-
PDF
- Split View
-
Views
-
Cite
Cite
Dorine Labrosse, Lugan Flacher, Thomas Bachelart, Ahcène Boumendjel, Incompatibility of Y-site-administered drugs: the case of acyclovir and ciprofloxacin, RPS Pharmacy and Pharmacology Reports, Volume 3, Issue 4, October 2024, rqae021, https://doi.org/10.1093/rpsppr/rqae021
- Share Icon Share
Abstract
Multiple drug administration is a common practice in hospitals and clinics. This is the case when multiple pathologies are treated. In this context, frequently, antivirals and antibiotics are co-administrated either simultaneously or sequentially ignoring potential incompatibilities. In this study, we focused on an antiviral (acyclovir) and an antibiotic (ciprofloxacin) compatibility/incompatibility when they are co-administrated.
In this study, we focused on the effect of drug ratio, pH, and delay by implementing robust high-performance liquid chromatography methods to determine the main factors leading to potential modification of concentration of one of the two drugs in the mixture.
The results showed that acyclovir is quantitatively recovered in all conditions related to pH, concentration, and time (up to 24 hours). However, ciprofloxacin is strongly altered depending on the acidic and basic conditions, the quantity of ciprofloxacin used, and the time after mixing the two drugs. The most valuable piece of information is that the ratio of acyclovir/ciprofloxacin is crucial.
The volume ratio of acyclovir/ciprofloxacin (prepared at 2 mg/ml each) should be calculated and managed to provide at least two-fold quantity of acyclovir versus ciprofloxacin or at least nine-fold quantity of ciprofloxacin versus acyclovir to maintain the administrated doses and prevent physicochemical interactions.
Introduction
Intravenous (IV) drug administration plays a pivotal role in the treatment of severe infections and heavy pathologies. To ensure effective patient care, healthcare professionals frequently encounter the need for simultaneous or sequential administration of multiple medications. However, the co-administration of drugs via IV injection, best known as Y-site administration raises concerns about incompatibility and the potential for physicochemical interactions (PCIs) that may compromise therapeutic efficacy, patient safety, or both [1, 2].
PCIs can take different forms: oxidation [3], hydrolysis [4] of the active compound, reaction with an excipient [5], and reactions with the solvent [6]. However, the most common is the precipitation of the active species due to acid/base reactions [7]. For each PCI to appear, certain conditions need to be met. Those can be the combination of two species that can react together, the presence of certain salts that are incompatible, or physico-chemical conditions such as the temperature, the concentration, the time of contact, or the pH.
The consequences of PCIs can be multiple. First, it can result in a loss of active ingredient (following precipitation or degradation) and therefore, an incorrect dose delivered to the patient. Catheters could become clogged due to the formation of a precipitate, preventing the medication to be delivered. In addition, the precipitates can cause embolism if they reach the general circulation, crystals could be deposited in the organs and cause inflammation. Following the gas release, gas bubbles could reach the patient’s systemic circulation, thereby creating a risk of gas embolism. All of these problems are likely to lead to a reduction in the effectiveness of medications, therapeutic failure, or even jeopardize the patient’s vital prognosis.
In this context, incompatibility studies are essential to assess the physical and chemical stability of drug combinations and to determine the potential for precipitation, pH changes, drug degradation, or the formation of byproducts with potential side effects [8–10]. Such investigations provide valuable insights into the feasibility and safety of co-administration, helping healthcare professionals make informed decisions regarding IV drug therapy and ensuring optimal patient outcomes [11, 12]. The incompatibility issue concerns the major classes of drugs and especially those bearing acidic and/or basic characters [7]. However, observing the incompatibility is not sufficient. For a prescription containing multiple injectable drugs that are incompatible, a safe administration would require sequential injection with rinsing of the catheter or the use of multiple lines. It seems necessary to identify the cause of the PCI and the parameters that govern them.
