-
PDF
- Split View
-
Views
-
Cite
Cite
Siyang Gao, Mingxin Yin, Mingyang Xu, He Zhang, Shuai Li, Yinxiao Han, Shujuan Ji, Xinyue Li, Guodong Du, Transcription factors PuPRE6/PuMYB12 and histone deacetylase PuHDAC9-like regulate sucrose levels in pear, Plant Physiology, Volume 194, Issue 3, March 2024, Pages 1577–1592, https://doi.org/10.1093/plphys/kiad628
- Share Icon Share
Abstract
The improvement of fruit quality, in particular sugar content, has been a major goal of plant breeding programmes for many years. Here, 2 varieties of the Ussurian pear (Pyrus ussuriensis), Nanguo, and its high-sucrose accumulation bud sport, Nanhong, were used to study the molecular mechanisms regulating sucrose transport in fruits. Comparative transcriptome analysis showed that in Nanhong fruit, an MYB transcription factor, PuMYB12, and a sucrose transporter protein, PuSUT4-like, were expressed at higher levels, while a paclobutrazol resistance transcription factor, PuPRE6, and a histone deacetylase (HDAC), PuHDAC9-like, were expressed at lower levels in Nanguo fruit. PuSUT4-like silencing and overexpression experiments in Nanguo pear showed that PuSUT4-like is essential for sucrose transportation. PuPRE6 and PuMYB12 act as antagonistic complexes to regulate PuSUT4-like transcription and sucrose accumulation. The histone deacetylation levels of the PuMYB12 and PuSUT4-like promoters were higher in Nanguo fruit than in Nanhong fruit, and Y1H assays showed that HDAC PuHDAC9-like bound directly to the promoters of PuMYB12 and PuSUT4-like. Our results uncovered transcription regulation and epigenetic mechanisms underlying sucrose accumulation in pears.
Introduction
Sweetness is a critical factor influencing fruit quality and consumer purchasing decisions. The composition and concentration of sugar at fruit maturity are influenced by metabolic and transport processes that occur during fruit development. Thus, an understanding of these processes and their regulation is critical for improving fruit quality (Li et al. 2018). In the Rosaceae family, sorbitol is the main transport form of photosynthetic products, and the sucrose content cannot be underestimated (Cheng et al. 2005; Zhou et al. 2006). Sucrose is transported from the photosynthetic tissues (source) to the heterotrophic tissues (sink) by sucrose transporter (SUT) proteins. SUTs have been found in a wide range of species and plant organs since an SUT cDNA from spinach (Spinacia oleracea L.) was originally isolated and characterized (Riesmeier et al. 1992). In land plants, SUTs are plasma membrane proteins and function as the main transporters mediating sucrose import or export. Moreover, since their original identification in spinach, SUTs have been identified in other plants and divided into different categories (Reinders et al. 2012). Importantly, SUTs have been functionally characterized in different plants, and the molecular mechanisms that regulate them have started to be identified. For example, sucrose homeostasis in barley (Hordeum vulgare) grain is affected by the downregulation of the SUTs HvSUT1 and HvSUT2 (Radchuk et al. 2017). Additionally, in Arabidopsis (Arabidopsis thaliana) leaves, carbon export is controlled by ubiquitinating and phosphorylating the SUT SUC2 (Xu et al. 2020). Of note, SUTs also play a role in the regulation of fruit sugar accumulation, as is the case of MdSUT4.1 in apple (Malus domestica) (Peng et al. 2020). In addition, SUTs play roles in regulating plant growth and development and other processes. For example, the in vitro overexpression of VvSUC27 (grapevine SUT 27) improves plant growth in the presence of sucrose (Cai et al. 2017), and IbSUT4 is involved in sweet potato [Ipomoea batatas (L.) Lam] growth and adaptation to abiotic stress (Wang et al. 2020a).
Several studies have demonstrated that gene expression is regulated by multiple transcription factors, such as MYB and basic helix-loop-helix (bHLH). MYB transcription factors are involved in plant development and respond to biotic and abiotic stress (Wang et al. 2022). For example, the GARP/MYB-related transcription factor AQUILO promotes cold tolerance in grapes, as well as raffinose family oligosaccharide accumulation (Sun et al. 2018). In Arabidopsis, the R2R3-MYB transcription factor, MYB49, regulates cadmium accumulation (Zhang et al. 2019). The biosynthesis of anthocyanin in tomato (Solanum lycopersicum) fruits is affected by the alternative splicing of the R2R3-MYB transcription factor encoded by the anthocyanin fruit gene (Colanero et al. 2019). In pear (Pyrus ussuriensis) fruit, flavonol biosynthesis is positively regulated by PbMYB12b (Zhai et al. 2019), whereas anthocyanin accumulation is negatively controlled by PbMYB120 (Song et al. 2020). Sucrose and R2R3-MYB transcription factors contribute to the biosynthesis of potato (Solanum tuberosum) flavonols (Lin et al. 2021). In addition, MYB transcription factors improve glucose release in sugarcane transgenics (Poovaiah et al. 2016).
Paclobutrazol resistances (PREs) are atypical bHLH transcription factors (Lee et al. 2006) coded by gene families involved in regulating plant growth and development via various signaling pathways. bHLH has been widely studied in apple fruit. Overexpression of MdbHLH3 increases carbohydrate levels in apple leaves and increases carbohydrate accumulation in the fruit (Yu et al. 2022). MdbHLH3 is phosphorylated and stabilized by MdHXK1 (glucose sensor hexokinase1), a glucose sensor, to stimulate anthocyanin synthesis (Hu et al. 2016). In apple leaves, MdbHLH3 regulates dehydratase–enolase–phosphatase complex 1 to modulate leaf senescence (Hu et al. 2020). In addition, MYB can interact with bHLH to form an MYB–bHLH complex to participate in the regulation of anthocyanin biosynthesis. In poplar (Populus spp.), PdMYB118 interacts with the bHLH transcription factor, PdTT8, to regulate wound-induced anthocyanin biosynthesis (Wang et al. 2020b). In Nicotiana benthamiana leaves, MYB and bHLH transcription factors control anthocyanin and non-flavonoid synthesis (Outchkourov et al. 2014). However, the effect of MYB and bHLH on sugar accumulation in pear fruit needs further investigation.
Histone acetylation regulates gene transcription by altering chromatin architecture, or recruiting protein complexes (Strahl and Allis 2000; Ma et al. 2013). Histone acetylation is a reversible process regulated by histone deacetylases (HDACs) and histone acetyltransferases (HACs), in which HDACs inhibit gene transcription, and HACs activate gene expression (Sridha and Wu 2006; Ma et al. 2013). HDACs are involved in the regulation of plant growth and development (Fu et al. 2018; Guo et al. 2018). For example, a genome-wide target mapping study showed that HDAC complex 1 regulates cucumber fruit cell proliferation (Zhang et al. 2020). In addition, HDAC HDA15 inhibits Arabidopsis hypocotyl cell elongation during photomorphogenesis by interacting with HY5 (Zhao et al. 2019). Studies have also shown that HAC is involved in the regulation of plant sugar accumulation. In Arabidopsis, sugar responses are regulated by WRKY18 and WRKY53 in coordination with HISTONE ACETYLTRANSFERASE1 (HAC1) (Chen et al. 2019a, 2019b), and sugar response and gene expression are affected by mutations in HAC1 (Heisel et al. 2013). Histone acetylation at the promoter of PuWRKY31 modulates sucrose accumulation in pear (Li et al. 2020b). Despite these insights, HDAC-mediated sucrose accumulation in fruits warrants further investigation.
