-
PDF
- Split View
-
Views
-
Cite
Cite
Alisha Guyett, Nicole Lovato, Jack Manners, Nicole Stuart, Barbara Toson, Bastien Lechat, Leon Lack, Gorica Micic, Siobhan Banks, Jillian Dorrian, Eva Kemps, Andrew Vakulin, Robert Adams, Danny J Eckert, Hannah Scott, Peter Catcheside, A circadian-informed lighting intervention accelerates circadian adjustment to a night work schedule in a submarine lighting environment, Sleep, Volume 47, Issue 11, November 2024, zsae146, https://doi.org/10.1093/sleep/zsae146
- Share Icon Share
Abstract
Night work has detrimental impacts on sleep and performance, primarily due to misalignment between sleep–wake schedules and underlying circadian rhythms. This study tested whether circadian-informed lighting accelerated circadian phase delay, and thus adjustment to night work, compared to blue-depleted standard lighting under simulated submariner work conditions.
Nineteen healthy sleepers (12 males; mean ± SD aged 29 ± 10 years) participated in two separate 8-day visits approximately 1 month apart to receive, in random order, circadian-informed lighting (blue-enriched and dim, blue-depleted lighting at specific times) and standard lighting (dim, blue-depleted lighting). After an adaptation night (day 1), salivary dim-light melatonin onset (DLMO) assessment was undertaken from 18:00 to 02:00 on days 2–3. During days 3–7, participants completed simulated night work from 00:00 to 08:00 and a sleep period from 10:00 to 19:00. Post-condition DLMO assessment occurred from 21:00 to 13:00 on days 7–8. Ingestible capsules continuously sampled temperature to estimate daily core body temperature minimum (Tmin) time. Tmin and DLMO circadian delays were compared between conditions using mixed effects models.
There were significant condition-by-day interactions in Tmin and DLMO delays (both p < .001). After four simulated night shifts, circadian-informed lighting produced a mean [95% CI] 5.6 [3.0 to 8.2] hours greater delay in Tmin timing and a 4.2 [3.0 to 5.5] hours greater delay in DLMO timing compared to standard lighting.
Circadian-informed lighting accelerates adjustment to shiftwork in a simulated submariner work environment. Circadian lighting interventions warrant consideration in any dimly lit and blue-depleted work environments where circadian adjustment is relevant to help enhance human performance, safety, and health.
Circadian rhythms reflect our body’s internal clocks that control the activity and timing of bodily functions, including our sleep–wake cycle. Shift-work leads to circadian misalignment, for which well-timed light exposure is one of the most potent methods to help retime the body-clock. This study found that blue-depleted lighting reduces body-clock adjustment to a 12-hour shift change, and that circadian-informed lighting produced around 3-fold faster adjustment to the new sleep–wake schedule. These findings are relevant to submariner and other shift-work contexts such as in healthcare, manufacturing, and mining industries where adaptation to a new shift schedule may be needed to enhance worker performance and safety, and mitigate health risks arising from maladjusted circadian timing.
Circadian rhythms are cyclical fluctuations in the activity of bodily processes, functions, and behaviors that occur across a ~24-hour cycle to support optimal wake function and sleep recovery [1]. Circadian misalignment arises when sleep–wake behaviors occur out-of-phase with the timing of the circadian system. A common example is when night workers perform duties when their circadian rhythms have primed their bodies for sleep [2]. During circadian misalignment, there is a greater likelihood of poor operational performance, unintended fall-asleep events with adverse safety consequences, and negative impacts on sleep and health [3]. Core body temperature and alertness are positively associated, and vigilance, problem-solving and decision-making are typically worst near the circadian “low” (core body temperature minimum, Tmin) when the body is primed for sleep. Accordingly, accidents are more common at this time [4]. Strategies to support circadian adjustment are therefore important to improve shift workers’ sleep, health, safety and productivity.
Circadian-based lighting interventions have potential as practical and effective countermeasures for circadian misalignment. The light–dark cycle is the strongest zeitgeber (time-giver) that helps to adjust circadian rhythms to a night work schedule. The intensity, spectral characteristics, and timing of the light exposure all contribute to the direction and magnitude of circadian rhythm retiming effects. Laboratory-based simulation studies have shown that medium intensity (∼1200 lux) or bright (≥5000 lux) light can help adjust circadian rhythms to a night work schedule [4, 5]. In particular, blue lighting (wavelength 450–530 nanometers, nm) has a profoundly stronger circadian effect than red lighting (620–750 nm) [6]. Lighting effects are also strongest when exposure occurs closer to Tmin, with phase delay effects occurring with exposure prior to Tmin and phase advance effects occurring with exposure following Tmin [4]. By considering and strategically manipulating the attributes of lighting, the circadian clock can be retimed to align with a different sleep–wake schedule. Many studies on lighting impacts in operational settings focus solely on specific time points relative to Tmin, neglecting the potential effects of lighting throughout the entire wake period, which could offer more practical insights. Given the major influence of light exposure on the circadian system, lighting interventions are likely to be amongst the most effective methods to accelerate circadian adjustments necessary for successful sleep–wake and performance adaptations to shift-work environments [7]. Lighting interventions could be particularly effective at addressing circadian misalignment issues in the dim, often red-shifted and thus blue-depleted lighting environment of submarines.
