-
PDF
- Split View
-
Views
-
Cite
Cite
Lixia Pan, Cassio Flavio Fonseca de Lima, Lam Dai Vu, Brigitte van de Cotte, Nancy De Winne, Kris Gevaert, Geert De Jaeger, Ive De Smet, Heterodimerization domains in MAP4 KINASEs determine subcellular localization and activity in Arabidopsis, Plant Physiology, Volume 195, Issue 3, July 2024, Pages 1807–1817, https://doi.org/10.1093/plphys/kiae176
- Share Icon Share
Abstract
Signal transduction relies largely on the activity of kinases and phosphatases that control protein phosphorylation. However, we still know very little about phosphorylation-mediated signaling networks. Plant MITOGEN-ACTIVATED PROTEIN KINASE KINASE KINASE KINASEs (MAP4Ks) have recently gained more attention, given their role in a wide range of processes, including developmental processes and stress signaling. We analyzed MAP4K expression patterns and mapped protein–MAP4K interactions in Arabidopsis (Arabidopsis thaliana), revealing extensive coexpression and heterodimerization. This heterodimerization is regulated by the C-terminal, intrinsically disordered half of the MAP4K, and specifically by the coiled coil motif. The ability to heterodimerize is required for proper activity and localization of the MAP4Ks. Taken together, our results identify MAP4K-interacting proteins and emphasize the functional importance of MAP4K heterodimerization. Furthermore, we identified MAP4K4/TARGET OF TEMPERATURE3 (TOT3) and MAP4K5/TOT3-INTERACTING PROTEIN 5 (TOI5) as key regulators of the transition from cell division to elongation zones in the primary root tip.
Introduction
Dynamic and reversible phosphorylation-mediated signaling requires kinases and phosphatases. The evolutionarily conserved MITOGEN-ACTIVATED PROTEIN KINASE (MAPK) cascades play pivotal roles in transducing extracellular stimuli into intracellular responses and typically involve 3 key modules: MAP KINASE KINASE KINASE (MAP3K), MAP KINASE KINASE (MAP2K), and MAP KINASE (MAPK) (Zhang and Zhang 2022). However, MAP4Ks have been positioned upstream of these classical MAP3K–MAP2K–MAPK cascades (Dan et al. 2001; Chuang et al. 2016). In the yeast (Saccharomyces cerevisiae), the kinase Sterile 20 protein (Ste20p) was identified as a putative regulator of a MAP kinase cascade in the mating pathway (Leberer et al. 1992). The phosphorylation of MAP3K Ste11 by Ste20p indicates that Ste20p acts as a MAP4K (Drogen et al. 2000). Based on the phylogenetic analysis of conserved domain and structure, the Ste20 family could be further divided into 2 subfamilies in mammals, fruit fly (Drosophila melanogaster), Caenorhabditis elegans, and other organisms: p21-activated kinase and germinal center kinase subfamilies (Dan et al. 2001). The structure of the kinase domain is conserved among p21-activated kinases (PAKs) and germinal center kinases (GCKs), and the kinase domain contains a MAP4K signature sequence, namely GTPyWMAPEv (Dan et al. 2001). However, the location of the kinase domain is at the C-terminus in the PAKs and at the N-terminus in the GCKs (Dan et al. 2001). Seven MAP4Ks have been reported in humans, and these MAP4Ks are essential for the regulation of cell apoptosis, cell survival, cell autophagy, and cell migration (Chuang et al. 2016). Moreover, human MAP4K family members are also core components of the evolutionarily conserved Hippo signaling pathway, which is important for tissue homeostasis, and its dysregulation contributes to tumorigenesis (Meng et al. 2015).
MAP4Ks are also highly conserved in plants, and in the green lineage, 3 major clades can be distinguished (Pan et al. 2021). In plants, the MAP4Ks have recently gained more attention, given their role in a wide range of processes, including developmental processes and stress signaling (Pan and De Smet 2020; Vu et al. 2021; Zhang et al. 2021b). The maize (Zea mays) MAIZE ATYPICAL RECEPTOR KINASE (MARK)-INTERACTING KINASE (MIK) and the potato (Solanum chacoense) MAP4K1 are important during embryogenesis (Pan and De Smet 2020). BLUE LIGHT SIGNALING1 (BLUS1)/MAP4K10 is a substrate of the blue light receptor phototropins (PHOT1 and PHOT2) and regulates stomatal opening (Schnabel et al. 2018). Both SERINE/THREONINE KINASE 1 (SIK1)/MAP4K3 and TARGET OF TEMPERATURE3 (TOT3)/MAP4K4 interact with the BOTRYTIS-INDUCED KINASE 1 (BIK1) in immunity (Zhang et al. 2018; Jiang et al. 2019). SIK1/MAP4K3 is also essential for cell proliferation, expansion, and root polarity (Xiong et al. 2016; Zhang et al. 2021b). TOT3/MAP4K4, TOT3-INTERACTING PROTEIN 5 (TOI5)/MAP4K5, and TOI4/MAP4K6 are involved in temperature-mediated growth (Vu et al. 2021).