Pear belongs to the Rosaceae family and is widely cultivated all around the globe. Here, we study the sugar content in the Nanguo clonal variety of Ussurian pear (P. ussuriensis). The sugar content of the Nanguo pear is an important index that affects its commercial value. In perennial fruits, new varieties produced by stem cells of the parent, possibly through genetic or epigenetic changes, are referred to as bud sport varieties (Furiya et al. 2009). A bud sport variety of Nanguo was identified on a farm in Anshan, Liaoning province, in 2000 and was called Nanhong in 2011. Nanhong fruit accumulates more sugar than Nanguo, and the underlying regulation mechanisms are not clear. Here, we compared the transcriptomes of Nanguo and Nanhong fruits by RNA sequencing (RNA-seq) and revealed that PuSUT4-like, PuMYB12, PuPRE6, and PuHDAC9-like could contribute to the higher sucrose accumulation in the Nanhong variant. We found that the expression levels of PuSUT4-like and PuMYB12 in Nanhong fruit were higher than that in Nanguo; however, the PuPRE6 and PuHDAC9-like expressions were low in Nanhong fruit. PuMYB12 activated PuSUT4-like transcription, while PuPRE6 inhibited the expressions of PuSUT4-like and PuMYB12; the higher expressions of PuSUT4-like and PuMYB12 in Nanhong fruit might be associated with lower PuHDAC9-like expression.
Results
Nanhong fruit accumulates more sucrose than Nanguo fruit
We analyzed the soluble solids content of Nanguo and Nanhong fruits (Fig. 1A) from the years 2019 and 2021 and found that Nanhong fruit had a higher soluble solid content than Nanguo fruit (Supplemental Fig. S1A and B). High performance liquid chromatography (HPLC) was used to further determine which sugars were present at high levels in Nanhong fruit. The sucrose, fructose, sorbitol, and glucose levels were analyzed; only the sucrose showed a higher accumulation from 105 to 135 days after full bloom (DAFB) (Fig. 1B to E), and the data are consistent with a 2019 study (Supplemental Fig. S1C to F). These results suggested that higher sucrose content led to higher sugar accumulation in Nanhong fruit.
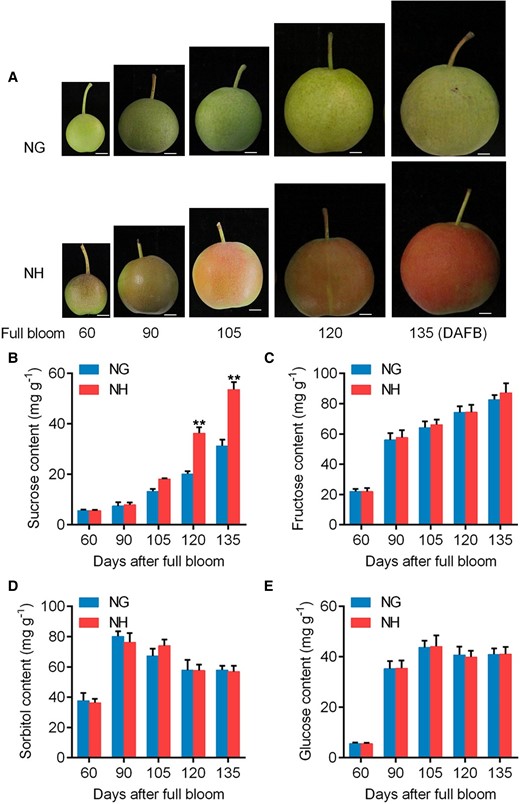
Pictures and sugar content of Nanguo (NG) and Nanhong (NH) fruits. A) Phenotype of NG and NH fruits collected on different DAFB in 2021. Scale bar, 1 cm. NG, Nanguo pear fruit; NH, Nanhong pear fruit. B to E) Sucrose B), fructose C), sorbitol D), and glucose E) contents of NG and NH fruits. Fruit samples were collected in 2021. Error bars represent SE obtained from 3 biological replicates. Asterisks indicate significant differences as determined by Student's t-test (**P < 0.01).
PuSUT4-like is highly expressed in Nanhong fruit and encodes a sucrose transporter
The transcriptomes of Nanguo and Nanhong fruits were compared by RNA-seq and compared with search for genes that might play a role in the higher sucrose accumulation in Nanhong fruit. This analysis revealed that 4 sugar transporters were highly expressed, among which a SUT gene showed the largest difference in expression (Supplemental Fig. S2A). Next, we investigated the SUT gene expression profiles in Nanguo and Nanhong fruits during development by reverse transcription quantitative PCR (RT-qPCR), and a higher SUT mRNA level was observed in Nanhong fruit at 105 to 135 DAFB in 2021 samples (Fig. 2A), and at 120 to 144 DAFB in 2019 samples (Supplemental Fig. S2B). Moreover, the trend of the SUT expression was consistent with that of the sucrose content (Fig. 1B; Supplemental Fig. S1C). According to the analysis of the phylogenetic tree, the SUT (rna36459) clustered with MdSUT4-like (MD15G1394000) and MdSUT4.1 (MD08G1209900) (Fig. 2B). The alignments of the amino acid sequences indicated that the SUT showed 96.47% similarity to MdSUT4-like (Supplemental Fig. S3) and 91.99% similarity to MdSUT4.1 (Supplemental Fig. S4). In addition, the conserved domain analysis of PuSUT4-like, MdSUT4-like, and MdSUT4.1 proteins revealed that their protein sequences were highly conserved (Supplemental Fig. S5). Accordingly, the SUT was named PuSUT4-like. Since the function of SUTs is determined by their localization, we examined the subcellular localization of PuSUT4-like in the protoplasts of maize (Zea mays). Through fluorescence microscopy, we found that the fluorescent signal of 35S:GFP-PuSUT4-like was localized to the plasma membrane only, whereas the GFP alone control was detected throughout the cytoplasm and nucleus (Fig. 2C).