In this study, we focused on two major drugs: acyclovir and ciprofloxacin (Fig. 1). Acyclovir (9-[(2-hydroxyethoxy)methyl]guanine), a nucleoside analogue of the guanosine family, is a widely used antiviral drug as tablets, topical creams, and IV injections [13]. Neutral acyclovir suffers from poor solubility in water (1.2–1.6 mg/ml at 25°C), limiting its absorption into the gastrointestinal tract when taken orally [14, 15]. Therefore, its injectable form is basic maintaining a deprotonated state of its hydroxyl group and increasing its solubility in water.
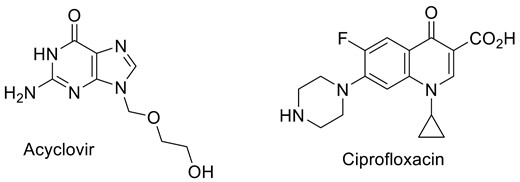
The usual acyclovir injection is 25 mg/ml combined with sodium hydroxide at 10.85–11.50 pH range. The most commonly used dose is 5–10 mg per kg of body weight, injected into a vein over a 1-hour period, and repeated every 8 hours for up to 10 days. Electrolyte/glucose diluent solutions are normally used, and literature recommends concentrations of below 7 mg/ml to moderate venous inflammation due to extravasation drug leakage outside the vein, with pain, swelling, or burning sensations.
Ciprofloxacin (1-cyclopropyl-6-fluoro-4-oxo-7-piperazin-1-ylquinoline-3-carboxylic acid) is a broad-spectrum antibiotic agent in the fluoroquinolone class used to treat bacterial infections [16].
Ciprofloxacin is available as an injection for IV infusion solutions at 0.2% (2 mg/ml) supplied in 200 ml infusion bags. Ciprofloxacin pH is 3.5–4.6 and not compatible with unstable drug agents at this pH. Ciprofloxacin can be infused over a period of at least 1 hour via direct infusion or a Y-type set. With “piggyback” administration, temporary discontinuation of other solutions is recommended during IV infusion. Diluting infusion into a larger vein is advised to minimize venous irritation and discomfort pain from the infusion.
Usual administration IV is 400 mg every 8–12 hours depending on the targeted treatment.
Its injectable form is acidic maintaining the protonation and the ammonium form of its secondary amine making ciprofloxacin soluble in water.
The mixture of those two preparations will possess a different pH that may not allow each drug to remain in its most soluble form and therefore causing a PCI by precipitation. The simultaneous administration of acyclovir and ciprofloxacin may be necessary in certain clinical scenarios, such as the treatment of patients with co-existing viral and bacterial infections or the prevention of secondary infections during viral outbreaks [17]. Therefore, it is crucial to evaluate the incompatibility of acyclovir and ciprofloxacin solutions when co-administered via IV infusion.
Although individual compatibility/incompatibility studies were conducted for acyclovir and ciprofloxacin with other medications [18, 19], limited or even non-existent scientific data regarding their compatibility when combined in a co-administration therapy (Y-site administration). Considering the widespread use of these drugs, the absence of comprehensive compatibility information recalls significant challenges in clinical practice. Consequently, healthcare professionals must resort to empirical approaches or rely on anecdotal evidence to guide the co-administration of these two drugs.
The aim of this study is to investigate the incompatibility of acyclovir and ciprofloxacin solutions during Y-site administration and identify conditions (ratios for a given concentration) in which the two drugs could be concomitantly administrated. Determine which parameters can lead to a PCI and identify which molecules are concerned. By employing rigorous analytical techniques and monitoring key physicochemical parameters, we aim to provide evidence-based recommendations regarding the feasibility and safety of their simultaneous administration.
Materials and methods
Products, solvents, and consumables
The following products, solvents, and consumables have been used: acyclovir (Mylan) powder for injectable solution, ciprofloxacin (Fresenius Kabi) 400 mg/200 ml, sodium chloride (Fresenius Kabi) 0.9% (9g/1000 ml), methanol HPLC Grade (Carl Roth), distilled water, phosphoric acid (Sigma-Aldrich) 85%, di-sodium hydrogenophosphate (Prolabo), sodium hydroxide tablets (ChemLab), hydrochloric acid (ChemLab), vials (Thermo Fisher Scientific), Eppendorf, and Falcon tubes.
