-
PDF
- Split View
-
Views
-
Cite
Cite
Yu Sun, Song Chen, Ming Pei, Comparative advantages of infrapatellar fat pad: an emerging stem cell source for regenerative medicine, Rheumatology, Volume 57, Issue 12, December 2018, Pages 2072–2086, https://doi.org/10.1093/rheumatology/kex487
- Share Icon Share
Abstract
Growing evidence indicates that infrapatellar fat pad (IPFP)-derived stem cells (IPFSCs) exert robust proliferation capacities and multilineage differentiation potentials. However, few papers summarize the advantages that the IPFP and IPFSCs have in regenerative medicine. In this review we delineate the development and anatomy of the IPFP by comparing it with an adjacent fibrous tissue, synovium, and a more frequently harvested fat depot, subcutaneous adipose tissue. Furthermore, we explore the similarities and differences of stem cells from these three tissues in terms of IPFSCs, synovium-derived stem cells and subcutaneous adipose tissue–derived stem cells in proliferation capacity and tri-lineage differentiation potentials, including chondrogenesis, osteogenesis and adipogenesis. Finally, we highlight the advantages of IPFSCs in regenerative medicine, such as the abundant accessibility and the ability to resist inflammation and senescence, two hurdles for cell-based tissue regeneration. Considering the comparative advantages of IPFSCs, the IPFP can serve as an excellent stem cell source for regenerative medicine, particularly for cartilage regeneration.
The infrapatellar fat pad is unique in niche stem cell proliferation and multilineage differentiation potential.
Infrapatellar fat pad–derived stem cells exhibit a unique proliferation capacity in an age-independent manner.
Infrapatellar fat pad–derived stem cells display an extraordinary capacity toward chondrogenesis.
Introduction
The incidence of tissue defects in cartilage, bone and adipose gradually increases due to various injuries and diseases, especially for age-related degenerative diseases. Autologous and allograft transplantation is a promising method, but most studies have failed to meet the threshold level of therapeutic requirements due to the limited capacity for regeneration and self-repair [1]. Recently, cell-based tissue engineering has provided a potential alternative for regenerative medicine by coordinating chemical factors, mechanical stimulation and various scaffolds [2–4]. Due to robust proliferative capacity and multilineage differentiation potentials, adult mesenchymal stem cells (MSCs) have been widely used as an engineering stem cell source for tissue regeneration [5–7].
Increasing evidence has shown that mesenchymal stem/progenitor cells from the infrapatellar fat pad [IPFP; IPFP-derived stem cells (IPFSCs)] exhibit unique properties in proliferation capacity and multilineage differentiation potential as well as abundant availability [6, 8]. To date, most review papers regarding IPFP tissue have focused on its role in OA [9, 10]. Few papers have summarized the advantages that IPFP and IPFSCs have in regenerative medicine. In this review we delineate the development and anatomy of IPFP by comparing it with an adjacent fibrous tissue, synovium, and a popular fat, subcutaneous adipose tissue (ScAT), considering that stem cell fate might depend on the site from which the stem cells were isolated [11]. Despite the fact that ScAT-derived stem cells (ScASCs) share similar immunophenotypes with IPFSCs [12, 13], under extreme starvation conditions, IPFP was preserved while ScAT was eliminated [10, 14]. Compared with ScAT, IPFP had remarkable amounts of dense connective tissue next to the cartilage [8], indicating that a discrepancy exists between IPFP and ScAT. Furthermore, we compare the similarities and differences of stem cells from these three tissues, in terms of IPFSCs, synovium-derived stem cells (SDSCs) and ScASCs, in proliferation capacity and tri-lineage differentiation potential, including chondrogenesis, osteogenesis and adipogenesis. Finally, we highlight the advantages of IPFSCs in regenerative medicine, such as their relative accessibility for orthopaedic surgeons and their ability to resist inflammation and senescence, two hurdles for cell-based tissue regeneration.
Development and anatomy
Previous studies have shown that adipose tissue originated from the mesoderm germ layer [15], but more recent animal study findings have revealed that adipose tissue in different anatomic locations may be derived from different embryonic origins and individual fat deposits are not functionally equivalent [16, 17] (Fig. 1). Despite being mostly structural adipose tissue, the IPFP, an intracapsular tissue, is adjacent to the synovium in the knee joint [9]. Increasing evidence has shown that IPFP develops as an enlargement of the synovial area with regard to structure and functionality [10].
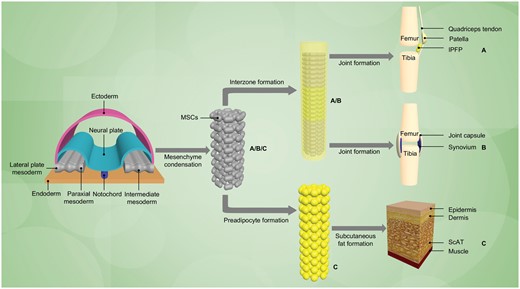
Schematic diagram for development of the IPFP, synovial membrane and ScAT
By sharing the same origin in development, the (A) IPFP and (B) synovium derive from a condensation of mesenchymal stem cells (MSCs), which have been used to model differentiating mesoderm [18] within the intermediate layer, called the interzonal mesenchyme [19]. With the upregulation of unique molecules, cavitation appears within the interzone [20] and then synovium joint formation under appropriate developmental cues. (C) ScAT is generally regarded as having a mesodermal origin [18]. Although the exact number of intermediate stages between the mesoderm/MSCs and mature adipocytes is uncertain, it is believed that the MSC gives rise to common early pre-adipocytes, which could differentiate into mature adipocytes under appropriate stimulatory conditions [21, 22]. It is notable that fat tissue present in the cephalic region has a neuro-ectodermal origin [23].
IPFP
The IPFP, also known as Hoffa’s fat pad, is a yellowish structure located intracapsularly and extrasynovially in the knee between the patellar tendon, femoral condyle and tibial plateau [9, 24]. The IPFP occupies space in the anterior knee compartment and separates the anterior structures of the knee joint, which can maintain the joint cavity by protecting articular surfaces like a cushion and facilitating the secretion of synovial fluid (SF) within the joint [25]. Due to its unique anatomic site, the IPFP is sometimes involved in pathological states such as Hoffa’s disease, post-arthroscopy fibrosis and localized nodular synovitis [26]. Histologically the IPFP is a mass of fibrous fatty tissue, mainly composed of synovial membrane and subsynovial adipose tissue [6]. Unlike ScAT, viewed as an endocrine and metabolic depot, the IPFP has higher vascularity and innervation, more closely resembling visceral fat [25, 27]. The IPFP, as a heterogeneous tissue, is regarded as adipose synovium or a synovial fat pad, which is more similar to synovium than ScAT, such as that harvested during liposuction [6].
Synovium
Synovium is a thin layer of tissue that borders the inner surface of the capsules of synovial joints. Macroscopically, synovium appears relatively white, covers joint spaces and can secrete SF for lubrication, oxygenation and nutrition to articular cartilage [28]. The synovium is the main area of pathology in many inflammatory joint diseases such as synovitis and RA [29, 30]. As a particular connective tissue, synovium is derived from a specialized mesoderm termed the interzonal mesenchyme [19]. Histologically, the mature synovium is composed of a fibrous external layer and an inner secretory layer [6, 31]. It has been reported that synovium and articular cartilage developed from the same pool of precursor cells had similar gene profiles and maintain a close relationship into adulthood [20, 32]. Synovium has considerable powers of regeneration following surgical synovectomy [33, 34].
ScAT
ScAT is a loose connective tissue mainly located immediately beneath the skin. ScAT is widely distributed throughout the human body and most of these adipose tissues are primarily in the regions of the abdomen and buttocks. The two types of classical fat tissue are white adipose tissue, which stores energy in the form of lipids, and brown adipose tissue, which generates body heat [17]. Additionally, beige adipose tissue, made of inducible ‘brown-like’ adipocytes and developed in white fat in response to various activators, is an intermediate type that can regulate metabolism [35]. Considered an endocrine system, ScAT secretes a number of bioactive substances that exhibit anti-inflammatory and immunomodulatory characteristics [36]. ScAT, generally regarded as having a mesodermal origin that is similar to bone and cartilage [23], contains a large number of cells that include adipocytes and stromal vascular fraction cells such as fibroblasts, vascular endothelial cells and a variety of immune cells [37]. ScAT has become an attractive source of adult stem cells due to its abundance and easy accessibility by liposuction or resection.
Comparison of stem cells derived from IPFP, synovium and ScAT
The ideal MSC source for regenerative medicine should maintain persistent proliferation capacity and exhibit excellent multidifferentiation potentials. Lopa et al. [13] found that human IPFSCs and donor-matched ScASCs exhibited similar immunophenotypes when they were undifferentiated, including CD13+, CD29+, CD44+, CD73+, CD90+, CD105+, CD166+, CD31− and CD45−. Despite an age-related decline in stem cell properties [38, 39], increasing reports have shown that IPFSCs are more similar to SDSCs than to ScASCs [6, 12, 13].
Proliferation
Many studies indicate that IPFSCs exert various proliferation properties (Table 1). The doubling time of human IPFSCs from passage 2 to 3 was ∼48–60 h [40, 41]. IPFSCs were reported to proliferate rapidly under stimulation by various environmental conditions, such as serum stimulation, low oxygen, TGF-β and basic fibroblast growth factor 2 [42–46]. Recently, some findings suggest that expansion on decellularized extracellular matrix (dECM) could significantly promote IPFSC proliferation [47, 48], possibly through the reduction of reactive oxygen species (ROS) in expanded cells [47].
. | Tissue donor . | Assay . | Results . | Reference . |
---|---|---|---|---|
Non-arthritis donor | Calf knee joints (3 months old) | PD and CFU-F assay | IPFSCs had lower proliferation than SDSCs | [49] |
Porcine knee joints (n = 3) (4 months old) | Cell yield and PDT | FGF-2 promoted IPFSC proliferation | [42] | |
Male C57BL/6J mice (fed a high-fat or a low-fat diet) | Cumulative fold increase | Obese IPFSCs had comparable proliferation in vitro to lean IPFSCs despite a trend toward increased proliferation in obese compared with lean donors | [50] | |
Minipigs (n = 2) (5 months old) | Cell counting | dECM expansion enhanced IPFSC proliferation and maintained stem cell morphology | [47] | |
Patients for partial meniscectomy or ACL reconstruction (n = 15) (26–68 years old) | PD | TFP supplementation significantly increased the proliferation rate of meniscus cells, IPFSCs and SDSCs, but lower than that of ACs | [45] | |
Young patients with ACL injury for reconstruction (n = 4) [17.2 (SD 0.7) years old] | Cell counting | IPFSCs had lower proliferative potential than SDSCs but higher than ScASCs | [6] | |
Young patients with acute meniscus or ACL tear (four male and two female) (mean age 22 years) | Proliferation index | dECM deposited by IPFSCs with or without AA promoted IPFSC proliferation | [48] | |
Arthritis donor | OA patients (n = 26) (mean age 75 years) | PDT | IPFSCs showed similar growth kinetics compared with BMSCs | [51] |
OA patients for TKA or ACI surgery (three male and three female) (35–79 years old) | PDT | IPFSCs had a significantly faster proliferation rate than SF-MSCs | [52] | |
OA patients for TKA (n = 6) | Cell counting | FGF-2 promoted IPFSC proliferation | [43] | |
OA patients for TKA (n = 5) [57 (SD 3) vs 86 (SD 4) years old] | Cell number | No significant difference was observed in proliferation rate of IPFSCs between two groups with different age ranges | [53] | |
OA patients for TKA (n = 6) | PD | No effect of progressive passaging was observed on IPFSC proliferation until passage 18 | [54] | |
OA patients for joint arthroplasty (one male and four female) (50–79 years old) | DNA content | Serum stimulation and low-oxygen environment promoted IPFSC proliferation | [44] | |
OA patients for TKA (n = 25) [70 (SD 8) years old] | DNA content | IPFSCs showed similar proliferation compared with ScASCs in a pellet culture with chondrogenic induction | [13] | |
OA elderly patients for TKA (n = 4) [70.5 (SD 9.2) years old] | Cell counting | IPFSCs had similar proliferative potential compared with SDSCs but higher than ScASCs | [6] | |
OA patients for joint arthroplasty (n = 7) | PD and PDT | Supplementation with FGF-2 and TGF-β3 significantly promoted IPFSC proliferation | [46] | |
Unknown donor | Female patient for TKA (n = 1) (52 years old) | DNA content | Greater IPFSC proliferation on porcine cartilage ECM-derived scaffolds over 28 days | [55] |
Female patients for TKA (65 and 68 years old) | Cell counting | IPFSCs had comparable proliferation to ScASCs on day 7 | [12] |
. | Tissue donor . | Assay . | Results . | Reference . |
---|---|---|---|---|
Non-arthritis donor | Calf knee joints (3 months old) | PD and CFU-F assay | IPFSCs had lower proliferation than SDSCs | [49] |
Porcine knee joints (n = 3) (4 months old) | Cell yield and PDT | FGF-2 promoted IPFSC proliferation | [42] | |
Male C57BL/6J mice (fed a high-fat or a low-fat diet) | Cumulative fold increase | Obese IPFSCs had comparable proliferation in vitro to lean IPFSCs despite a trend toward increased proliferation in obese compared with lean donors | [50] | |
Minipigs (n = 2) (5 months old) | Cell counting | dECM expansion enhanced IPFSC proliferation and maintained stem cell morphology | [47] | |
Patients for partial meniscectomy or ACL reconstruction (n = 15) (26–68 years old) | PD | TFP supplementation significantly increased the proliferation rate of meniscus cells, IPFSCs and SDSCs, but lower than that of ACs | [45] | |
Young patients with ACL injury for reconstruction (n = 4) [17.2 (SD 0.7) years old] | Cell counting | IPFSCs had lower proliferative potential than SDSCs but higher than ScASCs | [6] | |
Young patients with acute meniscus or ACL tear (four male and two female) (mean age 22 years) | Proliferation index | dECM deposited by IPFSCs with or without AA promoted IPFSC proliferation | [48] | |
Arthritis donor | OA patients (n = 26) (mean age 75 years) | PDT | IPFSCs showed similar growth kinetics compared with BMSCs | [51] |
OA patients for TKA or ACI surgery (three male and three female) (35–79 years old) | PDT | IPFSCs had a significantly faster proliferation rate than SF-MSCs | [52] | |
OA patients for TKA (n = 6) | Cell counting | FGF-2 promoted IPFSC proliferation | [43] | |
OA patients for TKA (n = 5) [57 (SD 3) vs 86 (SD 4) years old] | Cell number | No significant difference was observed in proliferation rate of IPFSCs between two groups with different age ranges | [53] | |
OA patients for TKA (n = 6) | PD | No effect of progressive passaging was observed on IPFSC proliferation until passage 18 | [54] | |
OA patients for joint arthroplasty (one male and four female) (50–79 years old) | DNA content | Serum stimulation and low-oxygen environment promoted IPFSC proliferation | [44] | |
OA patients for TKA (n = 25) [70 (SD 8) years old] | DNA content | IPFSCs showed similar proliferation compared with ScASCs in a pellet culture with chondrogenic induction | [13] | |
OA elderly patients for TKA (n = 4) [70.5 (SD 9.2) years old] | Cell counting | IPFSCs had similar proliferative potential compared with SDSCs but higher than ScASCs | [6] | |
OA patients for joint arthroplasty (n = 7) | PD and PDT | Supplementation with FGF-2 and TGF-β3 significantly promoted IPFSC proliferation | [46] | |
Unknown donor | Female patient for TKA (n = 1) (52 years old) | DNA content | Greater IPFSC proliferation on porcine cartilage ECM-derived scaffolds over 28 days | [55] |
Female patients for TKA (65 and 68 years old) | Cell counting | IPFSCs had comparable proliferation to ScASCs on day 7 | [12] |
AA: ascorbic acid/L-ascorbic acid phosphate; AC: articular chondrocyte; ACI: autologous chondrocyte implantation; ACL: anterior cruciate ligament; CFU-F: fibroblastic colony-forming unit; FGF-2: fibroblast growth factor 2; PDT: population doubling time; TFP: transforming growth factor beta, FGF-2, and platelet-derived growth factor bb; TKA: total knee arthroplasty.