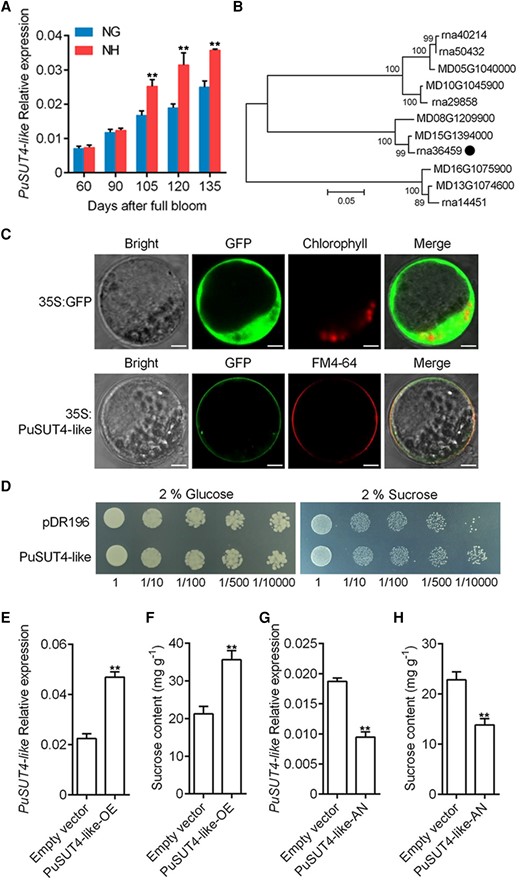
Expression, subcellular localization, and functional analysis of PuSUT4-like. A)PuSUT4-like mRNA expression in NG and NH fruits collected in 2021 was assessed by RT-qPCR. B) Phylogenetic tree derived from amino acid sequences of SUTs in pear and apple. PuSUT4-like was highlighted with the dot in the phylogenetic tree. Numbers indicate bootstrap test result with 1,000 replicate analyses. The scale bar represents 0.05 substitutions per site. C) The subcellular localization of transiently expressed 35S:GFP-PuSUT4-like was examined in protoplasts of maize leaves. FM4-64 was used as a plasma membrane marker. Scale bars, 5 μm. D)PuSUT4-like expression in SUSY7/ura yeast grown on 2% (w/v) sucrose or glucose as the sole carbon source. Numbers under the images indicate the dilution fold. E and F)PuSUT4-like was overexpressed in on-tree NG fruits at 120 DAFB, the infiltrated fruits were harvested 6 d after infiltration, and the expression level of PuSUT4-likeE) and sucrose content F) were measured. G and H)PuSUT4-like was silenced in on-tree NG fruits at 120 DAFB, the infiltrated fruits were harvested 6 d after infiltration, and the expression level of PuSUT4-likeG) and sucrose content H) were measured. Error bars represent SE obtained from 3 biological replicates. Asterisks indicate significant differences as determined by Student's t-test (**P < 0.01).
To provide evidence for PuSUT4-like functioning as a SUT, we cloned the SUT and transfected it into mutant yeast (Saccharomyces cerevisiae) SUSY7/ura cells (Li et al. 2020b) using the yeast expression vector pDR196. We used mutant strains carrying empty pDR196 vectors as controls. The transformants were grown on synthetic-deficient (SD) medium containing 2% sucrose or 2% glucose as the sole carbon source. The pDR196-PuSUT4-like and empty vector (pDR196) transformants grew well on 2% glucose-containing medium; however, only pDR196-PuSUT4-like transformants grew well on 2% sucrose-containing medium (Fig. 2D), suggesting that PuSUT4-like is a functional SUT. To further elucidate the importance of PuSUT4-like in sucrose transport, we overexpressed it in Nanguo fruit. Successful overexpression in fruits carrying the cDNA expression vector was confirmed by RT-qPCR analysis of PuSUT4-like mRNA levels (Fig. 2E). In addition, a higher sucrose content was detected in PuSUT4-like overexpressing fruit (PuSUT4-like-OE) compared with controls (Fig. 2F); however, no significant differences were observed in the contents of glucose, fructose, and sorbitol (Supplemental Fig. S6). Next, we silenced the expression of PuSUT4-like in Nanguo fruit (PuSUT4-like-AN) using an antisense construct approach. PuSUT4-like-AN fruits showed substantially suppressed expression of PuSUT4-like (Fig. 2G) and less accumulation of sucrose (Fig. 2H) compared with non-silenced control fruits. In contrast, no significant differences were observed in the contents of glucose, fructose, and sorbitol (Supplemental Fig. S7). Collectively, these results showed that PuSUT4-like is localized to the plasma membrane and is a SUT in pear fruit.
PuMYB12 and PuPRE6 play important roles in sucrose accumulation in pear
We analyzed the transcriptomic data and chose 8 transcription factors with the most significant differences, including 2 MYB and 4 bHLH genes, to create a heat map (Supplemental Fig. S8A). We found that an MYB transcription factor, PuMYB12, was significantly up-regulated in Nanhong fruit. Additionally, PuPRE6, an atypical bHLH transcription factor, was significantly less expressed in Nanhong pear. RT-qPCR analysis was carried out to confirm the PuMYB12 and PuPRE6 expression levels observed by RNA-seq. The results showed that PuMYB12 was more highly expressed in Nanhong fruit than in Nanguo fruit (Fig. 3A; Supplemental Fig. S8B), which was consistent with the trend of PuSUT4-like expression (Fig. 2A). On the other hand, PuPRE6 presented an opposite trend and was more highly expressed in Nanguo fruit (Fig. 3A; Supplemental Fig. S8C). Moreover, the cis-acting elements of the promoter were analyzed using PlantCARE; 8 MYB and 4 bHLH binding sites were identified (Supplemental Fig. S9). Then, we investigated the subcellular localization of PuMYB12 and PuPRE6 and found that they were both localized in the nucleus (Fig. 3B). To investigate the function of PuMYB12 and how it could affect PuSUT4-like expression and sucrose levels, we performed overexpression and loss of function experiments in Nanguo fruit. In the overexpressed (PuMYB12-OE) condition (Fig. 3C), we found that the expression of PuSUT4-like (Fig. 3D) and the sucrose content (Fig. 3E) were increased. Conversely, in PuMYB12 silenced fruit (PuMYB12-AN) (Fig. 3F), we observed significantly down-regulated PuSUT4-like expression (Fig. 3G) and less sucrose accumulation (Fig. 3H). Similar experiments were performed for PuPRE6. In this case, when PuPRE6 was overexpressed in fruit (PuPRE6-OE) (Fig. 3I), we found that PuSUT4-like expression (Fig. 3J) and sucrose accumulation (Fig. 3K) were significantly inhibited. Conversely, PuSUT4-like expression (Fig. 3) and sucrose accumulation (Fig. 3N) were significantly promoted in PuPRE6-silenced fruit (PuPRE6-AN) (Fig. 3L). These results suggested that PuMYB12 and PuPRE6 might play pivotal roles in regulating sucrose transport through PuSUT4-like transcription regulation in pear fruit.