Instruments and chromatographic conditions
Chromatographic analyses were performed using the Agilent 1100 series HPLC system (Agilent Technologies) equipped with degasser G1322A, binary pump G1312A, autosampler G1329A, thermostatted column compartment G1316A and MWD UV detector (G1365B). The compounds were separated on a Select® Peptide CSHTM C18, 150 × 4.6 mm, 2.5 μm, 130 Å column at 40°C. The injection volume was fixed at 10 µl. Mobile phase, composed of distilled water (containing Na2HPO4, 20 mM and phosphoric acid, pH = 3) and methanol (60/40, v/v), was used for isocratic elution at a flow rate of 0.5 ml/min. The detector monitored the elution at 205, 260, and 273 nm for simultaneous detection of acyclovir and ciprofloxacin over a period of 10 minutes. The obtained chromatograms were analyzed using ChemStation B.04.03 software.
Stock solution preparation
The sampling of adequate volume was realized with 200 µl and 1000 µl micropipettes (Eppendorf France). Ciprofloxacin stock solution was prepared directly by sampling the commercial solution 2 mg/ml, a concentration currently used in clinical practices [20]. Acyclovir stock solutions were prepared by weighting powder with a precision balance (Denver Instrument) and then, reconstituted in a falcon tube with sodium chloride 0.9% at 2 mg/ml. The solutions were manually stirred and sonicated for 1 minute with a Sonicator (Elma). For the screening experiment, stock solutions were prepared as follows: Ciprofloxacin (2 mg/ml); acyclovir (500 mg) was dissolved in NaCl 0.9% (100 ml) at 5 mg/ml.
Screening of solutions preparation by visual inspection
At the beginning of our investigation on acyclovir and ciprofloxacin, we performed a series of tests analyzed by visual inspection to have a glimpse of the behavior of those two drugs when administrated concomitantly. Acyclovir (concentration, reconstitution solvent) and ciprofloxacine (concentration, reconstitution solvent) were prepared at five volume ratios (1/1, 1/2, 2/1, 1/9, 9/1). Samples were prepared in 0.5 ml Eppendorf tubes (non-sterile), the appropriate volume of each drug (to reach the desired ratio) was added with a precision pipette and each sample was triplicated. The visual inspections were performed with the same background and the same light source and physical stability was assessed by two observers. A second and third visual inspection was performed at t = 2 hours (± 20 minutes) and at t = 13 hours (±1 hour). Each result was described according to the following nomenclature: Q0: clear solution; Q1: small amount; Q2: important amount; Q3: huge amount. The aspect of the solution is described as follows: P: particles/precipitate; C: crystals.
Assays of stability of acyclovir and ciprofloxaxin at different pH values
To study the behavior of single drug solutions, seven samples containing 1 ml of stock solution of acyclovir at 2 mg/ml (see stock solutions preparation, Supplementary Table S1) were prepared by adjusting the pH with successive additions of 10 µl of hydrochloric acid or sodium hydroxide solutions at different concentrations (100 µM, 1 mM, 10 mM, 100 mM, 1M, 6M). Tubes were shaken manually. The same method and conditions were implemented to study the behavior of ciprofloxacin. Two replicates for each drugs were realized. pH measurements were taken using a pH meter (FP20 Mettler Toledo). Once the pH was adjusted, an aliquot of each sample was diluted 50 times with high-performance liquid chromatography (HPLC) mobile phase (methanol and distilled water, phosphate buffer, pH = 3, 50/50, v/v) in Eppendorf tubes to reach a drug concentration in linearity range. To avoid sampling of crystals or precipitates, volume samplings with micropipettes were made delicately at the top of the Eppendorf tube. After dilution and agitation of tubes, samples were transferred in vials and visually inspected to check the absence or presence of precipitate and/or crystals. Then, RP-HPLC analysis was performed at 0, 2, 4, and 24 hours.
