-
PDF
- Split View
-
Views
-
Cite
Cite
Junling Huai, Nan Gao, Yuanyuan Yao, Yanxin Du, Qiang Guo, Rongcheng Lin, JASMONATE ZIM-domain protein 3 regulates photomorphogenesis and thermomorphogenesis through inhibiting PIF4 in Arabidopsis, Plant Physiology, Volume 195, Issue 3, July 2024, Pages 2274–2288, https://doi.org/10.1093/plphys/kiae143
- Share Icon Share
Abstract
Light and temperature are 2 major environmental factors that affect the growth and development of plants during their life cycle. Plants have evolved complex mechanisms to adapt to varying external environments. Here, we show that JASMONATE ZIM-domain protein 3 (JAZ3), a jasmonic acid signaling component, acts as a factor to integrate light and temperature in regulating seedling morphogenesis. JAZ3 overexpression transgenic lines display short hypocotyls under red, far-red, and blue light and warm temperature (28 °C) conditions compared to the wild type in Arabidopsis (Arabidopsis thaliana). We show that JAZ3 interacts with the transcription factor PHYTOCHROME-INTERACTING FACTOR4 (PIF4). Interestingly, JAZ3 spontaneously undergoes liquid–liquid phase separation (LLPS) in vitro and in vivo and promotes LLPS formation of PIF4. Moreover, transcriptomic analyses indicate that JAZ3 regulates the expression of genes involved in many biological processes, such as response to auxin, auxin-activated signaling pathway, regulation of growth, and response to red light. Finally, JAZ3 inhibits the transcriptional activation activity and binding ability of PIF4. Collectively, our study reveals a function and molecular mechanism of JAZ3 in regulating plant growth in response to environmental factors such as light and temperature.
Introduction
Plants are continuously influenced by external environmental factors, such as light and temperature, in their whole growth stages. To sense and adapt to the environments, plants have evolved sophisticated signal perception and transmission systems. In the darkness, seedlings develop elongated hypocotyls, closed cotyledons forming apical hooks, and undifferentiated etioplasts, which is termed as skotomorphogenesis. Upon light illumination, seedlings undergo photomorphogenesis, characterized by the inhibition of hypocotyl growth, promotion of cotyledon opening, synthesis of chlorophyll and anthocyanin, and development of functional chloroplasts (Von Arnim and Deng 1996). Plants have evolved various types of photoreceptors, such as phytochromes (phyA, phyB, etc.), cryptochromes (cry1 and cry2), phototropins, and UV RESISTANCE LOCUS 8 (UVR8), to perceive and convey light signals, which ultimately modulate plant growth and development (Rizzini et al. 2011; Galvao and Fankhauser 2015). The architectural adaptations to elevated ambient temperatures (below the heat stress range), such as the elongation of hypocotyls and petioles, are collectively referred to as thermomorphogenesis (Quint et al. 2016). Light and temperature antagonistically regulate hypocotyl growth: light inhibits hypocotyl elongation, whereas elevated temperature promotes the elongation.
Biochemical and genetic approaches have identified a large number of components involved in light and temperature signaling. CONSTITUTIVE PHOTOMORPHOGENIC 1 (COP1) was identified as a predominant repressor of photomorphogenesis, which directly targets positive regulators for degradation through the 26S proteasome pathway (Deng et al. 1991; Lau and Deng 2012). PHYTOCHROME-INTERACTING FACTORs (PIFs) are a class of basic helix–loop–helix (bHLH) transcription factors that negatively and redundantly regulate photomorphogenesis (Leivar and Quail 2011). Among them, PIF4 acts as a hub that integrates light and temperature signaling in regulating seedling morphogenesis (Proveniers and van Zanten 2013, Qi et al. 2022). Liquid–liquid phase separation (LLPS) is the phenomenon of a phase transition in which proteins or protein–nucleic-acid mixtures form liquid droplets or condensates (also called membraneless organelles) (Alberti et al. 2019). A recent study showed that phyB spontaneously undergoes LLPS to assemble liquid-like droplets driven by its C terminus. Light and temperature are separately perceived by phyB via allosteric changes and spontaneous phase separation (Chen et al. 2022). One of the evening complex components, EARLY FLOWERING 3 (ELF3), was also identified as a thermosensor in Arabidopsis. ELF3 harboring a predicted prion domain (PrD) forms speckles and undergoes a sharp LLPS in response to higher temperatures (Jung et al. 2020). However, the function and mechanism of LLPS in regulating light signaling remain largely unknown.
The light signal is often cross-linked with multiple endogenous hormones to regulate cell elongation and seedling morphogenesis (de Wit et al. 2016). Among the phytohormones, jasmonic acid (JA) not only acts as an important defense hormone but also regulates a variety of plant growth and developmental processes (Huang et al. 2017; Hu et al. 2023). The JA biosynthesis and signaling pathways have been well demonstrated (Acosta and Farmer 2010). An Ile-conjugated form of jasmonic acid (JA-Ile) binds its receptor, CORONATINE INSENSITIVE1 (COI1), which forms a SKP1-Cullin1-F-box protein (SCF) ubiquitin E3 ligase (Yan et al. 2009). This SCF complex interacts with JASMONATE ZIM-domain (JAZ) proteins, which are then ubiquitinated and degraded via the 26S proteasome in response to JA signaling (Thines et al. 2007; Hu et al. 2023). JAZ degradation releases transcription factors such as Myelocytomatosis protein 2 (MYC2) and subsequently activates the expression of JA-responsive genes (Fernandez-Calvo et al. 2011; Qi et al. 2011). Recent studies indicate that JA participates in light and temperature signaling. JA suppresses COP1 activity to stabilize COP1's targets, thereby inhibiting hypocotyl elongation and stimulating cotyledon unfolding in etiolated Arabidopsis seedlings (Zheng et al. 2017). MYC2/MYC3/MYC4 activates the expression of HY5, whose protein represses the expression of cell elongation-related genes (Yi et al. 2020). Methyl Jasmonate treatment represses hypocotyl elongation under warm temperature (Zhu et al. 2021). Warm temperature also leads to an increased expression of genes controlling JA catabolism and altered levels of bioactive JA-Ile (Zhu et al. 2021). However, the interaction among light, temperature, and JA signals is still unclear.
In this study, we found that transgenic Arabidopsis overexpressing JAZ3 exhibited short hypocotyls and large cotyledon angles under red, far-red, and blue light as well as warm temperature (28 °C) conditions. JAZ3 physically interacted with PIF4 in vivo and in vitro. JAZ3 formed liquid-like droplets through LLPS both in planta and in vitro. Furthermore, JAZ3 represses the activity of PIF4. Our study reveals the role of JAZ3 in regulating photomorphogenesis and thermomorphogenesis.
