-
PDF
- Split View
-
Views
-
Cite
Cite
Feifei Yi, Aosong Song, Kai Cheng, Jinlei Liu, Chenxiao Wang, Lili Shao, Shuang Wu, Ping Wang, Jiaxuan Zhu, Zhilin Liang, Ying Chang, Zongyan Chu, Chaowei Cai, Xuebin Zhang, Pei Wang, Aimin Chen, Jin Xu, David J Burritt, Luis Herrera-Estrella, Lam-Son Phan Tran, Weiqiang Li, Yingfan Cai, Strigolactones positively regulate Verticillium wilt resistance in cotton via crosstalk with other hormones, Plant Physiology, Volume 192, Issue 2, June 2023, Pages 945–966, https://doi.org/10.1093/plphys/kiad053
- Share Icon Share
Abstract
Verticillium wilt caused by Verticillium dahliae is a serious vascular disease in cotton (Gossypium spp.). V. dahliae induces the expression of the CAROTENOID CLEAVAGE DIOXYGENASE 7 (GauCCD7) gene involved in strigolactone (SL) biosynthesis in Gossypium australe, suggesting a role for SLs in Verticillium wilt resistance. We found that the SL analog rac-GR24 enhanced while the SL biosynthesis inhibitor TIS108 decreased cotton resistance to Verticillium wilt. Knock-down of GbCCD7 and GbCCD8b genes in island cotton (Gossypium barbadense) decreased resistance, whereas overexpression of GbCCD8b in upland cotton (Gossypium hirsutum) increased resistance to Verticillium wilt. Additionally, Arabidopsis (Arabidopsis thaliana) SL mutants defective in CCD7 and CCD8 putative orthologs were susceptible, whereas both Arabidopsis GbCCD7- and GbCCD8b-overexpressing plants were more resistant to Verticillium wilt than wild-type (WT) plants. Transcriptome analyses showed that several genes related to the jasmonic acid (JA)- and abscisic acid (ABA)-signaling pathways, such as MYELOCYTOMATOSIS 2 (GbMYC2) and ABA-INSENSITIVE 5, respectively, were upregulated in the roots of WT cotton plants in responses to rac-GR24 and V. dahliae infection but downregulated in the roots of both GbCCD7- and GbCCD8b-silenced cotton plants. Furthermore, GbMYC2 suppressed the expression of GbCCD7 and GbCCD8b by binding to their promoters, which might regulate the homeostasis of SLs in cotton through a negative feedback loop. We also found that GbCCD7- and GbCCD8b-silenced cotton plants were impaired in V. dahliae-induced reactive oxygen species (ROS) accumulation. Taken together, our results suggest that SLs positively regulate cotton resistance to Verticillium wilt through crosstalk with the JA- and ABA-signaling pathways and by inducing ROS accumulation.
Introduction
Verticillium wilt, caused by Verticillium dahliae (Sal’kova and Guseva 1965), is a serious soil-borne fungal disease affecting the growth and development of cotton (Gossypium spp.) plants (Cai et al. 2009; Song et al. 2020; Cai et al. 2021; Dadd-Daigle et al. 2021; Zhang et al. 2022). To date, there are no effective fungicides available to prevent V. dahliae infection, and germplasm collections of the susceptible cultivar upland cotton (Gossypium hirsutum) appear to contain little genetic resistance to V. dahliae (Wang et al. 2016b; Zhang et al. 2017, 2020). Transgenic approaches have been successful in providing resistance to pathogenic fungi and insects, and to improve fiber quality of cotton plants (Ribeiro et al. 2017; Long et al. 2018; Tong et al. 2021).