Compatibility studies
Drug mixtures preparation
From acyclovir and ciprofloxacin stock solutions at 2 mg/mL, drug mixtures were prepared at different volume ratios (1:1, 2:1, 1:2, 9:1, and 1:9) (for details, see Supplementary Table S1). All ratios (1:1, 1:2, 2:1, 1:9, and 9:1) were prepared in triplicate. Tubes were shaken manually. Before analyses, ratios 1:1, 2:1, and 1:2 were diluted 50 times and ratios 9:1 and 1:9, 20 times, with mobile phase (methanol and distilled water, phosphate buffer, pH = 3, 50/50, v/v) to obtain drug concentrations in the linearity ranges. To avoid sampling of crystals, volume sampling was made delicately at the top of the Eppendorf tube. After dilution and agitation of tubes, samples were transferred in vials and visually inspected to observe the presence/absence of solid impurities or crystals.
Preparation of controls for the two drugs
From acyclovir and ciprofloxacin stock solutions, controls were in triplicate for each ratio (1/1; 2/1; 1/2; 1/9; 9/1). Mimicking theoretical concentrations, controls were prepared by adding the corresponding volume of mobile phase (methanol and distilled water, phosphate buffer, pH = 3, 50/50, v/v). Each control was diluted 50 times to reach ratios 1:1, 2:1, and 1:2 and 20 times for ratios 9:1 and 1:9 with the same scheme as for drug mixtures.
Physical compatibility studies
The physical changes were checked with an unaided eye, facing daylight. In addition to the pH measurement, we particularly observed for each solution corresponding to different ratios: precipitation, turbidity, color change, and gas bubbles by comparison to the control solutions.
Chemical compatibility studies
RP-HPLC analyses were performed on diluting samples immediately after preparation and after 2, 4, and 24 hours of contact. For controls, RP-HPLC analyses were realized only after preparation. The obtained data concerned peak area and permitted to calculate the mass of each drug in mixtures. A recovery percentage was defined as the ratio between the measured mass and the theoretical mass of the drug in the mixture. Therefore, a chemical incompatibility was characterized by a recovery percentage less than 90%, i.e. a decrease of 10% (5% in the case of drugs with a narrow therapeutic margin) of measured concentration compared with the theoretical concentration. The data were processed using Excel software.
HPLC method validation
Validation of parameters of the HPLC method was assessed in accordance with International Conference on Harmonization guidelines concerning linearity as well as the limits of detection (LOD) and quantification (LOQ) [21, 22].
Standard solution preparation
An external calibration method is useful to quantify the amount of drug remaining in test samples. For acyclovir, seven samples were prepared from stock solution (2 mg/ml) in duplicate via successive dilution (0.2; 0.15; 0.1; 0.05; 0.025; 0.01, and 0.005 mg/ml) with methanol and distilled water, phosphate buffer, pH = 3, 50/50. For ciprofloxacin, five samples were prepared from stock solution (2 mg/ml) in triplicate via successive dilution (0.2; 0.1; 0.05; 0.005, and 0.0025 mg/ml) with methanol and distilled water, phosphate buffer, pH = 3, 50/50. After dilution, manual agitation, transfer in a vial, and visual inspection to verify the absence of impurities or precipitates were performed. Then, samples were immediately analyzed by RP-HPLC (isocratic method, 40% methanol over 10 minutes).
System suitability
System suitability testing was carried out by determining the peak retention time and the area, and by calculating the resolution, the selectivity factor, the number of theoretical plates, and the symmetry factor [21]. This test was performed on five replicates of the ratio 1:1 (v:v) and analyzed immediately after mixing (for more details, see Supplementary Table S2).