Submariners are a shift working population that have misaligned circadian rhythms and poor sleep [8–11]. Submarines have traditionally employed dim, blue-depleted lighting to preserve control room periscope operator night-vision, prevent light emissions and conserve power. This lighting environment may be particularly problematic for circadian system adjustment, particularly in crew members required to transition to an extended night shift-work schedule. Studies measuring oral temperature and melatonin secretion as markers of circadian timing and dim-light melatonin onset (DLMO) found that even after 42–67 days at sea, there was minimal evidence of circadian adjustment to night work schedules on-board a submarine [9, 10, 12]. In a recent 67-day field study in a small group of submariners (N = 9), DLMO timing remained unchanged at the end of the mission compared to pre-mission, after maintaining either diurnal sleep (N = 4) or nocturnal sleep (N = 5) “6hr on/6hr off” watch standing rotations [10]. Although it is difficult to generalize findings from such small observational studies, these data support that despite lengthy mission durations, submariners appear to continuously work in a state of chronic jetlag, potentially perpetuated by chronic dim lighting conditions and lack of normal zeitgebers. Chronic effects of circadian misalignment in submariners could help to explain a higher prevalence of multimorbidity (32.2%), defined as at least two chronic medical conditions, such as diabetes, chronic obstructive pulmonary disease, hypertension, and psychological disorders, compared to non-submariners (11.7%) [13]. Furthermore, the negative impacts of circadian misalignment on vigilance and work performance can be catastrophic in any shift-work context, including in military operations [14]. Multiple maritime accidents in the Defence sector have been at least partially attributed to disrupted sleep and circadian rhythms (e.g. John S McCain and Tanker Alnic MC, Singapore Strait [15]). Countermeasures are required to enhance circadian adjustment for shift workers, increasing the likelihood that submariners’ peak alertness coincides with operational demands and peak sleepiness aligns with off-shift sleep opportunities.
There is a profound lack of exposure to the natural light–dark solar cycle in submarines. Artificial lighting is typically dim (<10 lux) blue-depleted light (2400–3100 Kelvin [K]) [16], which is much dimmer than natural light and most workplace environments. Thus, the daily lighting cues that primarily drive daily circadian adjustment and sustained adjustment to the shift work-rest schedule changes are largely absent without lighting interventions. One study used two different fluorescent lamp types, one comprised of blue-enriched lighting (correlated color temperature [CCT] = 13 500 K high CCT, lux not reported) and the other blue-depleted lighting (CCT = 4100 K low CCT, lux not reported), applied under three watch schedules over two 11-day missions. In the high CCT condition, participant’s self-reported lower levels of sleepiness and showed greater activity device-estimated sleep efficiency, supporting that high CCT lighting promotes faster circadian adjustment [17]. A field study investigated the use of personalized blue–green light glasses as a countermeasure for circadian misalignment induced submariner fatigue [18]. Individuals randomized to the intervention were provided with personalized light transmission devices (PLTDs) with a peak wavelength of 470 nm that were worn for approximately 40 minutes upon waking (i.e. not based on circadian phase timing), combined with blue-blocking glasses to attenuate 400–510 nm light exposure from around 2 hours before going to sleep (lux not reported). Participants wearing PLTDs reported more sleep and demonstrated improved performance effectiveness, including reaction time and working memory improvements, compared to the control group [18]. Melatonin data were not collected in either study, so the magnitude of circadian phase shifts was not quantified.
Given promising but limited prior evidence needed to definitively establish if relatively simple lighting interventions can enhance circadian adjustment to night shift work, this study sought to test the effectiveness of a circadian-informed lighting intervention for accelerating circadian adjustment to simulated night work compared to standard submarine-like lighting in a controlled laboratory environment. To facilitate definitive proof-of-concept that a circadian-informed lighting intervention can accelerate circadian adjustment to night shift work in a submariner-like lighting environment, we selected healthy good sleepers, a within-participants randomized controlled cross-over study design, and daily 8 hour sleep opportunities more favorable for testing daily circadian adjustment effects than a more traditional submariner 6 hours on/6 hours off wake–sleep schedule. Based on predictions from a high-performance model of the circadian system [19], including the circadian systems phase response curve to light exposure, it was anticipated that relative to standard lighting, daily blue-enriched lighting exposure throughout specific periods of the work-shift would produce a more rapid phase delay to a night work schedule compared to conventional lighting similar to that on-board a submarine. Thus, the primary outcomes of this study were salivary DLMO and core body temperature minimum timing changes elicited by circadian-informed versus standard lighting. Secondary alertness and performance outcomes are reported elsewhere.
Materials and Methods
Study design
Further methodological details regarding this and a related study are reported in the Supplementary File. This was a randomized controlled, within-participants, counter-balanced cross-over study to examine the effect of a circadian-informed versus standard lighting on circadian phase delay following a 12-hour sleep–wake schedule change to four consecutive simulated night shifts. Participants experienced each lighting condition over 4 days within two separate 8-day laboratory stays, in random allocation order, with a 4-week washout period between visits (see Supplementary File for randomization). The primary study staff responsible for the lighting intervention were not blinded to the experimental condition. However, all other study staff, including those conducting analyses, and study participants, remained blinded to treatment allocation. The primary outcome was change in the timing of salivary DLMO, from pre- to post-lighting intervention. The daily change in the timing of Tmin, captured via ingested capsules, was a key secondary outcome. A target sample size of 24 participants was preplanned as six groups of four participants and was anticipated to be sufficient to detect modest differences in circadian timing shifts between conditions in a within-participants cross-over study design, with greater power than a between-participants design.
Research ethics approval
The study was approved by the Southern Adelaide Clinical Human Research Ethics Committee (protocol number: 86.20 Human Research Ethics Committee/20/SAC/91) and conducted at the Adelaide Institute for Sleep Health (Adelaide, South Australia).
Participants
Participants were recruited via posters on university noticeboards and online/social media platforms including Gumtree, Facebook, and Twitter. Prospective participants completed a self-reported screening questionnaire containing general health and lifestyle questions as well as the Insomnia Severity Index [20], Epworth Sleepiness Scale [21], and the morningness–eveningness questionnaire [22]. All participants were also screened for normal vision and ocular health.