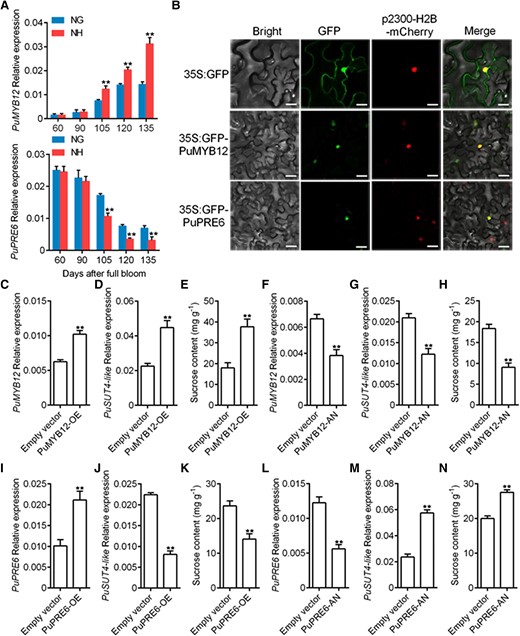
Expression, subcellular localization, and functional analysis of PuMYB12 and PuPRE6. A)PuMYB12 and PuPRE6 mRNA expression in NG and NH fruits collected in 2021. B) The subcellular localization of transiently expressed 35S:GFP-PuMYB12 or 35S:GFP-PuPRE6 were examined in N. benthamiana leaves. Transient expression of GFP alone was used as a control. p2300-H2B-mCherry was used as a nucleus marker. Scale bars, 25 μm. C to E)PuMYB12 was overexpressed in on-tree NG fruits at 120 DAFB, the infiltrated fruits were harvested 6 d after infiltration, and the expression level of PuMYB12C), PuSUT4-likeD), and sucrose content E) were measured. F to H)PuMYB12 was silenced in on-tree NG fruits at 120 DAFB, the infiltrated fruits were harvested 6 d after infiltration, and the expression level of PuMYB12F), PuSUT4-likeG) and sucrose content H) were measured. I to K)PuPRE6 was overexpressed in on-tree NG fruits at 120 DAFB, the infiltrated fruits were harvested 6 d after infiltration, and the expression level of PuPRE6I), PuSUT4-likeJ), and sucrose content K) were measured. L to N)PuPRE6 was silenced in on-tree NG fruits at 120 DAFB, the infiltrated fruits were harvested 6 d after infiltration, and the expression level of PuPRE6L), PuSUT4-likeM), and sucrose content N) were measured. Error bars represent SE obtained from 3 biological replicates. Asterisks indicate significant differences as determined by Student's t-test (**P < 0.01).
PuMYB12 and PuPRE6 bind to promoter of PuSUT4-like and regulate its transcription
A yeast 1-hybrid (Y1H) assay was performed to investigate whether the PuSUT4-like promoter was a direct transcriptional target of PuMYB12 and PuPRE6. The results presented in Fig. 4A and B show that both PuMYB12 and PuPRE6 could bind to the PuSUT4-like promoter in vitro. Then, chromatin immunoprecipitation-polymerase chain reaction (ChIP-PCR) was conducted to further analyze the in vivo binding of FLAG-tagged PuMYB12 and PuPRE6 to the PuSUT4-like promoter. PuMYB12-OE (Fig. 3C) and PuPRE6-OE (Fig. 3I) fruits were used to extract cross-linked chromatin samples, which were precipitated with anti-FLAG antibodies. The enhanced detection of the PuSUT4-like promoter in PuMYB12-OE or PuPRE6-OE fruit via PCR-based approaches showed that PuMYB12 (Fig. 4C) and PuPRE6 (Fig. 4D) bind to the PuSUT4-like promoter in vivo.
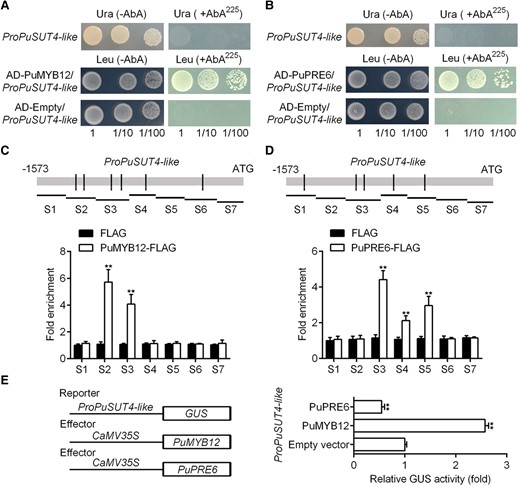
PuMYB12 promotes PuSUT4-like expression, while PuPRE6 inhibits the PuSUT4-like expression. A and B) Y1H analysis of PuMYB12 A) or PuPRE6 B) binding to the promoter of PuSUT4-like. Numbers under the panel indicate the serial 10-fold dilutions of samples. C and D). ChIP-PCR showing the binding of PuMYB12 C) or PuPRE6 D) to the PuSUT4-like promoter in vivo. Immunoprecipitated DNA values were normalized to input DNA values. E) PuMYB12 or PuPRE6 effector vectors together with the reporter vector containing the PuSUT4-like promoter were infiltrated into wild Nicotiana tabacum leaves to analyze the regulation of GUS activity. Error bars represent SE obtained from 3 biological replicates. Asterisks indicate significant differences as determined by Student's t-test (**P < 0.01).
The regulation of the PuSUT4-like promoter by PuMYB12 or PuPRE6 was examined by β-glucuronidase (GUS) activation assay in N. benthamiana leaves. The Pro35S:PuMYB12 and Pro35S:PuPRE6 effector constructs were generated by cloning the coding sequences (CDSs) of PuMYB12 or PuPRE6 into the pRI101 vector. Then, we coinfiltrated the Pro35S:PuMYB12 or Pro35S:PuPRE6 together with the PuSUT4-like:GUS plasmid. Figure 4E shows that the GUS activity significantly increased when Pro35S:PuMYB12 was cotransformed with ProPuSUT4-like:GUS, suggesting that PuMYB12 increased the activity of the PuSUT4-like promoter. In contrast, when Pro35S:PuPRE6 was cotransformed with ProPuSUT4-like:GUS, the GUS activity was further suppressed. Collectively, these results indicated that PuMYB12 directly up-regulates PuSUT4-like transcription, while PuPRE6 suppresses it.
PuPRE6 suppresses expression of PuMYB12 via transcriptional regulation
To investigate a possible relationship between PuPRE6 and PuMYB12, we analyzed PuMYB12 expression in PuPRE6-OE fruit, and PuPRE6 expression in PuMYB12-OE fruit. We found that the overexpression of PuMYB12 had no effect on PuPRE6 gene expression (Fig. 5A); however, overexpression of PuPRE6 inhibited PuMYB12 (Fig. 5B). To further investigate the regulatory role of PuPRE6 on PuMYB12, we conducted a Y1H assay to test whether the promoter of PuMYB12 was a direct transcriptional target of PuPRE6, and we found that PuPRE6 bound directly to the PuMYB12 promoter in vitro (Fig. 5C). To determine the in vivo binding potential of PuPRE6 to the PuMYB12 promoter, a ChIP assay was conducted. We found that PuPRE6 bound to the promoter of PuMYB12 in vivo (Fig. 5D). In agreement, GUS analysis revealed that PuPRE6 repressed the transcription of PuMYB12 (Fig. 5E). These results indicated that PuPRE6 played a decisive role in the accumulation of high sucrose in Nanhong pear by regulating the transcription of PuMYB12.