Precision, stability, specificity, linearity, range/limit of detection, and quantification
The accuracy of the method was represented by percent relative error (CV [%]), while the precision was represented by the relative standard deviation (SD). The accuracy (recovery) and the precision were estimated on the control samples (triplicate) at 5 concentrations (0.2 mg/ml, 0.67 mg/ml, 1 mg/ml, 1.33 mg/ml, and 1.8 mg/ml). For each concentration, the mean recovery percentage, the mean relative error (CV [%]), and the mean standard deviation are described in the Supplementary Information (Supplementary Table S4). The mean percent relative error and the mean recovery were found to be 1.42% and 97.5%, respectively, while the mean RSD % was 1.36%.
The specificity of the method was considered valid if no peaks other than acyclovir and ciprofloxacin appeared on the chromatograms. Linearity was established through the construction of calibration curves using two standard ranges containing seven standard solutions for acyclovir and three standard ranges containing five standard solutions for ciprofloxacin (See Standard solutions preparation) [21]. To assert linearity, the coefficient of determination (R2) had to be higher than 0.999 [23]. The detection and quantification limits were determined using the method based on the signal-to-noise ratio: LOD = (3 × h × m)/(2 × H) and LOQ = (10 × h × m)/(2 × H) (with h = higher fluctuation of the background noise in the blank, determined over a distance equal to five times the width at mid-height of the peak corresponding to the product concerned and located on either side of the place where the peak would be observed; H = height of the peak concerned; m = theoretical drug mass).
Results and discussion
Screening of drug mixtures at different ratios
Acyclovir and ciprofloxacin were mixed at five different ratios (volume/volume). The quantity of each drug is the same in each volume. Visual observation were made at three different time intervals. As given in Table 1, as expected at time zero, the solution was limpid and clear indicating the drugs are more likely to be intact and they are in their expected forms. After 2 hours, small amounts of crystals were observed except when the amount of acyclovir was in large excess (ratio 9/1). An important amount of crystals was observed after 24 hours for ratios 1/1, ½, and 1/9. However, no crystals were observed when acyclovir was prominent (ratios 2/1 and 9/1). These observations were confirmed by recovery studies using HPLC analyses (see section Compatibility/incompatibility studies by HPLC).
Ratio . | 1/1 . | 1/2 . | 2/1 . | 1/9 . | 9/1 . | ||||||||||
---|---|---|---|---|---|---|---|---|---|---|---|---|---|---|---|
Time (h) | 0 | 2 | 24 | 0 | 2 | 24 | 0 | 2 | 24 | 0 | 2 | 24 | 0 | 2 | 24 |
Observation | 0 | 1 | 2 | 0 | 1 | 2 | 0 | 1 | 0 | 0 | 1 | 2 | 0 | 0 | 0 |
Ratio . | 1/1 . | 1/2 . | 2/1 . | 1/9 . | 9/1 . | ||||||||||
---|---|---|---|---|---|---|---|---|---|---|---|---|---|---|---|
Time (h) | 0 | 2 | 24 | 0 | 2 | 24 | 0 | 2 | 24 | 0 | 2 | 24 | 0 | 2 | 24 |
Observation | 0 | 1 | 2 | 0 | 1 | 2 | 0 | 1 | 0 | 0 | 1 | 2 | 0 | 0 | 0 |
0: clear solution; 1: small amount of crystals; 2: important amount of crystals.
Ratio . | 1/1 . | 1/2 . | 2/1 . | 1/9 . | 9/1 . | ||||||||||
---|---|---|---|---|---|---|---|---|---|---|---|---|---|---|---|
Time (h) | 0 | 2 | 24 | 0 | 2 | 24 | 0 | 2 | 24 | 0 | 2 | 24 | 0 | 2 | 24 |
Observation | 0 | 1 | 2 | 0 | 1 | 2 | 0 | 1 | 0 | 0 | 1 | 2 | 0 | 0 | 0 |
Ratio . | 1/1 . | 1/2 . | 2/1 . | 1/9 . | 9/1 . | ||||||||||
---|---|---|---|---|---|---|---|---|---|---|---|---|---|---|---|
Time (h) | 0 | 2 | 24 | 0 | 2 | 24 | 0 | 2 | 24 | 0 | 2 | 24 | 0 | 2 | 24 |
Observation | 0 | 1 | 2 | 0 | 1 | 2 | 0 | 1 | 0 | 0 | 1 | 2 | 0 | 0 | 0 |
0: clear solution; 1: small amount of crystals; 2: important amount of crystals.