While the human MAP4K interactome revealed STRN4 as a common interactor of MAP4Ks and as a key STRIPAK complex component in Hippo pathway regulation (Seo et al. 2020), very little is known about the MAP4K protein–protein interaction network in plants. MAP4Ks display a long intrinsically disordered C-terminal half following the kinase domain (Chuang et al. 2016; Pan et al. 2021), but its role has not yet been explored. Furthermore, in humans, MAP4Ks share a conserved C-terminal citron-homology domain (CNH) that is essential for protein–protein interaction, but this domain is absent in plants (Chuang et al. 2016; Pan et al. 2021). However, a conserved coiled coil motif is located at a similar position of the CNH domain in the green lineage, and this motif is predicted to be involved in protein localization and protein–protein interaction (Pan et al. 2021). Here, we present the interactome of a subset of plant MAP4Ks and provide experimental evidence for the role of the C-terminal half of the protein and especially the coiled coil motif, in controlling protein–protein interactions and MAP4K subcellular localization.
Results and discussion
Arabidopsis MAP4Ks are expressed in unique and overlapping patterns
Previously, several Arabidopsis MAP4Ks were shown to play a role in thermomorphogenesis, stomatal opening, cell proliferation and expansion, root polarity, and innate immunity (Pan and De Smet 2020; Hosotani et al. 2021; Vu et al. 2021; Zhang et al. 2021a). However, little is known about the diverse developmental roles of MAP4Ks. In view of the increase in members throughout the plant evolution of subclade III (Pan et al. 2021), which is possibly associated with several newly arisen and distinct functions for these MAP4Ks, we focused on MAP4K4 to MAP4K9 [referred to as MAP4K(4-9)] (Fig. 1A). To explore in which developmental processes MAP4K(4-9) could be involved, we analyzed their expression patterns in a wide range of organs using pMAP4K(4-9)::GUS fusions. Detailed analyses of diverse plant organs revealed distinct and overlapping expression patterns in the root, shoot, and flowers (Fig. 1, B and C and Supplementary Fig. S1). Apart from MAP4K9, several MAP4Ks are coexpressed in various organs and tissues (Fig. 1, B and C). This is in agreement with previously observed overlapping roles of, e.g. TOT3/MAP4K4, TOI4/MAP4K6, and TOI5/MAP4K5 in (warm temperature–mediated) hypocotyl growth (Vu et al. 2021). Similarly, the expression patterns in the root suggest (partially) overlapping roles for TOT3 and TOI5 and for TOT3 and MAP4K8 in the primary root tip (Fig. 1D). Indeed, a tot3-2 toi5-2 double mutant displayed a shorter primary root compared with the wild-type control and single mutants (Fig. 1, E and F). Primary root length is determined by cell division and elongation (Beemster and Baskin 1998). Analyzing meristem size and cell number revealed that the tot3-2 toi5-2 double mutant has a significantly shorter primary root meristem with fewer cells than the wild-type control (Fig. 1, G to I). The partially overlapping TOT3 and TOI5 expression patterns in the primary root tip and the tot3-2 toi5-2 phenotype support that TOT3 and TOI5 are key regulators of the cell division to elongation transition.

Expression patterns of MAP4Ks underpinning their function. A) Cladogram of the MAP4K family in Arabidopsis. MPK3 was used as an outgroup. Other protein names are mentioned between parentheses. B) Summary of expression patterns of MAP4K(4-9). See Supplementary Fig. S1 for representative images. C)MAP4K coexpression network. The thickness of edges reflects the number of coexpressed organs or tissues. D) Expression pattern of MAP4K(4-9) in the primary root tip. Scale bars, 20 µm. E to I) MAP4K4 and MAP4K5 are key regulators of the cell division to elongation transitions. Phenotypic assay 5 d after germination. Col-0, tot3-2 (map4k4), toi5-2 (map4k5), and tot3-2 toi5-2 (map4k4 map4k5) seedlings grew under long day conditions at 21 °C. Representative images of the primary root (scale bars, 10 mm) E) and representative confocal microscopy images of the primary root meristem zone (scale bar, 50 µm) F). Seedlings in F) were stained with propidium iodide (PI) before imaging. White arrows indicate the end of the meristem zone. Quantification for primary root length G), the number of cortex cells in the meristem H), and meristem zone length I). Box plots show a median with Tukey-based whiskers and outliers. Center line, median; box limits, upper and lower quartiles; Tukey whiskers, (the 75th percentile plus 1.5× interquartile range, the 25th percentile minus 1.5× interquartile range, the interquartile range is the difference between the 25th and 75th percentiles); points, outliers. The number of individually measured seedlings (n) is indicated above the x-axis. Letters indicate significant differences based on 1-way ANOVA and Tukey's test (P < 0.01). The P-value for the genotypes is shown at the top.