Previous studies indicated that several phytohormones are involved in plant resistance to V. dahliae infection, including jasmonic acid (JA) (Cai et al. 2009; Song et al. 2020; Dadd-Daigle et al. 2021). JA is a well-known phytohormone that positively enhances pathogen resistance in plants (Howe et al. 2018; Li et al. 2019; Yang et al. 2019). In response to pathogen infection, isoleucine conjugate of JA (JA-Ile) accumulates and binds to the F-box protein CORONATINE INSENSITIVE 1 (COI1) receptor (Fonseca et al. 2009; Sheard et al. 2010). COI1, which binds JA-Ile, is associated with the S-PHASE KINASE ASSOCIATED PROTEIN 1 (SKP1) and CULLIN to form the SCFCOI1 complex (Wasternack and Strnad 2018; Gupta et al. 2021). The SCFCOI1 complex then recruits JASMONATE-ZIM-DOMAIN (JAZ) repressor proteins for ubiquitination and induces the degradation of the JAZ repressors via the 26S proteasome (Chini et al. 2007; Thines et al. 2007). JAZ proteins repress the activities of several transcription factors (TFs), such as MYELOCYTOMATOSIS 2 (MYC2), which activates the downstream JA-responsive gene expression (He et al. 2018; Wasternack and Strnad 2018; Li et al. 2019). Upon degradation of the JAZ proteins, MYC2 positively regulates the expression of the JA-responsive genes like PLANT DEFENSE FACTOR 1.2 (PDF1.2) and VEGETATIVE STAGE PROTEIN 2 (VSP2) (Chini et al. 2007; Pauwels et al. 2010). In upland cotton, virus-induced gene silencing (VIGS) of the CALCIUM-DEPENDENT PROTEIN KINASE 33 (GhCPK33) gene, a negative regulator of JA biosynthesis, leads to constitutively activated JA biosynthesis and enhanced resistance to V. dahliae infection (Hu et al. 2018). Overexpression of the repressor GhJAZ2 in cotton impairs plant responses to JA, by preventing the transcriptional activation of JA-responsive genes (e.g. GhPDF1.2 and GhVSP), which results in an increase in the susceptibility of transgenic cotton plants to insect herbivory and V. dahliae (He et al. 2018). Salicylic acid (SA)-mediated defense pathway has both synergistic and antagonistic effects with JA-mediated defense pathways (Zhang et al. 2018; Wang et al. 2020a). Accumulation of SA is perceived by NONEXPRESSOR OF GENES 1 (NPR1) and NPR1-LIKE PROTEIN 3 (NPR3)/NPR4, which contain a transactivation domain and do not contain DNA binding domain. NPR1 and NPR3/NPR4 receptors exert their transcriptional activity by interacting with TFs of the TGACG SEQUENCE-SPECIFIC BINDING PROTEIN family to activate SA-mediated responses (Zhou et al. 2000; Wu et al. 2012). In the resistant cultivar island cotton (Gossypium barbadense), the ENHANCED DISEASE SUSCEPTIBILITY 1 (GbEDS1) gene encodes a lipase-like protein that was shown to play a positive role in resistance to V. dahliae by regulating SA levels and H2O2 accumulation. Ectopic expression of GbEDS1 in Arabidopsis (Arabidopsis thaliana) leads to reduced expression of NPR1 and PATHOGENESIS RELATED PROTEIN1/5 (PR1/5) genes (Yan et al. 2016). Moreover, the NUCLEOTIDE-BINDING SITE LEUCINE-RICH REPEAT (NBS-LRR) gene GbaNA1 is induced by V. dahliae and by the phytohormones ethylene (ET), JA, and SA in island cotton, suggesting a link between the signaling pathways of these phytohormones in the cotton response to V. dahliae (Li et al. 2018a).
Reactive oxygen species (ROS) and the phenylpropanoid metabolic pathway both play pivotal roles in regulating plant growth, development, and responses to biotic and abiotic stresses (Torres and Dangl 2005; Kapulnik et al. 2011a; Suzuki et al. 2011; Guo et al. 2016; Zhang et al. 2019). Overexpression of RESPIRATORY BURST OXIDASE HOMOLOGS D (GhRbohD) or GbRboh5/18 encoding plant-specific NADPH oxidases in cotton enhanced plant resistance to V. dahliae by inducing ROS accumulation (Chang et al. 2020; Huang et al. 2021). In addition, cell wall modification through synthesis of lignin (increased lignification) and accumulation of antimicrobial compounds, which are the downstream products of the phenylpropanoid metabolic pathway, has been widely reported to participate in enhancing cotton resistance to V. dahliae (Naoumkina et al. 2010; Kapulnik et al. 2011a; Guo et al. 2016; Zhang et al. 2019). In a previous study, we showed that silencing the Gossypium australe CAROTENOID CLEAVAGE DIOXYGENASE 7 (GauCCD7) gene, which is potentially involved in the biosynthesis of strigolactones (SLs), decreased Verticillium wilt resistance, suggesting that SLs may positively regulate cotton resistance to V. dahliae (Cai et al. 2020). However, the SL-modulated mechanisms underlying Verticillium wilt resistance in cotton remain to be elucidated.