Influence of pH on recovery of acyclovir and ciprofloxacin
The influence of pH on the recovery of both drugs was studied at seven different pH ranging from 3 to 11 (Fig. 2). For acyclovir, the influence of pH was insignificant as the recovery was quantitative at different time intervals (Fig. 2, top panel). However, the pH and time effects on ciprofloxacin recovery are more marked compared with acyclovir (Fig. 2, bottom panel).
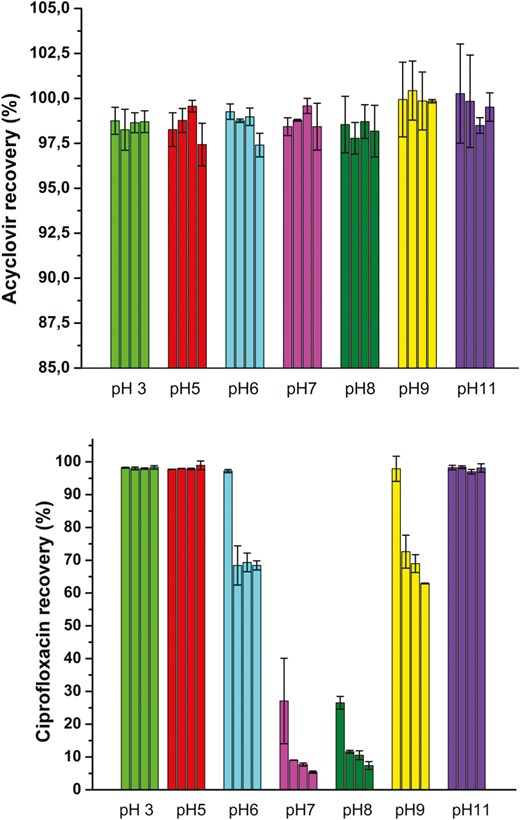
Recovery percentage of acyclovir (top panel) and ciprofloxacin (bottom panel) at different pH. For each pH conditions, the recovery was measured at 4 time points (0 hours, 2 hours, 4 hours, 24 hours). Experiment have been performed in duplicates.
At pH 7 and 8, the recovery of ciprofloxacin drops strongly even at time zero (<30%) reaching less than 10% after 24 hours. From the pKa values of ciprofloxacin (Fig. 3) [24, 25], at neutral pH and near the isoelectric point, the carboxyl and the secondary amino groups are mainly charged but the sum of the charges is close to zero and the molecules as a whole is neutral resulting in the solubility dropping (Fig. 3). At pH = 6, around 75% of the drug was recovered after 2, 4, and 24 hours. The same behavior is observed at basic conditions (pH = 8.72 and 9.24). At strong acidic or basic conditions, the recovery is almost total. As can be expected, ciprofloxacin is an amphoteric drug allowing that at acidic and basic conditions, the drug is in its ionized form which is correlated with higher solubility. Although it was reported that some issues can be raised regarding the stability of ciprofloxacin in acidic and basic conditions [26–28], in our case, we did not observe any degradation as evidenced by the recovery results and the absence of impurities on HPLC chromatogram.
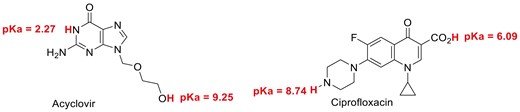
Compatibility/incompatibility studies by HPLC
Validation of the RP-HPLC method
In order to assess that the system is working perfectly, we conducted the system suitability testing regarding the retention time, the resolution, and the selectivity factor [21] (For more details, see Supplementary Table S2).