Arabidopsis MAP4K interactome identifies distinct and common MAP4K-interacting proteins
To pinpoint if and how MAP4K(4-9) indeed control distinct and overlapping processes, we focused on their interacting proteins. To obtain a comprehensive understanding of the MAP4K(4-9) protein–protein interaction network, we established Arabidopsis cells stably expressing each MAP4K family member fused to an NGSrhino or CGSrhino tag. These MAP4K-expressing cells were then subjected to affinity purification coupled with MS (AP-MS) to identify the associated proteins within each isolated protein complex. A complete list of the peptides and proteins identified in this study can be found in Supplementary Table S1. To refine the MAP4K AP-MS data, we focused on high-confidence interacting proteins (see “Materials and methods” section). The combined AP network embeds 179 interactions among 102 proteins, revealing distinct and common interactors (41.3% of the interactions are shared between at least 2 MAP4Ks; Fig. 2A and Supplementary Table S2). Because the stable cells collected for the AP-MS analysis were grown under normal culture conditions, the identified high-confidence MAP4K-interacting proteins for each MAP4K(4-9) Clade III member could be considered as the basal-state interactome of the Arabidopsis MAP4K kinase family. Gene Ontology (GO) and (predicted) localization analyses of the high-confidence MAP4K-interacting proteins suggested that they were widely distributed in the cells with different sub-cellular localization (Fig. 2B) and were involved in diverse molecular functions and biological processes (Fig. 2C). The interactome revealed not only distinct MAP4K(4-9)-interacting proteins, but also—in line with the coexpression analysis (Fig. 1B)—several MAP4K(4-9)-interacting proteins that are shared between 2 or more MAP4Ks (Fig. 2A). Surprisingly, while we only detected limited overlap in the expression pattern of MAP4K9 and MAP4K(4-8), the interactome revealed a high cointeraction between proteins interacting with MAP4K9 and MAP4K(4-8).
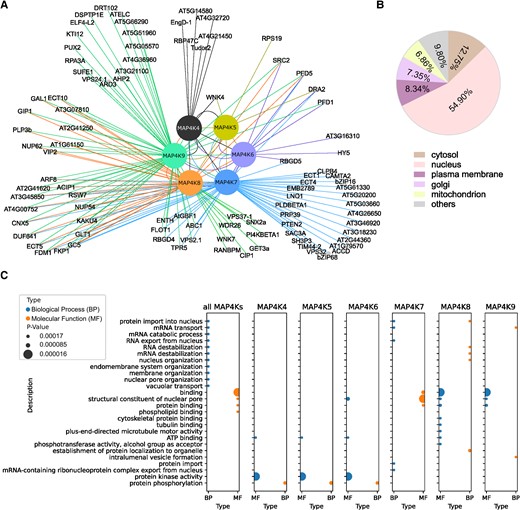
AP-MS analysis of the MAP4K protein interaction network. A) Visualization of the MAP4Ks interactome derived from AP-MS data. B) Cellular localization of MAP4K interactors based on Arabidopsis protein subcellular location data from SUBA5. C) GO enrichment of MAP4K interactors in biological process and molecular function.
Arabidopsis MAP4Ks form heterodimers
Intriguingly, the MAP4K(4-9) basal-state interactome revealed—in line with previous research in nonplant organisms (Glantschnig et al. 2002; Jin et al. 2012; Zhou et al. 2018)—frequent heterodimer formation among the MAP4K family members (Fig. 3A). Co-immunoprecipitation (co-IP) experiments (Supplementary Fig. S2A), yeast 2-hybrid (Y2H) experiments (Supplementary Fig. S2B), and bimolecular fluorescence complementation (BiFC) assays (Supplementary Fig. S2C) independently confirmed interactions between several of the MAP4Ks (Fig. 3A). Based on MAP4K(4-9) interactions, expression patterns, and functional redundancies (Vu et al. 2021; Fig. 1), it appears as if particular heterodimer combinations (or potentially higher order complexes) are associated with specific processes.
