftworkers, have aberrant light exposure and associated circadian rhythm misalignment and sleep disruption. Up to 30% of shiftworkers meet the diagnostic criteria for a shiftwork sleep disorder, marked by significant complaints of insomnia, excessive sleepiness, and functional impairments [9]. Chronic circadian misalignment and sleep disruption, such as in shiftwork are implicated in major health conditions, from depression to diabetes and an increased risk of errors, accidents, and injuries [10].
Optimized lighting can be an effective countermeasure for shiftwork-related adverse effects on health and safety. Controlling the key characteristics of light exposure, including the timing [11], intensity [12], duration [13] and spectrum [11] facilitates synchronization of the endogenous circadian pacemaker (Figure 1) to shifted sleep–wake and work schedules through robust phase resetting of the circadian pacemaker [14–16]. Additionally, visible light exposure has acute alerting effects, reduces sleepiness, improves mood, and neurobehavioral performance [1, 15, 17–19]. These improvements translate to improved safety and productivity [20–24].
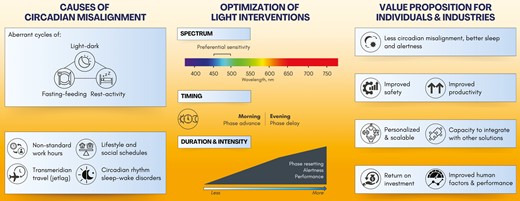
Figure 1.
Nonstandard work hours, such as in-shift work is one of the most common causes of circadian misalignment. Sleep- and circadian-informed lighting solutions are effective but underutilized in shiftwork management. When optimized for circadian adaptation, short-wavelength enriched light can suppress melatonin, induce alertness, and induce robust phase resetting. Although there is a monotonic relationship between duration and intensity with and non-visual responses (e.g. melatonin suppression, phase resetting, and alertness), the relationship is typically nonlinear such that even short duration and moderate intensity exposures can induce larger than expected responses compared to longer duration high-intensity exposures. Guyett, Scott, and colleagues tested the use of moderate-intensity, short-wavelength enriched, longer-duration light exposure to improve circadian adaptation, sleep, and neurobehavioral performance in the context of submariner work. Optimization of lighting depends on individual differences, organizational requirements, and work environments. To enable widespread adoption and implementation, we require larger studies testing the effectiveness and documenting return on investment. Further work must consider tailoring light interventions to address individual variability and ensure scalability through integration with other health and wellness initiatives.
Guyett and colleagues [25] evaluated the impact of ambient lighting optimized for shiftwork based on sleep and circadian principles on circadian phase resetting in a controlled laboratory setting. In an 8-day protocol, healthy, shift-work naïve individuals followed a simulated “submariner schedule” that included daytime sleep between 10:00 and 19:00 hours and overnight simulated work between midnight and 8:00 hours. In a 2-visit randomized crossover design, participants were exposed to either standard or optimized lighting. The timing, intensity, and duration of light exposure were optimized for circadian phase delays using the model of arousal dynamics [26, 27]. The sleep- and circadian-informed lighting paradigm consisted of a gradual transition to moderately bright, short-wavelength (blue) enriched light (wall-mounted LED lights, melanopic-EDI 238 lux [430 lux]), then to dim, short-wavelength depleted lighting (melanopic-EDI 3 lux [7 lux]) at group-average time of the core temperature minimum. Light timings were adjusted daily based on predicted phase delays. The optimized lighting schedule resulted in a ~1.5 hour per day delay shift of the central pacemaker, as measured using the gold-standard phase marker dim light melatonin onset (~6.5-hour total shift, ~50% of the target 12-hour shift in the work schedule). Scott and colleagues [28] extended these findings, documenting a 52-minute increase in the total sleep time, a 9% improvement in sleep efficiency, and marginal improvements in psychomotor performance.
These phase resetting results are consistent with previous studies in other simulated work schedules and conditions, such as space missions [29–31]. The neurobehavioral results align with other studies documenting that short-wavelength enriched light exposure during overnight shifts enhances alertness and operational performance in various environments [20, 23, 32–35], underscoring the positive impact of visible light in overall adaptation to shift work.