The calibration curves of acyclovir and ciprofloxacin were realized by reverse-phase high-performance liquid chromatography and are shown in Fig. 4. The calibration curve of acyclovir indicates a linearity of the peak area from 0.05 to 2 µg (mass concentration of 0.005 to 0.2 mg/ml), whereas for ciprofloxacin it indicates a linearity of the peak area from 0.025 to 2 µg of ciprofloxacin (mass concentration of 0.0025 to 0.2 mg/ml) (for more details, see Supplementary Table S3). These curves were used to monitor the percentage of acyclovir and ciprofloxacin in mixtures at different ratios and different time intervals.
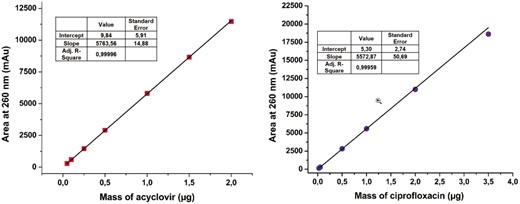
Calibration curve of acyclovir and ciprofloxacin. Calibration was realized at 260 nm on two standard ranges for acyclovir and for ciprofloxacin (n = 3).
Compatibility/incompatibility of acyclovir and ciprofloxacin mixture
The recovery of acyclovir and ciprofloxacin was measured by RP-HPLC at five different ratios (1/1; 2/1; 1/2; 9/1; and 1/9) and time intervals (from 0 to 24 hours) (Fig. 5). At ratio 1/1, the pH (7.9) was within the two pKa of ciprofloxacin (6.1 and 8.7) making the globally neutral form of ciprofloxacin (that possess the lowest solubility) the major species. In accordance with that observation, the recovery of ciprofloxacin is severely dropped after 2 hours of contact. After 24 hours, less than 20% of ciprofloxacin was recovered. At 2/1 ratio (pH = 8.6), a total recovery of both drugs is measured. Even though, the globally neutral form represents close to 50% of the total amount of ciprofloxacin, the lower concentration imposed by the drug ratio (0.66 mg/ml) allows it to remain below the solubility limit. When the latter ratio was inversed (from 2/1 to 1/2), the recovery of acyclovir was total whereas the recovery percentage of ciprofloxacin was around 35, 30, and 18% after 2, 4, and 24 intervals, respectively. The measured pH (6.3) which is close to the ciprofloxacin carboxylic acid pKa (6.1) also indicates that the globally neutral form represents close to 50% of the total amount. However, the concentration of ciprofloxacin (1.32 mg/ml) is much higher explaining why the ciprofloxacin precipitates. When the acyclovir is present in large excess (ratio 9/1), the recovery of both drugs is almost quantitative which is in agreement with what was observed with a ratio 2/1. The ratio 1/9 gave the same results as ratio 9/1 with both compounds recovered almost quantitatively. For those two ratios, the pH (9.7 and 5.1 for ratio 9/1 and 1/9, respectively) indicate that the main form of ciprofloxacin is a charged one making it soluble even at concentrations close to 2 mg/ml for the 1/9 ratio.
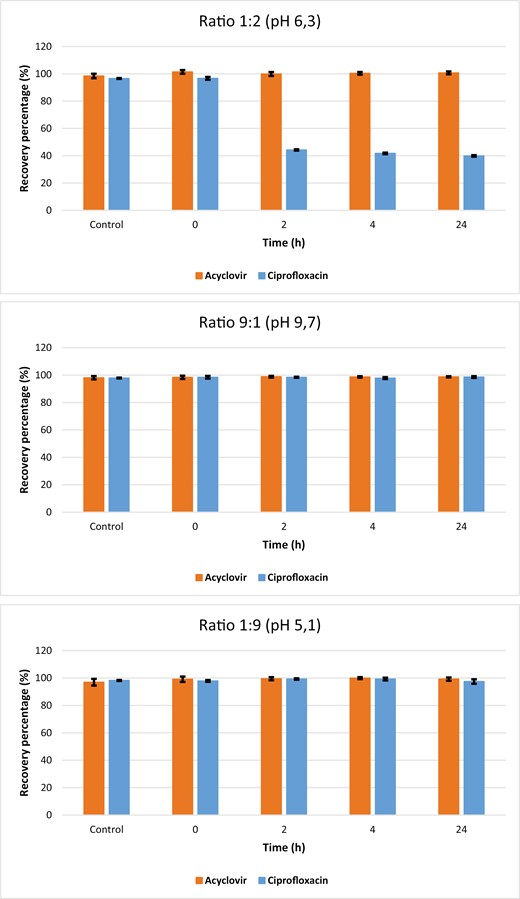
Recovery of acyclovir and ciprofloxacin at different ratios and time intervals. Each point has been performed in triplicate (n = 3).