. | Tissue donor . | Assay . | Results . | Reference . |
---|---|---|---|---|
Non-arthritis donor | Calf knee joints (3 months old) | PD and CFU-F assay | IPFSCs had lower proliferation than SDSCs | [49] |
Porcine knee joints (n = 3) (4 months old) | Cell yield and PDT | FGF-2 promoted IPFSC proliferation | [42] | |
Male C57BL/6J mice (fed a high-fat or a low-fat diet) | Cumulative fold increase | Obese IPFSCs had comparable proliferation in vitro to lean IPFSCs despite a trend toward increased proliferation in obese compared with lean donors | [50] | |
Minipigs (n = 2) (5 months old) | Cell counting | dECM expansion enhanced IPFSC proliferation and maintained stem cell morphology | [47] | |
Patients for partial meniscectomy or ACL reconstruction (n = 15) (26–68 years old) | PD | TFP supplementation significantly increased the proliferation rate of meniscus cells, IPFSCs and SDSCs, but lower than that of ACs | [45] | |
Young patients with ACL injury for reconstruction (n = 4) [17.2 (SD 0.7) years old] | Cell counting | IPFSCs had lower proliferative potential than SDSCs but higher than ScASCs | [6] | |
Young patients with acute meniscus or ACL tear (four male and two female) (mean age 22 years) | Proliferation index | dECM deposited by IPFSCs with or without AA promoted IPFSC proliferation | [48] | |
Arthritis donor | OA patients (n = 26) (mean age 75 years) | PDT | IPFSCs showed similar growth kinetics compared with BMSCs | [51] |
OA patients for TKA or ACI surgery (three male and three female) (35–79 years old) | PDT | IPFSCs had a significantly faster proliferation rate than SF-MSCs | [52] | |
OA patients for TKA (n = 6) | Cell counting | FGF-2 promoted IPFSC proliferation | [43] | |
OA patients for TKA (n = 5) [57 (SD 3) vs 86 (SD 4) years old] | Cell number | No significant difference was observed in proliferation rate of IPFSCs between two groups with different age ranges | [53] | |
OA patients for TKA (n = 6) | PD | No effect of progressive passaging was observed on IPFSC proliferation until passage 18 | [54] | |
OA patients for joint arthroplasty (one male and four female) (50–79 years old) | DNA content | Serum stimulation and low-oxygen environment promoted IPFSC proliferation | [44] | |
OA patients for TKA (n = 25) [70 (SD 8) years old] | DNA content | IPFSCs showed similar proliferation compared with ScASCs in a pellet culture with chondrogenic induction | [13] | |
OA elderly patients for TKA (n = 4) [70.5 (SD 9.2) years old] | Cell counting | IPFSCs had similar proliferative potential compared with SDSCs but higher than ScASCs | [6] | |
OA patients for joint arthroplasty (n = 7) | PD and PDT | Supplementation with FGF-2 and TGF-β3 significantly promoted IPFSC proliferation | [46] | |
Unknown donor | Female patient for TKA (n = 1) (52 years old) | DNA content | Greater IPFSC proliferation on porcine cartilage ECM-derived scaffolds over 28 days | [55] |
Female patients for TKA (65 and 68 years old) | Cell counting | IPFSCs had comparable proliferation to ScASCs on day 7 | [12] |
. | Tissue donor . | Assay . | Results . | Reference . |
---|---|---|---|---|
Non-arthritis donor | Calf knee joints (3 months old) | PD and CFU-F assay | IPFSCs had lower proliferation than SDSCs | [49] |
Porcine knee joints (n = 3) (4 months old) | Cell yield and PDT | FGF-2 promoted IPFSC proliferation | [42] | |
Male C57BL/6J mice (fed a high-fat or a low-fat diet) | Cumulative fold increase | Obese IPFSCs had comparable proliferation in vitro to lean IPFSCs despite a trend toward increased proliferation in obese compared with lean donors | [50] | |
Minipigs (n = 2) (5 months old) | Cell counting | dECM expansion enhanced IPFSC proliferation and maintained stem cell morphology | [47] | |
Patients for partial meniscectomy or ACL reconstruction (n = 15) (26–68 years old) | PD | TFP supplementation significantly increased the proliferation rate of meniscus cells, IPFSCs and SDSCs, but lower than that of ACs | [45] | |
Young patients with ACL injury for reconstruction (n = 4) [17.2 (SD 0.7) years old] | Cell counting | IPFSCs had lower proliferative potential than SDSCs but higher than ScASCs | [6] | |
Young patients with acute meniscus or ACL tear (four male and two female) (mean age 22 years) | Proliferation index | dECM deposited by IPFSCs with or without AA promoted IPFSC proliferation | [48] | |
Arthritis donor | OA patients (n = 26) (mean age 75 years) | PDT | IPFSCs showed similar growth kinetics compared with BMSCs | [51] |
OA patients for TKA or ACI surgery (three male and three female) (35–79 years old) | PDT | IPFSCs had a significantly faster proliferation rate than SF-MSCs | [52] | |
OA patients for TKA (n = 6) | Cell counting | FGF-2 promoted IPFSC proliferation | [43] | |
OA patients for TKA (n = 5) [57 (SD 3) vs 86 (SD 4) years old] | Cell number | No significant difference was observed in proliferation rate of IPFSCs between two groups with different age ranges | [53] | |
OA patients for TKA (n = 6) | PD | No effect of progressive passaging was observed on IPFSC proliferation until passage 18 | [54] | |
OA patients for joint arthroplasty (one male and four female) (50–79 years old) | DNA content | Serum stimulation and low-oxygen environment promoted IPFSC proliferation | [44] | |
OA patients for TKA (n = 25) [70 (SD 8) years old] | DNA content | IPFSCs showed similar proliferation compared with ScASCs in a pellet culture with chondrogenic induction | [13] | |
OA elderly patients for TKA (n = 4) [70.5 (SD 9.2) years old] | Cell counting | IPFSCs had similar proliferative potential compared with SDSCs but higher than ScASCs | [6] | |
OA patients for joint arthroplasty (n = 7) | PD and PDT | Supplementation with FGF-2 and TGF-β3 significantly promoted IPFSC proliferation | [46] | |
Unknown donor | Female patient for TKA (n = 1) (52 years old) | DNA content | Greater IPFSC proliferation on porcine cartilage ECM-derived scaffolds over 28 days | [55] |
Female patients for TKA (65 and 68 years old) | Cell counting | IPFSCs had comparable proliferation to ScASCs on day 7 | [12] |
AA: ascorbic acid/L-ascorbic acid phosphate; AC: articular chondrocyte; ACI: autologous chondrocyte implantation; ACL: anterior cruciate ligament; CFU-F: fibroblastic colony-forming unit; FGF-2: fibroblast growth factor 2; PDT: population doubling time; TFP: transforming growth factor beta, FGF-2, and platelet-derived growth factor bb; TKA: total knee arthroplasty.
As shown previously, IPFSCs have comparable advantages in proliferation capacity over other stem cells. By evaluating population doubling time and colony-forming capacity, English et al. [51] found that IPFSCs have similar initial growth kinetics compared with bone marrow stromal cells (BMSCs). Furthermore, Garcia et al. [52] reported that human IPFSCs had a significantly faster proliferation rate than donor-matched SF MSCs. These studies indicate that IPFSCs have superior proliferation capacity.
Among many stem cells, SDSCs had a high proliferation and self-renewal capacity [56, 57]. Lee et al. [49] has shown that bovine calf SDSCs and IPFSCs could be cultured for at least eight passages without morphology change and proliferation reduction; compared with IPFSCs, donor-matched SDSCs exhibited higher cumulative population doublings and colony-forming potential. However, other studies have shown contrary results that IPFSCs might have comparable capacity in proliferation [45]. Mochizuki et al. [6] found that elderly IPFSCs had similar proliferative potential to donor-matched human SDSCs, despite a lower level compared with SDSCs in young donors. Moreover, the increase in colony size and colony-forming efficiency between IPFSCs and SDSCs were comparable in a single-cell-derived culture.
Interestingly, ScASCs with inferior proliferation potential to SDSCs [57] exerted lower or comparable capacity in proliferation compared with IPFSCs [6, 12, 13]. Similarly, Mochizuki et al. [6] found a higher proliferative potential of IPFSCs and SDSCs than that of ScASCs in both young and elderly donors. The increase in colony size and colony-forming efficiency also confirmed the superior proliferation of SDSCs and IPFSCs to ScASCs in a single-cell-derived culture [6]. A report from Ding et al. [12] demonstrated similar proliferation in terms of cell counting between IPFSCs and ScASCs on day 7 cultures.
Multidifferentiation
Previous studies have demonstrated the capacities of IPFSCs, SDSCs and ScASCs to undergo chondrogenic, osteogenic and adipogenic differentiation in vitro and in vivo [6, 8]. Increasing evidence indicates the role of local tissue microenvironment in guiding the ‘fate’ of the MSCs toward a particular lineage [11]. Despite similar expression of MSC surface markers, IPFSCs have unique capacities in multidifferentiation compared with SDSCs and ScASCs [6, 13].
Chondrogenesis
Increasing evidence has demonstrated that IPFSCs can differentiate toward a chondrogenic lineage (Table 2) [44, 47, 58, 50] after stimulation with growth factors either in the proliferation phase for pre-treatment using fibroblast growth factor 2 [42, 43] or in the differentiation induction phase for treatment using TGF-β and bone morphogenetic protein (BMP) [6, 59]. This chondrogenic induction of IPFSCs was also observed when cultured in various scaffolds such as the three-dimensional poly (L-lactic-co-epsilon-caprolactone) scaffold [41], agarose hydrogel [60, 61], electrospun poly(l-lactide) fibre [62] or porcine cartilage ECM-derived scaffold [55]. Furthermore, numerous studies have shown that other growth conditions such as hypoxia (5% oxygen) and hydrostatic pressure could dynamically increase chondrogenesis and formation of cartilaginous tissues during pellet and scaffold culture [44, 46, 58, 61, 63]. Intra-articular injection of ex vivo expanded IPFSCs could significantly reduce pain and improve function in the human OA knee [64, 65]. Animal studies have shown that in vivo implantation of IPFSCs encapsulated in various scaffolds results in the formation of cartilage [40, 66]. Recent reports have demonstrated that IPFSCs can produce increased cartilaginous tissues after seeding on TGF-β3 binding cartilage ECM-derived scaffold and then implanting subcutaneously into nude mice [55, 67]. However, attention should also be paid to previous reports in which IPFSCs expanded on scaffolds failed to develop stable cartilaginous tissues after subcutaneous implantation into nude mice, suggesting inferior chondrogenic capacity of IPFSCs potentially due to fibrous dedifferentiation or resorption in vivo [45, 68].