SLs are carotenoid-derived phytohormones that were originally isolated from cotton root exudates as a germination stimulant of Striga lutea (Cook et al. 1966). Later investigations revealed that SLs also function in promoting hyphal branching of arbuscular mycorrhizal fungi (Akiyama et al. 2005). The SL-biosynthetic precursor carlactone (CL) is derived from trans-β-carotene via a series of reactions by the isomerase DWARF 27 (D27), CCD7 and CCD8 in Arabidopsis plastids (Alder et al. 2012). CL is then transported into the cytoplasm, converted to carlactonoic acid by MORE AXILLARY GROWTH 1 (MAX1, a cytochrome P450 monooxygenase), and eventually converted to SLs in Arabidopsis (Mashiguchi et al. 2021). On the other hand, in Lotus (Lotus japonicus), CL is converted to 18-hydroxycarlactonoic acid by a MAX1 homolog, LjMAX1 (Mori et al. 2020), while it is converted to orobanchol in rice (Oryza sativa) via two P450 enzymes, Os1G0700900/CYP711A2 and Os1G0701400/CYP711A3 (Zhang et al. 2014). In terms of signal transduction, in rice, SLs are perceived by the receptor D14 (an α/β hydrolase) (Arite et al. 2009; Gaiji et al. 2012). Interaction of SL-bound D14 with D3 (an F-box protein) (Ishikawa et al. 2005) triggers the ubiquitin ligase complex to target D53 suppressor for degradation in rice (Jiang et al. 2013; Zhou et al. 2016; Fang et al. 2020). D53 in rice and its orthologous SUPPRESSOR OF MAX2-LIKE 6 (SMXL6), 7, and 8 in Arabidopsis have been reported to have transcriptional repression activities through recruitment of core repressor TOPLESS proteins, which contain the ET-responsive element binding factor-associated amphiphilic repression motif (Jiang et al. 2013; Soundappan et al. 2015; Wang et al. 2020b). Recent investigations indicated that SMXL6 also functions as an auto-regulated TF in repression of the expression of SMXL6, 7, and 8. Furthermore, SMXL6 can act as a transcriptional co-repressor to repress the expression of BRANCHED 1 (BRC1) and PRODUCTION OF ANTHOCYANIN PIGMENT 1 (PAP1) by interaction with TOPLESS (TPL)/TPL RELATED (TPR) proteins (Wang et al. 2020b). BRC1 and PAP1 are known to participate in shoot branching and anthocyanin biosynthesis in Arabidopsis, respectively (Borevitz et al. 2000; Gonzalez-Grandio et al. 2013). Another independent study reported that D53 interacts with BRASSINAZOLE RESISTANT 1 (OsBZR1) to inhibit the expression of FINE CULM1 (a rice ortholog of the Arabidopsis BRC1), resulting in reduced tillering in rice (Fang et al. 2020).