Results
JAZ3 is involved in regulating photomorphogenesis and thermomorphogenesis
The genome of Arabidopsis thaliana contains 13 JAZ genes (JAZ1–JAZ13), which are divided into 5 subgroups (Chini et al. 2016; Guo et al. 2018). To study the function of JAZ genes in photomorphogenesis and thermomorphogenesis, we generated transgenic lines overexpressing each of the JAZ genes fused with GREEN FLUORESCENCE PROTEIN (GFP) and driven by the 35S promoter. In this study, we focused on the role of JAZ3, since the 35S:JAZ3-GFP plants showed altered morphogenic phenotypes. Immunoblotting assay showed that the JAZ3-GFP fusion protein was accumulated in 3 independent transgenic lines of 35S:JAZ3-GFP (Supplementary Fig. S1A). JAZ3-GFP fusion protein could be degraded upon 6 h of JA treatment (Supplementary Fig. S1B), indicating that the exogenous JAZ3 is functional. These 35S:JAZ3-GFP seedlings exhibited short hypocotyls and big cotyledons compared with Col and the jaz3 mutant, under continuous red, far-red, and blue light conditions, but not in darkness (Fig. 1, A to D). The cotyledon angles and cotyledon areas of 35S:JAZ3-GFP were larger than those of Col under red light (Supplementary Fig. S2, A and B). There was no significant difference between the jaz3 mutant and the Col wild type (Fig. 1, A to D). We also found that these transgenic lines showed short hypocotyls under low intensities (2 and 5 µmol m−2 s−1) of white light compared with Col (Supplementary Fig. S3). Moreover, we generated overexpression transgenic plants of JAZ3 fused with a Flag tag (35S:JAZ3-cFlag), which showed photomorphogenic phenotypes similar to those of 35S:JAZ3-GFP under red light conditions (Supplementary Fig. S4).
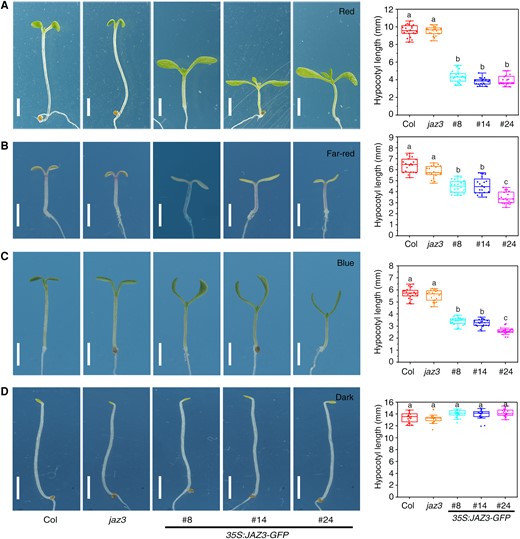
Overexpression of JAZ3 confers enhanced photomorphogenesis under different light conditions. Seedling phenotypes of Col, the jaz3 mutant, and the 35S:JAZ3-GFP transgenic lines grown under continuous red (A), far-red (B), blue (C) light conditions, or in the dark (D) for 5 d. Charts on the right indicate the average hypocotyl length of seedlings. Scale bars, 2 mm. Charts on the right indicate the average hypocotyl length of seedlings. Data are presented as boxplots. Each dot represents the datapoint of plant hypocotyl length (n = 20). The central line indicates the mean value. The bounds of the box show the first and third quartiles. Whiskers indicate a 1.5 × interquartile range between the first and third quartiles. Different letters above bars represent significant differences among different seedlings at the P < 0.05 level by 1-way ANOVA test.
Next, we investigated whether JAZ3 was involved in the response to increased temperature. Seedlings were grown under continuous white light (50 µmol m−2 s−1) at 22 or 28 °C conditions for 7 d. There were no obvious differences among the mutant, transgenic lines, and wild-type seedlings at 22 °C. However, the 35S:JAZ3-GFP lines had significantly shorter hypocotyls than Col and jaz3 when grown under the same light intensity at 28 °C (Fig. 2). Together, these results indicate that JAZ3 is involved in regulating seedling morphogenesis in response to both light and warm temperature.
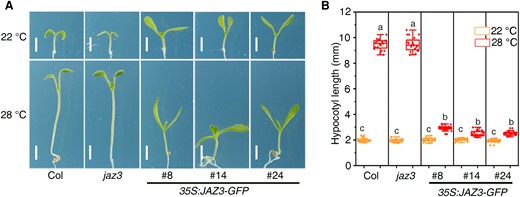
Overexpression of JAZ3 represses thermomorphogenesis. A) Seedling phenotypes of Col, jaz3, and the 35S:JAZ3-GFP transgenic lines grown under 22 or 28 °C conditions for 7 d. Scale bars, 2 mm. B) Average hypocotyl length of seedlings shown in (A). Data are presented as boxplots. Each dot represents the datapoint of plant hypocotyl length (n = 20). The central line indicates the mean value. The bounds of the box show the first and third quartiles. Whiskers indicate a 1.5 × interquartile range between the first and third quartiles. Different letters above bars represent significant differences among different seedlings at the P < 0.05 level by 1-way ANOVA test.
Regulation of JAZ3 by light and temperature
We detected the expression pattern of JAZ3 in cop1-4 and different photoreceptor mutants under different light conditions. As shown in Supplementary Fig. S5A, JAZ3 mRNA level was decreased in the cop1-4 mutant compared with Col when seedlings were grown in darkness. The JAZ3 transcript level was slightly increased in the phyA-211 mutant compared with Col under far-red light. However, the expression levels of JAZ3 were not significantly affected in the phyB-9 and cry1-304 mutants under red and blue light, respectively. Furthermore, the expression of JAZ3 was not significantly changed during the 22 to 28 °C transition (Supplementary Fig. S5B).
Next, we investigated whether light and temperature regulate protein accumulation of JAZ3. Interestingly, during the dark-to-light transition, JAZ3 was gradually increased and then decreased (Supplementary Fig. S5C). Warm temperature also induced protein accumulation of JAZ3 (Supplementary Fig. S5D). These results indicate that JAZ3 can be regulated by light and temperature.
JAZ3 interacts with PIF4
PIF4 is a key transcription factor in mediating photomorphogenesis and thermomorphogenesis (Qi et al. 2022). We therefore investigated whether JAZ3 could interact with PIF4. Since full-length PIF4 has self-activation activity, a truncated version of PIF4 without the activation domain was fused with the GAL4 DNA-binding domain (GBD-PIF4-ΔAD) (Zhu et al. 2016). We found that GBD-PIF4-ΔAD could interact with JAZ3 fused with the GAL4 activation domain (GAD-JAZ3), but not GAD itself, in the yeast 2-hybrid assay (Fig. 3A). Next, we conducted firefly luciferase (LUC) complementation imaging (LCI) assay. JAZ3 was fused with the N-terminal half of LUC (JAZ3-nLUC) and PIF4 was fused with the C-terminal half of LUC (cLUC-PIF4). Strong LUC luminescence was reconstituted in Nicotiana benthamiana leaves co-infiltrated with JAZ3-nLUC and cLUC-PIF4 constructs compared with their corresponding controls (Fig. 3B).