Despite accumulating evidence, the adoption of circadian-informed lighting in shift-work industries remains limited. This is likely precipitated by the paucity of value proposition evaluations [36, 37], partly due to limited sample sizes in research studies that often preclude generalizability. While targeted studies provide the essential proof-of-concept across diverse operational contexts, large-scale studies enabling generalization and assessing effectiveness are warranted. Evaluation of returns on investment across different operational settings is also limited [37–39], impeding institutional adoption. Research outcomes, such as phase shifts or improved cognitive performance are promising, but their translation to industry-specific key performance indicators is not readily clear, which minimizes enthusiasm and organizational buy-in for such technologies. Example performance indicators from an industry perspective may include operational readiness in defense; sick leave, inpatient stay duration in health care; reduced errors, and accidents in mining etc.
Figure 1 While emerging evidence strongly supports the implementation of sleep- and circadian-informed lighting (“SCHIL”) interventions, it is important to recognize that optimization is highly context-specific and not a “one-size fits all” approach [40]. Complete adaptation may be feasible and useful for submariners, where shifts follow a relatively stable pattern, but may be impractical for rapidly rotating shiftwork rosters, including healthcare, transportation, and service industries. Many of these workers may work multiple jobs, exchange shifts, or have changing work environments, which can lead to inconsistent light exposure schedules. In cases where continuous exposure may not be possible, workers may still benefit from bursts or brief pulses of light exposure to promote circadian adaptation [11, 31], or even partial adaptation (i.e. shifting melatonin peak into the first half of the daytime sleep episode) [41]. In other settings where circadian adaptation is not practical or possible, other lighting interventions aimed at acute alertness or fatigue mitigation may be the most feasible solution [23, 32, 42].
In addition to context-specific lighting interventions, personalized therapies are becoming increasingly vital, recognized as a strategic goal in sleep medicine [43]. There are extensive interindividual differences in sensitivity to light and non-visual responses to light exposure, including melatonin suppression, circadian resetting, and acute alerting effects [7, 44–49]. Personalized interventions that account for individual differences in circadian physiology, personal needs, operational circumstances, and schedules are more likely to succeed long-term [50–52] and reduce the need for extensive workplace lighting modifications [32].
Personalized lighting can integrate with other lifestyle, health, and wellness initiatives, making interventions more scalable and adaptable across industries. The model of arousal dynamics, which informed the circadian lighting solution in Guyett, Scott, and colleagues’ research [25, 28] has been used for delivering tailored sleep timing recommendations [53, 54], providing opportunities for much-needed multicomponent support for shift workers [55]. Personalized interventions can empower individuals to make informed choices, which may improve their engagement and overall implementation of such interventions.
Emerging evidence also indicates that while light exposure is the main time cue for setting the central pacemaker, non-photic stimuli such as meals may be the main time cue for resetting peripheral circadian rhythms [56–59]. Given that the goal is to reduce system-wide (i.e. whole animal) circadian disruption, future work needs to examine the interaction and optimization of both photic and non-photic cues to achieve comprehensive circadian adaptation and improvements in overall health.
Conclusion
Sleep- and circadian-informed lighting schedules are minimally intrusive, cost-effective, and scalable, especially in restrictive environments. The science of SCHIL interventions promoting circadian adaptation and improving neurocognitive performance is growing in robustness, with newer studies [25, 28] extending the wealth of evidence. Going forward, the value proposition and personalization of such interventions need to be carefully approached for their adoption and success in workplaces.
Disclosure Statement
Financial disclosures: PV and SAR have no financial disclosures related to the present editorial. SAR was supported in part by NIH-NHLBI R01HL159207, R01HL162102. Nonfinancial disclosures: PV holds patent for (1) Systems and methods for monitoring and control of sleep patterns (International Application No. PCT/AU2021/050126); through Monash University’s Entrepreneurship program, PV has explored the development of a digitalized sleep support for shift workers. SAR holds patents for (1) Method and device for preventing alterations in circadian rhythm (U.S. patent application Ser. No. 10/525,958), and (2) Methods and devices for improving sleep performance in patient exposed to light at night (U.S. Application No. 61/810,985); SAR owns equity in Melcort Inc.; has provided paid consulting services to Sultan & Knight Limited, Bambu Vault LLC, Lucidity Lighting Inc.; and has received honoraria as an invited speaker and travel funds from Starry Skies Lake Superior, University of Minnesota Medical School, PennWell Corp., and Seoul Semiconductor Co. Ltd., FALK FOUNDATION E.V.; SAR has received grant/research support from Seoul Semiconductor Co. Ltd., Biological Innovation and Optimization Systems, LLC, Merck & Co., Inc., Pfizer Inc., Vanda Pharmaceuticals Inc., Lighting Science Group, NIH, and NASA. These interests were reviewed and managed by Brigham and Women’s Hospital and Mass General Brigham in accordance with their conflicts-of-interest policies.