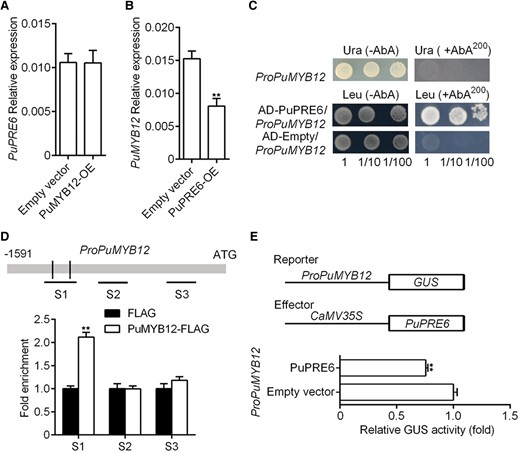
PuPRE6 suppresses PuMYB12 expression. A) mRNA expression of PuPRE6 in PuMYB12 overexpressing fruit, and fruit samples were the same as in Fig. 3C. B) mRNA expression of PuMYB12 in PuPRE6 overexpressing fruit, and fruit samples were the same as in Fig. 3I. C) Y1H analysis of PuPRE6 binding to the PuMYB12 promoter. Numbers under the panel indicate the serial 10-fold dilutions of samples. D) ChIP-PCR analysis of PuPRE6 binding to PuMYB12 promoter in vivo. Immunoprecipitated DNA values were normalized to input DNA values. E) Effector vector of PuPRE6 together with the reporter vector containing the PuMYB12 promoter were infiltrated into wild N. tabacum leaves to analyze the regulation of GUS activity. Error bars represent Se obtained from 3 biological replicates. Asterisks indicate significant differences as determined by Student's t-test (**P < 0.01).
Expression of PuMYB12 and PuSUT4-like is related to histone deacetylation levels
According to the results of the transcriptome analysis, we found a HDAC gene, PuHDAC9-like, that was more highly expressed in Nanguo fruit than in Nanhong fruit (Fig. 6A; Supplemental Fig. S10). We reasoned that the lower expressions of PuMYB12 and PuSUT4-like in Nanguo fruit might be associated with the decreased deacetylation of histone protein. Thus, we analyzed the H3ac and H4ac levels in the promoter and CDS regions of PuMYB12 and PuSUT4-like in both pear variants, and higher levels of histone acetylation in PuMYB12 and PuSUT4-like promoter and CDS regions were found in Nanhong (Fig. 6B to F). To ascertain the role of PuHDAC9-like in regulating the histone acetylation levels of PuMYB12 and PuSUT4-like genes, we performed a Y1H assay, which showed that PuHDAC9-like could directly bind to the promoters of PuMYB12 and PuSUT4-like (Fig. 6G and H). To understand the PuHDAC9-like expression profiles in Nanguo and Nanhong fruits, we compared their CDSs; however, no differences were observed (Supplemental Fig. S11). In addition, no differences were observed in the promoter regions of PuHDAC9-like between Nanguo and Nanhong fruits (Supplemental Fig. S12).
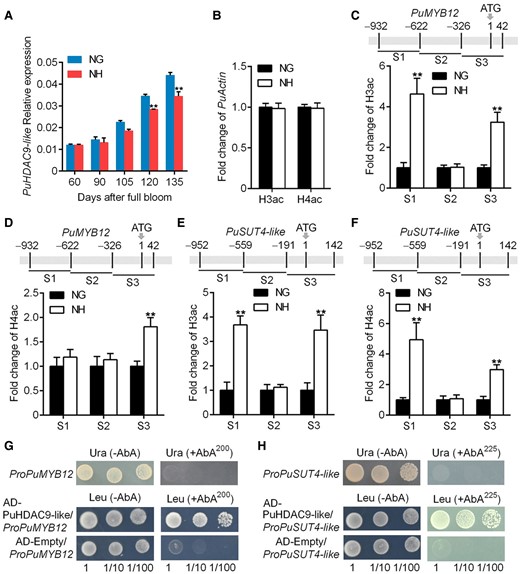
Expression of PuHDAC9-like and histone acetylation of PuMYB12 and PuSUT4-like between NG and NH fruits. A)PuHDAC9-like mRNA expression in NG and NH fruits collected in 2021. B) Histone acetylation level of PuActin chromatin determined by H3ac or H4ac analysis in NG and NH fruits. The amount of immunoprecipitated DNA was normalized to the amount of input DNA. C and D) Histone acetylation level at different regions of the PuMYB12 chromatin determined by H3ac C) or H4ac D) analysis in NG and NH fruits. E and F) The histone acetylation level at different regions of the PuSUT4-like chromatin determined by H3ac E) or H4ac F) analysis in NG and NH fruits. G and H) Y1H analysis of PuHDAC9-like binding to the promoter of PuMYB12G) or PuSUT4-likeH). Numbers under the panel indicate the serial 10-fold dilutions of samples. Error bars represent Se obtained from 3 biological replicates. Asterisks indicate significant differences as determined by Student's t-test (**P < 0.01).
Discussion
Sugars are not only essential for supplying energy but also for providing carbon skeletons for intracellular biomolecules, as well as playing a pivotal role in determining fruit quality (Ayre 2011). The sugar content of fruits is a critical determinant of their sweetness; however, details on the highly complex molecular mechanisms that underlie sugar accumulation remain scarce. Nanhong is a red bud sport variety of Nanguo, and its red skin makes it more popular with consumers. More importantly, Nanhong accumulates a larger amount of sugar. In our study, the regulation of PuPRE6 and PuMYB12 antagonistic action resulted in higher expression of PuSUT4-like and higher sucrose content in Nanhong fruit than in Nanguo fruit. In addition, the low level of histone deacetylation of the PuMYB12 and PuSUT4-like promoters affected sucrose accumulation in Nanhong fruit.
Sucrose transporter increases sucrose content of Nanhong fruit
In Rosaceae, sorbitol is the predominant carbohydrate transport form. Nevertheless, a nonnegligible amount of sucrose is present (Priestley 1983; Zhang et al. 2014). In this study, we found that the increased sucrose accumulation resulted in the increased sugar content in Nanhong fruit in the years 2019 and 2021 (Supplemental Fig. S1A and B). In contrast, through 2 yr of measurement, we found that there was no difference in sorbitol content between Nanguo and Nanhong fruits. Sucrose transport occurs through plasmodesmata by plasma membrane-localized SUT proteins (Sun et al. 2019; Ko et al. 2022). It is then transported to the fruit and metabolized by enzymes, such as sucrose phosphate synthase, sucrose synthase, and invertase (Moriguchi et al. 1992; Sturm et al. 1999). Therefore, sucrose accumulation in fruits depends on its transportation and metabolism.
We compared the transcriptomes of Nanguo and Nanhong fruits. The genes associated with sucrose metabolism did not show differential expression. However, PuSUT4-like had the highest expression in Nanhong fruit than in Nanguo fruit (Fig. 2A; Supplemental Fig. S2B). In apple, MdSUT4.1 expression negatively associated with fruit sucrose accumulation (Peng et al. 2020). Typically, proteins encoded by homologous genes have a comparable function in controlling plant development and physiological processes. As mentioned above, PuSUT4-like may play a role in sucrose transport in pear fruit. To validate this hypothesis, a subcellular localization study in maize protoplasts was conducted. We found that the PuSUT4-like protein localized to the plasma membrane and provided a basis for its role in sucrose transport (Fig. 2C). The heterologous expression of PuSUT4-like in yeast showed that it encodes a functional SUT (Fig. 2D). Furthermore, we overexpressed and silenced PuSUT4-like expression in Nanguo fruit and found that PuSUT4-like plays a role in increasing sucrose accumulation in pear fruit (Fig. 2E to H). These results indicate that the SUT4 gene may not play the same role in sucrose accumulation, suggesting functional divergence between the homologous genes. Although our study initially clarified that the high sucrose accumulation in Nanhong pear was associated with the high expression of PuSUT4-like, the reason for sucrose in Nanhong pear starting to increase at 105 DAFB still needs to be determined through further analysis.