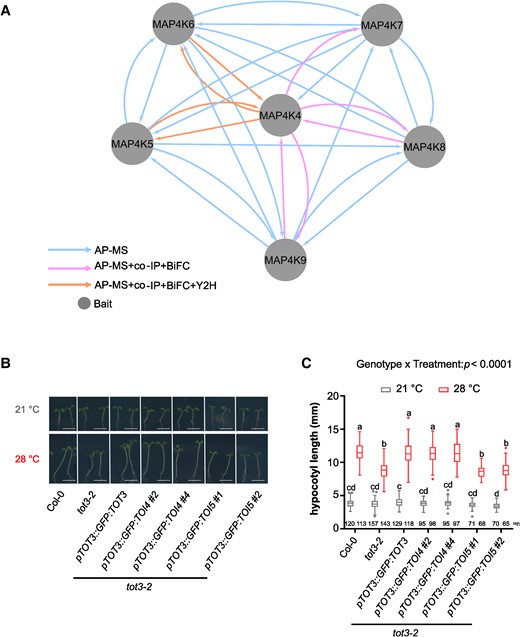
Arabidopsis MAP4Ks form heterodimers. A) MAP4K(4-9) heterodimer interactions. Integrated node and edge attributes are shown next to the network. Hypocotyl length for indicated lines at 21 and 28 °C in short-day conditions. Representative pictures B) and hypocotyl length quantification C). Scale bar, 5 mm. Box plots show median with Tukey-based whiskers and outliers. Center line, median; box limits, upper and lower quartiles; Tukey whiskers (the 75th percentile plus 1.5× interquartile range, the 25th percentile minus 1.5× interquartile range, the interquartile range is the difference between the 25th and 75th percentiles); points, outliers. The number of individually measured seedlings (n) from 4 independent replicate experiments is indicated above the x-axis. Letters indicate significant differences based on 2-way ANOVA and Tukey's test (P < 0.01) (C). P-value is shown at the top.
Arabidopsis TOT3 and TOI4 are functionally interchangeable in thermomorphogenesis
Using TOT3, TOI4, and TOI5 as representative heterodimerizing MAP4Ks, we explored to what extent these MAP4Ks are interchangeable. Previously, we showed that tot3-2 displayed a shorter hypocotyl at 28 °C than Col-0 (Vu et al. 2021). By expressing TOI5 or TOI4 under the TOT3 promoter, we assessed the ability of TOI5 or TOI4 to rescue the tot3-2 hypocotyl phenotype at 28 °C (Supplementary Fig. S3). A pTOT3::GFP:TOT3 construct complemented the tot3-2 hypocotyl phenotype at 28 °C (Fig. 3, B and C; Vu et al. 2021). Similarly, a pTOT3::GFP:TOI4 construct is able to rescue the tot3-2 hypocotyl phenotype at 28 °C (Fig. 3, B and C). However, the pTOT3::GFP:TOI5 construct did not rescue the tot3-2 hypocotyl phenotype at 28 °C (Fig. 3, B and C). This suggested that some MAP4Ks are interchangeable, at least in the context of warm temperature–mediated hypocotyl growth, but that TOI5 requires a functional TOT3 for its activity.
C-terminal half of Arabidopsis TOT3 is required for interaction and localization
The C-terminal half from the end of the kinase domain to the utmost C-terminal amino acid of MAP4Ks is largely intrinsically disordered (Pan et al. 2021). Since intrinsically disordered regions are involved in protein–protein interactions and protein localization (Lee et al. 2017; Cornish et al. 2020; Trivedi and Nagarajaram 2022; Kibar and Vingron 2023), we explored to what extent the MAP4K C-terminal half is necessary for heterodimerization and localization. For this, we again used TOT3–TOI5 as a representative pair. Using a Y2H assay and co-IP, we showed that removing the C-terminal half abolishes the interaction between TOT31-296 and TOI5 (Fig. 4, A to C). In addition, the TOT3 protein lacking the C-terminal half also displayed a different localization, namely GFP:TOT31-305 accumulated more in the nucleus (Fig. 4D). While a pTOT3::GFP:TOT3 construct complemented the tot3-2 hypocotyl phenotype at 28 °C (Vu et al. 2021), a TOT3 protein variant that lacked the C-terminal half (3xHA:TOT31-308) could not rescue the tot3-2 short hypocotyl phenotype at 28 °C (Fig. 4, E and F and Supplementary Fig. S3). Previously, we identified a conserved C-terminal coiled-coil motif (TOT3623-672) in these MAP4Ks (Fig. 4A; Pan et al. 2021), which could be involved in protein–protein interactions and protein localization (Mier et al. 2017; Georgoulia and Bjelic 2021). Here, we explored to what extent the TOT3 C-terminal coiled-coil motif is indeed necessary for TOT3 heterodimerization and localization. Using a Y2H assay and co-IP, we showed that removing the coiled-coil motif abolishes the interaction between TOT31-610 and TOI5 (Fig. 4, B and C). In addition, the TOT3 protein lacking the C-terminal coiled-coil motif displayed a different localization, namely GFP:TOT31-610 accumulated more in the nucleus (Fig. 4D). Furthermore, a TOT3 protein variant that lacked the C-terminal coiled-coil motif (GFP:TOT31-610) could not rescue the tot3-2 short hypocotyl phenotype at 28 °C (Fig. 4, E and F and Supplementary Fig. S3). In conclusion, the C-terminal half and the C-terminal coiled-coil motif are important for TOT3 function, specifically protein–protein interactions and localization.