References
1.Cajochen
C
, Zeitzer
JM
, Czeisler
CA
, Dijk
DJ.
Dose-response relationship for light intensity and ocular and electroencephalographic correlates of human alertness
.
Behav Brain Res.
2000
;
115
(
1
):
75
–
83
. doi:
10.1016/s0166-4328(00)00236-9 2.James
FO
, Cermakian
N
, Boivin
DB.
Circadian rhythms of melatonin, cortisol, and clock gene expression during simulated night shift work
.
Sleep.
2007
;
30
(
11
):
1427
–
1436
. doi:
10.1093/sleep/30.11.1427 3.Kervezee
L
, Cuesta
M
, Cermakian
N
, Boivin
DB.
Simulated night shift work induces circadian misalignment of the human peripheral blood mononuclear cell transcriptome
.
Proc Natl Acad Sci U S A.
2018
;
115
(
21
):
5540
–
5545
. doi:
10.1073/pnas.1720719115 4.Burns
AC
, et al. .
Day and night light exposure are associated with psychiatric disorders: an objective light study in >85,000 people
.
Nat Ment Health
.
2023
;
1
:
853
–
862
. doi:
10.1038/s44220-023-00135-8 5.Duffy
JF
, Kronauer
RE
, Czeisler
CA.
Phase-shifting human circadian rhythms: influence of sleep timing, social contact and light exposure
.
J Physiol.
1996
;
495
(
Pt 1
):
289
–
297
. doi:
10.1113/jphysiol.1996.sp021593 6.Duffy
JF
, Wright
KP.
Entrainment of the human circadian system by light
.
J Biol Rhythms.
2005
;
20
(
4
):
326
–
338
. doi:
10.1177/0748730405277983 7.Phillips
AJK
, Vidafar
P
, Burns
AC
, et al. .
High sensitivity and interindividual variability in the response of the human circadian system to evening light
.
Proc Natl Acad Sci U S A.
2019
;
116
(
24
):
12019
–
12024
. doi:
10.1073/pnas.1901824116 8.Rimmer
DW
, Boivin
DB
, Shanahan
TL
, Kronauer
RE
, Duffy
JF
, Czeisler
CA.
Dynamic resetting of the human circadian pacemaker by intermittent bright light
.
Am J Physiol Regul Integr Comp Physiol.
2000
;
279
(
5
):
R1574
–
R1579
. doi:
10.1152/ajpregu.2000.279.5.R1574 9.Pallesen
S
, Bjorvatn
B
, Waage
S
, Harris
A
, Sagoe
D.
Prevalence of shift work disorder: a systematic review and meta-analysis
.
Front Psychol.
2021
;
12
:
638252
. doi:
10.3389/fpsyg.2021.638252 10.Sletten
TL
, Cappuccio
FP
, Davidson
AJ
, Van Cauter
E
, Rajaratnam
SM
, Scheer
FA.
Health consequences of circadian disruption
.
Sleep.
2020
;
43
(
1
). doi:
10.1093/sleep/zsz194 11.St Hilaire
MA
, Ámundadóttir
ML
, Rahman
SA
, et al. .
The spectral sensitivity of human circadian phase resetting and melatonin suppression to light changes dynamically with light duration
.
Proc Natl Acad Sci
.
2022
;
119
(
51
):
e2205301119
. doi:
10.1073/pnas.2205301119 12.Zeitzer
JM
, Dijk
DJ
, Kronauer
R
, Brown
E
, Czeisler
C.
Sensitivity of the human circadian pacemaker to nocturnal light: melatonin phase resetting and suppression
.
J Physiol.
2000
;
526
(
Pt 3
):
695
–
702
. doi:
10.1111/j.1469-7793.2000.00695.x 13.Rahman
SA
, St Hilaire
MA
, Chang
AM
, et al. .
Circadian phase resetting by a single short-duration light exposure
.
JCI Insight
.
2017
;
2
(
7
):
e89494
. doi:
10.1172/jci.insight.89494 14.Czeisler
CA
, Johnson
MP
, Duffy
JF
, Brown
EN
, Ronda
JM
, Kronauer
RE.
Exposure to bright light and darkness to treat physiologic maladaptation to night work
.