PuMYB12 and PuPRE6 synergistically regulate sucrose accumulation in pear and PuPRE6 works upstream of PuMYB12
Transcription factors are important regulators of gene expression (Li et al. 2020a). Our RNA-seq results revealed that PuMYB12 and PuPRE6 expression was significantly different between the Nanguo and Nanhong fruits (Supplemental Fig. S8A). The sequencing results were further validated using RT-qPCR and the expression levels of these 2 genes were in agreement with the RNA-seq data (Fig. 3A; Supplemental Fig. S8B and C). Then, the analysis of the PuSUT4-like promoter (Supplemental Fig. S9) suggested that PuMYB12 and PuPRE6 might play a role in regulating its expression. Based on these results, we selected PuMYB12 and PuPRE6 as the research objects.
The MYB transcription factor family is one of the most important transcription regulatory factors in plants. Numerous studies have analyzed the MYB12 gene involved in the biosynthesis of flavonoid and anthocyanin, such as in Arabidopsis (Mehrtens et al. 2005), tomato (Luo et al. 2008), and grape (Matus et al. 2008). Moreover, in tomato, MYB12 substantially contributes to salt tolerance (Kashyap et al. 2020). However, in this study, we showed strong evidence of the involvement of PuMYB12 in regulating sugar accumulation in pear fruit. Thus, the present research advances our understanding of MYB12 regulatory mechanisms. MYB plays a vital role in anthocyanin synthesis (An et al. 2020; Liu et al. 2023); our study showed that MYB contributes to sugar accumulation in pear fruit. Simultaneous regulation of flavor and coloration will significantly enhance fruit quality formation and breeding efficiency, emphasizing the crucial role of MYB.
bHLH transcription factors are widespread in plants, and bHLH transcription factors comprise the second-largest plant transcription factor family. Most bHLH proteins have been identified, primarily in Arabidopsis, rice, and tomato, where they control plant development, fruit ripening, anthocyanin biosynthesis, and hormone-signaling responses (Wang et al. 2018; Zhao et al. 2018). bHLH is involved in regulating starch and sugar accumulation. For example, in N. benthamiana pollen, starch accumulation and starch–sugar conversion are closely related to the bHLH transcription factor NtMYC2 (Bian et al. 2021). MdbHLH3 binds to the promoter of a phosphofructokinase gene and activates its expression, thereby promoting the accumulation of soluble sugar in apple (Yu et al. 2022). In this study, we hypothesized that PuPRE6 participated in the regulation of sucrose accumulation in fruits. To test this hypothesis, we investigated the function of the PuPRE6 gene in Nanguo fruit. Overexpression of PuPRE6 significantly suppressed PuSUT4-like expression and inhibited sucrose content (Fig. 3I to K). Moreover, PuPRE6 silencing had the opposite effect (Fig. 3L to N). These results indicate that PuPRE6 inhibits sucrose accumulation in pear fruit by repressing the transcription of PuSUT4-like.
The protein–protein interactions between R2R3-MYB and bHLH transcription factors conservatively control anthocyanin biosynthesis in plants. For example, Zhou et al. (2019) found that the PpMYB18 protein competes with the MYB activator PpMYB10 for binding to the bHLHs, balancing proanthocyanidin and anthocyanin accumulation with a fine-tuning regulatory loop in peach. MdbHLH33 interacts with MdMYB305 to facilitate its combination with downstream sugar-related genes, thereby increasing fruit sugar accumulation in apple (Zhang et al. 2023). In the present study, we showed that PuMYB12 is a transcriptional activator, and PuPRE6 is a transcriptional repressor. To explore a regulatory role between PuMYB12 and PuPRE6, we analyzed the expression level of PuPRE6 in PuMYB12-OE and control fruits, and no difference was found (Fig. 5A). In contrast, in PuPRE6-OE fruit, we detected that the expression of PuMYB12 was lower than that of control fruit (Fig. 5B). According to these results, we speculated that the PuPRE6 transcription factor functions upstream of PuMYB12. Furthermore, we found that PuPRE6 bound to the PuMYB12 promoter and negatively regulated its transcription (Fig. 5C to E).
Collectively, these results indicate that PuPRE6 regulates sucrose accumulation through 2 mechanisms, one by directly inhibiting the transcription of PuSUT4-like and the other by regulating the transcription of PuMYB12, thereby regulating PuSUT4-like expression. Our results may supply evidence for the involvement of bHLH and MYB transcription factors in regulating pear fruit sucrose accumulation and provide information for understanding the quality formation of fleshy fruit.
Histone deacetylation is essential for sucrose accumulation in pear
The acetylation of histones is an important regulatory mechanism. HACs add an acetyl group to conserved lysine amino acids on the N-terminus of histones to promote gene expression, whereas HDACs reduce HACs activity by removing acetyl groups from histones, thereby inhibiting gene expression (Chen et al. 2019a, 2019b; Ning et al. 2019; Zhang et al. 2020). HDACs function as cofactors recruited by transcription factors to regulate chromatin remodeling, transcription factor activity, and gene transcription (Jia et al. 2014). HDACs play important roles in regulating fruit ripening (Hu et al. 2022), leaf senescence (Huang et al. 2022), cell proliferation (Zhang et al. 2020), and carotenoid accumulation (Guo et al. 2017). HACs participate in regulating sugar accumulation (Heisel et al. 2013; Chen et al. 2019a, 2019b; Li et al. 2020b). However, whether sugar accumulation is mediated by HDAC remains unknown. In the present study, we show that an HDAC gene, PuHDAC9-like, was expressed at significantly lower levels in the Nanhong pear (Fig. 6A). To investigate potential differences in histone deacetylation levels of PuMYB12 and PuSUT4-like between Nanguo and Nanhong fruits, we compared the H3ac and H4ac levels in the promoter and CDS regions, and higher levels of histone deacetylation were found in the Nanguo fruit (Fig. 6B to F). We also observed that PuHDAC9-like bound to the promoter regions of PuMYB12 and PuSUT4-like (Fig. 6G and H). These results demonstrate that the higher expressions of PuHDAC9-like in Nanguo fruit could increase the histone deacetylation levels of PuMYB12 and PuSUT4-like, thereby inhibiting their expressions. Although our study offers further evidence supporting the importance of histone deacetylation in regulating sucrose accumulation in pear fruit, we did not elucidate why PuHDAC9-like is differentially expressed between Nanguo and Nanhong fruits, and the reasons certainly require additional study.