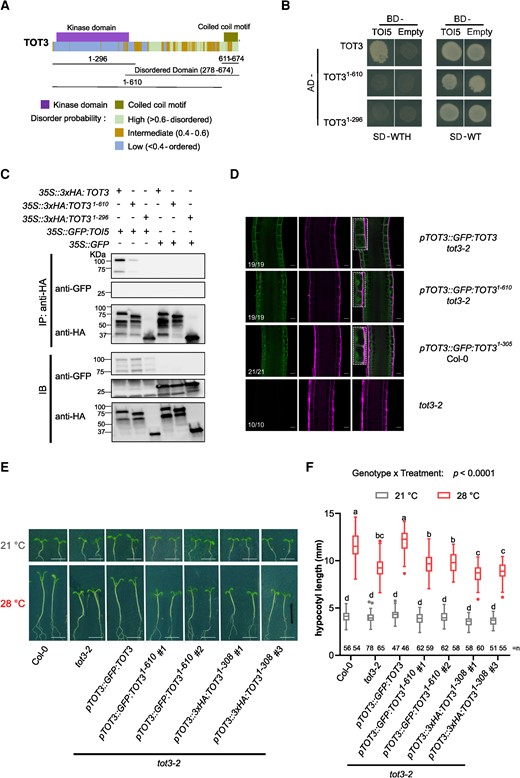
The C-terminal half and coiled coil domain are essential for TOT3 functions. A) Schematic of TOT3 protein domains and motifs. B) Y2H assay between TOT3 lacking the C-terminus (1-296) and the coiled coil motif (1-610) and TOI5. The experiment was done in 3 replicates with same results. C) Co-IP between TOT3 lacking the C-terminus (1-296) and the coiled coil motif (1-610) and TOI5. The experiment was done in 2 replicates with similar results. D) Localization of TOT3 and TOT3 lacking the C-terminus (1-296) or the coiled coil motif (1-610) fused to GFP in the primary root meristem and transition zone. The inset shows TOT3 localization in 2 cells. The numbers indicate the number of seedlings corresponding to the representative localization out of total number of seedlings analyzed. Scale bar, 20 µm. Green, GFP; magenta, propidium iodide. Representative pictures E) and hypocotyl length quantification for truncated TOT3 constructs in tot3-2 at 21 and 28 °C in short-day conditions F). Scale bar, 5 mm. Box plots show median with Tukey-based whiskers and outliers. Center line, median; box limits, upper and lower quartiles; Tukey whiskers (1.5× interquartile range, the 25th percentile minus 1.5× interquartile range, the interquartile range is the difference between the 25th and 75th percentiles); points, outliers. The number of individually measured seedlings (n) from 2 independent replicate experiments is indicated above the x-axis. Letters indicate significant differences based on 2-way ANOVA and Tukey's test (P < 0.01) F). P-value is shown at the top. -WT, without Leu and Trp; -WTH, without Leu, Trp and His; IP, immunoprecipitation; IB, immunoblot.
Conclusions
Previously, we identified the membrane-associated protein kinase MAP4K4/TOT3 as a key player in plant responses to warm temperature (Vu et al. 2021). We showed that MAP4K4/TOT3 interacts with related MAP4Ks (MAP4K6/TOI4 and MAP4K5/TOI5) and that these related kinases also play a role in thermomorphogenesis (Vu et al. 2021). In this study, we provide valuable insight into the functional roles and interactions of MAP4K proteins within the context of plant cell biology and thermomorphogenesis. One notable aspect is the identification of distinct and overlapping gene expression patterns of MAP4Ks in different organs, tissues, and cells. This implies that these MAP4K proteins, likely as heterodimers, may be involved in multiple biological processes. For instance, we demonstrated that MAP4K4/TOT3 and MAP4K6/TOI4 are exchangeable in thermomorphogenesis. Since MAP4K4/TOT3, e.g., also regulates plant innate immunity (Jiang et al. 2019), it will be intriguing to explore whether similar heterodimers also function in that process. The expression of both MAP4K7 and MAP4K8 in stomata and pollen raises intriguing questions about their potential contributions to stomata development and fertilization. Here, we also pinpointed a role for the MAP4K4/TOT3–MAP4K5/TOI5 pair in primary root growth. Several hormone and nutrient signaling pathways play a key role in the cell division to elongation transition in the primary root (Zluhan-Martínez et al. 2021; Jia et al. 2022), and it remains to be analyzed if and how TOT3 interacts with these pathways. We previously showed that TOT3 impinges on brassinosteroid-mediated hypocotyl growth control under warm temperature (Vu et al. 2021), and possibly TOT3 has a similar role in the primary root. In addition, the MAP4K protein–protein interaction landscape identified ∼100 MAP4K interactors, which further supports their involvement in a wide range of cellular events. This opens up avenues for investigating the diverse roles of MAP4Ks in various signaling pathways, including plant innate immunity and root architecture.