The eligibility criteria were partly based on Defence Navy entry requirements, but with additional criteria designed to recruit healthy, good sleepers to specifically test the acute effectiveness of the lighting intervention with minimal risk of confounding from potential chronic shift-work effects. The specific criteria were: (1) aged ≥18 years, (2) normal health and fitness, (3) body mass index >18.5 and <32.9 kg/m², (4) no reported signs or symptoms consistent with a sleep disorder (insomnia, sleep apnea or any other clinical sleep disorder), (5) not a night worker, (6) nonsmoker and no excessive consumption of alcohol (<10 standard drinks/wk) or caffeine (<250 mg/day), (7) no transmeridian travel across multiple time zones within 2 months of participation, (8) intermediate chronotype (not <12 or >17 on the morningness–eveningness questionnaire), and (9) a reported habitual bedtime between 22:00 and 00:30, wake up time between 07:00 and 09:00, and typical total sleep time of 7–9 hours.
Lighting conditions
See Supplementary File for details regarding the description of the experimental setting, timeline of light exposure, lighting level characteristics and light measurement devices.
The standard lighting condition.
The standard lighting condition was designed to replicate lighting levels on-board submarines [16]. The lighting approximated a submarine control-room (dim-red light: ~7 lux, ~2400 K) during the simulated night work, off-shift recreational rooms (dim-white light: 15–20 lux, ~3100 K) during rest periods, and sleep environments (very dark conditions: <1 lux, <10 lux if light needed to be turned on during the sleep opportunity, e.g. during bathroom breaks) during the sleep opportunities.
Circadian-informed lighting condition.
The circadian-informed lighting condition was designed around a lighting exposure schedule predicted to achieve near maximal circadian delay adjustment to night work, based on a validated model of average group-level light exposure and circadian system effects on psychomotor vigilance task performance [23]. This condition consisted of a gradual transition to moderately bright, blue-enriched lighting (around 200 lux, similar to office lighting). Blue-enriched lighting then continued up until just prior to the model-predicted group-average daily time of the core temperature minimum, after which lighting gradually transitioned, to dim, blue-depleted lighting (<10 lux) to avoid circadian advancement effects. The same lighting schedule was simultaneously administered in all rooms to all participants, with the same daily prolongation in blue-enriched timing exposure to account for the anticipated group-average circadian delay in the core body temperature minimum time, which remained unknown until final analysis once all data collection was completed.
Study measures
Salivary melatonin collection.
Salivary DLMO is widely accepted as the gold-standard measure of circadian timing [24]. Standardized saliva sample collection procedures were followed to ensure that samples were collected under dim lighting conditions (<10 lux), participants remained sedentary and seated, and their pre-sample meals specifically excluded food items known to confound melatonin (e.g. bananas and nuts) [25]. See Supplementary File for radioimmunoassay procedures.
DLMO timing estimation.
DLMO was defined as the estimated time at which melatonin concentration first reached or exceeded 10 pM for at least 2 consecutive samples (or 1 hour) [24], using linear interpolation across the last sample below and the first of the two consecutive samples ≥10 pM. Shifts in the timing of the melatonin rhythm were assessed as the difference in DLMO time from pre- to post-intervention.
To assess the degree of melatonin suppression with the circadian compared to standard lighting condition on the first night work shift, two saliva samples were also collected after midnight (00:30 and 01:00 see Figure 1) [6]. This was designed to evaluate the anticipated melatonin suppression effect of blue-enriched compared to dim, blue-depleted lighting condition at a time when melatonin was expected to be rising on the first day after extended wake.
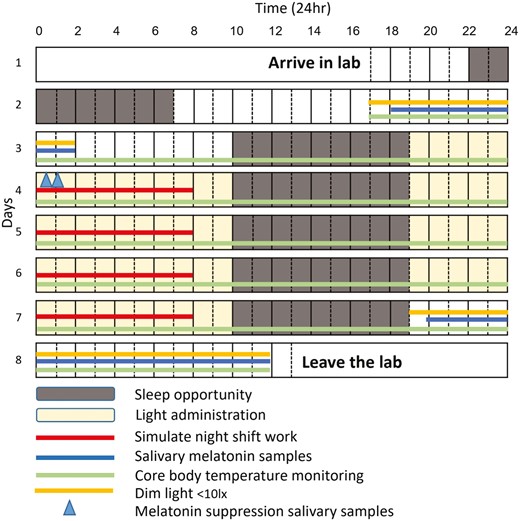
Core body temperature.
Core body temperature was continuously recorded via wireless ingestible capsules that transmitted data to an external recorder at 30 second intervals (e-Celsius Performance, BodyCap, Caen, France; mean ± SD accuracy ± 0.2 ± 0.1°C). The first capsule was ingested prior to initial DLMO data collection followed by further capsule ingestion as required after each capsule was passed (staff manually checked the external monitor every 2–3 hours during wake periods) to minimize data loss.
Temperature data were processed using Python (v3.7.6, SciPy v1.9.1) [26], which excluded non-physiological temperature extremes (<36 and >38°C) and rates of change (0.2°C/min), typically associated with settling time from ingestion, capsule expulsion, and meal or fluid ingestion effects. 48-hour windowed double cosine functions with 24-hour overlap were then fitted to all available data, from which daily Tmin and maximum were estimated [27]. The correlation coefficient between measured and cosine curve fit data ranged from r = .79 to .98 with a mean r = .90.
Study protocol
An overview of the protocol is outlined in Figure 1. Additional participant activity details are presented in Supplementary File.