. | Tissue donor . | Treatment . | Results . | References . |
---|---|---|---|---|
Non-arthritis donor | Calf knee joints (3 months old) | Chondrogenic induction (A2P, BSA, DEX, SP, proline, ITS, TGF-β1, BMP-7) in a pellet culture system | Confirmed in vitro chondrogenic capacity and secretion of SZP but inferior to SDSCs | [49] |
Immature porcine (4 months old) | Encapsulated in agarose followed by chondrogenic induction (SP, A2P, proline, linoleic acid, BSA, ITS, DEX, TGF-β3) for 21 days and then implantation into nude mice for 28 days or maintained for a similar period in vitro | Confirmed in vitro chondrogenic capacity but inferior to SDSCs, both of which failed to form stable cartilaginous tissues in vivo | [68] | |
Porcine (n = 2) (4 months old) | Encapsulated in agarose hydrogel and induced in chondrogenic medium (SP, proline, A2P, BSA, ITS, DEX, TGF-β3) in a hypoxic environment (5% vs 20%) | Low oxygen tension enhanced IPFSC chondrogenesis and produced constructs with superior mechanical functionality to chondrocytes | [61] | |
Porcine knee joints (n = 3) (4 months old) | Expanded with FGF-2 then capsulated in agarose hydrogel in chondrogenic induction (SP, proline, A2P, BSA, ITS, DEX, TGF-β3) | Increased in vitro chondrogenic capacity | [42] | |
Porcine (n = 2) (4 months old) | Encapsulated in agarose hydrogel followed by chondrogenic induction (SP, proline, A2P, linoleic acid, BSA, ITS, DEX, TGF-β3) | Confirmed robust in vitro chondrogenic capacity of IPFSCs and BMSCs but inferior to chondrocytes in mechanical functionality | [60] | |
Porcine (n = 2) (4 months old) | Pellet culture was subjected to 10 MPa of cyclic HP (4 h/day) and chondrogenic induction with TGF-β3 (0, 1 and 10 ng/mL) for 14 days | HP enhanced in vitro chondrogenic capacity of SDSCs and IPFSCs in the presence of 1 ng/ml TGF-β3 | [58] | |
Minipigs (n = 2) (5 months old) | dECM expansion (deposited by IPFSCs versus SDSCs) followed by chondrogenic induction (DEX, proline, A2P, ITS, TGF-β3 vs TGF-β3 and BMP-6) in a pellet culture system | dECM expansion and/or co-GF treatment promoted IPFSC chondrogenesis | [47] | |
Male C57BL/6J mice (fed a high-fat or a low-fat diet) | Chondrogenic induction (ITS, ascorbate, proline, TGF-β3, BMP-6) in a pellet culture system | Decreased in vitro chondrogenic capacity of IPFSCs from obese mice compared with lean mice | [50] | |
Male patient for ACL reconstruction (n = 1) (26 years old) | Seeded onto porcine decellularized articular cartilage scaffold followed by chondrogenic induction (SP, proline, linoleic acid, A2P, BSA, ITS, DEX, TGF-β3) | Confirmed in vitro chondrogenic capacity | [69] | |
Young patients with acute meniscus or ACL tear (four male and two female) (mean age 22 years) | Expanded on dECM deposited by IPFSCs followed by chondrogenic induction (A2P, proline, DEX, ITS, TGF-β3) in a pellet culture system | Increased in vitro chondrogenic capacity | [48] | |
Young patients with ACL injury for reconstruction (n = 4) [17.2 (SD 0.7) years old] | Chondrogenic induction (TGF-β3, DEX, A2P, proline, pyruvate, ITS, BMP-2) in a pellet culture system | Comparable chondrogenic capacity to SDSCs but higher levels compared with ScASCs | [6] | |
Arthritis donor | OA patients (n = 26) (mean age 75 years) | Chondrogenic induction (pyruvate, proline, A2P, BSA, ITS, DEX, TGF-β3) in a pellet culture system | Comparable in vitro chondrogenic capacity to cartilage-derived cells and BMSCs at early-passage cultures | [51] |
OA patients for TKA (n = 3) (69, 71 and 72 years old) | Chondrogenic induction (FBS, ITS, DEX, A2P, TGF-β3, BMP-6) in a pellet culture system for 28 days | Confirmed in vitro chondrogenic capacity | [59] | |
OA patients for TKA (two males and three females) (67–81 years old) | Chondrogenic induction (FBS, ITS, AA, DEX, TGF-β1) in a pellet culture system | Higher in vitro chondrogenic capacity than ScASCs but inferior to chondrocytes | [70] | |
OA patients for TKA (n = 7) (three male and four female) [71.4 (SD 8.3) years old] | Monolayer culture in chondrogenic induction (StemPro chondrogenesis supplement) and PBMCs stimulation | Confirmed in vitro chondrogenic capacity and increased by PBMCs | [71] | |
OA patients for TKA (n = 3) (67, 69 and 72 years old) | Chondrogenic induction (ITS, A2P, DEX, TGF-β3, IGF-1) in a pellet culture system under hypoxia (5% O2vs 20% O2) | Hypoxic conditions enhanced chondrogenic capacity by upregulated HIF2α expression | [63] | |
OA patients for TKA (n = 6) | Expanded with FGF-2 followed by chondrogenic induction (ITS, A2P, DEX, TGF-β3, IGF-1) in pellet culture for 14 days | Increased in vitro chondrogenic capacity | [43] | |
OA patients (n = 25) (8 male and 17 female) (mean age 54.1 years) | Prepared with PRP and percutaneously injected into arthritic knees | Intra-articular injection reduced pain and improved function | [64] | |
OA patients (n = 18) (6 male and 12 female) (mean age 54.6 years) | Prepared with PRP and injected into arthritic knees | Intra-articular injection reduced pain and improved function | [65] | |
OA patients for joint arthroplasty (one male and four female) (50–79 years old) | Suspended in agarose hydrogels followed by chondrogenic induction (SP, proline, A2P, linoleic acid, BSA, ITS, DEX, TGF-β3) with serum, low oxygen and HP | Increased chondrogenesis with serum stimulation and low oxygen; HP improved mechanical functionality within hydrogels | [44] | |
OA patients for joint arthroplasty (n = 5) (one male and four female) (50–79 years old) | Suspended in agarose hydrogel and maintained in chondrogenic medium (SP, proline, A2P, linoleic acid, BSA, ITS, DEX, TGF-β3) with or without BMP-6 followed by HP | Increased in vitro chondrogenic capacity with higher cell densities and BMP-6; additional cyclic HP improved dynamic modulus | [72] | |
OA patients for joint arthroplasty or patients without OA for ligament reconstruction | Pellet culture or seeded onto polyester transwell inserts or electrospun PLLA fibre followed by chondrogenic induction (SP, proline, A2P, linoleic acid, DEX, BSA, ITS, TGF-β3, BMP-6) | Comparable chondrogenic capacity of IPFSCs from OA to healthy donors; cyclic HP increased OA IPFSC chondrogenesis | [62] | |
OA patients for TKA (n = 25) [70 (SD 8) years old] | Chondrogenic induction in a pellet culture system (L-glutamine, SP, serum albumin, ITS, DEX, A2P, TGF-β1) | Superior in vitro chondrogenic capacity compared with knee ScASCs | [13] | |
OA patients for joint replacement (n = 18) | Exposed to OA chondrocyte extracts then grown on PLGA scaffolds | Promoted chondrogenic differentiation in monolayer culture and PLGA scaffolds | [73] | |
OA elderly patients for TKA (n = 4) [70.5 (SD 9.2) years old] | Chondrogenic induction (TGF-β3, DEX, A2P, proline, pyruvate, ITS, BMP-2) in a pellet culture system | Comparable chondrogenic capacity to SDSCs but higher levels compared with ScASCs | [6] | |
OA patients for joint arthroplasty (n = 7) | Expanded with FGF-2 followed by chondrogenic induction (SP, proline, BSA, linoleic acid, ITS, A2P, DEX, TGF-β3) in a pellet culture system or encapsulated in agarose or fibrin hydrogels with altered oxygen tension | Increased chondrogenic capacity in the presence of FGF-2 was further enhanced by a low-oxygen environment | [46] | |
RA patients (n = 24) and OA patients (n = 18) for TKA | Chondrogenic induction (SP, ITS, A2P, DEX, TGF-β1) in a pellet culture system with or without TNF | Similar in vitro chondrogenic capacity between RA and OA; TNF decreased chondrogenesis in both groups | [74] | |
Unknown donor | Female patient for TKA (n = 1) (52 years old) | Coupling freshly isolated CD44+ IPFSCs with a TGF-β3 eluting pig cartilage ECM-derived scaffold followed by implantation | Enhanced chondrogenesis after implantation into nude mice | [55] |
Female patients for TKA (n = 2) (65 and 68 years old) | Chondrogenic induction (FBS, TGF-β1, A2P, insulin) or cultured in HA microenvironment in monolayer culture | Higher chondrogenic differentiation than BMSCs, ScASCs and WJSCs; enhanced chondrogenesis by 25% HA | [12] | |
Patients for TKA (n = 3) and one patient for ACL surgery | Freshly isolated IPFSCs seeded on fibrin/porcine cartilage ECM hydrogels with TGF-β3 followed by implantation into nude mice | Increased chondrogenic differentiation in vitro and in vivo | [67] | |
Patients for TKA (n = 5) (mean age 74 years) | Chondrogenic induction (FBS, IGF-I, FGF-2, GH, A2P, transferrin) with fibrin glue followed by 6 week implantation in SCID mice | Confirmed in vitro chondrogenic capacity and histological appearance of cartilage regeneration in vivo | [40] | |
Patients (three male and three female) for TKA or ACI surgery (35–79 years old) | Chondrogenic induction (FBS, ITS, AA, DEX, TGF-β1) in a pellet culture system | Similar in vitro chondrogenic capacity compared with SF MSCs | [52] | |
Patients (13 for TKA and 10 for ACL reconstruction) [52.4 (SD 4.4) years old] | Chondrogenic induction (TGF-β3, SP, A2P, DEX, ITS) in a pellet culture system | Confirmed in vitro chondrogenic capacity that was superior to BMSCs but inferior to PSCs | [75] | |
Patients for TKA (n = 53) (mean age 72 years) | Seeded on PLA-CPL scaffold and induced in chondrogenic medium (ITS, TGF-β1, FCS, A2P, L-glutamine) | Confirmed in vitro chondrogenic capacity | [41] | |
Patients for partial meniscectomy or ACL reconstruction (n = 15) (26–68 years old) | Expanded with TFP followed by chondrogenic induction in a pellet culture system | Enhanced in vitro chondrogenic capacity | [45] | |
Patients for joint replacement | Chondrogenic induction (FBS, ITS, AA, DEX, TGF-β) or suspended in hyaluronic acid followed by implantation to repair rat osteochondral defect with matrilin-3 | Enhanced in vitro and in vivo chondrogenic capacity | [66] | |
Patients for TKA (n = 16) [68 (SD 11) years old] | Chondrogenic induction (ITS, A2P, DEX, TGF-β1) in an alginate bead culture | Confirmed in vitro chondrogenic capacity | [8] |
. | Tissue donor . | Treatment . | Results . | References . |
---|---|---|---|---|
Non-arthritis donor | Calf knee joints (3 months old) | Chondrogenic induction (A2P, BSA, DEX, SP, proline, ITS, TGF-β1, BMP-7) in a pellet culture system | Confirmed in vitro chondrogenic capacity and secretion of SZP but inferior to SDSCs | [49] |
Immature porcine (4 months old) | Encapsulated in agarose followed by chondrogenic induction (SP, A2P, proline, linoleic acid, BSA, ITS, DEX, TGF-β3) for 21 days and then implantation into nude mice for 28 days or maintained for a similar period in vitro | Confirmed in vitro chondrogenic capacity but inferior to SDSCs, both of which failed to form stable cartilaginous tissues in vivo | [68] | |
Porcine (n = 2) (4 months old) | Encapsulated in agarose hydrogel and induced in chondrogenic medium (SP, proline, A2P, BSA, ITS, DEX, TGF-β3) in a hypoxic environment (5% vs 20%) | Low oxygen tension enhanced IPFSC chondrogenesis and produced constructs with superior mechanical functionality to chondrocytes | [61] | |
Porcine knee joints (n = 3) (4 months old) | Expanded with FGF-2 then capsulated in agarose hydrogel in chondrogenic induction (SP, proline, A2P, BSA, ITS, DEX, TGF-β3) | Increased in vitro chondrogenic capacity | [42] | |
Porcine (n = 2) (4 months old) | Encapsulated in agarose hydrogel followed by chondrogenic induction (SP, proline, A2P, linoleic acid, BSA, ITS, DEX, TGF-β3) | Confirmed robust in vitro chondrogenic capacity of IPFSCs and BMSCs but inferior to chondrocytes in mechanical functionality | [60] | |
Porcine (n = 2) (4 months old) | Pellet culture was subjected to 10 MPa of cyclic HP (4 h/day) and chondrogenic induction with TGF-β3 (0, 1 and 10 ng/mL) for 14 days | HP enhanced in vitro chondrogenic capacity of SDSCs and IPFSCs in the presence of 1 ng/ml TGF-β3 | [58] | |
Minipigs (n = 2) (5 months old) | dECM expansion (deposited by IPFSCs versus SDSCs) followed by chondrogenic induction (DEX, proline, A2P, ITS, TGF-β3 vs TGF-β3 and BMP-6) in a pellet culture system | dECM expansion and/or co-GF treatment promoted IPFSC chondrogenesis | [47] | |
Male C57BL/6J mice (fed a high-fat or a low-fat diet) | Chondrogenic induction (ITS, ascorbate, proline, TGF-β3, BMP-6) in a pellet culture system | Decreased in vitro chondrogenic capacity of IPFSCs from obese mice compared with lean mice | [50] | |
Male patient for ACL reconstruction (n = 1) (26 years old) | Seeded onto porcine decellularized articular cartilage scaffold followed by chondrogenic induction (SP, proline, linoleic acid, A2P, BSA, ITS, DEX, TGF-β3) | Confirmed in vitro chondrogenic capacity | [69] | |
Young patients with acute meniscus or ACL tear (four male and two female) (mean age 22 years) | Expanded on dECM deposited by IPFSCs followed by chondrogenic induction (A2P, proline, DEX, ITS, TGF-β3) in a pellet culture system | Increased in vitro chondrogenic capacity | [48] | |
Young patients with ACL injury for reconstruction (n = 4) [17.2 (SD 0.7) years old] | Chondrogenic induction (TGF-β3, DEX, A2P, proline, pyruvate, ITS, BMP-2) in a pellet culture system | Comparable chondrogenic capacity to SDSCs but higher levels compared with ScASCs | [6] | |
Arthritis donor | OA patients (n = 26) (mean age 75 years) | Chondrogenic induction (pyruvate, proline, A2P, BSA, ITS, DEX, TGF-β3) in a pellet culture system | Comparable in vitro chondrogenic capacity to cartilage-derived cells and BMSCs at early-passage cultures | [51] |