As a class of phytohormones, SLs play various roles in plant growth, development, and tolerance and resistance to abiotic and biotic stresses (Kapulnik et al. 2011b; Ha et al. 2014; Visentin et al. 2016; Ma et al. 2017; Xiong et al. 2020). With respect to plant growth and development, SLs inhibit lateral shoot branching (Gomez-Roldan et al. 2008; Umehara et al. 2008), promote primary root growth, and suppress lateral root development/adventitious root formation in Arabidopsis under normal growth conditions (Kapulnik et al. 2011a; Ruyter-Spira et al. 2011). In rice, SLs inhibit tiller outgrowth and mesocotyl elongation in darkness (Umehara et al. 2008; Hu et al. 2014). Regarding environmental stresses, SLs positively regulate plant responses to salt and drought stresses (Ha et al. 2014; Li and Tran 2017; Xiong et al. 2020), and enhance resistance of Arabidopsis to leafy gall syndrome as a result of infection with the biotrophic actinomycete Rhodococcus fascians (Stes et al. 2015). SL signaling also enhances resistance of tomato (Solanum lycopersicum) to the necrotrophic foliar fungal pathogens Botrytis cinerea and Alternaria alternata (Torres-Vera et al. 2014; Xu et al. 2019), and to root-knot nematodes (RKNs) (Lahari et al. 2019). This effect is associated with variations in the levels of JA, abscisic acid (ABA), and SA (Torres-Vera et al. 2014; Xu et al. 2019). However, SLs enhance the susceptibility of rice to RKN infection by antagonizing the JA pathway (Lahari et al. 2019), suggesting that SLs may have different roles for RKN resistance in different plant species.
Here, we deeply investigated the role of SLs in cotton resistance to V. dahliae using several approaches. First, we showed that exogenous application of the SL analog rac-GR24 or overexpression of the SL-biosynthetic GbCCD8b gene increased cotton Verticillium wilt resistance. Second, in support of the gain-of-function results, we found that the suppression of SL biosynthesis using a synthetic SL inhibitor or by knocking down the SL genes GbCCD7 and GbCCD8b decreased resistance of cotton plants to V. dahliae infection. Several genes related to JA-, SA-, and ABA-signaling pathways were found to be affected by rac-GR24 treatment after V. dahliae infection. Furthermore, we found that the MYC2 TF suppresses the expression of GbCCD7 and GbCCD8b genes, suggesting its role in regulating the SL homeostasis in cotton. We also discovered that knock-down of GbCCD7 and GbCCD8b decreased ROS accumulation in the leaves of silenced cotton plants. Together, the results of this study suggest that SLs positively regulate cotton defense against Verticillium wilt through crosstalk with other hormone signaling pathways, a MYC2-mediated negative feedback loop, and by inducing the accumulation of ROS.
Results
Expression of CCD7 and CCD8 were induced by V. dahliae infection in cotton roots
To understand the function of SLs in the response of cotton plants to V. dahliae infection, we first analyzed the homologous gene structures of CCD7 and CCD8 in both island cotton Hai7124 and upland cotton Jimian11. There are two homologous genes of CCD7 (CCD7-A12 and CCD7-D12), and four homologous genes of CCD8, namely CCD8a (CCD8a-A06 and CCD8a-D06) and CCD8b (CCD8b-A07 and CCD8b-D07) in each of the two cotton varieties, and the structures (exons/introns) of these homologs are highly similar (Figure 1A). The expression patterns of the two SL biosynthesis-related genes, CCD7 and CCD8a/b, were analyzed in different organs of the two cotton varieties. GbCCD7/GhCCD7 and GbCCD8b/GhCCD8b were most highly expressed in the roots, while GbCCD8a/GhCCD8a were most highly expressed in the stems of both cultivars (Figure 1, B and C).