Participants self-selected their own usual bedtime and maintained a regular daily sleep–wake schedule for the 2-week baseline, and 4-week washout period between the two lab visits. Regular timing was subsequently confirmed through sleep diaries and under-mattress sensor data [28]. Participants arrived at the sleep laboratory by 18:00 on day 1 for study setup after all returned a negative COVID-19 rapid antigen lateral flow test prior to entering the laboratory. Participants were instrumented for polysomnography and were carefully monitored throughout the study protocol by research staff and video feeds, except at shower times. To minimize potential confounding effects of exercise and meals on circadian outcomes, all activity was kept to a minimum, with only very low intensity exercise (walking, stretching) permitted from 20:30 to 21:30 each day, and all activities carefully regimented at pre-specified time-points.
Participants commenced their first sleep opportunity from 22:00 on day 1 and were then woken up at 07:00 on day 2, to complete an extended 27-hour wake period from 07:00 on day 2 to 10:00 on day 3, undertaking quiet, sedentary tasks. At 17:30, participants ingested an initial core body temperature capsule. Between 18:00 (day 2) and 02:00 (day 3), participants underwent the baseline salivary DLMO protocol, with half-hourly samples (n = 17 sample per participant) collected under dim lighting conditions (<10 lux). Each subsequent sleep opportunity occurred from 10:00 to 19:00 on day 3 to day 7. Meals were regulated throughout the participant’s stay (see Supplementary File for further details).
Following the sleep opportunity, participants were awakened at 19:00, and had free time in the laboratory. From 00:00 to 08:00, participants completed 8 hours of computer based cognitive performance testing under “simulated shift work” conditions (i.e. participants restricted to room, no use of phone, no recreational use of computer unless on break). The cognitive tasks undertaken during the “simulated shift work” tested the duties and responsibilities typical of submariners in the control room. These tasks encompassed sustained attention, reaction time, planning, and risk assessment, mirroring the cognitive functions required for their work. Participants then had free time until 10:00, when lights were turned off for a sleep opportunity from 10:00 to 19:00. This protocol was repeated across days 3–7.
After waking from the final sleep opportunity on day 7, participants undertook an extended 17-hour wake period from 19:00 on day 7 to 13:00 on day 8, for a repeat salivary DLMO protocol from 21:00 to 13:00 under dim lighting conditions (<10 lux; n = 33 samples per participant).
Statistical analyses
The effects of lighting condition (circadian-informed versus standard lighting) and day (days 2–8) on changes in DLMO timing (first sustained 10 pM crossing point) and Tmin timing were examined using mixed-effects model analysis. Mixed-model analysis was also conducted to examine the effect of lighting condition (circadian-informed vs. standard) on melatonin suppression during the initial night shift across two melatonin samples and melatonin concentration across repeated samples. The analysis employed a mixed-effects model that considered the potential for carry-over effects, visit (first vs. second laboratory stay) effects, sequence (lighting condition order) effects, and scaling. Where relevant, estimated marginal means were examined using Bonferroni-corrected pairwise contrasts for which only adjusted p-values are reported. Model assumptions were examined for homoscedasticity, outliers, and normality. Data are presented as means ± standard deviation (SD) or 95% confidence interval (CI). p-values < .05 were considered statistically significant. Statistical analyses for the main analysis were conducted in Stata (version 16) and IBM SPSS (version 27) was used for descriptive statistical analysis of participant demographic data.
Results
Participants
A total of 158 people replied to study advertisements. 64 met the study eligibility criteria and 28 consented to participate and were randomly assigned to allocation order in six separate groups. Four participants withdrew due to illness, three due to personal issues, one due to a scheduling issue that precluded participation and a further participant was unable to maintain a regular bedtime prior to the first in-laboratory visit and withdrew from the study. In total, 19 participants aged from 18 to 60 years completed the full study protocol, and their demographics and self-reported sleep characteristics at screening are presented in Table 1. One participant had no DLMO baseline in the circadian intervention as the samples did not reach the 10 pM threshold. In the standard condition, three participants were missing Tmin values on day 2, one participant on day 3 and one participant did not have any Tmin data. In the circadian-informed condition, one participant was missing Tmin from day 5.
Participant characteristic . | Result (n = 19) . |
---|---|
Age, y | 28.7 (10.4) |
Sex, N (%) female | 7 (37%) |
Body mass index, kg/m2 | 24.8 (3.9) |
Lifestyle characteristics | |
Number of standard alcoholic drinks per week | 0.3 (0.5) |
Number of caffeinated drinks per day | 0.9 (0.9) |
Sleep characteristics | |
Habitual bedtime, hh:mm | 22:51 (01:09) |
Habitual wake up time, hh:mm | 07:29 (00:56) |
Habitual total sleep time, h | 7.7 (0.9) |
Morningness–eveningness questionnaire score | 15.2 (2.4) |
Insomnia severity index | 4.0 (3.9) |
Epworth sleepiness scale | 3.8 (3.2) |
Participant characteristic . | Result (n = 19) . |
---|---|
Age, y | 28.7 (10.4) |
Sex, N (%) female | 7 (37%) |
Body mass index, kg/m2 | 24.8 (3.9) |
Lifestyle characteristics | |
Number of standard alcoholic drinks per week | 0.3 (0.5) |
Number of caffeinated drinks per day | 0.9 (0.9) |
Sleep characteristics | |
Habitual bedtime, hh:mm | 22:51 (01:09) |
Habitual wake up time, hh:mm | 07:29 (00:56) |
Habitual total sleep time, h | 7.7 (0.9) |
Morningness–eveningness questionnaire score | 15.2 (2.4) |
Insomnia severity index | 4.0 (3.9) |
Epworth sleepiness scale | 3.8 (3.2) |
Data are mean (standard deviation) unless otherwise indicated.