OA patients for TKA (n = 3) (69, 71 and 72 years old) | Chondrogenic induction (FBS, ITS, DEX, A2P, TGF-β3, BMP-6) in a pellet culture system for 28 days | Confirmed in vitro chondrogenic capacity | [59] | |
OA patients for TKA (two males and three females) (67–81 years old) | Chondrogenic induction (FBS, ITS, AA, DEX, TGF-β1) in a pellet culture system | Higher in vitro chondrogenic capacity than ScASCs but inferior to chondrocytes | [70] | |
OA patients for TKA (n = 7) (three male and four female) [71.4 (SD 8.3) years old] | Monolayer culture in chondrogenic induction (StemPro chondrogenesis supplement) and PBMCs stimulation | Confirmed in vitro chondrogenic capacity and increased by PBMCs | [71] | |
OA patients for TKA (n = 3) (67, 69 and 72 years old) | Chondrogenic induction (ITS, A2P, DEX, TGF-β3, IGF-1) in a pellet culture system under hypoxia (5% O2vs 20% O2) | Hypoxic conditions enhanced chondrogenic capacity by upregulated HIF2α expression | [63] | |
OA patients for TKA (n = 6) | Expanded with FGF-2 followed by chondrogenic induction (ITS, A2P, DEX, TGF-β3, IGF-1) in pellet culture for 14 days | Increased in vitro chondrogenic capacity | [43] | |
OA patients (n = 25) (8 male and 17 female) (mean age 54.1 years) | Prepared with PRP and percutaneously injected into arthritic knees | Intra-articular injection reduced pain and improved function | [64] | |
OA patients (n = 18) (6 male and 12 female) (mean age 54.6 years) | Prepared with PRP and injected into arthritic knees | Intra-articular injection reduced pain and improved function | [65] | |
OA patients for joint arthroplasty (one male and four female) (50–79 years old) | Suspended in agarose hydrogels followed by chondrogenic induction (SP, proline, A2P, linoleic acid, BSA, ITS, DEX, TGF-β3) with serum, low oxygen and HP | Increased chondrogenesis with serum stimulation and low oxygen; HP improved mechanical functionality within hydrogels | [44] | |
OA patients for joint arthroplasty (n = 5) (one male and four female) (50–79 years old) | Suspended in agarose hydrogel and maintained in chondrogenic medium (SP, proline, A2P, linoleic acid, BSA, ITS, DEX, TGF-β3) with or without BMP-6 followed by HP | Increased in vitro chondrogenic capacity with higher cell densities and BMP-6; additional cyclic HP improved dynamic modulus | [72] | |
OA patients for joint arthroplasty or patients without OA for ligament reconstruction | Pellet culture or seeded onto polyester transwell inserts or electrospun PLLA fibre followed by chondrogenic induction (SP, proline, A2P, linoleic acid, DEX, BSA, ITS, TGF-β3, BMP-6) | Comparable chondrogenic capacity of IPFSCs from OA to healthy donors; cyclic HP increased OA IPFSC chondrogenesis | [62] | |
OA patients for TKA (n = 25) [70 (SD 8) years old] | Chondrogenic induction in a pellet culture system (L-glutamine, SP, serum albumin, ITS, DEX, A2P, TGF-β1) | Superior in vitro chondrogenic capacity compared with knee ScASCs | [13] | |
OA patients for joint replacement (n = 18) | Exposed to OA chondrocyte extracts then grown on PLGA scaffolds | Promoted chondrogenic differentiation in monolayer culture and PLGA scaffolds | [73] | |
OA elderly patients for TKA (n = 4) [70.5 (SD 9.2) years old] | Chondrogenic induction (TGF-β3, DEX, A2P, proline, pyruvate, ITS, BMP-2) in a pellet culture system | Comparable chondrogenic capacity to SDSCs but higher levels compared with ScASCs | [6] | |
OA patients for joint arthroplasty (n = 7) | Expanded with FGF-2 followed by chondrogenic induction (SP, proline, BSA, linoleic acid, ITS, A2P, DEX, TGF-β3) in a pellet culture system or encapsulated in agarose or fibrin hydrogels with altered oxygen tension | Increased chondrogenic capacity in the presence of FGF-2 was further enhanced by a low-oxygen environment | [46] | |
RA patients (n = 24) and OA patients (n = 18) for TKA | Chondrogenic induction (SP, ITS, A2P, DEX, TGF-β1) in a pellet culture system with or without TNF | Similar in vitro chondrogenic capacity between RA and OA; TNF decreased chondrogenesis in both groups | [74] | |
Unknown donor | Female patient for TKA (n = 1) (52 years old) | Coupling freshly isolated CD44+ IPFSCs with a TGF-β3 eluting pig cartilage ECM-derived scaffold followed by implantation | Enhanced chondrogenesis after implantation into nude mice | [55] |
Female patients for TKA (n = 2) (65 and 68 years old) | Chondrogenic induction (FBS, TGF-β1, A2P, insulin) or cultured in HA microenvironment in monolayer culture | Higher chondrogenic differentiation than BMSCs, ScASCs and WJSCs; enhanced chondrogenesis by 25% HA | [12] | |
Patients for TKA (n = 3) and one patient for ACL surgery | Freshly isolated IPFSCs seeded on fibrin/porcine cartilage ECM hydrogels with TGF-β3 followed by implantation into nude mice | Increased chondrogenic differentiation in vitro and in vivo | [67] | |
Patients for TKA (n = 5) (mean age 74 years) | Chondrogenic induction (FBS, IGF-I, FGF-2, GH, A2P, transferrin) with fibrin glue followed by 6 week implantation in SCID mice | Confirmed in vitro chondrogenic capacity and histological appearance of cartilage regeneration in vivo | [40] | |
Patients (three male and three female) for TKA or ACI surgery (35–79 years old) | Chondrogenic induction (FBS, ITS, AA, DEX, TGF-β1) in a pellet culture system | Similar in vitro chondrogenic capacity compared with SF MSCs | [52] | |
Patients (13 for TKA and 10 for ACL reconstruction) [52.4 (SD 4.4) years old] | Chondrogenic induction (TGF-β3, SP, A2P, DEX, ITS) in a pellet culture system | Confirmed in vitro chondrogenic capacity that was superior to BMSCs but inferior to PSCs | [75] | |
Patients for TKA (n = 53) (mean age 72 years) | Seeded on PLA-CPL scaffold and induced in chondrogenic medium (ITS, TGF-β1, FCS, A2P, L-glutamine) | Confirmed in vitro chondrogenic capacity | [41] | |
Patients for partial meniscectomy or ACL reconstruction (n = 15) (26–68 years old) | Expanded with TFP followed by chondrogenic induction in a pellet culture system | Enhanced in vitro chondrogenic capacity | [45] | |
Patients for joint replacement | Chondrogenic induction (FBS, ITS, AA, DEX, TGF-β) or suspended in hyaluronic acid followed by implantation to repair rat osteochondral defect with matrilin-3 | Enhanced in vitro and in vivo chondrogenic capacity | [66] | |
Patients for TKA (n = 16) [68 (SD 11) years old] | Chondrogenic induction (ITS, A2P, DEX, TGF-β1) in an alginate bead culture | Confirmed in vitro chondrogenic capacity | [8] |
GF: growth factor; HA: hyaluronan/hyaluronan acid; HP: hydrostatic pressure; PLA-CPL: poly(l-lactic-co-ɛ-caprolactone); PLGA: poly(DL-lactic-co-glycolic acid; PRP: platelet-rich plasma; SCID: severe combined immunodeficient mice; SZP: superficial zone protein; TKA: total knee arthroplasty; WJSC: Wharton’s jelly stem cells.
. | Tissue donor . | Treatment . | Results . | References . |
---|---|---|---|---|
Non-arthritis donor | Calf knee joints (3 months old) | Chondrogenic induction (A2P, BSA, DEX, SP, proline, ITS, TGF-β1, BMP-7) in a pellet culture system | Confirmed in vitro chondrogenic capacity and secretion of SZP but inferior to SDSCs | [49] |
Immature porcine (4 months old) | Encapsulated in agarose followed by chondrogenic induction (SP, A2P, proline, linoleic acid, BSA, ITS, DEX, TGF-β3) for 21 days and then implantation into nude mice for 28 days or maintained for a similar period in vitro | Confirmed in vitro chondrogenic capacity but inferior to SDSCs, both of which failed to form stable cartilaginous tissues in vivo | [68] | |
Porcine (n = 2) (4 months old) | Encapsulated in agarose hydrogel and induced in chondrogenic medium (SP, proline, A2P, BSA, ITS, DEX, TGF-β3) in a hypoxic environment (5% vs 20%) | Low oxygen tension enhanced IPFSC chondrogenesis and produced constructs with superior mechanical functionality to chondrocytes | [61] | |
Porcine knee joints (n = 3) (4 months old) | Expanded with FGF-2 then capsulated in agarose hydrogel in chondrogenic induction (SP, proline, A2P, BSA, ITS, DEX, TGF-β3) | Increased in vitro chondrogenic capacity | [42] | |
Porcine (n = 2) (4 months old) | Encapsulated in agarose hydrogel followed by chondrogenic induction (SP, proline, A2P, linoleic acid, BSA, ITS, DEX, TGF-β3) | Confirmed robust in vitro chondrogenic capacity of IPFSCs and BMSCs but inferior to chondrocytes in mechanical functionality | [60] | |
Porcine (n = 2) (4 months old) | Pellet culture was subjected to 10 MPa of cyclic HP (4 h/day) and chondrogenic induction with TGF-β3 (0, 1 and 10 ng/mL) for 14 days | HP enhanced in vitro chondrogenic capacity of SDSCs and IPFSCs in the presence of 1 ng/ml TGF-β3 | [58] | |
Minipigs (n = 2) (5 months old) | dECM expansion (deposited by IPFSCs versus SDSCs) followed by chondrogenic induction (DEX, proline, A2P, ITS, TGF-β3 vs TGF-β3 and BMP-6) in a pellet culture system | dECM expansion and/or co-GF treatment promoted IPFSC chondrogenesis | [47] | |
Male C57BL/6J mice (fed a high-fat or a low-fat diet) | Chondrogenic induction (ITS, ascorbate, proline, TGF-β3, BMP-6) in a pellet culture system | Decreased in vitro chondrogenic capacity of IPFSCs from obese mice compared with lean mice | [50] | |
Male patient for ACL reconstruction (n = 1) (26 years old) | Seeded onto porcine decellularized articular cartilage scaffold followed by chondrogenic induction (SP, proline, linoleic acid, A2P, BSA, ITS, DEX, TGF-β3) | Confirmed in vitro chondrogenic capacity | [69] | |
Young patients with acute meniscus or ACL tear (four male and two female) (mean age 22 years) | Expanded on dECM deposited by IPFSCs followed by chondrogenic induction (A2P, proline, DEX, ITS, TGF-β3) in a pellet culture system | Increased in vitro chondrogenic capacity | [48] | |
Young patients with ACL injury for reconstruction (n = 4) [17.2 (SD 0.7) years old] | Chondrogenic induction (TGF-β3, DEX, A2P, proline, pyruvate, ITS, BMP-2) in a pellet culture system | Comparable chondrogenic capacity to SDSCs but higher levels compared with ScASCs | [6] | |
Arthritis donor | OA patients (n = 26) (mean age 75 years) | Chondrogenic induction (pyruvate, proline, A2P, BSA, ITS, DEX, TGF-β3) in a pellet culture system | Comparable in vitro chondrogenic capacity to cartilage-derived cells and BMSCs at early-passage cultures | [51] |
OA patients for TKA (n = 3) (69, 71 and 72 years old) | Chondrogenic induction (FBS, ITS, DEX, A2P, TGF-β3, BMP-6) in a pellet culture system for 28 days | Confirmed in vitro chondrogenic capacity | [59] | |
OA patients for TKA (two males and three females) (67–81 years old) | Chondrogenic induction (FBS, ITS, AA, DEX, TGF-β1) in a pellet culture system | Higher in vitro chondrogenic capacity than ScASCs but inferior to chondrocytes | [70] | |
OA patients for TKA (n = 7) (three male and four female) [71.4 (SD 8.3) years old] | Monolayer culture in chondrogenic induction (StemPro chondrogenesis supplement) and PBMCs stimulation | Confirmed in vitro chondrogenic capacity and increased by PBMCs | [71] | |
OA patients for TKA (n = 3) (67, 69 and 72 years old) | Chondrogenic induction (ITS, A2P, DEX, TGF-β3, IGF-1) in a pellet culture system under hypoxia (5% O2vs 20% O2) | Hypoxic conditions enhanced chondrogenic capacity by upregulated HIF2α expression | [63] | |
OA patients for TKA (n = 6) | Expanded with FGF-2 followed by chondrogenic induction (ITS, A2P, DEX, TGF-β3, IGF-1) in pellet culture for 14 days | Increased in vitro chondrogenic capacity | [43] | |
OA patients (n = 25) (8 male and 17 female) (mean age 54.1 years) | Prepared with PRP and percutaneously injected into arthritic knees | Intra-articular injection reduced pain and improved function | [64] | |
OA patients (n = 18) (6 male and 12 female) (mean age 54.6 years) | Prepared with PRP and injected into arthritic knees | Intra-articular injection reduced pain and improved function | [65] | |
OA patients for joint arthroplasty (one male and four female) (50–79 years old) | Suspended in agarose hydrogels followed by chondrogenic induction (SP, proline, A2P, linoleic acid, BSA, ITS, DEX, TGF-β3) with serum, low oxygen and HP | Increased chondrogenesis with serum stimulation and low oxygen; HP improved mechanical functionality within hydrogels | [44] | |
OA patients for joint arthroplasty (n = 5) (one male and four female) (50–79 years old) | Suspended in agarose hydrogel and maintained in chondrogenic medium (SP, proline, A2P, linoleic acid, BSA, ITS, DEX, TGF-β3) with or without BMP-6 followed by HP | Increased in vitro chondrogenic capacity with higher cell densities and BMP-6; additional cyclic HP improved dynamic modulus | [72] | |
OA patients for joint arthroplasty or patients without OA for ligament reconstruction | Pellet culture or seeded onto polyester transwell inserts or electrospun PLLA fibre followed by chondrogenic induction (SP, proline, A2P, linoleic acid, DEX, BSA, ITS, TGF-β3, BMP-6) | Comparable chondrogenic capacity of IPFSCs from OA to healthy donors; cyclic HP increased OA IPFSC chondrogenesis | [62] | |
OA patients for TKA (n = 25) [70 (SD 8) years old] | Chondrogenic induction in a pellet culture system (L-glutamine, SP, serum albumin, ITS, DEX, A2P, TGF-β1) | Superior in vitro chondrogenic capacity compared with knee ScASCs | [13] | |
OA patients for joint replacement (n = 18) | Exposed to OA chondrocyte extracts then grown on PLGA scaffolds | Promoted chondrogenic differentiation in monolayer culture and PLGA scaffolds | [73] | |