Finally, we built a model of how transcription regulation and epigenetics control pear fruit sugar accumulation (Fig. 7). Nanhong fruit presented higher PuSUT4-like and PuMYB12 expression and lower PuPRE6 and PuHDAC9-like expression than Nanguo fruit. In this system, PuPRE6 inhibits the transcription of both PuMYB12 and PuSUT4-like by binding to their promoters, whereas PuMYB12 enhances the expression of PuSUT4-like. Moreover, the high expression levels of PuMYB12 and PuSUT4-like are associated with the high acetylation levels of their promoters and CDSs in Nanhong fruit.
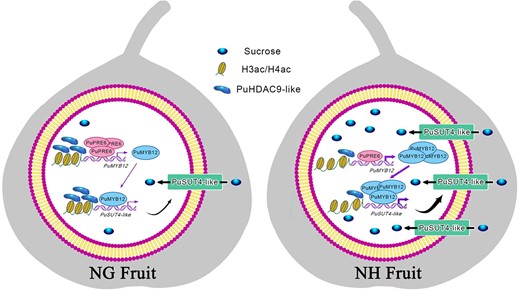
Molecular mechanism model of sucrose accumulation coregulated by transcriptional regulation and epigenetics in pear fruit. Molecular mechanism model of transcriptional regulation and epigenetic regulation of sucrose accumulation in pear fruit. NG, Nanguo pear fruit; NH, Nanhong pear fruit.
Materials and methods
Plant material and treatments
The experimental fruits are Ussurian pear (P. ussuriensis) cv. “Nanguo” and its bud sport variety (Nanhong), which were obtained from the orchard (Anshan, China).
Fruits were collected at 60, 90, 120, 144 DAFB (commercial harvest) in 2019, and 60, 90, 105, 120, 135 DAFB (commercial harvest) in 2021, and immediately transported to the laboratory. Following the measurement of sugar content, slices of flesh from each of the 5 fruits in each group were pooled, frozen in liquid nitrogen and then stored at −80 °C for subsequent RNA extraction and gene expression analysis.
Measurements of soluble solids and sugar content
The soluble solids and sugar content were measured according to previously reported by Li et al. (2020b). HPLC (Agilent Technologies, 1260 Series) was used to measure soluble sugar content. Firstly, adding 8 mL of 80% (v/v) ethanol to the sample, and then boiling in a water bath for 30 min at about 80 °C. The homogenates were centrifuged for 5 min at 10,000 × g in order to collect the supernatant. This sample was heated in a boiling water bath, cooled to room temperature (25 ± 0.5 °C), diluted with 1 mL of ultrapure water and then filtered by a 0.45 μm membrane. Analyses were performed on an Agilent 1260 HPLC system.
RNA-seq
RNA-seq was performed with total RNA isolated from Nanguo and Nanhong at 120 DAFB. As in Huang et al (2014), Trapnell et al (2010), Biomarker performed RNA-seq analysis, including library creation, sequencing, and bioinformatics analysis (www.biomarker.com.cn). Sequencing was performed on an Illumina HiSeq 2100 system. As described in the preceding section, 3 biological replicates of the total RNA were taken from 3 groups of fruit for RNA-seq. Under the accession number PRJNA937911 all of the raw data were submitted to the NCBI Sequence Read Archive.
Gene expression analysis
As described by Li et al. (2020b), total RNA was extracted, cDNA was synthesized. In the following step, RT-qPCR was conducted using the cDNA template. The sequence information of each gene was derived from the RNA-seq data. The RT-qPCR analysis was conducted with SYBR Premix ExTaq II Kit (Cat. no. RR820, TaKaRa) on an Applied Biosystems 7500 Real-Time PCR System, as previously described (Tan et al. 2013). To determine gene expression levels, the pear PuActin gene was used as an internal control. RNA extracted from each group of fruits was analyzed in triplicate. Primer3 software (https://bioinfo.ut.ee/primer3-0.4.0/) was used to design primers for each gene and all primers were listed in Supplemental Data Set 1.
Gene structures, heat map, and phylogenetic tree analysis
The alignments of the amino acid sequences were carried out using DNAMAN software. Gene structures were drawn using the NCBI database. An online platform (https://www.biocloud.net/) was used to generate the heat map. Transcription factor genes for making heat maps from RNA-seq data are listed in Supplemental Data Set 2. Sugar transporter genes for making heat maps from RNA-seq data are listed in Supplemental Data Set 3. To classify the PuSUTs, their amino acid sequences were aligned with those of the SUT genes from apple using MEGA5 with default parameters. The resulting data matrix was used to construct phylogenetic tree using the neighbor-joining method. The bootstrap values were estimated from 1,000 replicates. The predicted amino acid sequences were obtained from GDR (https://www.rosaceae.org/blast).
Subcellular localization
In a previous paper (Li et al. 2020b), we described the preparation of protoplasts of maize (Z. mays) leaves. The CDSs of PuSUT4-like, PuMYB12, and PuPRE6 were cloned into the KpnI and EcoRI sites downstream of GFP in the pRI101 vector to generate the Pro35S:GFP:PuSUT4-like, Pro35S:GFP:PuMYB12, and Pro35S:GFP:PuPRE6 constructs. As a control, the empty vector (Pro35S:GFP) was used. The Pro35S:GFP:PuSUT4-like or Pro35S:GFP were transformed into the protoplasts of maize leaves according to Li et al. (2020b). The cell membrane was stained with FM4-64 N-(3-triethylammoniumpropyl)-4-(6-(4-(diethylamino) phenyl) hexatrienyl) pyridinium dibromide (Cat. no. T3166, Thermo Fisher Scientific). The PuMYB12 and PuPRE6 constructs with the nuclear marker (NF-YA4-mCherry) were coinfiltrated into N. benthamiana leaves using Agrobacterium tumefaciens infiltration. The fluorescence was observed with a confocal microscope (TCS SP8, Leica, Germany) with the following properties: detector, PMT; intensity, 5%, gain, 670 to 730. Laser/collection bandwidths were 488 nm/498 to 550 nm for GFP, 561 nm/606 to 624 nm for mCherry, 488 nm/706 to 756 nm for chloroplast, and 561 nm/591 to 666 nm for FM4-64. There were at least 3 repeats of all transient expression assays. In Supplemental Data Set 1, we list the primers that we used.
Yeast complementation assay-heterologous expression of PuSUT4-like
According to Li et al. (2020b), complementation assays were performed in yeast cells with the CDS of PuSUT4-like cloned into pDR196 using SmaI and SalI restriction enzyme sites to form the pDR196-PuSUT4-like construct. We used the pDR196 empty vector as a control. By using the lithium acetate method, the constructs were transformed into the yeast mutant SUSY7. The cells were grown overnight to an OD600 of 0.5 in liquid medium SD (Cat. no. PM2271, Coolaber; http://www.coolaber.com/) with 2% (w/v) glucose as the only carbon source without uracil. Afterwards, serial dilutions were performed and we dropped different dilutions of this cell suspension on solid synthetically defined (lack ura) medium (pH 4.0) supplemented with 2% (w/v) glucose or sucrose as the sole carbon source. Cells were grown for 2 d at 30 °C on plates with glucose, and those were grown for 4 d at 30 °C on medium with sucrose.