Participant characteristic . | Result (n = 19) . |
---|---|
Age, y | 28.7 (10.4) |
Sex, N (%) female | 7 (37%) |
Body mass index, kg/m2 | 24.8 (3.9) |
Lifestyle characteristics | |
Number of standard alcoholic drinks per week | 0.3 (0.5) |
Number of caffeinated drinks per day | 0.9 (0.9) |
Sleep characteristics | |
Habitual bedtime, hh:mm | 22:51 (01:09) |
Habitual wake up time, hh:mm | 07:29 (00:56) |
Habitual total sleep time, h | 7.7 (0.9) |
Morningness–eveningness questionnaire score | 15.2 (2.4) |
Insomnia severity index | 4.0 (3.9) |
Epworth sleepiness scale | 3.8 (3.2) |
Participant characteristic . | Result (n = 19) . |
---|---|
Age, y | 28.7 (10.4) |
Sex, N (%) female | 7 (37%) |
Body mass index, kg/m2 | 24.8 (3.9) |
Lifestyle characteristics | |
Number of standard alcoholic drinks per week | 0.3 (0.5) |
Number of caffeinated drinks per day | 0.9 (0.9) |
Sleep characteristics | |
Habitual bedtime, hh:mm | 22:51 (01:09) |
Habitual wake up time, hh:mm | 07:29 (00:56) |
Habitual total sleep time, h | 7.7 (0.9) |
Morningness–eveningness questionnaire score | 15.2 (2.4) |
Insomnia severity index | 4.0 (3.9) |
Epworth sleepiness scale | 3.8 (3.2) |
Data are mean (standard deviation) unless otherwise indicated.
Melatonin light suppression
During the first night-shift, there was a significant effect of condition F(1, 54.66) = 73.4, p = .001 indicating significantly greater melatonin suppression with circadian-informed (mean ± SD; 4.0 ± 8.0 pM) compared to standard lighting (36.6 ± 23.3 pM), but with no sample number or interaction effects (F(1, 48.92) = 2.53, p = .118 and F(1, 48.92) = 1.12, p = .295, respectively). See melatonin concentration at the ~48 hours protocol time in Figure 2.
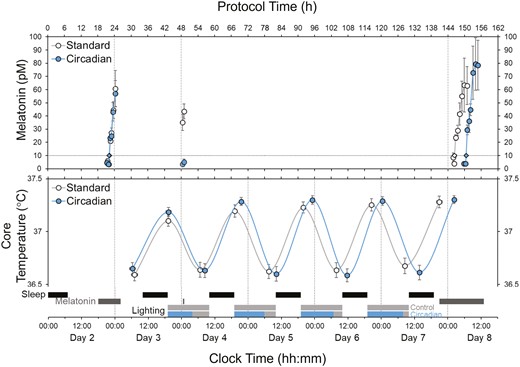
Dim-light melatonin onset concentrations (top panel), core body temperature (middle panel), relative to the study protocol timings (bottom panel) and hours into protocol (top × axis). Solid circles represent the circadian-informed condition, open circles represent the standard lighting condition. Values are mean ± SEM. The dotted line indicates the threshold 10 pM from which DLMO time was derived.
Dim-light melatonin onset
DLMO times are presented in the top panel of Table 2 and shown in Figure 2. There was a significant condition-by-time interaction on DLMO timing (F (1,51.72) = 29.83, p = .001), and significant effects of condition (F (1,51.67) = 30.82, p = .001) and time (F (1,51.72) = 110 228.56, p = .001), with a 4.2 ± 2.7 hour greater delay in DLMO timing from pre- to post-lighting intervention with the circadian-informed versus standard lighting (Table 2). Sequence and visit effects were not significant.
Dim-Light Melatonin Onset and Core Body Temperature Minimum Times (Clock Times for DLMO and Core Temperature Minimum Times and Hours for Their Corresponding Differences Δ)
. | Clock time (mean ± SD) . | Mean [95% CI] difference, h . | . | |
---|---|---|---|---|
Day . | Standard . | Circadian . | circadian-standard . | P . |
DLMO | ||||
2 | 22:14 ± 1:20 | 22:06 ± 1:15 | −0.1 [−0.7 to 0.5] | .919 |
8 | 02:24 ± 3:02 | 06:31 ± 1:45 | 4.1 [2.9 to 5.4] | <.001 |
Δh | 4.2 ± 3.0 | 8.2 ± 1.2 | 4.2 [3.0 to 5.5] | <.001 |
Δh/day | 0.7 ± 0.5 | 1.4 ± 0.2 | 0.7 [0.5 to 0.9] | <.001 |
Core temperature minimum | ||||
3 | 06:31 ± 3:28 | 06:22 ± 4:14 | −0.7 [−2.9 to 1.6] | .804 |
4 | 06:46 ± 2:30 | 08:26 ± 2:50 | 1.5 [0.4 to 2.6] | .036 |
5 | 07:30 ± 4:40 | 10:13 ± 1:54 | 2.7 [1.5 to 3.9] | <.001 |
6 | 07:52 ± 2:01 | 11:20 ± 2:39 | 3.3 [2.6 to 3.9] | <.001 |