OA elderly patients for TKA (n = 4) [70.5 (SD 9.2) years old] | Chondrogenic induction (TGF-β3, DEX, A2P, proline, pyruvate, ITS, BMP-2) in a pellet culture system | Comparable chondrogenic capacity to SDSCs but higher levels compared with ScASCs | [6] | |
OA patients for joint arthroplasty (n = 7) | Expanded with FGF-2 followed by chondrogenic induction (SP, proline, BSA, linoleic acid, ITS, A2P, DEX, TGF-β3) in a pellet culture system or encapsulated in agarose or fibrin hydrogels with altered oxygen tension | Increased chondrogenic capacity in the presence of FGF-2 was further enhanced by a low-oxygen environment | [46] | |
RA patients (n = 24) and OA patients (n = 18) for TKA | Chondrogenic induction (SP, ITS, A2P, DEX, TGF-β1) in a pellet culture system with or without TNF | Similar in vitro chondrogenic capacity between RA and OA; TNF decreased chondrogenesis in both groups | [74] | |
Unknown donor | Female patient for TKA (n = 1) (52 years old) | Coupling freshly isolated CD44+ IPFSCs with a TGF-β3 eluting pig cartilage ECM-derived scaffold followed by implantation | Enhanced chondrogenesis after implantation into nude mice | [55] |
Female patients for TKA (n = 2) (65 and 68 years old) | Chondrogenic induction (FBS, TGF-β1, A2P, insulin) or cultured in HA microenvironment in monolayer culture | Higher chondrogenic differentiation than BMSCs, ScASCs and WJSCs; enhanced chondrogenesis by 25% HA | [12] | |
Patients for TKA (n = 3) and one patient for ACL surgery | Freshly isolated IPFSCs seeded on fibrin/porcine cartilage ECM hydrogels with TGF-β3 followed by implantation into nude mice | Increased chondrogenic differentiation in vitro and in vivo | [67] | |
Patients for TKA (n = 5) (mean age 74 years) | Chondrogenic induction (FBS, IGF-I, FGF-2, GH, A2P, transferrin) with fibrin glue followed by 6 week implantation in SCID mice | Confirmed in vitro chondrogenic capacity and histological appearance of cartilage regeneration in vivo | [40] | |
Patients (three male and three female) for TKA or ACI surgery (35–79 years old) | Chondrogenic induction (FBS, ITS, AA, DEX, TGF-β1) in a pellet culture system | Similar in vitro chondrogenic capacity compared with SF MSCs | [52] | |
Patients (13 for TKA and 10 for ACL reconstruction) [52.4 (SD 4.4) years old] | Chondrogenic induction (TGF-β3, SP, A2P, DEX, ITS) in a pellet culture system | Confirmed in vitro chondrogenic capacity that was superior to BMSCs but inferior to PSCs | [75] | |
Patients for TKA (n = 53) (mean age 72 years) | Seeded on PLA-CPL scaffold and induced in chondrogenic medium (ITS, TGF-β1, FCS, A2P, L-glutamine) | Confirmed in vitro chondrogenic capacity | [41] | |
Patients for partial meniscectomy or ACL reconstruction (n = 15) (26–68 years old) | Expanded with TFP followed by chondrogenic induction in a pellet culture system | Enhanced in vitro chondrogenic capacity | [45] | |
Patients for joint replacement | Chondrogenic induction (FBS, ITS, AA, DEX, TGF-β) or suspended in hyaluronic acid followed by implantation to repair rat osteochondral defect with matrilin-3 | Enhanced in vitro and in vivo chondrogenic capacity | [66] | |
Patients for TKA (n = 16) [68 (SD 11) years old] | Chondrogenic induction (ITS, A2P, DEX, TGF-β1) in an alginate bead culture | Confirmed in vitro chondrogenic capacity | [8] |
. | Tissue donor . | Treatment . | Results . | References . |
---|---|---|---|---|
Non-arthritis donor | Calf knee joints (3 months old) | Chondrogenic induction (A2P, BSA, DEX, SP, proline, ITS, TGF-β1, BMP-7) in a pellet culture system | Confirmed in vitro chondrogenic capacity and secretion of SZP but inferior to SDSCs | [49] |
Immature porcine (4 months old) | Encapsulated in agarose followed by chondrogenic induction (SP, A2P, proline, linoleic acid, BSA, ITS, DEX, TGF-β3) for 21 days and then implantation into nude mice for 28 days or maintained for a similar period in vitro | Confirmed in vitro chondrogenic capacity but inferior to SDSCs, both of which failed to form stable cartilaginous tissues in vivo | [68] | |
Porcine (n = 2) (4 months old) | Encapsulated in agarose hydrogel and induced in chondrogenic medium (SP, proline, A2P, BSA, ITS, DEX, TGF-β3) in a hypoxic environment (5% vs 20%) | Low oxygen tension enhanced IPFSC chondrogenesis and produced constructs with superior mechanical functionality to chondrocytes | [61] | |
Porcine knee joints (n = 3) (4 months old) | Expanded with FGF-2 then capsulated in agarose hydrogel in chondrogenic induction (SP, proline, A2P, BSA, ITS, DEX, TGF-β3) | Increased in vitro chondrogenic capacity | [42] | |
Porcine (n = 2) (4 months old) | Encapsulated in agarose hydrogel followed by chondrogenic induction (SP, proline, A2P, linoleic acid, BSA, ITS, DEX, TGF-β3) | Confirmed robust in vitro chondrogenic capacity of IPFSCs and BMSCs but inferior to chondrocytes in mechanical functionality | [60] | |
Porcine (n = 2) (4 months old) | Pellet culture was subjected to 10 MPa of cyclic HP (4 h/day) and chondrogenic induction with TGF-β3 (0, 1 and 10 ng/mL) for 14 days | HP enhanced in vitro chondrogenic capacity of SDSCs and IPFSCs in the presence of 1 ng/ml TGF-β3 | [58] | |
Minipigs (n = 2) (5 months old) | dECM expansion (deposited by IPFSCs versus SDSCs) followed by chondrogenic induction (DEX, proline, A2P, ITS, TGF-β3 vs TGF-β3 and BMP-6) in a pellet culture system | dECM expansion and/or co-GF treatment promoted IPFSC chondrogenesis | [47] | |
Male C57BL/6J mice (fed a high-fat or a low-fat diet) | Chondrogenic induction (ITS, ascorbate, proline, TGF-β3, BMP-6) in a pellet culture system | Decreased in vitro chondrogenic capacity of IPFSCs from obese mice compared with lean mice | [50] | |
Male patient for ACL reconstruction (n = 1) (26 years old) | Seeded onto porcine decellularized articular cartilage scaffold followed by chondrogenic induction (SP, proline, linoleic acid, A2P, BSA, ITS, DEX, TGF-β3) | Confirmed in vitro chondrogenic capacity | [69] | |
Young patients with acute meniscus or ACL tear (four male and two female) (mean age 22 years) | Expanded on dECM deposited by IPFSCs followed by chondrogenic induction (A2P, proline, DEX, ITS, TGF-β3) in a pellet culture system | Increased in vitro chondrogenic capacity | [48] | |
Young patients with ACL injury for reconstruction (n = 4) [17.2 (SD 0.7) years old] | Chondrogenic induction (TGF-β3, DEX, A2P, proline, pyruvate, ITS, BMP-2) in a pellet culture system | Comparable chondrogenic capacity to SDSCs but higher levels compared with ScASCs | [6] | |
Arthritis donor | OA patients (n = 26) (mean age 75 years) | Chondrogenic induction (pyruvate, proline, A2P, BSA, ITS, DEX, TGF-β3) in a pellet culture system | Comparable in vitro chondrogenic capacity to cartilage-derived cells and BMSCs at early-passage cultures | [51] |
OA patients for TKA (n = 3) (69, 71 and 72 years old) | Chondrogenic induction (FBS, ITS, DEX, A2P, TGF-β3, BMP-6) in a pellet culture system for 28 days | Confirmed in vitro chondrogenic capacity | [59] | |
OA patients for TKA (two males and three females) (67–81 years old) | Chondrogenic induction (FBS, ITS, AA, DEX, TGF-β1) in a pellet culture system | Higher in vitro chondrogenic capacity than ScASCs but inferior to chondrocytes | [70] | |
OA patients for TKA (n = 7) (three male and four female) [71.4 (SD 8.3) years old] | Monolayer culture in chondrogenic induction (StemPro chondrogenesis supplement) and PBMCs stimulation | Confirmed in vitro chondrogenic capacity and increased by PBMCs | [71] | |
OA patients for TKA (n = 3) (67, 69 and 72 years old) | Chondrogenic induction (ITS, A2P, DEX, TGF-β3, IGF-1) in a pellet culture system under hypoxia (5% O2vs 20% O2) | Hypoxic conditions enhanced chondrogenic capacity by upregulated HIF2α expression | [63] | |
OA patients for TKA (n = 6) | Expanded with FGF-2 followed by chondrogenic induction (ITS, A2P, DEX, TGF-β3, IGF-1) in pellet culture for 14 days | Increased in vitro chondrogenic capacity | [43] | |
OA patients (n = 25) (8 male and 17 female) (mean age 54.1 years) | Prepared with PRP and percutaneously injected into arthritic knees | Intra-articular injection reduced pain and improved function | [64] | |
OA patients (n = 18) (6 male and 12 female) (mean age 54.6 years) | Prepared with PRP and injected into arthritic knees | Intra-articular injection reduced pain and improved function | [65] | |
OA patients for joint arthroplasty (one male and four female) (50–79 years old) | Suspended in agarose hydrogels followed by chondrogenic induction (SP, proline, A2P, linoleic acid, BSA, ITS, DEX, TGF-β3) with serum, low oxygen and HP | Increased chondrogenesis with serum stimulation and low oxygen; HP improved mechanical functionality within hydrogels | [44] | |
OA patients for joint arthroplasty (n = 5) (one male and four female) (50–79 years old) | Suspended in agarose hydrogel and maintained in chondrogenic medium (SP, proline, A2P, linoleic acid, BSA, ITS, DEX, TGF-β3) with or without BMP-6 followed by HP | Increased in vitro chondrogenic capacity with higher cell densities and BMP-6; additional cyclic HP improved dynamic modulus | [72] | |
OA patients for joint arthroplasty or patients without OA for ligament reconstruction | Pellet culture or seeded onto polyester transwell inserts or electrospun PLLA fibre followed by chondrogenic induction (SP, proline, A2P, linoleic acid, DEX, BSA, ITS, TGF-β3, BMP-6) | Comparable chondrogenic capacity of IPFSCs from OA to healthy donors; cyclic HP increased OA IPFSC chondrogenesis | [62] | |
OA patients for TKA (n = 25) [70 (SD 8) years old] | Chondrogenic induction in a pellet culture system (L-glutamine, SP, serum albumin, ITS, DEX, A2P, TGF-β1) | Superior in vitro chondrogenic capacity compared with knee ScASCs | [13] | |
OA patients for joint replacement (n = 18) | Exposed to OA chondrocyte extracts then grown on PLGA scaffolds | Promoted chondrogenic differentiation in monolayer culture and PLGA scaffolds | [73] | |
OA elderly patients for TKA (n = 4) [70.5 (SD 9.2) years old] | Chondrogenic induction (TGF-β3, DEX, A2P, proline, pyruvate, ITS, BMP-2) in a pellet culture system | Comparable chondrogenic capacity to SDSCs but higher levels compared with ScASCs | [6] | |
OA patients for joint arthroplasty (n = 7) | Expanded with FGF-2 followed by chondrogenic induction (SP, proline, BSA, linoleic acid, ITS, A2P, DEX, TGF-β3) in a pellet culture system or encapsulated in agarose or fibrin hydrogels with altered oxygen tension | Increased chondrogenic capacity in the presence of FGF-2 was further enhanced by a low-oxygen environment | [46] | |
RA patients (n = 24) and OA patients (n = 18) for TKA | Chondrogenic induction (SP, ITS, A2P, DEX, TGF-β1) in a pellet culture system with or without TNF | Similar in vitro chondrogenic capacity between RA and OA; TNF decreased chondrogenesis in both groups | [74] | |
Unknown donor | Female patient for TKA (n = 1) (52 years old) | Coupling freshly isolated CD44+ IPFSCs with a TGF-β3 eluting pig cartilage ECM-derived scaffold followed by implantation | Enhanced chondrogenesis after implantation into nude mice | [55] |
Female patients for TKA (n = 2) (65 and 68 years old) | Chondrogenic induction (FBS, TGF-β1, A2P, insulin) or cultured in HA microenvironment in monolayer culture | Higher chondrogenic differentiation than BMSCs, ScASCs and WJSCs; enhanced chondrogenesis by 25% HA | [12] | |
Patients for TKA (n = 3) and one patient for ACL surgery | Freshly isolated IPFSCs seeded on fibrin/porcine cartilage ECM hydrogels with TGF-β3 followed by implantation into nude mice | Increased chondrogenic differentiation in vitro and in vivo | [67] | |
Patients for TKA (n = 5) (mean age 74 years) | Chondrogenic induction (FBS, IGF-I, FGF-2, GH, A2P, transferrin) with fibrin glue followed by 6 week implantation in SCID mice | Confirmed in vitro chondrogenic capacity and histological appearance of cartilage regeneration in vivo | [40] | |
Patients (three male and three female) for TKA or ACI surgery (35–79 years old) | Chondrogenic induction (FBS, ITS, AA, DEX, TGF-β1) in a pellet culture system | Similar in vitro chondrogenic capacity compared with SF MSCs | [52] | |
Patients (13 for TKA and 10 for ACL reconstruction) [52.4 (SD 4.4) years old] | Chondrogenic induction (TGF-β3, SP, A2P, DEX, ITS) in a pellet culture system | Confirmed in vitro chondrogenic capacity that was superior to BMSCs but inferior to PSCs | [75] | |
Patients for TKA (n = 53) (mean age 72 years) | Seeded on PLA-CPL scaffold and induced in chondrogenic medium (ITS, TGF-β1, FCS, A2P, L-glutamine) | Confirmed in vitro chondrogenic capacity | [41] | |
Patients for partial meniscectomy or ACL reconstruction (n = 15) (26–68 years old) | Expanded with TFP followed by chondrogenic induction in a pellet culture system | Enhanced in vitro chondrogenic capacity | [45] | |
Patients for joint replacement | Chondrogenic induction (FBS, ITS, AA, DEX, TGF-β) or suspended in hyaluronic acid followed by implantation to repair rat osteochondral defect with matrilin-3 | Enhanced in vitro and in vivo chondrogenic capacity | [66] | |
Patients for TKA (n = 16) [68 (SD 11) years old] | Chondrogenic induction (ITS, A2P, DEX, TGF-β1) in an alginate bead culture | Confirmed in vitro chondrogenic capacity | [8] |
GF: growth factor; HA: hyaluronan/hyaluronan acid; HP: hydrostatic pressure; PLA-CPL: poly(l-lactic-co-ɛ-caprolactone); PLGA: poly(DL-lactic-co-glycolic acid; PRP: platelet-rich plasma; SCID: severe combined immunodeficient mice; SZP: superficial zone protein; TKA: total knee arthroplasty; WJSC: Wharton’s jelly stem cells.