The role of the intrinsically disordered C-terminal half and of the coiled coil domain in Arabidopsis MAP4K heterodimer formation and localization can also provide valuable insight into these cellular processes in other organisms. Indeed, the coiled coil domain of MAP4K4/TOT3 is vital for TOT3 interactions with other MAP4Ks to control thermomorphogenesis. It is, however, still an open question how the MAP4K coiled-coil domain impacts the larger MAP4K interactome. Our phosphoproteome data on MAP4K4/TOT3 indicated that this intrinsically disordered C-terminal half is also subjected to intense phosphorylation, impacting the activity of the protein (Willems et al. 2019; Vu et al. 2021; De Smet et al. 2023; Supplementary Fig. S4). How phosphorylation impacts MAP4K heterodimer formation and localization will be an area of future study.
Taken together, this study advances our understanding of MAP4K proteins and their roles in various biological processes and underscores the importance of exploring the interactions and functions of these proteins in different cellular contexts.
Materials and methods
Plant materials and growth conditions
All Arabidopsis (Arabidopsis thaliana) plants used in this study were in the Col-0 reference accession genetic background and referred to as wild type. The following Arabidopsis mutants were used in this study: tot3-2, toi5-2, and double mutant tot3-2 toi5-2 (Vu et al. 2021). Seeds were surface-sterilized by 70% (v/v) ethanol, plated on half-strength MS medium with 1% (w/v) agar (per liter: 2.15 g of MS salts, 0.1 g of myo-inositol, 0.5 g of MES, 10 g of sucrose, and 8 g of plant tissue culture agar; pH 5.7). Seeds were stratified at 4 °C for 2 d in the dark and then moved to 21 °C under continuous light for germination for 48 h. For GUS staining of young seedlings and western blot, seedlings grew in the continuous light growth chamber at 21 °C for 5 d after germination. For GUS staining of flowers, seedlings grew on half-strength MS medium for 2 wk and were then transferred to the soil under long-day conditions (16 h day/8 h night) at 21 °C for another 4 wk. For primary root phenotyping, germinated seedlings were grown under long-day conditions (16 h day/8 h night) at 21 °C for 5 d. For hypocotyl phenotyping, germinated seedlings were grown under short-day conditions (8 h day/16 h night) at 21 °C or at 28 °C for 7 d. Images were taken with a Canon scanner, and measurements were recorded using Fiji ImageJ (https://imagej.net/Fiji).
Generation of constructs and transgenic lines
PCR was performed using iProof High-Fidelity DNA Polymerase or Q5 High-Fidelity polymerase according to the manufacturer protocol with indicated primer pairs (Supplementary Table S3). The CDSs of MAP4K7 (1785 bp), MAP4K8 (4721 bp), and MAP4K9 (2878 bp) were synthesized (Twist Bioscience, USA). The GoldenGate system (Lampropoulos et al. 2013) and Gateway cloning system (Hartley et al. 2000; Karimi et al. 2007) were used to generate the constructs in this study (Supplementary Table S4). Plant constructs were transformed into Agrobacterium tumefaciens C58C1 using the freeze–thaw method (Weigel and Glazebrook 2006). Plant transformation was performed using the floral dip method (Clough and Bent 1998).
Phylogenetic tree of Arabidopsis MAP4Ks
MITOGEN-ACTIVATED PROTEIN KINASE 3 (MPK3) was added as an outgroup of MAP4Ks. Alignment of all Arabidopsis MAP4K and MPK3 protein sequences was performed in CLC Main Workbench 20.03 (CLC Bio-Qiagen, Aarhus, Denmark) with default setup. Subsequently, the phylogenetic tree was visualized using the Interactive Tree Of Live version 4 (iTOL; Letunic and Bork 2019).
Histochemical GUS staining
Seedlings (7 days after germination (DAG)) or flowers (6-week-old plants) were fixated in cold 90% (v/v) acetone for 1 h and then rinsed twice with NT buffer (100 mM Tris/50 mM NaCl). Then, samples were transferred to a GUS solution (500 µL 100 mM K3[Fe(CN)6] + 600 µL 500 µg/mL X-Gluc + 28.8 mL NT-buffer) and incubated at 37 °C in darkness until sufficiently strong staining was observed. The plants were rinsed with NT buffer to remove the staining agents and mounted on glass slides in 80% (v/v) lactic acid. Finally, pictures were taken using an Olympus BX51 microscope.