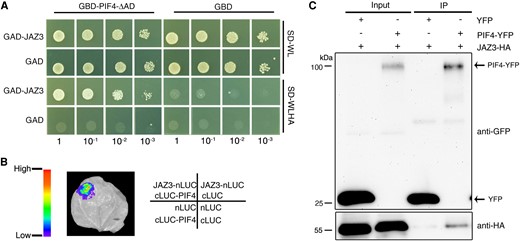
JAZ3 interacts with PIF4. A) Yeast 2-hybrid assay examining the interaction between JAZ3 and PIF4. PIF4 was fused to the GAL4 DNA-binding domain (GBD); JAZ3 was fused to the GAL4 activation domain (GAD). SD-WL, medium lacking Trp (W) and Leu (L); SD-WLHA, medium lacking Trp (W), Leu (L), His (H), and Ade (A). B) LCI assay showing the interaction between JAZ3 and PIF4 in N. benthamiana leaves. JAZ3 and PIF4 were fused to the N-terminal half and C-terminal half of firefly LUC, respectively. C) Co-IP assay. Plasmids containing PIF4-YFP and/or JAZ3-HA were co-transformed into Arabidopsis protoplasts. The immunoprecipitation assay was performed using anti-GFP-agarose, and proteins were analyzed by immunoblotting with anti-GFP and anti-HA antibodies. IP, immunoprecipitation.
To further verify their interaction, we performed a semi-in vivo co-immunoprecipitation (Co-IP) assay in Arabidopsis protoplasts. JAZ3 was tagged with Hemagglutinin (HA) and PIF4 was fused with yellow fluorescence protein (YFP). The constructs were co-overexpressed in protoplasts for 16 h and protein extracts were subsequently immunoprecipitated with anti-GFP beads. JAZ3-HA was successfully pulled down when co-expressed with PIF4-YFP, but not with YFP alone (Fig. 3C). These results indicate that JAZ3 physically interacts with PIF4 in vitro and in vivo. In addition, we found that some of the other JAZ proteins also interacted with PIF4 in the yeast and LCI assay (Supplementary Fig. S6, A and B).
JAZ3 undergoes LLPS in vivo and in vitro
To examine the subcellular localization of JAZ3, we infiltrated JAZ3-GFP into N. benthamiana leaves. Surprisingly, we found that JAZ3 protein formed speckles in the nucleus (Fig. 4A). We then examined the dynamics of JAZ3-GFP speckles using fluorescence recovery after photobleaching (FRAP) assay. The fluorescent intensity of JAZ3-GFP speckles rapidly recovered within about 10 s after photobleaching, indicating that these speckles are highly dynamic (Fig. 4, B and C). Previous results showed that some proteins employ their PrD to drive phase separation (Molliex et al. 2015; Fang et al. 2019; Jung et al. 2020). Protein structure prediction (Lancaster et al. 2014) indicated that JAZ3 contains a PrD domain in the middle region (Fig. 4D).
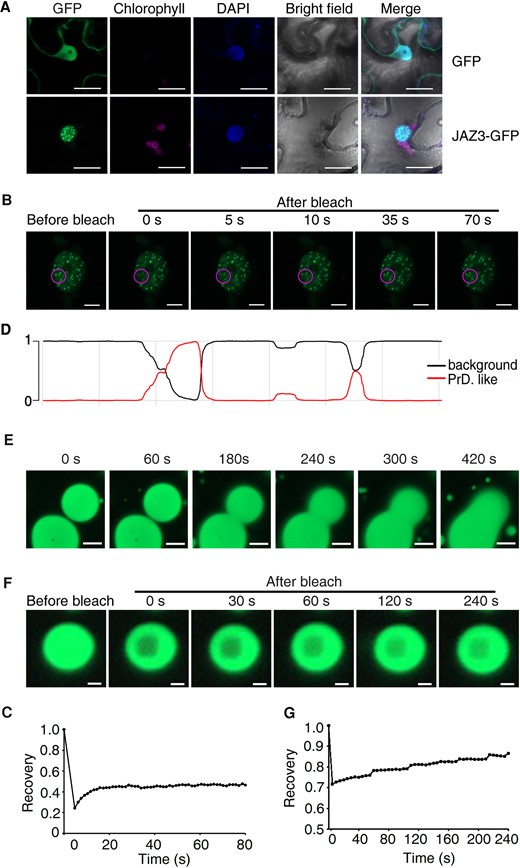
JAZ3 undergoes LLPS in vivo and in vitro. A) GFP fluorescence of 35S:GFP or 35S:JAZ3-GFP transiently expressed in N. benthamiana leaves. DAPI staining shows nuclei. Scale bar, 10 µm. B) FRAP experiment of JAZ3-GFP speckles. Time 0 indicates the time of the photobleaching pulse; the purple circle shows the bleaching area. Scale bar, 5 µm. C) Plot showing the time course of the recovery rate after photobleaching JAZ3-GFP speckles of (B). D) Prediction of the PrD (denoted by the red line reaching 1) of JAZ3 protein by the Prion-Like Amino Acid Composition (PLAAC) algorithm. E) Time-lapse live images showing the fusion of recombinant JAZ3-GFP protein droplets in the presence of 10% (w/v) PEG. Scale bar, 10 µm. F) FRAP experiment of the JAZ3-GFP droplets. Time 0 indicates the time of the photobleaching pulse. Scale bar, 1 µm. G) Plot showing the time course of the recovery rate after photobleaching His-JAZ3-GFP-MBP droplets of (F).
Next, we examined whether JAZ3 could undergo phase separation in vitro. JAZ3-GFP was fused with His tag and maltose-binding protein (MBP) at its N- and C-terminus, respectively. The His-JAZ3-GFP-MBP construct was expressed in Escherichia coli and recombinant proteins were purified. The His-JAZ3-GFP-MBP recombinant protein formed spherical droplets by the addition of crowding agent polyethylene glycol (PEG) (Supplementary Fig. S7). JAZ3 droplets fused into big droplets rapidly when contacting each other (Fig. 4E). FRAP experiments were performed to detect the mobility of the JAZ3 droplets. We found that JAZ3 fluorescence signal recovered gradually after photobleaching (Fig. 4, F and G). These results suggest that JAZ3 undergoes LLPS. We also analyzed the structure of the other JAZ proteins and did not find any PrD domain, suggesting the specificity of JAZ3.
JAZ3 promotes PIF4 with LLPS ability
Previous studies showed that PIF3 and PIF7 proteins can migrate to nuclear speckles following light treatment and co-localize with phyB (Bauer et al. 2004; Leivar et al. 2008). We wondered whether PIF4 could form nuclear speckles. PIF4 does not harbor a typical PrD domain (Supplementary Fig. S8A; Lancaster et al. 2014). When transiently expressed in N. benthamiana leaves, PIF4-GFP fusion proteins formed nuclear speckles (Supplementary Fig. S8B), as previously mentioned (Luo et al. 2022). The FRAP experiment showed that the fluorescence signal of PIF4-GFP could not recover after bleaching (Supplementary Fig. S8C). These observations suggest that PIF4 could not undergo LLPS.