As shown previously, IPFSCs have superior chondrogenesis [12, 75] or at least comparable chondrogenesis [51] over BMSCs despite inferior chondrogenesis relative to native chondrocytes [45, 60, 70] and IPFP perivascular stem cells [75]. IPFSCs also have comparable chondrogenic capacity to SDSCs. Mochizuki et al. [6] showed that both IPFSCs and SDSCs had higher chondrogenic potentials than those in ScASCs; however, there was no significant difference between IPFSCs and SDSCs either in young or elderly donors. This finding might be due to the unique local microenvironment in which IPFP is in close contact with synovium and SF. However, conflicting reports exist. Vinardell et al. [68] found that SDSCs produced more glycosaminoglycan and had more collagen content than IPFSCs when these cells were encapsulated in agarose hydrogels and incubated in chondrogenic medium for 49 days. Compared with ScASCs, IPFSCs have superior chondrogenic potential [6, 12, 13, 70]. These data support the notion that IPFSCs are more committed toward a chondrogenic lineage, possibly due to the unique anatomic location of the IPFP, adjacent to articular cartilage [11]. Interestingly, IPFSCs exhibited comparable (and in many cases superior) chondrogenic capacity to articular chondrocyte cultures [51].
Osteogenesis
The osteogenic capacities of IPFSCs have been demonstrated in both in vitro [8, 60, 71] and in vivo models [40, 76] (Table 3). IPFSCs from obese mice showed significantly enhanced osteogenic capacities compared with lean mice [50], but no significant difference was detected between young and elderly OA patients [6, 53]. Increasing evidence has shown that IPFSCs have comparable osteogenic capacities with BMSCs [51] and higher osteogenic potential than SF MSCs [52]. Interestingly, IPFSCs had a similar alizarin red S-positive colony-forming efficiency compared with SDSCs, indicating no difference in osteogenic potential between these two cells [6]. IPFSCs also possess a diverse osteogenic potential compared with ScASCs. In contrast to a report showing a comparable osteogenic capacity with IPFSCs [77], knee ScASCs from human OA donors responded more efficiently to osteogenic induction and produced higher levels of calcified matrix and alkaline phosphatase than donor-matched IPFSCs [13]. Dragoo et al. [40] also found that, in osteogenic induction with ascorbate and inorganic phosphate, IPFSCs only produced bone precursor proteins; however, when cultured with recombinant human BMP2 or transduced with the BMP2 gene, IPFSCs were able to differentiate toward bone formation, indicating the osteoinductive properties of BMP2 [78] rather than an inherent osteogenic potential of IPFSCs.
. | Tissue donor . | Treatment . | Results . | Reference . |
---|---|---|---|---|
Non-arthritis donor | Male C57BL/6J mice (fed a high-fat or a low-fat diet) | Osteogenic induction (BGP, AA, retinoic acid, BMP-2) | Higher osteogenic capacity of IPFSCs from obese mice compared with lean mice | [50] |
Porcine (n = 2) (4 months old) | Osteogenic induction (DEX, BGP, AA) | Confirmed in vitro osteogenic capacity | [60] | |
Young patients with ACL injury for reconstruction (n = 4) [17.2 (SD 0.7) years old] | Osteogenic induction (DEX, A2P, BGP) | Comparable osteogenic capacity with SDSCs but higher levels compared with ScASCs | [6] | |
Arthritis donor | OA patients for TKA (n = 7) (four female and three male) [71.4 (SD 8.3) years old] | Osteogenic induction (A2P, BGP, DEX) | Confirmed in vitro osteogenic capacity | [71] |
OA patients (n = 26) (mean age 75 years) | Osteogenic induction (DEX, A2P, BGP) | Similar osteogenic capacity to cartilage-derived cells and normal BMSCs | [51] | |
OA patients for TKA (n = 5) [57 (SD 3) vs 86 (SD 4) years old] | Osteogenic induction (FCS, BGP, DEX, A2P) | Similar osteogenic potential of IPFSCs between two age groups | [53] | |
OA patients for TKA (n = 25) [70 (SD 8) years old] | Osteogenic induction (BGP, DEX, A2P, cholecalciferol) | Inferior osteogenic capacity compared with knee ScASCs | [13] | |
OA patients for TKA (n = 7) | Osteogenic induction (BGP, A2P, DEX) | Comparable osteogenic capacity with periarticular ScASCs | [77] | |
OA elderly patients for TKA (n = 4) [70.5 (SD 9.2) years old] | Osteogenic induction (DEX, A2P, BGP) | Comparable osteogenic capacity with SDSCs but higher levels compared with ScASCs | [6] | |
Unknown donor | Female patients for TKA (n = 2) (65 and 68 years old) | Osteogenic induction (FBS, DEX, BGP, AA) or cultured in HA microenvironment | Confirmed in vitro osteogenic capacity and enhanced by HA microenvironment | [12] |
Patients for TKA (n = 5) (53–86 years old, mean age 74 years) | Transfected with adBMP2 followed by osteogenic induction (A2P, BGP) or seeded onto collagen type I sponges and implanted into SCID mice | Confirmed in vitro osteogenic capacity and in vivo formation of bone | [40] | |
Patients for TKA (n = 5) (mean age 73.6 years) | Transfected with adBMP2 combined with osteogenic induction followed by seeding onto collagen type I sponges and implanting into SCID mice | Confirmed in vivo bone formation with no difference compared with ScASCs | [76] | |
Patients for TKA or ACI surgery (three male and three female) (35–79 years old) | Osteogenic induction (FCS, BGP, DEX, AA) | Greater osteogenic capacity in vitro than SF MSCs | [52] | |
Patients for TKA (n = 53) (43–89 years old, mean age 72 years) | Osteogenic induction (BGP, A2P, BMP-2) | Confirmed in vitro osteogenic capacity | [41] | |
Patients for TKA (n = 16) [68 (SD 11.1) years old] | Osteogenic induction (FBS, A2P, BGP, DEX, 1, 25 vitamin D3) | Confirmed in vitro osteogenic capacity | [8] |
. | Tissue donor . | Treatment . | Results . | Reference . |
---|---|---|---|---|
Non-arthritis donor | Male C57BL/6J mice (fed a high-fat or a low-fat diet) | Osteogenic induction (BGP, AA, retinoic acid, BMP-2) | Higher osteogenic capacity of IPFSCs from obese mice compared with lean mice | [50] |
Porcine (n = 2) (4 months old) | Osteogenic induction (DEX, BGP, AA) | Confirmed in vitro osteogenic capacity | [60] | |
Young patients with ACL injury for reconstruction (n = 4) [17.2 (SD 0.7) years old] | Osteogenic induction (DEX, A2P, BGP) | Comparable osteogenic capacity with SDSCs but higher levels compared with ScASCs | [6] | |
Arthritis donor | OA patients for TKA (n = 7) (four female and three male) [71.4 (SD 8.3) years old] | Osteogenic induction (A2P, BGP, DEX) | Confirmed in vitro osteogenic capacity | [71] |
OA patients (n = 26) (mean age 75 years) | Osteogenic induction (DEX, A2P, BGP) | Similar osteogenic capacity to cartilage-derived cells and normal BMSCs | [51] | |
OA patients for TKA (n = 5) [57 (SD 3) vs 86 (SD 4) years old] | Osteogenic induction (FCS, BGP, DEX, A2P) | Similar osteogenic potential of IPFSCs between two age groups | [53] | |
OA patients for TKA (n = 25) [70 (SD 8) years old] | Osteogenic induction (BGP, DEX, A2P, cholecalciferol) | Inferior osteogenic capacity compared with knee ScASCs | [13] | |
OA patients for TKA (n = 7) | Osteogenic induction (BGP, A2P, DEX) | Comparable osteogenic capacity with periarticular ScASCs | [77] | |
OA elderly patients for TKA (n = 4) [70.5 (SD 9.2) years old] | Osteogenic induction (DEX, A2P, BGP) | Comparable osteogenic capacity with SDSCs but higher levels compared with ScASCs | [6] | |
Unknown donor | Female patients for TKA (n = 2) (65 and 68 years old) | Osteogenic induction (FBS, DEX, BGP, AA) or cultured in HA microenvironment | Confirmed in vitro osteogenic capacity and enhanced by HA microenvironment | [12] |
Patients for TKA (n = 5) (53–86 years old, mean age 74 years) | Transfected with adBMP2 followed by osteogenic induction (A2P, BGP) or seeded onto collagen type I sponges and implanted into SCID mice | Confirmed in vitro osteogenic capacity and in vivo formation of bone | [40] | |
Patients for TKA (n = 5) (mean age 73.6 years) | Transfected with adBMP2 combined with osteogenic induction followed by seeding onto collagen type I sponges and implanting into SCID mice | Confirmed in vivo bone formation with no difference compared with ScASCs | [76] | |
Patients for TKA or ACI surgery (three male and three female) (35–79 years old) | Osteogenic induction (FCS, BGP, DEX, AA) | Greater osteogenic capacity in vitro than SF MSCs | [52] | |
Patients for TKA (n = 53) (43–89 years old, mean age 72 years) | Osteogenic induction (BGP, A2P, BMP-2) | Confirmed in vitro osteogenic capacity | [41] | |
Patients for TKA (n = 16) [68 (SD 11.1) years old] | Osteogenic induction (FBS, A2P, BGP, DEX, 1, 25 vitamin D3) | Confirmed in vitro osteogenic capacity | [8] |
A2P: ascorbate acid-2-phosphate; BGP: B-glycerophosphate/beta-glycerol phosphate/glycerol phosphate; DEX: dexamethasone; FBS: fetal bovine serum; FCS: fetal calf serum.