N Engl J Med.
1990
;
322
(
18
):
1253
–
1259
. doi:
10.1056/NEJM199005033221801 15.Czeisler
CA
, Dijk
DJ.
Use of bright light to treat maladaptation to night shift work and circadian rhythm sleep disorders
.
J Sleep Res.
1995
;
4
(
S2
):
70
–
73
. doi:
10.1111/j.1365-2869.1995.tb00231.x 16.Vetter
C
, Pattison
PM
, Houser
K
, et al. .
A Review of human physiological responses to light: implications for the development of integrative lighting solutions
.
LEUKOS
.
2022
;
18
(
3
):
387
–
414
. doi:
10.1080/15502724.2021.1872383 18.Bedrosian
TA
, Nelson
RJ.
Timing of light exposure affects mood and brain circuits
.
Transl Psychiatry.
2017
;
7
(
1
):
e1017
–
e1017
. doi:
10.1038/tp.2016.262 19.Meyer
N
, Harvey
AG
, Lockley
SW
, Dijk
DJ.
Circadian rhythms and disorders of the timing of sleep
.
Lancet (London, England)
.
2022
;
400
(
10357
):
1061
–
1078
. doi:
10.1016/S0140-6736(22)00877-7 20.Chen
Y
, Broman
AT
, Priest
G
, Landrigan
CP
, Rahman
SA
, Lockley
SW.
The effect of blue-enriched lighting on medical error rate in a University Hospital ICU
.
Jt Comm J Qual Patient Saf
.
2021
;
47
(
3
):
165
–
175
. doi:
10.1016/j.jcjq.2020.11.007 21.Juslén
H
, Wouters
M
, Tenner
A.
The influence of controllable task-lighting on productivity: a field study in a factory
.
Appl Ergon.
2007
;
38
(
1
):
39
–
44
. doi:
10.1016/j.apergo.2006.01.005 22.Juslén
HT
, Wouters
MCHM
, Tenner
AD.
Lighting level and productivity: a field study in the electronics industry
.
Ergonomics.
2007
;
50
(
4
):
615
–
624
. doi:
10.1080/00140130601155001 23.Sletten
TL
, Raman
B
, Magee
M
, et al. .
A blue-enriched, increased intensity light intervention to improve alertness and performance in rotating night shift workers in an operational setting
.
Nat Sci Sleep
.
2021
;
13
:
647
–
657
. doi:
10.2147/NSS.S287097 24.Wu
CJ
, Huang
TY
, Ou
SF
, Shiea
JT
, Lee
BO.
Effects of lighting interventions to improve sleepiness in night-shift workers: a systematic review and meta-analysis
.
Healthcare
.
2022
;
10
(
8
):
1390
. doi:
10.3390/healthcare10081390 25.Guyett
A
, Lovato
N
, Manners
J
, et al. .
A circadian-informed lighting intervention accelerates circadian adjustment to a night work schedule in a submarine lighting environment
.
Sleep.
2024
;
47
(
11
):
1
–
9
. doi:
10.1093/sleep/zsae146 26.Knock
SA
, Magee
M
, Stone
JE
, et al. .
Prediction of shiftworker alertness, sleep, and circadian phase using a model of arousal dynamics constrained by shift schedules and light exposure
.
Sleep.
2021
;
44
(
11
). doi:
10.1093/sleep/zsab146 27.Postnova
S
, Lockley
SW
, Robinson
PA.
Sleep propensity under forced desynchrony in a model of arousal state dynamics
.
J Biol Rhythms.
2016
;
31
(
5
):
498
–
508
. doi:
10.1177/0748730416658806 28.Scott
H
, Guyett
A
, Manners
J
, et al. .
Circadian-informed lighting improves vigilance, sleep, and subjective sleepiness during simulated night shift work
.
Sleep.
2024
;
47
(
11
):
1
–
13
. doi:
10.1093/sleep/zsae173 29.Grant
LK
, Kent
BA
, Rahman
SA
, et al. .
The effect of a dynamic lighting schedule on neurobehavioral performance during a 45-day simulated space mission
.
SLEEP Adv
.
2024
;
5
(
1
):
zpae032
. doi:
10.1093/sleepadvances/zpae032 30.Rahman
SA
, Kent
BA
, Grant
LK
, et al. .
Effects of dynamic lighting on circadian phase, self-reported sleep and performance during a 45-day space analog mission with chronic variable sleep deficiency
.