Affinity purification analysis
AP analysis for MAP4K4/TOT3 was described previously (Vu et al. 2021). For the other MAP4Ks, the Gateway system was used to recombine the MAP4K5 CDS (2178 bp), MAP4K6 CDS (2136 bp), MAP4K8 genomic DNA (4721 bp), and MAP4K9 genomic DNA (2878 bp) into the pGNGSrhino vector (Invitrogen). MAP4K7 CDS was recombined into both pGNGSrhino and pGCGSrhino vectors separately. The constructs were transformed into A. thaliana PSB-D cells (Van Leene et al. 2015). Three GSrhino-based pull downs on Arabidopsis cell suspension cultures expressing each of the GSrhino-tagged baits were performed as described (Van Leene et al. 2022) using 1% digitonin in extraction buffer and 0.2% digitonin in wash buffer. On-bead digested samples were analyzed on a Q Exactive (ThermoFisher Scientific), and copurified proteins were identified with Mascot (Matrix Science) using standard procedures (Van Leene et al. 2015). The identified protein list was filtered versus a large dataset of AP-MS experiments, similarly as described before (Van Leene et al. 2015; Van Leene et al. 2022). The baits in the large AP-MS dataset are functionally grouped into baitgroups, and baits related to the bait of interest are removed from this large dataset before comparison. For each identified protein, a Normalized Spectral Abundance Factor (NSAF) is calculated. The Ln-transformed mean NSAF of all proteins identified are compared with the Ln-transformed mean NSAF of the same protein in the large dataset by a 2-tailed t-test. Proteins that comply with the following criteria are considered significantly enriched vs. the large dataset and are retained in the Filtered set: (i) 2-peptide identifications present in at least 2 of 3 replicates are significantly enriched with a mean NSAF ratio ≥ 10 AND a -Log10(P-value) ≥ 10 or with a mean NSAF ratio ≥20 AND a -Log10(P-value) ≥8 and (ii) 1-peptide identifications present in at least 3 replicates, which are detected in at least one replicate with 2 peptides, are significantly enriched with a mean NSAF ratio ≥20 AND a -Log10(P-value) ≥50.
GO analysis
GO enrichment analyses were performed using Dicots PLAZA 5.0 workbench (Van Bel et al. 2022). All detected putative MAP4K interactors were enriched for GO categories using the whole species as the background model. Significance settings were set at a P-value cut off <0.05, and Bonferroni correction for multiple testing was used. Cellular localization of all interactors was determined through SUBA5 (https://suba.live/).
Statistical analyses
Statistical analyses were performed by GraphPad Prism 8.0.1 software for Windows (Boston, MA, USA). We performed Tukey's 1-way ANOVA for multiple comparisons for primary root length data. For hypocotyl length data, we performed Tukey's 2-way ANOVA for multiple comparisons. Details on the number of biological replicates and samples are included in the figure legends.
Nicotiana benthamiana infiltration
For transient coagroinfiltration of Nicotiana benthamiana leaves, Agrobacteria containing the indicated constructs and P19 were grown in 5 mL LB supplemented with appropriate antibiotics at 28 °C for 1 to 2 d. Then, 500 μL was inoculated in 10-mL LB supplemented with 10 mM MES pH 5.6, 10 µM acetosyringone, as well as the antibiotics and grown at 28 °C overnight. The pellet was spun down and resuspended with infiltration buffer (10 mM MgCl2, 10 mM MES [pH 5.6], 100 µM acetosyringone) to a final OD600 of 1.0. Equal volumes of 3 agrobacteria with selected constructs were mixed well, and the mix was infiltrated in 5- to 6-wk-old N. benthamiana leaves using a syringe. The signal was checked by confocal microscopy after 72 h to make sure the protein expressed well.
Co-immunoprecipitation
Total proteins were extracted with buffer containing 150 mM Tris·HCl, pH 7.5, 150 mM NaCl, 10% (v/v) glycerol, 100 mM EDTA, 1 mM sodium molybdate, 1 mM NaF, 10 mM DTT, 1% (v/v) NP-40, 1 mM phenylmethylsulfonyl fluoride (PMSF), and EDTA-free protease inhibitor mixture complete (Roche). The protein concentration was measured using the Qubit Protein Assay Kit (Qubit Protein Assay Kit), and an equal amount of total protein was used for immunoprecipitation. Twenty-five microliters of preequilibrated HA-Trap_MA beads (Thermo Fisher) was prewashed 3 times with 700 μL wash buffer (20 mM Tris·HCl, pH 7.5, 150 mM NaCl, and 0.5% [v/v] NP-40). The protein homogenates were incubated with HA-Trap_MA beads and rotated for 2 h at 4 °C to maximize protein binding. Subsequently, the solution was removed, and the beads were washed 3 times with wash buffer (20 mM Tris-HCl pH 7.5, 150 mM NaCl, and 0.5% [v/v] NP-40). Finally, 1× sample buffer (Bio-Rad) was added to elute protein from the beads.
Western blot
The method for protein extraction was as described for co-IP. Protein samples were heated at 70 °C for 10 min and then separated on 4% to 20% SDS-PAGE stain-free protein gel (Bio-Rad Laboratories, Inc., USA), followed by transferring onto a Trans-Blot Turbo Mini PVDF Transfer Packs (Bio-Rad Laboratories, Inc., USA). For blocking and antibody dilutions, 5% (w/v) milk powder in Tris-buffered saline with 0.1% Tween® 20 (TBST) solution (20 mM Tris-HCl, 150 mM NaCl, 0.1% [v/v] Tween 20, and adjusted PH to 7.5 with HCl) was used. For protein detection, the following antibodies were used: monoclonal anti-GFP horseradish peroxidase coupled (1:5,000; Miltenyi Biotech) and anti-HA (1:5000; Abcam).