. | Tissue donor . | Treatment . | Results . | Reference . |
---|---|---|---|---|
Non-arthritis donor | Male C57BL/6J mice (fed a high-fat or a low-fat diet) | Osteogenic induction (BGP, AA, retinoic acid, BMP-2) | Higher osteogenic capacity of IPFSCs from obese mice compared with lean mice | [50] |
Porcine (n = 2) (4 months old) | Osteogenic induction (DEX, BGP, AA) | Confirmed in vitro osteogenic capacity | [60] | |
Young patients with ACL injury for reconstruction (n = 4) [17.2 (SD 0.7) years old] | Osteogenic induction (DEX, A2P, BGP) | Comparable osteogenic capacity with SDSCs but higher levels compared with ScASCs | [6] | |
Arthritis donor | OA patients for TKA (n = 7) (four female and three male) [71.4 (SD 8.3) years old] | Osteogenic induction (A2P, BGP, DEX) | Confirmed in vitro osteogenic capacity | [71] |
OA patients (n = 26) (mean age 75 years) | Osteogenic induction (DEX, A2P, BGP) | Similar osteogenic capacity to cartilage-derived cells and normal BMSCs | [51] | |
OA patients for TKA (n = 5) [57 (SD 3) vs 86 (SD 4) years old] | Osteogenic induction (FCS, BGP, DEX, A2P) | Similar osteogenic potential of IPFSCs between two age groups | [53] | |
OA patients for TKA (n = 25) [70 (SD 8) years old] | Osteogenic induction (BGP, DEX, A2P, cholecalciferol) | Inferior osteogenic capacity compared with knee ScASCs | [13] | |
OA patients for TKA (n = 7) | Osteogenic induction (BGP, A2P, DEX) | Comparable osteogenic capacity with periarticular ScASCs | [77] | |
OA elderly patients for TKA (n = 4) [70.5 (SD 9.2) years old] | Osteogenic induction (DEX, A2P, BGP) | Comparable osteogenic capacity with SDSCs but higher levels compared with ScASCs | [6] | |
Unknown donor | Female patients for TKA (n = 2) (65 and 68 years old) | Osteogenic induction (FBS, DEX, BGP, AA) or cultured in HA microenvironment | Confirmed in vitro osteogenic capacity and enhanced by HA microenvironment | [12] |
Patients for TKA (n = 5) (53–86 years old, mean age 74 years) | Transfected with adBMP2 followed by osteogenic induction (A2P, BGP) or seeded onto collagen type I sponges and implanted into SCID mice | Confirmed in vitro osteogenic capacity and in vivo formation of bone | [40] | |
Patients for TKA (n = 5) (mean age 73.6 years) | Transfected with adBMP2 combined with osteogenic induction followed by seeding onto collagen type I sponges and implanting into SCID mice | Confirmed in vivo bone formation with no difference compared with ScASCs | [76] | |
Patients for TKA or ACI surgery (three male and three female) (35–79 years old) | Osteogenic induction (FCS, BGP, DEX, AA) | Greater osteogenic capacity in vitro than SF MSCs | [52] | |
Patients for TKA (n = 53) (43–89 years old, mean age 72 years) | Osteogenic induction (BGP, A2P, BMP-2) | Confirmed in vitro osteogenic capacity | [41] | |
Patients for TKA (n = 16) [68 (SD 11.1) years old] | Osteogenic induction (FBS, A2P, BGP, DEX, 1, 25 vitamin D3) | Confirmed in vitro osteogenic capacity | [8] |
. | Tissue donor . | Treatment . | Results . | Reference . |
---|---|---|---|---|
Non-arthritis donor | Male C57BL/6J mice (fed a high-fat or a low-fat diet) | Osteogenic induction (BGP, AA, retinoic acid, BMP-2) | Higher osteogenic capacity of IPFSCs from obese mice compared with lean mice | [50] |
Porcine (n = 2) (4 months old) | Osteogenic induction (DEX, BGP, AA) | Confirmed in vitro osteogenic capacity | [60] | |
Young patients with ACL injury for reconstruction (n = 4) [17.2 (SD 0.7) years old] | Osteogenic induction (DEX, A2P, BGP) | Comparable osteogenic capacity with SDSCs but higher levels compared with ScASCs | [6] | |
Arthritis donor | OA patients for TKA (n = 7) (four female and three male) [71.4 (SD 8.3) years old] | Osteogenic induction (A2P, BGP, DEX) | Confirmed in vitro osteogenic capacity | [71] |
OA patients (n = 26) (mean age 75 years) | Osteogenic induction (DEX, A2P, BGP) | Similar osteogenic capacity to cartilage-derived cells and normal BMSCs | [51] | |
OA patients for TKA (n = 5) [57 (SD 3) vs 86 (SD 4) years old] | Osteogenic induction (FCS, BGP, DEX, A2P) | Similar osteogenic potential of IPFSCs between two age groups | [53] | |
OA patients for TKA (n = 25) [70 (SD 8) years old] | Osteogenic induction (BGP, DEX, A2P, cholecalciferol) | Inferior osteogenic capacity compared with knee ScASCs | [13] | |
OA patients for TKA (n = 7) | Osteogenic induction (BGP, A2P, DEX) | Comparable osteogenic capacity with periarticular ScASCs | [77] | |
OA elderly patients for TKA (n = 4) [70.5 (SD 9.2) years old] | Osteogenic induction (DEX, A2P, BGP) | Comparable osteogenic capacity with SDSCs but higher levels compared with ScASCs | [6] | |
Unknown donor | Female patients for TKA (n = 2) (65 and 68 years old) | Osteogenic induction (FBS, DEX, BGP, AA) or cultured in HA microenvironment | Confirmed in vitro osteogenic capacity and enhanced by HA microenvironment | [12] |
Patients for TKA (n = 5) (53–86 years old, mean age 74 years) | Transfected with adBMP2 followed by osteogenic induction (A2P, BGP) or seeded onto collagen type I sponges and implanted into SCID mice | Confirmed in vitro osteogenic capacity and in vivo formation of bone | [40] | |
Patients for TKA (n = 5) (mean age 73.6 years) | Transfected with adBMP2 combined with osteogenic induction followed by seeding onto collagen type I sponges and implanting into SCID mice | Confirmed in vivo bone formation with no difference compared with ScASCs | [76] | |
Patients for TKA or ACI surgery (three male and three female) (35–79 years old) | Osteogenic induction (FCS, BGP, DEX, AA) | Greater osteogenic capacity in vitro than SF MSCs | [52] | |
Patients for TKA (n = 53) (43–89 years old, mean age 72 years) | Osteogenic induction (BGP, A2P, BMP-2) | Confirmed in vitro osteogenic capacity | [41] | |
Patients for TKA (n = 16) [68 (SD 11.1) years old] | Osteogenic induction (FBS, A2P, BGP, DEX, 1, 25 vitamin D3) | Confirmed in vitro osteogenic capacity | [8] |
A2P: ascorbate acid-2-phosphate; BGP: B-glycerophosphate/beta-glycerol phosphate/glycerol phosphate; DEX: dexamethasone; FBS: fetal bovine serum; FCS: fetal calf serum.
Adipogenesis
Given that IPFP is primarily composed of adipose tissue, IPFSCs exhibit adipogenic potential [6, 8, 12, 54, 60, 71] (Table 4). Recent reports have show that IPFSCs from obese mice have increased adipogenesis compared with lean mice; free fatty acids enhanced adipogenesis of IPFSCs from lean mice [50]. Hyaluronan supplementation increased adipogenesis of IPFSCs [12]. Interestingly, He and Pei [47] demonstrated that dECM deposited from IPFSCs and SDSCs did not enhance adipogenic differentiation of IPFSCs. Increasingly, data have shown that IPFSCs have higher adipogenesis compared with BMSCs [51], SF MSCs [52] and chondrocytes [51], indicating the favourable capacities of IPFSCs under adipogenic induction. IPFSCs also had comparable adipogenic capacities compared with SDSCs [6]. Interestingly, IPFSCs had similar capacities of adipogenesis compared with ScASCs in young and elderly donors [6, 77]. These findings are in line with a report from Lopa et al. [13] in which IPFSCs displayed similar adipogenic potential compared with ScASCs from the peri-articular knee by upregulating adipogenic markers, although differentiated ScASCs had higher LEP (leptin) expression compared with differentiated IPFSCs.
. | Tissue donor . | Treatment . | Results . | Reference . |
---|---|---|---|---|
Non-arthritis donor | Male C57BL/6J mice (fed a high-fat or a low-fat diet) | Adipogenic induction (biotin, pantothenate, insulin, DEX, IBMX, rosiglitazone) | Higher adipogenic capacity of IPFSCs from obese mice compared with lean mice | [50] |
Porcine (n = 2) (4 months old) | Adipogenic induction (DEX, IBMX, indomethacin) | Confirmed in vitro adipogenic capacity | [60] | |
Young patients with ACL injury for reconstruction (n = 4) [17.2 (SD 0.7) years old] | Adipogenic induction (DEX, IBMX, indomethacin) | Comparable adipogenic capacity to SDSCs and ScASCs | [6] | |
Arthritis donor | OA elderly patients for TKA (n = 4) [70.5 (SD 9.2) years old] | Adipogenic induction (DEX, IBMX, indomethacin) | Comparable adipogenic capacity to SDSCs and ScASCs | [6] |
OA patients (n = 26) (mean age 75 years) | Adipogenic induction (IBMX, indomethacin, hydrocortisone) | Higher adipogenic capacity compared with cartilage-derived cells and BMSCs | [51] | |
OA patients for TKA (n = 6) | Adipogenic induction (DEX, insulin, IBMX, indomethacin) | Confirmed in vitro adipogenic capacity | [54] | |
OA patients for TKA (n = 25) [70 (SD 8) years old] | Adipogenic induction (DEX, insulin, IBMX, indomethacin) | Similar adipogenic capacity compared to knee ScASCs | [13] | |
OA patients for TKA (n = 7) | Adipogenic induction (IBMX, biotin, pantothenate, rosiglitazone, DEX, insulin) | Comparable adipogenic capacity to peri-articular ScASCs | [77] | |
OA patients for TKA (n = 7) (three male and four female) [71.4 (SD 8.3) years old] | Adipogenic induction (StemPro adipogenesis supplement) | Confirmed in vitro adipogenic capacity | [71] | |
Unknown donor | Female patients for TKA (65 and 68 years old) | Adipogenic induction (DEX, insulin, IBMX, indomethacin) or cultured in HA microenvironment | Confirmed in vitro adipogenic capacity and enhanced by HA microenvironment | [12] |
Patients for TKA or ACI surgery (n = 6) (three male and three female) (35–79 years old) | Adipogenic induction (FCS, ITS, IBMX, DEX, indomethacin) | Greater in vitro adipogenic capacity than SF MSCs | [52] | |
Patients for TKA (n = 53) (mean age 72 years) | Adipogenic induction (L-glutamine, insulin, IBMX, DEX, indomethacin) | Confirmed in vitro adipogenic capacity | [41] | |
Patients for TKA (n = 16) [68 (SD 11) years old] | Adipogenic induction (FBS, DEX, insulin, IBMX, rosiglitazone) | Confirmed in vitro adipogenic capacity | [8] |
. | Tissue donor . | Treatment . | Results . | Reference . |
---|---|---|---|---|
Non-arthritis donor | Male C57BL/6J mice (fed a high-fat or a low-fat diet) | Adipogenic induction (biotin, pantothenate, insulin, DEX, IBMX, rosiglitazone) | Higher adipogenic capacity of IPFSCs from obese mice compared with lean mice | [50] |
Porcine (n = 2) (4 months old) | Adipogenic induction (DEX, IBMX, indomethacin) | Confirmed in vitro adipogenic capacity | [60] | |
Young patients with ACL injury for reconstruction (n = 4) [17.2 (SD 0.7) years old] | Adipogenic induction (DEX, IBMX, indomethacin) | Comparable adipogenic capacity to SDSCs and ScASCs | [6] | |
Arthritis donor | OA elderly patients for TKA (n = 4) [70.5 (SD 9.2) years old] | Adipogenic induction (DEX, IBMX, indomethacin) | Comparable adipogenic capacity to SDSCs and ScASCs | [6] |
OA patients (n = 26) (mean age 75 years) | Adipogenic induction (IBMX, indomethacin, hydrocortisone) | Higher adipogenic capacity compared with cartilage-derived cells and BMSCs | [51] | |
OA patients for TKA (n = 6) | Adipogenic induction (DEX, insulin, IBMX, indomethacin) | Confirmed in vitro adipogenic capacity | [54] | |
OA patients for TKA (n = 25) [70 (SD 8) years old] | Adipogenic induction (DEX, insulin, IBMX, indomethacin) | Similar adipogenic capacity compared to knee ScASCs | [13] | |
OA patients for TKA (n = 7) | Adipogenic induction (IBMX, biotin, pantothenate, rosiglitazone, DEX, insulin) | Comparable adipogenic capacity to peri-articular ScASCs | [77] | |
OA patients for TKA (n = 7) (three male and four female) [71.4 (SD 8.3) years old] | Adipogenic induction (StemPro adipogenesis supplement) | Confirmed in vitro adipogenic capacity | [71] | |
Unknown donor | Female patients for TKA (65 and 68 years old) | Adipogenic induction (DEX, insulin, IBMX, indomethacin) or cultured in HA microenvironment | Confirmed in vitro adipogenic capacity and enhanced by HA microenvironment | [12] |
Patients for TKA or ACI surgery (n = 6) (three male and three female) (35–79 years old) | Adipogenic induction (FCS, ITS, IBMX, DEX, indomethacin) | Greater in vitro adipogenic capacity than SF MSCs | [52] | |
Patients for TKA (n = 53) (mean age 72 years) | Adipogenic induction (L-glutamine, insulin, IBMX, DEX, indomethacin) | Confirmed in vitro adipogenic capacity | [41] | |
Patients for TKA (n = 16) [68 (SD 11) years old] | Adipogenic induction (FBS, DEX, insulin, IBMX, rosiglitazone) | Confirmed in vitro adipogenic capacity | [8] |
IBMX: 1-methyl-3-isobutylxantine/isobutylmethylxanthine.