J Pineal Res.
2022
;
73
(
4
):
e12826
. doi:
10.1111/jpi.12826 31.Rahman
SA
, St., Hilaire
MA
, Grant
LK
, et al. .
Dynamic lighting schedules to facilitate circadian adaptation to shifted timing of sleep and wake
.
J Pineal Res.
2022
;
73
(
1
):
e12805
. doi:
10.1111/jpi.12805 32.Olson
JA
, Artenie
DZ
, Cyr
M
, Raz
A
, Lee
V.
Developing a light-based intervention to reduce fatigue and improve sleep in rapidly rotating shift workers
.
Chronobiol Int.
2020
;
37
(
4
):
573
–
591
. doi:
10.1080/07420528.2019.1698591 33.Querstret
D
, O’Brien
K
, Skene
DJ
, Maben
J.
Improving fatigue risk management in healthcare: a systematic scoping review of sleep-related/fatigue-management interventions for nurses and midwives
.
Int J Nurs Stud.
2020
;
106
:
103513
. doi:
10.1016/j.ijnurstu.2019.103513 34.Sletten
TL
, Ftouni
S
, Nicholas
CL
, et al. .
Randomised controlled trial of the efficacy of a blue-enriched light intervention to improve alertness and performance in night shift workers
.
Occup Environ Med.
2017
;
74
(
11
):
792
–
801
. doi:
10.1136/oemed-2016-103818 35.Van de Putte
E
, Kindt
S
, Bracke
P
, et al. .
The influence of integrative lighting on sleep and cognitive functioning of shift workers during the morning shift in an assembly plant
.
Appl Ergon.
2022
;
99
:
103618
. doi:
10.1016/j.apergo.2021.103618 36.Jones
BA.
Spillover health effects of energy efficiency investments: Quasi-experimental evidence from the Los Angeles LED streetlight program
.
J Environ Econ Manage.
2018
;
88
:
283
–
299
. doi:
10.1016/j.jeem.2018.01.002 37.Kim
AA
, Wang
S
, McCunn
LJ.
Building value proposition for interactive lighting systems in the workplace: combining energy and occupant perspectives
.
J Build Eng
.
2019
;
24
:
100752
. doi:
10.1016/j.jobe.2019.100752 38.Adhvaryu
A
, Kala
N
, Nyshadham
A.
The light and the heat: productivity co-benefits of energy-saving technology
.
Rev Econom Stat
.
2020
;
102
(
4
):
779
–
792
. doi:
10.1162/rest_a_00886 40.Houser
KW
, Esposito
T.
Human-centric lighting: foundational considerations and a five-step design process
.
Front Neurol.
2021
;
12
:
630553
. doi:
10.3389/fneur.2021.630553 41.Eastman
CI
, Martin
SK.
How to use light and dark to produce circadian adaptation to night shift work
.
Ann Med.
1999
;
31
(
2
):
87
–
98
. doi:
10.3109/07853899908998783 42.Shen
L
, Varma
P
, Murray
JM
, Sletten
TL
.
Sleep, Circadian Rhythms and Shift Work: adopting personalised approaches to managing shift work and circadian misalignment
. In:
Jackson
ML
, Drummond
SPA
, eds.
Advances in the Psychobiology of Sleep and Circadian Rhythms
.
Routledge
;
2023
.
43.Zee
PC
, Badr
MS
, Kushida
C
, et al. .
Strategic opportunities in sleep and circadian research: report of the Joint Task Force of the Sleep Research Society and American Academy of Sleep Medicine
.
Sleep.
2014
;
37
(
2
):
219
–
227
. doi:
10.5665/sleep.3384 44.Burns
AC
, Phillips
AJK
, Rutter
MK
, Saxena
R
, Cain
SW
, Lane
JM.
Genome-wide gene by environment study of time spent in daylight and chronotype identifies emerging genetic architecture underlying light sensitivity
.
Sleep.
2023
;
46
(
3
). doi:
10.1093/sleep/zsac287 45.Chellappa
SL.
Individual differences in light sensitivity affect sleep and circadian rhythms
.
Sleep.
2021
;
44
(
2
). doi:
10.1093/sleep/zsaa214 46.Dumont
M
, Benhaberou-Brun
D
, Paquet
J.
Profile of 24-h light exposure and circadian phase of melatonin secretion in night workers
.
J Biol Rhythms.