Bimolecular fluorescence complementation
The 2 in 1 BiFC analysis were performed according to the protocol described previously (Mehlhorn et al. 2018). For this, constructs containing 2 gene sequences of interest were introduced into the pBiFCt-2in1-NN vector by Gateway system (Supplementary Table S4). Constructs were transferred to A. tumefaciens C58 cells for N. benthamiana infiltration. N. benthamiana leaf infiltration followed the protocol described in the “Nicotiana benthamiana infiltration” section. After 3 d, leaf disks were collected for confocal imaging using a Leica SP8 confocal microscope with white light laser. Images were captured with a hybrid detector (HyD) at 488 nm (43% intensity) laser excitation and 500 to 540 nm with gain 94.2 for YELLOW FLUORESCENT PROTEIN (YFP) and 561 nm (6.7% intensity) laser excitation and 590 to 640 nm with gain 86.9 for RED FLUORESCENT PROTEIN (RFP). Gating technology with lifetime between 0.3 and 6 ns was applied for auto-fluorescence removal.
Y2H assay
Y2H analysis was performed using the GAL4 system. The bait protein was expressed as a fusion to the DNA binding domain (BD), which is in pGBT9 gate, while the prey protein was expressed as a fusion to the activation domain (AD) in pGAD424 gate. Bait and prey plasmids were cotransformed into the S. cerevisiae strain PJ69-4A. The transformants were selected by their growth on synthetic dextrose (SD) minimal medium lacking tryptophan (Trp) and leucine (Leu) (SD-WT medium), which are the nutritional selection markers for pGBT9 and pGAD424, respectively. We selected 3 positive independent transformants grown in the SD-WT liquid medium overnight at 30 °C and diluted the overnight culture to 1/10 (v/v). The positive interaction of expressed fusion proteins was then determined by 1/10 (v/v) overnight cultures’ growth on SD lacking Trp, Leu, and histidine (His) (SD-WTH medium) at 30 °C for at least 3 d.
Confocal microscope for TOT3 and truncated TOT3 localization
Seedlings were grown on continuous light at 21 °C conditions for 5 d after germination and then stained in 10 mg L−1 propidium iodide for 2 min and rinsed in water for 30 s. Confocal microscopy was performed using a Leica SP8 inverted confocal microscope with white light laser. Images were captured with a HyD. GFP was excited at 488 nm (88.5% intensity) and detected at 498 to 530 nm with gain 164.9. Propidium iodide was excited at 561 nm (1.86%) and detected at 578 to 640 nm with gain 28.6. Gating technology with lifetime between 0.3 and 6 ns was applied for auto-fluorescence removal.
Accession numbers
Sequence data from this article can be found in the GenBank/EMBL data libraries under the following accession numbers: MAP4K4/TOT3 (At5g14720), MAP4K5/TOI5 (At4g24100), MAP4K6/TOI4 (At4g10730), MAP4K7 (At1g70430), MAP4K8 (At1g79640), and MAP4K9 (At1g23700).
Author contributions
L.P., L.D.V., and I.D.S.: conceptualization; L.P. C.F.F.d.L., L.D.V., B.v.d.C., and N.D.W.: investigation; L.P and I.D.S.: writing—original draft; all authors: writing—review and editing; G.D.J., K.G., and I.D.S.: supervision.
Supplementary data
The following materials are available in the online version of this article.
Supplementary Figure S1. Expression pattern of MAP4K(4-9).
Supplementary Figure S2. Validation of the interaction between MAP4K4 and MAP4K(5-9).
Supplementary Figure S3. Western blot for tot3-2 complementing lines.
Supplementary Figure S4. Summary of TOT3-detected phosphorylation sites.
Supplementary Table S1. Protein identification details obtained with the Q Exactive (Thermo Fisher Scientific) and Mascot Distiller software (Matrix Science) combined with the Mascot search engine (Matrix Science) using the Mascot Daemon interface.
Supplementary Table S2. Overview of MAP4K interactors.
Supplementary Table S3. List of primers used in this study.
Supplementary Table S4. List of constructs used in this study.
Funding
This work was supported by the Research Foundation, Flanders (FWO.OPR.2019.0009.01). L.P. was supported by the China Scholarship Council for a predoctoral fellowship (201806870020) and by a UGent Bijzonder Onderzoeksfonds doctoral mandate (01CD0523). L.D.V. was supported by a UGent Bijzonder Onderzoeksfonds postdoctoral mandate (01P12219).
Data availability
All material will be made available upon reasonable request to the corresponding author ([email protected]).
Dive Curated Terms
The following phenotypic, genotypic, and functional terms are of significance to the work described in this paper:
References
Author notes
The author responsible for distribution of materials integral to the findings presented in this article in accordance with the policy described in the Instructions for Authors (https://dbpia.nl.go.kr/plphys/pages/General-Instructions) is: Ive De Smet ([email protected]).
Conflict of interest statement. None declared.