Y1H assay
The PuMYB12, PuPRE6, PuHDAC9-like CDSs were introduced into the pGADT7 vector using the NdeI and EcoRI restriction sites. The PuSUT4-like, PuMYB12 promoter fragments were cloned into the pAbAi vector using the KpnI and SalI restriction sites. The binding of transcription factor to the promoters was assayed using the Matchmaker Gold Y1H Library Screening System kit (Cat. no. 630491; Clontech) and performed as reported (Li et al. 2022).
GUS analysis
The promoter sequences of PuSUT4-like and PuMYB12 were introduced into a GUS reporter gene in the pBI101 vector using the BamHI and HindIII restriction sites. The CDs regions of PuMYB12 and PuPRE6 were cloned into the pRI101 vector using restriction enzyme with NdeI and SacI to generate the effector constructs. The reporter and effector vectors were transformed into A. tumefaciens strain EHA105, and wild N. benthamiana leaves were used for coinfiltration. Then, the measurements of GUS activity were performed as previously described (Li et al. 2020b).
A. tumefaciens infiltration (overexpression and silencing experiments)
To overexpress PuMYB12, PuPRE6, and PuSUT4-like expression in pear fruit, the full CDS regions were cloned into the pRI101 vector with NdeI and SacI restriction sites to generate the overexpression constructs. To silence PuMYB12, PuPRE6, and PuSUT4-like expression, the full CDS were ligated into the pRI101 vector in the reverse orientation to generate antisense constructs. The recombinant constructs were transformed into A. tumefaciens strain EHA105, and the preparation of infiltration buffer and fruit infiltration were performed as previously described (Li et al. 2020b). Briefly, the infiltration buffer (about 100 μL) was injected into on-tree Nanguo pear fruits at 120 DAFB using a 1 mL sterile syringe. One side of the pear was injected with the infiltrating target constructs, and on the other side, the empty pRI101 was used as control. Three injections were performed on each side of fruit. All infiltrated fruits were harvested on Day 7 after infiltration, and the infiltrated areas were carefully separated for subsequent experiments. One pear was considered as a biological replicate, and at least 10 biological replicates were done.
ChIP-PCR analysis
The PuPRE6 and PuMYB12 CDSs were connected to the pRI101-3 × FLAG with NdeI and SmaI restriction sites, and transformed into the A. tumefaciens strain EHA105, and then injected into the pear fruits as described above. The preliminary treatment of pear fruits for the ChIP-PCR assay was as described in Li et al. (2020b). The ChIP assay was performed using the SimpleChip Plus Sonication Chromatin IP Kit according to the manufacturer's instructions (Cat. no. 56383; Cell Signaling Technology, Danvers, MA, USA). qPCR analysis was used to determine the amount of immunoprecipitated chromatin. Each ChIP assay was repeated 3 times. Primers were listed in Supplemental Data Set 1.
Analysis of histone acetylation levels
The samples used for analyzing the histone acetylation levels corresponded to were Nanguo and Nanhong pears harvested at 135 DAFB (commercial harvest) in 2021. Antiacetyl-histone H3 and H4 (Millipore, Billerica, MA) specific antibodies for immunoprecipitation. To analyze the histone acetylation level of the PuMYB12 or PuSUT4-like promoter, ChIP-PCR assay was performed as described by Li et al. (2020b). The promoters of PuMYB12 and PuSUT4-like were divided for the analysis to assess enrichment. The used primers are listed in Supplemental Data Set 1.
Statistical analysis
The data were analyzed using SPSS, version 19.0 (IBM Inc., Chicago, Illinois, USA), and the 1-way ANOVA was performed. Independent sample t-test was used to determine the significance of the treatment effects at the level of 5%.
Accession numbers
Sequence data from this article can be found in GenBank libraries under accession numbers PuSUT4-like (XM_048583180), PuMYB12 (XM_048584589), PuPRE6 (XM_009371042), PuHDAC9-like (XM_009365998), and PuActin (AF386514).
Acknowledgments
We thank Prof. Wang Aide (Shenyang Agricultural University, Shenyang, China) for providing the plasmids. We thank Dr Tong Li (Shenyang Agricultural University, Shenyang, China) for revisions and valuable suggestions on this manuscript. We thank Editage for technical editing of the manuscript.
Author contributions
G.D. and X.L. designed the experiments, analyzed the data, and wrote the manuscript. S.G. performed most of the experiments. M.Y. extracted the RNA. M.X. and H.Z. measured the sugar content. S.L. and Y.H. analyzed the data. S.J. revised the manuscript.
Supplemental data
The following materials are available in the online version of this article.
Supplemental Figure S1. Sugar contents of NG and NH fruits during development.
Supplemental Figure S2. Heat map of sugar transporter genes with differential expression between NG and NH fruits from the RNA-seq data and expression of PuSUT4-like.
Supplemental Figure S3. Sequence alignment of PuSUT4-like and MdSUT4-like amino acid sequences.
Supplemental Figure S4. Sequence alignment of PuSUT4-like and MdSUT4.1 amino acid sequences.
Supplemental Figure S5. Conserved domain analysis of PuSUT4-like, MdSUT4-like, and MdSUT4.1 proteins.
Supplemental Figure S6.PuSUT4-like was overexpressed in on-tree NG fruits at 120 DAFB, the infiltrated fruits were harvested 6 d after infiltration, and the glucose, fructose and sorbitol contents were measured.
Supplemental Figure S7.PuSUT4-like was silenced in on-tree NG fruits at 120 DAFB, the infiltrated fruits were harvested 6 d after infiltration, and the glucose, fructose and sorbitol contents were measured.
Supplemental Figure S8. Heat map of transcription factors with differential expression between NG and NH fruits from the RNA-seq data and expression of PuMYB12 and PuPRE6.
Supplemental Figure S9. Diagram of cis-acting elements on PuSUT4-like promoter.
Supplemental Figure S10. Expression of PuHDAC9-like in NG and NH fruits sampled in 2019 as determined by reverse transcription quantitative PCR (RT-qPCR.
Supplemental Figure S11. Sequencing alignment results of PuHDAC9-like coding sequence in NG and NH fruits.
Supplemental Figure S12. Sequencing alignment results of PuHDAC9-like promoter sequence in NG and NH fruits.
Supplemental Data Set 1. List of the primers used in this work.
Supplemental Data Set 2. Transcription factor genes for making heat maps from RNA-seq data.
Supplemental Data Set 3. Sugar transporter genes for making heat maps from RNA-seq data.
Funding
This work was supported by the National Natural Science Foundation of China (32102349), National Pear Industry Technology System Project (CARS-28-15), and the China Postdoctoral Science Foundation (2021MD703852).
Dive Curated Terms
The following phenotypic, genotypic, and functional terms are of significance to the work described in this paper:
References
Author notes
The author responsible for distribution of materials integral to the findings presented in this article in accordance with the policy described in the Instructions for Authors (https://dbpia.nl.go.kr/plphys/pages/General-Instructions) is Xinyue Li ([email protected]).
Conflict of interest statement. None declared.