7 | 08:23 ± 2:56 | 13:05 ± 1:44 | 4.5 [3.5 to 5.6] | <.001 |
Δh | 1.8 ± 3.3 | 6.7 ± 4.1 | 5.6 [3.0 to 8.2] | <.001 |
Δh/day | 0.4 ± 0.8 | 1.7 ± 1.0 | 1.4 [0.7 to 2.1] | <.001 |
. | Clock time (mean ± SD) . | Mean [95% CI] difference, h . | . | |
---|---|---|---|---|
Day . | Standard . | Circadian . | circadian-standard . | P . |
DLMO | ||||
2 | 22:14 ± 1:20 | 22:06 ± 1:15 | −0.1 [−0.7 to 0.5] | .919 |
8 | 02:24 ± 3:02 | 06:31 ± 1:45 | 4.1 [2.9 to 5.4] | <.001 |
Δh | 4.2 ± 3.0 | 8.2 ± 1.2 | 4.2 [3.0 to 5.5] | <.001 |
Δh/day | 0.7 ± 0.5 | 1.4 ± 0.2 | 0.7 [0.5 to 0.9] | <.001 |
Core temperature minimum | ||||
3 | 06:31 ± 3:28 | 06:22 ± 4:14 | −0.7 [−2.9 to 1.6] | .804 |
4 | 06:46 ± 2:30 | 08:26 ± 2:50 | 1.5 [0.4 to 2.6] | .036 |
5 | 07:30 ± 4:40 | 10:13 ± 1:54 | 2.7 [1.5 to 3.9] | <.001 |
6 | 07:52 ± 2:01 | 11:20 ± 2:39 | 3.3 [2.6 to 3.9] | <.001 |
7 | 08:23 ± 2:56 | 13:05 ± 1:44 | 4.5 [3.5 to 5.6] | <.001 |
Δh | 1.8 ± 3.3 | 6.7 ± 4.1 | 5.6 [3.0 to 8.2] | <.001 |
Δh/day | 0.4 ± 0.8 | 1.7 ± 1.0 | 1.4 [0.7 to 2.1] | <.001 |
Dim-Light Melatonin Onset and Core Body Temperature Minimum Times (Clock Times for DLMO and Core Temperature Minimum Times and Hours for Their Corresponding Differences Δ)
. | Clock time (mean ± SD) . | Mean [95% CI] difference, h . | . | |
---|---|---|---|---|
Day . | Standard . | Circadian . | circadian-standard . | P . |
DLMO | ||||
2 | 22:14 ± 1:20 | 22:06 ± 1:15 | −0.1 [−0.7 to 0.5] | .919 |
8 | 02:24 ± 3:02 | 06:31 ± 1:45 | 4.1 [2.9 to 5.4] | <.001 |
Δh | 4.2 ± 3.0 | 8.2 ± 1.2 | 4.2 [3.0 to 5.5] | <.001 |
Δh/day | 0.7 ± 0.5 | 1.4 ± 0.2 | 0.7 [0.5 to 0.9] | <.001 |
Core temperature minimum | ||||
3 | 06:31 ± 3:28 | 06:22 ± 4:14 | −0.7 [−2.9 to 1.6] | .804 |
4 | 06:46 ± 2:30 | 08:26 ± 2:50 | 1.5 [0.4 to 2.6] | .036 |
5 | 07:30 ± 4:40 | 10:13 ± 1:54 | 2.7 [1.5 to 3.9] | <.001 |
6 | 07:52 ± 2:01 | 11:20 ± 2:39 | 3.3 [2.6 to 3.9] | <.001 |
7 | 08:23 ± 2:56 | 13:05 ± 1:44 | 4.5 [3.5 to 5.6] | <.001 |
Δh | 1.8 ± 3.3 | 6.7 ± 4.1 | 5.6 [3.0 to 8.2] | <.001 |
Δh/day | 0.4 ± 0.8 | 1.7 ± 1.0 | 1.4 [0.7 to 2.1] | <.001 |
. | Clock time (mean ± SD) . | Mean [95% CI] difference, h . | . | |
---|---|---|---|---|
Day . | Standard . | Circadian . | circadian-standard . | P . |
DLMO | ||||
2 | 22:14 ± 1:20 | 22:06 ± 1:15 | −0.1 [−0.7 to 0.5] | .919 |
8 | 02:24 ± 3:02 | 06:31 ± 1:45 | 4.1 [2.9 to 5.4] | <.001 |
Δh | 4.2 ± 3.0 | 8.2 ± 1.2 | 4.2 [3.0 to 5.5] | <.001 |
Δh/day | 0.7 ± 0.5 | 1.4 ± 0.2 | 0.7 [0.5 to 0.9] | <.001 |
Core temperature minimum | ||||
3 | 06:31 ± 3:28 | 06:22 ± 4:14 | −0.7 [−2.9 to 1.6] | .804 |
4 | 06:46 ± 2:30 | 08:26 ± 2:50 | 1.5 [0.4 to 2.6] | .036 |
5 | 07:30 ± 4:40 | 10:13 ± 1:54 | 2.7 [1.5 to 3.9] | <.001 |
6 | 07:52 ± 2:01 | 11:20 ± 2:39 | 3.3 [2.6 to 3.9] | <.001 |
7 | 08:23 ± 2:56 | 13:05 ± 1:44 | 4.5 [3.5 to 5.6] | <.001 |
Δh | 1.8 ± 3.3 | 6.7 ± 4.1 | 5.6 [3.0 to 8.2] | <.001 |
Δh/day | 0.4 ± 0.8 | 1.7 ± 1.0 | 1.4 [0.7 to 2.1] | <.001 |
Core body temperature
Core body temperature minimum times are presented in the bottom panel of Table 2 and shown in Figure 2. There was a significant condition-by-day interaction on daily Tmin shift (F (4, 146.04) = 6.88, p = .001), and significant effects of condition (F (1,149.32) = 51.65, p = .001) and time (F (4,146.02) = 13 876.87, p = .001), with a 5.6 ± 5.0 hour greater delay at the final Tmin time-point with circadian-informed versus standard lighting after four simulated night shifts. A significant difference between lighting conditions was apparent from the second shift (day 4) onwards. Sequence and visit effects were not significant.