Next, we investigated whether the nuclear speckles of PIF4 co-localized with JAZ3. JAZ3-GFP and PIF4-mCherry constructs were co-expressed in the N. benthamiana leaves and the merged fluorescence signals showed that PIF4 and JAZ3 co-localized in the same speckles (Fig. 5A). As expected, the recombinant mCherry-PIF4 proteins were diffused and could not form droplets in the presence of PEG (Supplementary Fig. S7). However, when mCherry-PIF4 was incubated with JAZ3-GFP, it formed liquid droplets and co-localized with JAZ3-GFP (Fig. 5B). FRAP analysis showed that the intensity of mCherry-PIF4 fluorescence signal was recovered completely in the presence of JAZ3-GFP (Fig. 5, C and D). Salt often affects the ability of LLPS (Ouyang et al. 2020). With the increase of salt ion concentration, the droplets formed by JAZ3 and PIF4 were gradually irregular and diffused (Supplementary Fig. S9). These results suggest that JAZ3 may promote PIF4 with LLPS ability in vitro.
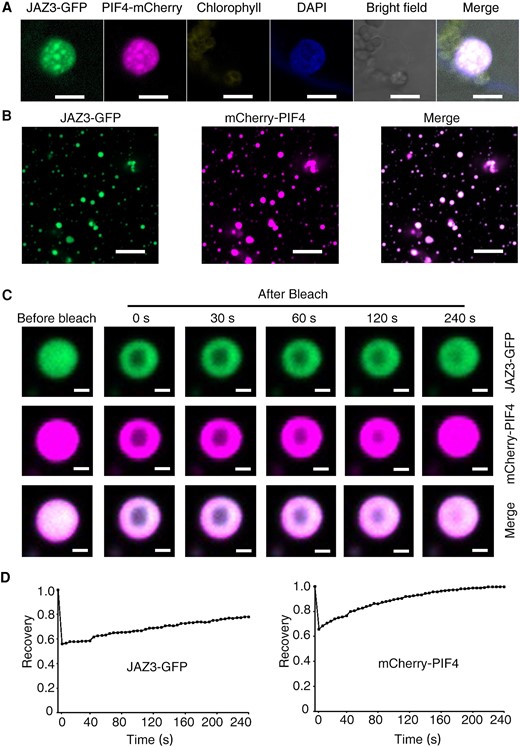
JAZ3 promotes PIF4 with LLPS ability. A) Fluorescence imaging of 35S:JAZ3-GFP or 35S:PIF4-mCherry transiently co-expressed in N. benthamiana leaves. DAPI staining shows nuclei. Scale bar, 10 µm. B) Co-localization of recombinant protein droplets of JAZ3-GFP and mCherry-PIF4 in the presence of 10% PEG. Scale bar, 5 µm. C) FRAP experiment of co-incubated droplets of JAZ3-GFP and mCherry-PIF4. Time 0 indicates the time of the photobleaching pulse. Scale bar, 1 µm. D) Plot showing the time course of the recovery rate after photobleaching JAZ3-GFP and mCherry-PIF4 droplets of (C).
Characterization of the transcriptome of JAZ3
To investigate how JAZ3 regulates photomorphogenesis and thermomorphogenesis, we used high throughput transcriptome RNA sequencing (RNA-seq) to analyze global gene expression changes between Col and 35S:JAZ3-GFP (Line #24) under continuous red light and 28 °C conditions for 5 and 7 d, respectively. Genes with more than 1.5-fold change were considered to be differentially expressed (P < 0.05). Under red light, 1,071 genes were upregulated and 1,632 genes were downregulated in 35S:JAZ3-GFP compared with Col (Supplementary Data Set S1). Under 28 °C conditions, 629 genes were upregulated and 1,477 genes were downregulated in 35S:JAZ3-GFP compared with Col (Supplementary Data Set S1). Gene ontology (GO) enrichment analysis revealed that the downregulated genes under red light are largely involved in response to auxin, endogenous stimuli, hormones, cell wall organization, or biogenesis and act in the extracellular region, cell periphery, and cell wall (Supplementary Fig. S10A). Genes upregulated in 35S:JAZ3-GFP under red light are mostly involved in response to stimuli, stress, oxygen-containing compounds, and abscisic acid, and function in chloroplast and thylakoid (Supplementary Fig. S10B). A majority of downregulated genes under warm temperature participate in response to stimulus, stress, and chemicals, and take effect in cell periphery, intrinsic component of the membrane, and plasma membrane (Supplementary Fig. S11A). The genes upregulated under warm temperature are mostly enriched in response to stimuli, radiation, light stimulus, and hormones and function in chloroplast, plastic part, and plastid stroma (Supplementary Fig. S11B).
We compared transcriptomic changes of 35S:JAZ3-GFP under red light with 28 °C condition. There were 1,095 genes co-regulated by the red light and 28 °C condition, indicating a substantial overlap between the 2 pathways (Fig. 6A). We also compared transcriptomic changes of 35S:JAZ3-GFP under red light with PIF4-regulated genes (Oh et al. 2012) and found that 660 genes were co-regulated by both JAZ3 and PIF4 (Fig. 6B). GO analysis revealed that these co-regulated genes are involved in response to auxin, auxin-activated signaling pathway, and regulation of growth (Fig. 6C), suggesting that JAZ3 and PIF4 may jointly regulate the expression of downstream genes in controlling hypocotyl growth.
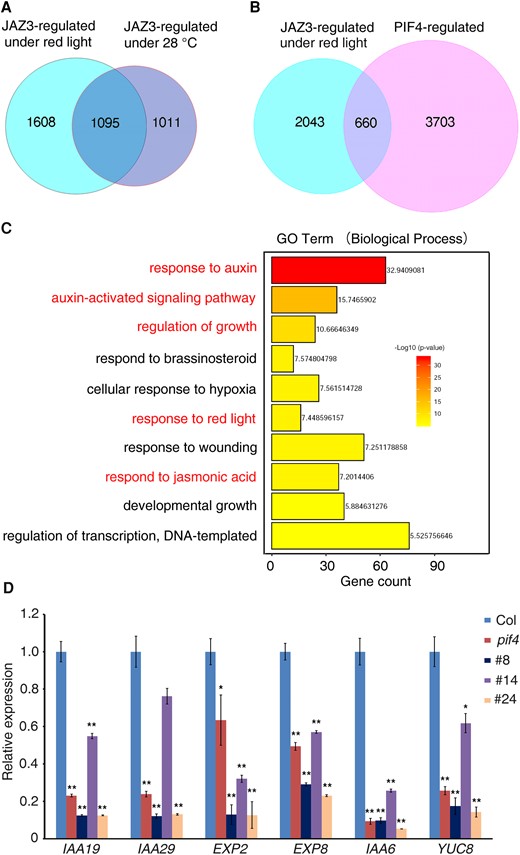
Transcriptomic analyses of JAZ3- and PIF4-regulated genes. A) Venn diagram of genes differentially regulated by JAZ3 under red light and 28 °C condition. B) Venn diagram of genes differentially regulated by JAZ3 and PIF4. Differentially regulated genes by JAZ3 under red light were analyzed with PIF4-regulated genes. C) GO analysis (biological process) of JAZ3 and PIF4 co-regulated genes. D) Confirmation of JAZ3 and PIF4 co-regulated genes by RT-qPCR. Relative expression was normalized to the level of Actin control and the relative transcript levels in Col were set 1. Data are means ± Sd of 3 biological repeats. Asterisks indicate significant differences based on the Student's t-test (**P < 0.01; *P < 0.05).