. | Tissue donor . | Treatment . | Results . | Reference . |
---|---|---|---|---|
Non-arthritis donor | Male C57BL/6J mice (fed a high-fat or a low-fat diet) | Adipogenic induction (biotin, pantothenate, insulin, DEX, IBMX, rosiglitazone) | Higher adipogenic capacity of IPFSCs from obese mice compared with lean mice | [50] |
Porcine (n = 2) (4 months old) | Adipogenic induction (DEX, IBMX, indomethacin) | Confirmed in vitro adipogenic capacity | [60] | |
Young patients with ACL injury for reconstruction (n = 4) [17.2 (SD 0.7) years old] | Adipogenic induction (DEX, IBMX, indomethacin) | Comparable adipogenic capacity to SDSCs and ScASCs | [6] | |
Arthritis donor | OA elderly patients for TKA (n = 4) [70.5 (SD 9.2) years old] | Adipogenic induction (DEX, IBMX, indomethacin) | Comparable adipogenic capacity to SDSCs and ScASCs | [6] |
OA patients (n = 26) (mean age 75 years) | Adipogenic induction (IBMX, indomethacin, hydrocortisone) | Higher adipogenic capacity compared with cartilage-derived cells and BMSCs | [51] | |
OA patients for TKA (n = 6) | Adipogenic induction (DEX, insulin, IBMX, indomethacin) | Confirmed in vitro adipogenic capacity | [54] | |
OA patients for TKA (n = 25) [70 (SD 8) years old] | Adipogenic induction (DEX, insulin, IBMX, indomethacin) | Similar adipogenic capacity compared to knee ScASCs | [13] | |
OA patients for TKA (n = 7) | Adipogenic induction (IBMX, biotin, pantothenate, rosiglitazone, DEX, insulin) | Comparable adipogenic capacity to peri-articular ScASCs | [77] | |
OA patients for TKA (n = 7) (three male and four female) [71.4 (SD 8.3) years old] | Adipogenic induction (StemPro adipogenesis supplement) | Confirmed in vitro adipogenic capacity | [71] | |
Unknown donor | Female patients for TKA (65 and 68 years old) | Adipogenic induction (DEX, insulin, IBMX, indomethacin) or cultured in HA microenvironment | Confirmed in vitro adipogenic capacity and enhanced by HA microenvironment | [12] |
Patients for TKA or ACI surgery (n = 6) (three male and three female) (35–79 years old) | Adipogenic induction (FCS, ITS, IBMX, DEX, indomethacin) | Greater in vitro adipogenic capacity than SF MSCs | [52] | |
Patients for TKA (n = 53) (mean age 72 years) | Adipogenic induction (L-glutamine, insulin, IBMX, DEX, indomethacin) | Confirmed in vitro adipogenic capacity | [41] | |
Patients for TKA (n = 16) [68 (SD 11) years old] | Adipogenic induction (FBS, DEX, insulin, IBMX, rosiglitazone) | Confirmed in vitro adipogenic capacity | [8] |
. | Tissue donor . | Treatment . | Results . | Reference . |
---|---|---|---|---|
Non-arthritis donor | Male C57BL/6J mice (fed a high-fat or a low-fat diet) | Adipogenic induction (biotin, pantothenate, insulin, DEX, IBMX, rosiglitazone) | Higher adipogenic capacity of IPFSCs from obese mice compared with lean mice | [50] |
Porcine (n = 2) (4 months old) | Adipogenic induction (DEX, IBMX, indomethacin) | Confirmed in vitro adipogenic capacity | [60] | |
Young patients with ACL injury for reconstruction (n = 4) [17.2 (SD 0.7) years old] | Adipogenic induction (DEX, IBMX, indomethacin) | Comparable adipogenic capacity to SDSCs and ScASCs | [6] | |
Arthritis donor | OA elderly patients for TKA (n = 4) [70.5 (SD 9.2) years old] | Adipogenic induction (DEX, IBMX, indomethacin) | Comparable adipogenic capacity to SDSCs and ScASCs | [6] |
OA patients (n = 26) (mean age 75 years) | Adipogenic induction (IBMX, indomethacin, hydrocortisone) | Higher adipogenic capacity compared with cartilage-derived cells and BMSCs | [51] | |
OA patients for TKA (n = 6) | Adipogenic induction (DEX, insulin, IBMX, indomethacin) | Confirmed in vitro adipogenic capacity | [54] | |
OA patients for TKA (n = 25) [70 (SD 8) years old] | Adipogenic induction (DEX, insulin, IBMX, indomethacin) | Similar adipogenic capacity compared to knee ScASCs | [13] | |
OA patients for TKA (n = 7) | Adipogenic induction (IBMX, biotin, pantothenate, rosiglitazone, DEX, insulin) | Comparable adipogenic capacity to peri-articular ScASCs | [77] | |
OA patients for TKA (n = 7) (three male and four female) [71.4 (SD 8.3) years old] | Adipogenic induction (StemPro adipogenesis supplement) | Confirmed in vitro adipogenic capacity | [71] | |
Unknown donor | Female patients for TKA (65 and 68 years old) | Adipogenic induction (DEX, insulin, IBMX, indomethacin) or cultured in HA microenvironment | Confirmed in vitro adipogenic capacity and enhanced by HA microenvironment | [12] |
Patients for TKA or ACI surgery (n = 6) (three male and three female) (35–79 years old) | Adipogenic induction (FCS, ITS, IBMX, DEX, indomethacin) | Greater in vitro adipogenic capacity than SF MSCs | [52] | |
Patients for TKA (n = 53) (mean age 72 years) | Adipogenic induction (L-glutamine, insulin, IBMX, DEX, indomethacin) | Confirmed in vitro adipogenic capacity | [41] | |
Patients for TKA (n = 16) [68 (SD 11) years old] | Adipogenic induction (FBS, DEX, insulin, IBMX, rosiglitazone) | Confirmed in vitro adipogenic capacity | [8] |
IBMX: 1-methyl-3-isobutylxantine/isobutylmethylxanthine.
The value of IPFP in regenerative medicine
IPFSCs have some unique properties in inflammation, senescence, accessibility and in vivo functionality compared with SDSCs and ScASCs.
Inflammation
The IPFP of OA patients has inflammatory phenotypes [79] that can secrete various pro-inflammatory factors such as TNF-α, IL-1β, IL-6, IL-6 receptor (IL-6R) and adipokines [80–82]. The inflammatory factors secreted by IPFP could subsequently influence stem cell properties. For example, Skalska et al. [74] found a similar expression of surface phenotypes of IPFSCs from both RA and OA patients and a comparable effect of chondrogenic induction that was decreased by supplementation of TNF in the medium. Wei et al. [83] demonstrated that conditioned medium from the IPFP of OA and traumatically injured joints inhibited the chondrogenesis of BMSCs by decreasing type II collagen and aggrecan expression. They also found that conditioned medium from IPFP-derived macrophages decreased the expression of hyaline cartilage genes, suggesting that resident macrophages may be responsible for deterioration in MSC-based cartilage repair [84].
Much evidence has shown that macrophages and T cells, followed by mast cells, are the most prominent immune cells contributing to inflammation in the synovium and IPFP [85]. Thus IPFP from OA was more active and secreted greater amounts of inflammatory factors, such as IL-6, IL-8 and prostaglandin E2, than ScAT [79, 80, 87]. Eymard et al. [87, 88] showed that IPFP-conditioned media from OA patients could induce autologous fibroblast-like synoviocytes to secrete greater levels of pro-inflammatory and pro-degenerative factors than those from ScAT, also suggesting that the IPFP of OA had a stronger pro-inflammatory capacity than that of ScAT.
Increasing studies have reported that chondrogenic differentiation could be inhibited when MSCs are exposed to the circulating inflammatory environment [86, 89]. However, IPFSCs from OA still exerted strong properties of proliferation and multidifferentiation [6, 8, 51, 71]. For example, IPFSCs from OA proliferated rapidly and had high growth kinetics and tri-lineage differentiation (chondrogenic, osteogenic and adipogenic) capacities that were superior or comparable to SDSCs and ScASCs [6, 12, 13]. Manferdini et al. [90] demonstrated that IPFSCs could downregulate the expressions of inflammatory factors such as IL-1β, IL-6 and IL-8 produced by chondrocytes or synoviocytes during co-culture. Therefore these studies indicated that IPFSCs could exert anti-inflammatory effects by undergoing robust proliferation and multidifferentiation in the presence of inflammation.
In OA, synovium may be heterogeneous and can vary in thickness due to fibrosis, haemorrhagic changes or inflammation [91], which affects the number and properties of SDSCs harvested from synovium [91, 92]. The secondary inflammation in synovium could further alter its cellular composition and stem cell populations [6]. In contrast, the recognition of inflammatory changes in the IPFP of an OA knee was less frequent compared with synovitis [51, 91]. Therefore IPFP may be a better stem cell source for tissue engineering compared with synovium.
Senescence
Much evidence has indicated that the capacities of MSCs for expansion and differentiation decreased with increasing passages in vitro and donor age [38]. This passage-dependent cell senescence was also found in the reduction of proliferation and differentiation capacity of IPFSCs. Pizzute et al. [48] demonstrated that dECM deposited by IPFSCs provided a ‘niche’-like microenvironment for cell expansion, but the viability and chondrogenic potential of IPFSCs decreased after expansion by multiple passage cells, indicating that replicative senescence may affect the proliferation rate and differentiation potentials [38]. However, no significant effect of passage number was found on population doublings of IPFSCs and surface epitope profile was maintained in the IPFSCs of both passages 10 and 18 [54]. Another report confirmed that IPFSCs preserved their chondrogenic and adipogenic potentials during extended passaging [51].
Despite the influence of donor age on BMSC proliferative capacities [93, 94] and chondrogenic [95], osteogenic [93, 96] and adipogenic differentiation [95], the independence of donor age in the expansion and differentiation potentials of IPFSCs also has been reported. Liu et al. [62] demonstrated that IPFSCs isolated from OA donors displayed comparable chondrogenic capacity to healthy donors without OA after a 28 day pellet culture. Khan et al. [53] also showed that IPFSCs from young and elderly donors had similar proliferation rates and osteogenic differentiation and were not affected by increasing age. Moreover, Mochizuki et al. [6] revealed that no remarkable differences were observed between young and elderly donors in terms of the proliferative ability of the cells at passage 1 as well as the chondrogenic, osteogenic and adipogenic potentials of IPFSCs. These studies indicated that IPFSCs could maintain consistent proliferation and multidifferentiation with increasing age, which may provide a sufficient stem cell source for tissue regeneration. However, there are an increasing number of studies that indicate a declining trend in proliferation rate and multilineage differentiation capacity of aged MSCs. The variability in species and interlaboratory differences in MSC isolation and culture might partially account for conflicting reports on the influence of aging on MSCs [97].
The abundant accessibility of IPFSCs
During knee replacement surgery due to chronic impingement and pain, the IPFP is routinely removed as surgical waste. There is little clinical evidence that taking partial biopsies of the IPFP is detrimental to patients [98, 99] and IPFP removed during surgery has considerable powers of regeneration similar to synovium [24, 34]; therefore, obtaining a large amount of IPFP without adverse effects is feasible for regenerative medicine. Given the anatomic location, the IPFP can be easily obtained from a biopsy during arthroscopy or open resection. Sufficient amounts of IPFP tissue can be harvested with a lower degree of invasiveness and possibly fewer complications compared with bone marrow aspiration [13, 40]. Although synovium can be obtained arthroscopically with a low incidence of complications, there is a small amount of synovium in the joint and difficulty separating it from surrounding tissues limits its extensive application [32, 100]. In addition, ScAT from lipoaspiration or open procedures is closely associated with complications such as scarring, surface irregularities or skin necrosis, haematoma, skin discoloration, temporary bruising and numbness [101, 102]. Therefore harvesting stem cells from the IPFP may be an excellent method for tissue engineering, especially for the treatment of chondral or osteochondral defects [6, 60].
IPFP tissue contains a large number of stem cells. Compared with a bone marrow aspirate of 30 ml producing ∼1 × 105 cells [103], the mean cell yield from one IPFP (mean volume 21 ml) was 5.5 × 106 cells [40]. Another report showed that 7.5 × 106 stem cells are usually isolated from 5 g of IPFP tissue [43]. Interestingly, Mochizuki et al. [6] demonstrated that the nucleated cell number per milligram of IPFP tissue was relatively less than synovium but substantially more than ScAT in young and elderly donors. Taken together, the comparison of stem cell yield efficiency and number, as well as associated complications during harvest, may support IPFSCs as an excellent stem cell source for costly and lengthy tissue-culture expansion.
In vivo functionality of IPFSCs
As mentioned above, the IPFP within the knee joint is a non-cartilaginous but intra-articular tissue that is primarily located between the joint capsule and the synovial membrane. It is composed of a relatively large amount of subsynovial adipose tissue and a thin layer of superficial synovium. Like MSCs from other sources, IPFSCs residing in IPFP tissue have a fibroblast-like appearance [12] and express similar cell phenotypes [43]. Studies have shown that IPFSCs stain poorly for low-affinity nerve growth factor receptor and STRO-1, which are surface markers expressed on freshly isolated BMSCs—a so-called gold standard MSC [43, 63, 54]. Moreover, IPFSCs express higher levels of CD34, CD45 [12] and CD166 [70] compared with BMSCs, indicating that IPFSCs are not identical to BMSCs [12]. However, both IPFSCs and BMSCs adhere to the International Society for Cellular Therapy criteria and share the same cell surface phenotypes, such as CD73, CD90 and CD105 [12, 70].
Given that IPFSCs possess significant multidifferentiation potential [8, 40, 51], intra-articular injection of IPFSCs into human OA knees and the in vivo implantation of IPFSCs into animals have been used for cartilage repair [64–66]. Furthermore, IPFSCs were able to release into the SF of the joint space, forming SF MSCs and promoting cartilage resurfacing [8, 104]. An IPFSC colony grown from canine fat pad digest adhered to superficial injured cartilage when injected into a canine joint cavity, suggesting that IPFSCs might have a role in native spontaneous joint repair in vivo [105]. In addition, involvement of the IPFP in the neo-vascularization of an anterior cruciate ligament graft suggests its role in the optimization of the graft’s revascularization and ultimate viability [106]. This evidence indicates that IPFSCs have unique advantages in regenerative medicine.
Conclusion and perspective
As an intracapsular adipose tissue that contains MSC populations with similarities to synovium and ScAT, the IPFP may be considered a superior engineering stem cell source for regenerative medicine. This review reports an increasing body of evidence showing that IPFSCs have similar properties to SDSCs in terms of cell morphologic features, proliferation capacity, chondrogenesis, osteogenesis and adipogenesis; both IPFSCs and SDSCs have superior properties compared with ScASCs. Moreover, elucidating IPFSC characteristics and derived tissues’ response to inflammation and senescence are important to demonstrate the IPFP’s advantages as an engineering stem cell source. Finally, the anatomic location, relative accessibility and minimal risk of complications make the IPFP a good source of stem cells for regenerative medicine.
However, some limitations exist to prevent further investigation into potential clinical applications. First, researchers have typically used IPFP tissues from patients undergoing knee surgery with a pathological diagnosis such as OA or anterior cruciate ligament injury. Data from these diseased samples may not be identical to those from healthy samples. Additional studies are necessary to confirm the distinctions of IPFSCs between healthy and degenerative tissues as well as injured tissues. Second, despite the fact that a certain number of reports cited in this article are from donor-matched studies, individual reports are also included to supplement and consolidate our conclusions. In the future, more experiments are needed for a donor-matched comparison of stem cells from different depots. Lastly, current studies mainly use two-dimensional in vitro expansion of IPFSCs, which is different from the three-dimensional in vivo environment. Recent studies have shown that dECM can mimic the organization of native ECM in vivo to rejuvenate stem cells’ proliferation and chondrogenic potential [4, 47, 48, 107]. Utilization of dECM to promote the properties of IPFSCs may ultimately benefit clinical application for tissue regeneration, particularly for cartilage engineering and regeneration.
Acknowledgements
We thank Suzanne Danley for editing the manuscript and Dr Jeffrey M. Gimble (Center for Stem Cell Research & Regenerative Medicine, Tulane University, New Orleans, LA, USA) for constructive advice.
Funding: This work was supported by research grants from the Musculoskeletal Transplant Foundation the National Institutes of Health (1R03AR062763-01A1 and 1R01AR067747-01A1 to M.P.) and a Study Abroad Scholarship from Jiangsu Province and Jiangsu Province Youth Medical Talents (QNRC2016344) to Y.S.
Disclosure statement: The authors have declared no conflicts of interest.
Comments