Discussion
This study found that a circadian-informed lighting strategy produced an approximately 2–3 fold faster circadian adjustment to night work compared to standard, dim, blue-depleted lighting. Under the standard lighting condition, both the timing of DLMO and Tmin shifted only by approximately 30 minutes per day across 4 days, compared to around 1.5 hours per day with the circadian-informed lighting. While longer-term extrapolation of these findings requires caution, if these daily shifts persisted, circadian-informed lighting would be expected to achieve full circadian adjustment to a 12-hour sleep–wake schedule shift within around 7 days compared to ~3 weeks with standard lighting if the average shift of ~30 minutes per day persisted. However, given evidence of no discernible circadian adjustment after around 60 days in a submarine [10], conventional submariner conditions may not be sufficient to achieve circadian adjustment to shift work at all. Thus, the findings from this study strongly support that circadian-informed lighting should be considered to counteract circadian misalignment in submariners and potentially in other shift-work occupations.
These findings build on a substantial body of existing literature indicating that bright blue-enriched light exposure has a profound influence on circadian biology [29, 30], including marked melatonin suppression during light exposure [29]. The finding of substantially accelerated circadian delay with blue-enriched lighting exposure during the work shift is consistent with a previous study of more intense lighting exposure (~3000 K, ~300 lux) during the work shift, which was found to improve alertness and performance in active-duty service members working night-shift schedules on a high-security watchfloor [31]. However, the present approach differs from previous studies that manipulated light only in the morning or evening [32]. Passively controlling overhead lighting is potentially also more practical and effective than briefer but more intense device-delivered lighting protocols [18], although interindividual differences in Tmin timing and light sensitivity appear likely to further influence the circadian adjustment efficacy of any lighting manipulation [33]. Thus, personalized adaptive lighting interventions more specifically designed around individual circadian and work-place factors may ultimately be more effective. Nonetheless, this group-level lighting intervention is clearly efficacious at phase delaying individuals in a comparatively short timeframe.
The current study supports the potential benefits of circadian-informed lighting interventions in shift-work settings and demonstrates that lighting should be considered as part of a shift-work management strategy to assist circadian adjustment. From an organizational perspective, this minimally intrusive and automated lighting strategy is likely to be feasible to support improved work performance and safety. Faster circadian adjustment to shift-work schedules could also improve health and well-being, and reduce sick leave and personnel attrition and turnover [34]. Combining this lighting intervention with other strategies (e.g. melatonin administration and regular food consumption) could potentially further improve adjustments to, and satisfaction with, night-work schedules [35, 36]. Despite potential benefits, implementing such an intervention in real-world settings requires careful consideration of other factors, such as personnel acceptance and uptake, and the ease of integration with existing light sources (e.g. from computer screens and smartphones). There are also unique considerations within the submariner context, including movement between different workspaces and likely unavoidable last-minute work schedule changes further impacting sleep and circadian effects on subsequent work-shift performance. Nonetheless, improvements in circadian adjustment from circadian-informed lighting appear likely to be achievable under these challenging operational conditions with an appropriately considered approach.
While the tightly controlled study design has methodological strengths, there are some notable limitations. This study was designed around the submariner work context, but in a sample of only healthy non-shift workers. Thus, findings may not generalize to other workplaces where participant demographics, health status, lighting conditions, and work practices may have variable effects on circadian outcomes. We used a within-participants crossover design for which several participants were unable to return for the second study visit, somewhat reducing study power. Nevertheless, large effects of circadian lighting on both DLMO and Tmin timing were clearly demonstrated, and may have been more difficult to detect with a parallel groups design. The study did not have baseline core temperature measures or use salivary collection over a longer nocturnal period to assess daily shifts in the overall melatonin secretion profile. However, more intensive measures are costly and impractical and there were clear and consistent large pre-versus post melatonin profile and daily Tmin shifts.
The current study clearly demonstrates considerable potential for circadian-informed lighting interventions to markedly improve the central circadian clock adjustment to night work. There are several areas for future research to further explore the efficacy and implementation of such interventions. While our primary focus was on the circadian response to light cues, it is important to acknowledge that peripheral clocks, which help to regulate downstream molecular and physiological processes involved in body and organ functions, may show differential re-timing effects [37]. In a submariner context, lighting interventions applied to different shift-work schedules, such as the more classic 6 on/6 off schedule, could be particularly useful. Shift-work contexts such as hospitals and manufacturing plants, and other 24/7 operations could substantially benefit from improved circadian lighting, enhancing employees’ well-being by considering light’s impact on circadian rhythms. Future lighting interventions may benefit from more specific consideration of blue-enriched light exposure timing relative to daily changes in the circadian Tmin, to better personalize lighting interventions to specific individuals or work-groups on similar work shifts. This approach is also likely to be particularly efficacious in maintaining stable circadian rhythms in workplaces with relatively stable work shifts. Ultimately, adaptive circadian lighting is likely to be the most effective at rapidly changing shift-work schedules and accelerated recovery from each shift-work change.
Supplementary material
Supplementary material is available at SLEEP online.
Author Contributions
A.G., N.L., J.M., N.S., B.L., L.L., G.M., S.B., J.D., E.K., A.V., R.A., D.J.E., H.S., and P.C. developed the study concepts and aims. A.G., J.M., N.S., B.L., H.S., and P.C. performed data extraction. A.G., B.T., H.S., and P.C. performed data analysis. All authors provided important insight on data interpretation and drafting of the manuscript. All authors approve of the final version of the manuscript.
Acknowledgments
We gratefully acknowledge the support from Defence Science and Technology Group personnel and our colleagues at Flinders University, University of South Australia, and University of Adelaide. We are particularly grateful to the study participants, casual staff and students who assisted with data collection and analysis, and David Kennaway and Mark Salkeld for melatonin assays. We express appreciation to Ranjay Chakraborty for conducting the eye examination at Flinders Vision.