Among the 660 co-regulated genes, we selected several representative genes, including INDOLE-3-ACETIC ACID INDUCIBLE 19 (IAA19), IAA29, YUCCA8 (YUC8), and EXP2 for further analysis by reverse transcription quantitative PCR (RT-qPCR). The transcript levels of all 6 genes were decreased in 35S:JAZ3-GFP transgenic lines and the pif4 mutant compared with the Col wild type (Fig. 6D), consistent with the RNA-seq results. These results indicate that JAZ3 and PIF4 may have both distinct and overlapping roles in regulating downstream gene expression.
JAZ3 inhibits the transcriptional activation activity and binding ability of PIF4
To examine the functional relationship between JAZ3 and PIF4, we crossed the 35S:JAZ3-GFP line (Kanamycin resistance) with 35S:PIF4-GFP line (Basta resistance), and the double homozygous line was obtained. The expression of JAZ3-GFP and PIF4-GFP protein was confirmed by western blot (Supplementary Fig. S12). The 35S:JAZ3-GFP 35S:PIF4-GFP seedlings had intermediate hypocotyl lengths compared with the 2 parent lines and were similar to the wild type (Fig. 7, A and B), suggesting that JAZ3 and PIF4 antagonistically regulate hypocotyl growth.
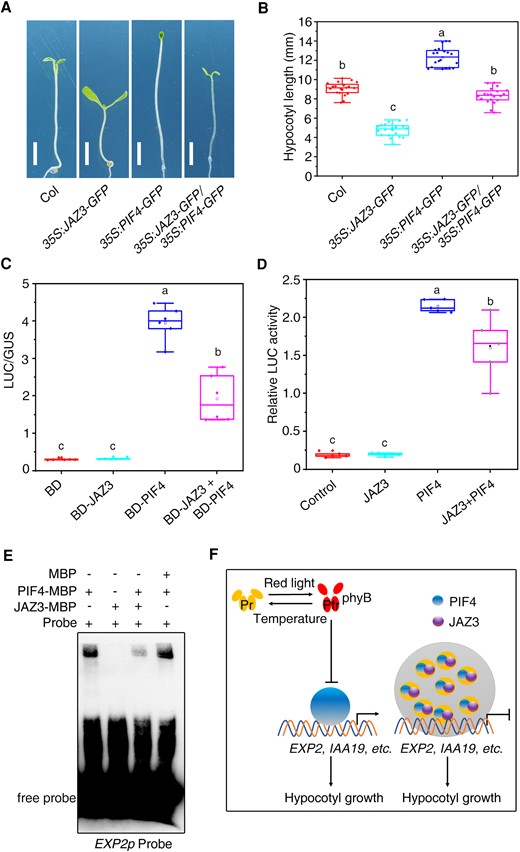
JAZ3 represses the binding of PIF4 to the downstream gene. A) Seedling phenotype of various genotypes grown under continuous red light for 5 d. B) Average hypocotyl length of seedlings shown in (A). Data are presented as boxplots. Each dot represents the datapoint of plant hypocotyl length (n = 20). C) Relative LUC activity in a transient expression assay. Different effectors were co-transformed with the GAL4p:LUC reporter and 35S:GUS internal control into Arabidopsis protoplasts. Relative activity was expressed as the ratio of LUC to GUS. Data are presented as boxplots. Each dot represents the datapoint of 1 biological replicate (n = 5). D) Transient expression assay of EXP2p:LUC reporter. JAZ3 and/or PIF4 were co-expressed with the EXP2p:LUC reporter gene in Arabidopsis protoplasts. Relative LUC activity was normalized to the Renilla (REN) internal control. Data are presented as boxplots. Each dot represents the datapoint of 1 biological replicate (n = 5). For (C), (D), and (E), the central line indicates the mean value. The bounds of the box show the first and third quartiles. Whiskers indicate a 1.5× interquartile range between the first and third quartiles. Different letters above bars represent significant differences among different seedlings at the P < 0.05 level by 1-way ANOVA test. E) EMSA assay. Recombinant proteins were incubated with the biotin-labeled oligonucleotides of EXP2. F) A proposed working model for the coordinated regulation of photo- and thermo-morphogenesis by JAZ3. Red light promotes the active form (Pfr) of phyB, while warm temperature triggers transformation of Pfr into Pr form. PhyB represses the PIF4 activity. JAZ3 undergoes phase separation to recruit PIF4 and repress its transcription activity to the downstream genes, such as EXP2. Arrows and bars represent positive and negative regulation, respectively.
Next, we fused JAZ3 and PIF4 with the GAL4 DNA-binding domain (BD) and assessed the transcriptional activity using a transient LUC expression system in Arabidopsis protoplasts (Jing et al. 2013). While BD-PIF4 drastically activated LUC expression, BD-JAZ3 did not affect LUC activity compared to the BD control (Fig. 7C). Strikingly, the transcriptional activation activity of PIF4 was significantly suppressed by the addition of JAZ3 (Fig. 7C). To further confirm this regulation, we generated a reporter construct by fusing the EXP2 promoter (1 kb upstream of the translation start code) to driving LUC (EXP2p:LUC) and performed a transient LUC assay in protoplasts. Overexpression of PIF4 activated EXP2p:LUC expression, whereas co-expression of JAZ3 inhibited the LUC activity by PIF4 (Fig. 7D). These results indicate that JAZ3 represses the transcriptional activation activity of PIF4 toward downstream gene expression.
To further investigate the repressive ability of JAZ3 to PIF4, we performed an electrophoretic mobility shift assay (EMSA) using JAZ3-MBP and PIF4-MBP recombinant proteins and DNA probes of an EXP2 promoter fragment containing a G-box motif (−313 to −318 bp). As shown in Fig. 7E, PIF4-MBP bound to the G-box-containing probes of EXP2, whereas JAZ3-MBP failed to bind to the probes. Strikingly, the addition of JAZ3-MBP, but not MBP itself, effectively suppressed the binding ability of PIF4, implying that JAZ3 represses the transcriptional activation activity of PIF4 partly via interfering with PIF4's binding to the targets.
Discussion
JAZ3 is a regulatory factor involved in photomorphogenesis and thermomorphogenesis
Light and temperature are 2 major environmental factors that affect plant growth and development. Accumulating evidence support the emerging signaling networks involved in photomorphogenesis and thermomorphogenesis (Casal and Balasubramanian 2019; Wang and Lin 2020; Cheng et al. 2021). Previous studies have revealed that components of the JA signaling pathway, such as MYC2, MYC3, and MYC4, are involved in regulating the light and temperature responses (Yi et al. 2020; Zhu et al. 2021); however, the underlying mechanisms are largely unknown. In this study, we generated overexpression transgenic lines of JAZ3 and showed that the 35S:JAZ3-GFP seedlings displayed reduced hypocotyl elongation under continuous red, far-red, blue, and weak white light conditions (Fig. 1, Supplementary Fig. S3). The 35S:JAZ3-cFlag transgenic lines had similar short hypocotyl phenotype when tested under red light (Supplementary Fig. S4). In addition, overexpression of JAZ3 led to increased cotyledon size and angle (Supplementary Fig. S2, A and B). Our data indicate that JAZ3 can modulate both hypocotyl and cotyledon growth and development. Moreover, the 35S:JAZ3-GFP seedlings were less sensitive to warm temperature-promoted hypocotyl growth than the wild type (Fig. 2).