Interactions between acyclovir and ciprofloxacin are not documented. However, this does not necessarily mean that no interactions exist. Our study highlights clearly that caution should be taken when these drugs are concomitantly taken, via Y-site administration. Particularly, three parameters must be monitored, the pH, the initial concentration, and the ratio of the two drugs (that strongly influence the pH and the final concentration). These three parameters are tightly linked to the amphoteric characteristics of drugs even though, the acyclovir and ciprofloxacin are globally incompatible, they can still be co-administered if the appropriate conditions are used.
Conclusion
This study intends to fill the existing knowledge gap and contribute to the optimization of IV drug therapy, enhancing patient care and promoting rational prescribing practices. By systematically evaluating the compatibility of these commonly used medications, we can ensure safer and more effective treatment options, thereby improving patient outcomes and advancing the field of pharmacotherapy. The most striking information deals with the ratio of acyclovir/ciprofloxacin used for Y-site IV administration. An equal ratio leads to bad recovery of ciprofloxacin. Excess of acyclovir versus ciprofloxacin (ratio 2/1 and higher) leads to almost full recovery of both drugs. When one of the drugs is present in large excess (ratios 1/9 and 9/1), a full recovery of both drugs was observed.
The results obtained from this investigation show that mixtures of acyclovir (2 mg/ml)/ciprofloxacin (2 mg/ml) at volume ratios 9/1, 2/1, and 1/9 are physically and chemically compatible in simulated Y site administration for a period of 24 hours ensuring recovery of at least 90% of the initial amount of each drug. Moreover, parenteral injection of formulation with a pH greater than 9 may cause necrosis while a pH lower than 3 may cause pain and phlebitis [29, 30]. The active forms of ciprofloxacin and acyclovir are the neutral ones mainly due to their lipophilicity. Upon entering the patient system, drugs undergo simultaneous dilution and pH changes toward pH 7.4 due to blood buffering effects. We showed that at 2 mg/ml acyclovir was fully recovered whatever pH conditions were used. For ciprofloxacin, the 2/1 ratio contained mostly the neutral form and remained soluble at a pH close to physiological value.
Therefore, the volume ratio 2/1 (acyclovir/ciprofloxacin) possessing a pH of 8.7 should prevent PCI before entering the patient system and limit risks due to blood buffering.
The observations reported in this study are valid for the formulations tested and additional experiments should be conducted if another formulation is used. In vivo solubility testing to assess the risk of precipitation upon dilution in blood has not been performed. Even though the concentration of acyclovir and ciprofloxacin tested are below the administration recommendations maximum, such study may provide useful insights.
Acknowledgements
The authors are thankful to Dr. Edwige NICOLLE (Faculty of Pharmacy of Grenoble) for the instructive discussion.
Author contributions
D.L. performed the experiments. T.B. performed a part of the experiments, supervised the HPLC studies, participated in the project conceptualization, and participated in the manuscript writing. L.F. participated in the project conceptualization. A.B. supervised the study and wrote the original draft of the manuscript.
Conflict of interest
The authors declare no conflict of interest.
Funding
None declared.
Data availability
The data presented in this manuscript are included in the published articles or available from the corresponding author upon reasonable request.