Data Availability
Data are available from the authors upon reasonable request subject to data sharing and ethics approvals.
Financial disclosure
Financial disclosure: This research was supported by the Maritime Division of the Defence Science and Technology Group through the Research Network for Undersea Decision Superiority. This research was also financially supported by the office for the Deputy Vice Chancellor of Research (Flinders University), the College of Medicine and Public Health (Flinders University), REDARC, and Compumedics Ltd. Nonfinancial disclosure: A.G., N.L., J.M., N.S., B.L., L.L., G.M., S.B., J.D., E.K., A.V., R.A., D.J.E., H.S., and P.C. all reported support for the present manuscript: Research Network for Undersea Decision Superiority. Research funding paid to institution: Flinders University, College of Medicine and Public Health (Flinders University), Compumedics Ltd, REDARC. A.G., J.M., N.S., G.M., D.J.E., H.S., and P.C. reported in-kind contribution Flinders University Travel funding to present parent study findings at a conference. NL reported grants or contracts from any entity unrelated to this work from National Health and Medical Research Council of Australia Grant, Philips Respironics Research grant, The Hospital Research Foundation Research grant, ResMed Foundation Research grant. Receipt of equipment, materials, drugs, medical writing, gifts, or other services unrelated to this work from ResMed Provision and Philips Provision. BT reported no disclosures. BL reported the following disclosures unrelated to the current work. Grants or contracts from National Health and Medical Research Council of Australia (BL is funded by an NHMRC Investigator Grants Emerging Leadership 1 (2025886)). Funds paid to institution: Withings Ltd In-kind contribution for unrelated work. Receipt of equipment, materials, drugs, medical writing, gifts, or other services: Withings Ltd In-kind contribution for unrelated work. LL reported disclosures unrelated to the current work from Royalties or licenses: Re-time Pty Ltd. Payment or honoraria for lectures, presentations, speakers bureaus, manuscript writing, or educational events: National Judicial College Association Workshop on sleep and insomnia. Support for attending meetings and/or travel: Re-time Pty. Ltd. Patents planned, issued, or pending: Re-time Pty. Ltd. Stock or stock options: Re-time Pty. Ltd. GM reported grants or contracts from any entity unrelated to this work from Flinders University Research. JD reported the following disclosures unrelated to the current work. Grants or contracts from any entity unrelated to this work from National Institute for Forest Products Innovation, Australian Bluegum Plantations, OneFortyOne, Hancock Victorian Plantations, SFM, HQPlantations Pty Ltd, Midway Ltd, Timberlands Pacific, SA Water, Rail Industry Safety and Standards Board and National Health and Medical Research Council. Royalties or licenses: Royalties from Cambridge University Press. Payment or honoraria for lectures, presentations, speakers bureaus, manuscript writing, or educational events: Human Factors and Ergonomics Society. Leadership or fiduciary role in other board, society, committee, or advocacy group, paid or unpaid: Resilient Youth Australia Scientific Advisory. EK and SB reported grants or contracts from any entity unrelated to this work from Australian Research Council Research, Flinders University Research, College of Education, Psychology, and Social Work (Flinders University). AV reported the following disclosures unrelated to the current work. Research funding from National Health and Medical Research Council of Australia, Philips Respironics and ResMed. Research/equipment support from ResMed, Phillips, Withings and Sleeptite. Leadership or fiduciary roles within various boards, societies, committees, and advocacy groups Academic Chair for the Sleep Health Foundation Consumer Reference Panel Chair of the Steering Committee for the Australian Sleep and Alertness Consortium (ASAC), Board member for both the Sleep Health Foundation and the Australasian Sleep Association, Various unsalaried leadership positions within institutional and professional contexts. Patents planned, issued, or pending: PCT/AU2019/051147 Decision Support Software System for Sleep Disorder Identification Decision support algorithms for online sleep disorder screening survey. This work is unrelated to the submitted manuscript. RA reported the following disclosures unrelated to the current work. Grants or contracts from any entity unrelated to this work from National Health and Medical Research Council of Australia Grant, Withings Ltd and ResMed Foundation Grant. Leadership or fiduciary role in other board, society, committee, or advocacy group, paid or unpaid: Sleep Health Foundation Board member. Receipt of equipment, materials, drugs, medical writing, gifts, or other services unrelated to the current work from Withings Ltd, ResMed and Philips. Outside the current work, DJE has had research grants from Bayer, Takeda, Invicta Medical, Apnimed, Withings, Eli Lilly and has served on Scientific Advisory Boards for Apnimed, Invicta, Mosanna and as a consultant for Bayer, Invicta Medical, Apnimed, and Mosanna. Speaker fees paid to individual for work outside the current project Nox Medical, Philips and ResMed. Support for attending meetings: Takeda Travel funding to present study findings at an international conference for work outside the current project. Patents planned, issued, or pending: OSA endo-phenotyping treatment algorithm (PCT/AU2020/050506) No revenue to date (work outside the current project). DJE is supported by a National Health and Medical Research Council of Australia Investigator Grant (1196261). HS reports consultancy and/or research support unrelated to this work from Re-Time Pty Ltd, Compumedics Ltd, the American Academy of Sleep Medicine Foundation, Flinders University and withings Ltd. PC reported the following disclosures unrelated to the current work. Grant and equipment support from the National Health and Medical Research Council of Australia, Medical Research Future Fund, Minister for Innovation and Skills, South Australia/Science, Technology and Commercialization, Flinders Foundation, Invicta Medical, Garnet Passe and Rodney Williams Foundation, and a Flinders University collaboration grant with Compumedics Ltd. Patents planned, issued, or pending: OSA decision support tool US-20210327584-A1 License fee paid to institution to support a PhD stipend.
References
Author notes
Co-senior authors.
Comments