Consistent with its biological roles, the protein levels of JAZ3 were mildly regulated by light and temperature (Supplementary Fig. S5, C and D). Surprisingly, jaz3 mutant did not show hypocotyl elongation difference under the tested light and 28 °C conditions (Figs. 1 and 2), further suggesting that the JAZ proteins may have redundant functions. Different double or high-order mutants among the jaz mutants shall help to address this issue. The roles of the other JAZs in regulating light and temperature responses require further investigation. Nevertheless, our study identifies JAZ3 as a component of the light and temperature signaling pathways.
JAZ3 interacts with PIF4 and inhibits its transcriptional activation activity and binding ability
As an important light and temperature signaling component, PIF4 binds to thousands of target genes to regulate their expression, such as IAA19 and YUC8, to promote plant hypocotyl elongation and growth (Franklin et al. 2011; Oh et al. 2012; Huai et al. 2018). At the same time, PIF4 is regulated at multiple levels, such as transcription, posttranslational modification, DNA-binding ability, transcriptional activity, and protein stability (Qiu 2020). Previous results stated that lots of proteins interact with PIF4 and control the posttranslational regulation or the downstream transcriptional regulation, including photoreceptors, E3 ubiquitin ligase chromatin remodeling factors, transcriptional factors, kinase, histone deacetylases, and so on (Xu and Zhu 2021). Overall, these PIF-interacting proteins can be divided into positive regulatory factors and negative regulatory factors.
In the dark, phyB exists in the biologically inactive Pr form, while under red light, phyB transforms into a biologically active Pfr state and interacts with PIF4. PIF4 is phosphorylated and subsequently ubiquitinated and degraded through the 26S proteasome-mediated degradation system (Lorrain et al. 2008). Under low blue light, the physical interactions between CRYs and PIF4/5 are facilitated and they are associated with the promoters of the growth-promoting genes to promote hypocotyl growth (Pedmale et al. 2016). Under high temperature, CRY1 interacts directly with PIF4 and suppresses the transcription activity of PIF4 in a blue light-dependent manner (Ma et al. 2016). SEU coordinates with PIF4 to activate auxin biosynthetic and responsive gene expression (Huai et al. 2018). PIF4-mediated thermomorphogenetic control of growth-promoting gene expression also requires the necessary transcriptional coactivator HEMERA (HMR) (Qiu et al. 2019). PIF4 and HMR physically interacted with MED14 and recruited the Mediator complex MED14 to activate auxin-related growth-promoting genes when plants sense moderate increases in ambient temperature (Bajracharya et al. 2022).
In our research, JAZ3 interacts with PIF4 directly in vivo and in vitro. According to the RNA-seq results, the genes regulated by the JAZ3 and PIF4 are largely overlapped (Fig. 6B). The transient LUC expression assay and the transient LUC reporter assay both indicate that JAZ3 can repress the transcriptional activity of PIF4 (Fig. 7, C and D). EMSA assay also confirmed that JAZ3 represses the transcriptional activity of PIF4 toward downstream gene expression (Fig. 7E). The multiple protein–protein interactions among PIF4 and PIF-interacting proteins form complex regulatory networks that enable plants to adapt to light or temperature changes.
JAZ3 promotes PIF4 with LLPS ability and represses its function
Previous results mentioned that JAZ1 formed nuclear speckles in the Nicotiana tabacum Bright Yellow 2 cells (Grunewald et al. 2009). But whether the JAZ1 protein possesses the phase separation ability had not been illustrated. In our study, we provide conclusive evidence that JAZ3 protein, which is a repressor of JA signaling, undergoes phase separation in vivo and in vitro (Fig. 4). Our results expand the research field of phase separation.
As a key hub integrating light signaling and temperature signaling, the LLPS of PIF4 has not been elucidated before. Though they form nuclear speckles in N. benthamiana, the intensity of fluorescence signal could not recover after bleaching (Supplementary Fig. S8, B and C). Recombinant mCherry-PIF4 protein was diffuse and could not form droplets (Supplementary Fig. S7). Amazingly, when mixed with JAZ3, the PIF4 protein formed liquid and co-localized with JAZ3 (Fig. 5B). At the same time, the intensity of PIF4 fluorescence recovered completely after FRAP (Fig. 5, C and D). These results show that JAZ3 may promote PIF4 with LLPS ability in vitro. We speculate that JAZ3 forms speckles and wraps PIF4 to suppress the transcriptional activation of PIF4 to the downstream genes (Fig. 7F). We found that PIF4 is promoted with LLPS ability by the JAZ3 protein. JAZ3-mediated LLPS may inhibit the transcriptional ability of PIF4 in an effective manner.
Recent works on LLPS suggest that it is a critical aspect of chromatin compartmentalization. JAZ3 drives the formation of JAZ3-PIF4 condensates, which in turn compact and organize chromatin regions. The condensates may recruit other histone deacetylases or histone acetyltransferases to facilitate chromatin remodeling and modification, which in turn promote or inhibit the downstream gene expression. Recently, MED25 was reported to form a complex with PIF1/PIF3 and HDA19 and form liquid-like condensates through phase separation to suppress target gene expression and promote skotomorphogenesis (Guo et al. 2023). So, it seems that the regulatory mechanism is widely present and beneficial for plants to better adapt to changes in the external environment.
Materials and methods
Plant materials, growth conditions, and phenotypic analyses
Arabidopsis (A. thaliana) pif4-1 (SALK _140393C), phyA-211, phyB-9, cry1-304, and cop1-4 (Jing et al. 2013) are in the Col ecotype background. The jaz3 (SALK _067825) mutant was obtained from the Arabidopsis Biological Resource Center and is in the Col ecotype background. 35S:PIF4-GFP transgenic line was constructed by our laboratory (Huai et al. 2018).
Seeds were sterilized, sown on 1X Murashige and Skoog (MS) medium (Phyto Technology Laboratories) supplemented with 1% (w/v) sucrose and 1% (w/v) agar (Sigma), and incubated at 4 °C in darkness for 3 d. For the photomorphogenesis experiments, the seedlings were grown in darkness or under continuous far-red (12 µmol m−2 s−1), red (40 µmol m−2 s−1), blue (10 µmol m−2 s−1) light, or white light (50 µmol m−2 s−1) for 5 d or as indicated. For thermomorphogenic treatments, the seedlings were grown under continuous white light (50 µmol m−2 s−1) at 22 or 28 °C for 7 d. All light was supplied by light-emitting diodes. For examination of hypocotyl length, cotyledon angle, and cotyledon area, the seedlings were placed on MS plates, photographed with a digital camera (Olympus), and measured using NIH ImageJ software (http://rsbweb.nih.gov/ij/).