2001
;
16
(
5
):
502
–
511
. doi:
10.1177/074873001129002178 47.McGlashan
EM
, Poudel
GR
, Jamadar
SD
, Phillips
AJK
, Cain
SW.
Afraid of the dark: light acutely suppresses activity in the human amygdala
.
PLoS One.
2021
;
16
(
6
):
e0252350
. doi:
10.1371/journal.pone.0252350 48.Stone
J
, Mcglashan
E
, Quin
N
, et al. .
The role of light sensitivity and intrinsic circadian period in predicting individual circadian timing
.
J Biol Rhythms.
2020
;
35
:
628
–
640
. doi:
10.1177/0748730420962598 49.Stone
JE
, Sletten
TL
, Magee
M
, et al. .
Temporal dynamics of circadian phase shifting response to consecutive night shifts in healthcare workers: role of light–dark exposure
.
J Physiol.
2018
;
596
(
12
):
2381
–
2395
. doi:
10.1113/JP275589 50.Barger
LK
, Zhang
Y
, Lammers-van Der Holst
HM
, et al. .
Feasibility, effectiveness, and acceptability of an afternoon-evening sleep schedule in older nightshift workers
.
Sleep Adv
.
2024
;
5
(
1
):
zpae010
. doi:
10.1093/sleepadvances/zpae010 51.Stone
JE
, Postnova
S
, Sletten
TL
, Rajaratnam
SMW
, Phillips
AJK.
Computational approaches for individual circadian phase prediction in field settings
.
Curr Opin Syst Biol
.
2020
;
22
:
39
–
51
. doi:
10.1016/j.coisb.2020.07.011 52.Varma
P
, Postnova
S
, Phillips
AJK
, et al. .
Pilot feasibility testing of biomathematical model recommendations for personalising sleep timing in shift workers
.
J Sleep Res.
2023
;
n/a
(
n/a
):
e14026
. doi:
10.1111/jsr.14026 53.Song
YM
, Choi
SJ
, Park
SH
, Lee
SJ
, Joo
EY
, Kim
JK.
A real-time, personalized sleep intervention using mathematical modelling and wearable devices
.
Sleep.
2023
;
46
. doi:
10.1093/sleep/zsad179 54.Varma
P
, Postnova
S
, Knock
S
, et al. .
SleepSync: Early testing of a personalised sleep–wake management smartphone application for improving sleep and cognitive fitness in defence shift workers
.
Clocks Sleep
.
2024
;
6
(
2
):
267
–
280
. doi:
10.3390/clockssleep6020019 55.Tout
AF
, Tang
NKY
, Sletten
TL
, et al. .
Current sleep interventions for shift workers: a mini review to shape a new preventative, multicomponent sleep management programme
.
Front Sleep
.
2024
;
3
:
01
. doi:
10.3389/frsle.2024.1343393 56.Grant
LK
, St Hilaire
MA
, Brainard
GC
, Czeisler
CA
, Lockley
SW
, Rahman
SA.
Endogenous circadian regulation and phase resetting of clinical metabolic biomarkers
.
J Pineal Res.
2021
;
71
(
2
):
e12752
. doi:
10.1111/jpi.12752 57.Heyde
I
, Oster
H.
Differentiating external zeitgeber impact on peripheral circadian clock resetting
.
Sci Rep.
2019
;
9
(
1
):
20114
. doi:
10.1038/s41598-019-56323-z 58.Kent
BA
, Rahman
SA
, St Hilaire
MA
, et al. .
Circadian lipid and hepatic protein rhythms shift with a phase response curve different than melatonin
.
Nat Commun.
2022
;
13
(
1
):
681
. doi:
10.1038/s41467-022-28308-6 59.Wehrens
SMT
, Christou
S
, Isherwood
C
, et al. .
Meal timing regulates the human circadian system
.
Curr Biol.
2017
;
27
(
12
):
1768
–
1775.e3
. doi:
10.1016/j.cub.2017.04.059
© The Author(s) 2024. Published by Oxford University Press on behalf of Sleep Research Society.
This is an Open Access article distributed under the terms of the Creative Commons Attribution-NonCommercial License (
https://creativecommons.org/licenses/by-nc/4.0/), which permits non-commercial re-use, distribution, and reproduction in any medium, provided the original work is properly cited. For commercial re-use, please contact
[email protected] for reprints and translation rights for reprints. All other permissions can be obtained through our RightsLink service via the Permissions link on the article page on our site—for further information please contact
[email protected].
Comments