Plasmid construction
The coding sequences of genes were amplified using high-fidelity pfu DNA polymerase (Invitrogen). The coding sequence of JAZs and PIF4/PIF4-Δad were cloned into pEASY-Blunt vector (TransGen), respectively, to generate pEASY-JAZs and pEASY-PIF4. The JAZs and PIF4 fragments were cut from the pEASY-JAZs or pEASY-PIF4 vectors independently using EcoRI/MfeI and SalI/XhoI and cloned into the pRI-GFP or pRI-cFlag vector modified by our lab to generate 35S:JAZs-GFP, 35S:JAZ3-cFlag, and 35S:PIF4-GFP. The PIF4 fragment was cloned into pRI-mCherry vector modified by our lab also using EcoRI and SalI to generate 35S:PIF4-mCherry.
To construct the vectors for the yeast 2-hybrid assay, pEASY-JAZs were digested with EcoRI/MfeI and SalI/XhoI and cloned into pGADT7 vector (Clontech) to generate GAD-JAZs. PIF4-ΔAD were cloned into pGBKT7 vector using EcoRI and SalI to generate GBD-PIF4-ΔAD.
To construct vectors for transient expression in Arabidopsis mesophyll protoplasts, PIF4 was ligated into pUC18-YFP to generate pUC18-PIF4-YFP. The JAZ3 was constructed into pUC18-3HA vector to produce pUC18-JAZ3-HA.
To construct the vectors for the LCI assay, the full-lengths of PIF4 and JAZs were cloned into pCAMBIA1300-cLUC and pCAMBIA1300-nLUC vectors (Chen et al. 2008) to generate cLUC-PIF4 and JAZs-nLUC, respectively.
To construct the vectors for the transient LUC activity assay, the full-lengths of PIF4 and JAZ3 were cloned into pSAT-GAL4BD vector, resulting in BD-PIF4 and BD-JAZ3, respectively.
To construct the vectors for the transient LUC reporter assay, the promoter of EXP2 was amplified and cloned into pGreenII 0800-LUC vector to generate EXP2p:LUC. PIF4 was cloned into the pUC18-3HA vector to produce pUC18-PIF4-HA.
To construct the vectors for in vitro protein expression, JAZ3 was cloned into a modified pET11 expression vector (Novagen) to generate His-JAZ3-GFP-MBP by homoeologous recombination (Fang et al. 2019). PIF4 was inserted into the modified pET11 expression vector, in which GFP was replaced by mCherry to generate His-mCherry-PIF4-MBP.
To construct the vectors for in vitro protein expression for EMSA, JAZ3 and PIF4 were cloned into the modified pMAL-c5X-1 vector independently to generate pMAL-JAZ3 and pMAL-PIF4 vectors.
Yeast 2-hybrid assay
The GAD-JAZs and GBD-PIF4-ΔAD constructs were co-transformed into yeast Y2H Gold strain. The yeast clones were suspended in proper sterile water, dropped on the SD/-Trp-Leu and SD/-Trp-Leu-His-Ade dropout plates, and incubated at 28 °C for 3 d. The growth of yeast clones on the SD/-Trp-Leu-His-Ade dropout plates represents an interaction.
LCI assay
The cLUC-PIF4 and JAZs-nLUC constructs were transformed into Agrobacterium tumefaciens strain GV3101. The LCI assay was performed by agroinfiltration in N. benthamiana as previously described (Liu et al. 2010). After 2 d of incubation, the N. benthamiana leaves were cut and sprayed with 2 µM LUC (dissolved in 0.02% Triton X100, v/v). The interactions were measured using a NightSHADE LB 985 imaging system (Berthold Technologies).
Protoplasts transformation and Co-IP assay
Arabidopsis mesophyll protoplasts were isolated from 4-wk-old Col-0 plants as previously described (Yoo et al. 2007). Fifty micrograms of pUC18-JAZ3-HA, pUC18-PIF4-YFP, or pUC18-YFP were co-transformed separately into Arabidopsis protoplasts and incubated in the dark for 16 h. Then, the protoplasts were collected and lysed with 1 mL protoplast IP buffer [25 mM Tris-HCl pH7.5, 150 mM NaCl, 1 mM EDTA, 1% (v/v) Triton X-100, 1× complete protease inhibitor cocktail (Roche) and 50 µM MG132]. Extracts were incubated with 15 µL anti-GFP-agarose beads (LABLEAD, Beijing) at 4 °C for 1 h. The beads were washed 5 times with protoplast washing buffer (25 mM Tris-HCl pH 7.5, 150 mM NaCl, 1 mM EDTA, 0.5% Triton X-100) and boiled in 2× SDS loading buffer at 95 °C for 5 min. The protein samples were analyzed by western blot with anti-GFP (TransGen) and anti-HA (MBL) antibodies.
Immunoblotting
Total plant proteins were extracted with extraction buffer [50 mM Tris-HCl, pH 7.5, 150 mM NaCl, 10 mM MgCl2, 0.1% (v/v) Tween 20, 1 mM PMSF, and 1× complete protease inhibitor cocktail]. The protein concentration was determined using Bradford Assay Reagent (Bio-Rad) and a UV spectrophotometer (OD595). The protein samples were boiled by adding 10× SDS loading buffer at 95 °C for 5 min and fractionated on 8% SDS–PAGE gels. After being transferred to polyvinylidene fluoride membranes, the proteins were immunoblotted with anti-GFP (TransGen, HT801) and anti-Actin (CWBIO, CW0264M) primary antibodies and subsequently with horseradish peroxidase-conjugated secondary antibody (CWBIO, CW0102S). The signals were captured with a Chemiluminescence Imaging System (Biostep).
RNA-seq analysis
Col and JAZ3-GFP seedlings were grown under continuous red light for 5 d or 28 °C for 7 d and harvested for RNA-seq analysis as previously described (Zhang et al. 2017). Each genotype was analyzed 3 times. Genes with more than 1.5-fold change in expression and P < 0.05 were considered to be differentially expressed genes.
Protein expression and purification
The His-JAZ3-GFP -MBP and His-mCherry-PIF4-MBP plasmids were introduced into E. coli stain BL21 (DE3) separately. The recombinant fusion proteins were induced by isopropyl β-D-1-thiogalactopyranoside at 16 °C for 16 h and purified using Ni-nitrilotriacetic acid agarose (Qiagen, 30210) following the manufacturer's instructions.
The pMAL-JAZ3, pMAL-PIF4 vectors and pMAL-c5X-1 plasmids were introduced into E. coli stain BL21 (DE3) separately and induced by isopropyl β-D-1-thiogalactopyranoside at 16 °C for 12 h. These proteins were purified by the Dextrin Sepharose High Performance (Cytiva, 28935597).
In vivo and in vitro phase separation assay
For the in vivo phase separation assay, 35S:JAZ3-GFP, 35S:PIF4-GFP, and 35S:PIF4-mCherry were transformed or co-transformed into N. benthamiana by agroinfiltration. After 2 d of incubation, the N. benthamiana leaves were cut and visualized using a Zeiss LSM 980 confocal microscope. As a nucleus marker, DAPI dye solution was injected into the corresponding N. benthamiana leaves before microscopic observation.
For the in vitro phase separation assay, His-JAZ3-GFP-MBP and His-mCherry-PIF4-MBP proteins were cleaved with TEV protease for 2 h. The proteins were diluted into desired concentrations with Tris-HCl (pH 7.5) and NaCl. His-JAZ3-GFP-MBP and His-mCherry-PIF4-MBP were mixed with 10% (w/v) final concentration of PEG 8000 (Sigma) for droplet assembly. Droplets were visualized in a 384-well microscopy plate (Cellvis) using a Zeiss LSM 980 confocal microscope.
Transient LUC activity assay
Combinations of the effector construct, GAL4p:LUC reporter plasmid, and 35S:GUS internal control were transformed into Arabidopsis protoplasts and incubated in darkness for 16 h. Then, the protoplasts were lysed and resuspended with 100 µL lysis reagent (Promega). Fifteen microliters of protein extract were mixed with 5 µL of LUC assay substrate (Promega), and LUC activity was determined with a Modular Luminescence Kit (Promega). For the GUS assay, 5 µL of protein extract was incubated with 45 µL of GUS assay buffer [50 mM sodium phosphate pH 7.0, 1 mM 4-methylumbelliferyl β-D-glucuronide, 10 mM EDTA, 10 mM β-mercaptoethanol, 0.1% (w/v) sarkosyl, 0.1% (v/v) Triton X-100] at 37 °C for 30 min, and the reaction was stopped by the addition of 950 mL of 0.2 M Na2CO3. The fluorescence was measured using a UV Fluorescence Optical Kit (Promega). Relative LUC activity is calculated by the ratio of LUC/GUS.
Transient LUC reporter assay
Five micrograms of the EXP2p:LUC reporter plasmid containing a REN expression cassette was co-transformed with 5 mg of JAZ3-3HA, and/or PIF4-3HA effector plasmids into Arabidopsis protoplasts. After 16 h of incubation in the dark, protoplasts were lysed and the activities of LUC and REN were measured with a Modulus Luminometer/Fluorometer (Promega).
FRAP assay
In vivo experiment: FRAP of 35S:JAZ3-GFP and 35S:PIF4-GFP condensates in N. benthamiana leaves was performed using Zeiss LSM980 confocal microscope. A region of 35S:JAZ3-GFP or 35S:PIF4-GFP condensate was bleached using a 488 nm laser pulse (50% intensity). Recovery was recorded for every 2.5 s for a total of 70 s after bleaching.
In vitro experiments: In vitro FRAP experiments were carried out with samples in 384-well microscopy plates using a Zeiss LSM980 confocal microscope. A small area of the droplet was bleached with a 488 nm laser pulse (561 nm for the mCherry-PIF4). Recovery was recorded for every 2.5 s after bleaching. For all FRAP experiments, recoveries were measured as the fluorescence intensity of photobleached area normalized to the intensity of the same area before bleaching.
Electrophoretic mobility shift assay
The synthetic complementing oligonucleotides of EXP2 promoter were annealed and biotinylated with the EMSA Probe Biotin Labeling Kit (Beyotime, GS008). EMSA experiment was performed using the LightShift EMSA Optimization and Control Kit (Pierce, 20148X) according to the manufacturer's protocol. Briefly, 5 µg MBP, PIF4-GST and JAZ3-MBP were incubated separately or together with the biotin-labeled probe for 20 min at room temperature. The reaction products were separated on 6% nondenaturing polyacrylamide gel and transferred to the nylon membrane (Merck Millipore, INYC00010). The membrane was cross-linked with UV light, soaked with the Chemiluminescent Nucleic Acid Detection Module (Pierce, 89880), and captured with a Chemiluminescence Imaging System (Biostep). The oligonucleotide sequence is shown in Supplementary Table S1.
RT-qPCR
Total RNA was isolated using the Universal Plant Total RNA Extraction Kit (BioTeke). cDNA was synthesized using oligo (dT)18 primer and reverse transcriptase (Invitrogen). qPCR was performed using SYBR Premix ExTaq Kit (Takara) in a LightCycler 480 (Roche) according to the manufacturer’s instructions. Three technical replicates were carried out, and the expression levels were normalized to those of the Actin gene. Each assay was performed at least 3 times with similar results, and 1 representative result is shown.
Accession numbers
Sequence data from this article can be found in the GenBank/EMBL data libraries under accession numbers JAZ1 (AT1G19180), JAZ2 (AT1G74950), JAZ3 (AT3G17860), JAZ4 (AT1G48500), JAZ5 (AT1G17380), JAZ6 (AT1G72450), JAZ7 (AT2G34600), JAZ8 (AT1G30135), JAZ9 (AT1G70700), JAZ10 (AT5G13220), JAZ11 (AT3G43440), JAZ12 (AT5G20900), JAZ13 (AT3G22275), PIF4 (AT2G43010), IAA6 (AT1G52830), IAA19 (AT3G15540), IAA29 (AT4G32280), EXP2 (AT5G05290), EXP8 (AT2G40610), YUC8 (AT4G28720), and Actin (AT3G18780).
Acknowledgments
We thank Dr Xiaofeng Fang (Tsinghua University) for providing the modified pET11 expression vector.
Author contributions
J.H. and N.G. performed most of the experiments; Y.Y. performed the EMSA experiment; Y.D. and Q.G. contributed to data analysis and material preparation; J.H. and R.L. designed the experiments and wrote the paper.
Supplementary data
The following materials are available in the online version of this article.
Supplementary Figure S1. Confirmation of JAZ3-GFP protein.
Supplementary Figure S2. Overexpression of JAZ3 confers large cotyledon angles and cotyledon areas.
Supplementary Figure S3. Overexpression of JAZ3 confers short hypocotyls under weak white light.
Supplementary Figure S4. Overexpression of JAZ3-cFlag confers short hypocotyls under red light.
Supplementary Figure S5. Expression patterns of JAZ3.
Supplementary Figure S6. JAZ proteins interact with PIF4.
Supplementary Figure S7. JAZ3 undergoes phase separation in vitro.
Supplementary Figure S8. PIF4 cannot undergo phase separation in vivo and in vitro.
Supplementary Figure S9. Effect of salt ion concentration on the phase separation of JAZ3 and PIF4.
Supplementary Figure S10. GO analysis of JAZ3-regulated genes under red light.
Supplementary Figure S11. GO analysis of JAZ3-regulated genes under 28 °C.
Supplementary Figure S12. Confirmation of JAZ3-GFP and PIF4-GFP protein accumulation.
Supplementary Table S1. Primer sequences used in this study.
Supplementary Data Set S1. Genes regulated by JAZ3 under red light and 28 °C conditions.
Funding
This work was supported by grants from the National Key Research and Development Program of China (2022YFF1001701) and the National Natural Science Foundation of China (32270262).
Dive Curated Terms
The following phenotypic, genotypic, and functional terms are of significance to the work described in this paper:
References
Author notes
Junling Huai and Nan Gao contributed equally to this work.
The author responsible for distribution of materials integral to the findings presented in this article in accordance with the policy described in the Instructions for Authors (https://dbpia.nl.go.kr/plphys/pages/General-Instructions) is: Rongcheng Lin ([email protected]).
Conflict of interest statement. The authors declare no conflict of interest.