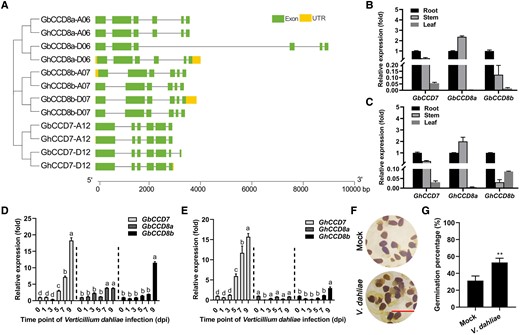
The SL biosynthesis-related CCD7 and CCD8a/b homologous genes and their expression patterns in island Hai7124 and upland Jimian11 cotton cultivars with or without V. dahliae infection. A) The phylogenetic tree and exon/intron structures of CCD7 and CCD8 (CCD8a and CCD8b). The phylogenetic tree was constructed by the NJ method, with 1,000 bootstrap replicates. The capital letters A and D stand for the sub-genomes originated from two diploid progenitor species Gossypium arboreum (A-genome donor) and G. raimondii (D-genome donor), respectively. The numbers next to the capital A/D letters stand for chromosome numbers. The green boxes indicate exons, the yellow boxes indicate untranslated regions, and the gray lines represent introns. B and C) The expression patterns of GbCCD7 and GbCCD8a/b (B), and GhCCD7 and GhCCD8a/b (C) in different organs of island (Hai7124, B) and upland (Jimian11, C) cotton varieties. Data are means ± SD of three biological replicates (n = 3; three independent plants/biological replicate). The expression value in roots was normalized as 1. D and E) The transcript levels of GbCCD7 and GbCCD8a/b in the roots of island cotton (D), and GhCCD7 and GhCCD8a/b in the roots of upland cotton (E) after 0, 1, 3, 5, 7, and 9 dpi with V. dahliae infection. Data are means ± SD of three biological replicates, and value of 0 dpi was normalized as 1 (n = 3; three independent plants/biological replicate). Different letters indicate statistically significant differences in expression levels of each gene between before treatment (0 dpi) and after treatment (1,3,5, 7, and 9 dpi) with V. dahliae as determined by a one-way ANOVA analysis using the Tukey's honestly significant difference (HSD) test (P < 0.05). F and G) Representative pictures of O. aegyptiaca seed germination (F) and germination percentages (G) under the treatments with the extracts from roots of V. dahliae-uninfected (Mock) and V. dahliae-infected plants (Hai7124) at 9 dpi. Data are means ± SD of three biological replicates (n = 3; ≥30 seeds/biological replicate). Bar = 5 mm. Asterisks indicate statistically significant differences between Mock and V. dahliae-infected plants as determined by a Student's t-test (**P < 0.01). dpi, day-post-inoculation.
Because the root is the first organ affected by V. dahliae infection, we mainly focused on roots in the following experiments. After the application of V. dahliae spore suspension (1 × 107 spores mL−1) to the soil, the expression levels of GbCCD7, GhCCD7, GbCCD8a/b, and GhCCD8a/b were measured in the roots of both Hai7124 and Jimian11 plants, respectively. In both cotton varieties, the expression levels of CCD7 and CCD8b began to increase at 5 and 9 d-post-inoculation (dpi), respectively (Figure 1, D and E). The transcript levels of CCD8a showed a slight increase after 5 dpi in the resistant Hai7124 (Figure 1D), but remained unchanged in the susceptible Jimian11 during the entire course of treatment (Figure 1E). After 9 dpi, the transcript levels of GbCCD7 and GbCCD8b were 15- and 18-fold higher, respectively, in Hai7124, while those of GhCCD7 and GhCCD8b were 3- and 11-fold higher, respectively, in Jimian11, when compared with transcript levels at 0 dpi (Figure 1, D and E). A seed germination assay of parasitic Orobanche aegyptiaca, which is very sensitive to SLs (Cook et al. 1966), was then performed to compare the SL contents in the cotton root extracts from the resistant Hai7124 with and without V. dahliae infection. Results revealed that the germination percentage of O. aegyptiaca seeds treated with the root extract from V. dahliae-infected plants was higher than that of O. aegyptiaca seeds treated with root extract from V. dahliae-uninfected plants (Mock) (Figure 1, F and G). These results suggest that V. dahliae infection induced the accumulation of endogenous SLs in the roots of cotton plants, most probably through upregulating the expression of the SL-biosynthetic genes CCD7 and CCD8b, and that the higher V. dahliae resistance of the island Hai7124 cultivar than the upland Jimian11 might be associated with the higher expression levels of CCD7 and CCD8a/b at 9 dpi.
Exogenous applications of rac-GR24 and the SL biosynthesis inhibitor TIS108 increased cotton Verticillium wilt resistance and susceptibility, respectively
To explore the function of SLs in Verticillium wilt resistance in cotton, 14-d-old seedlings of susceptible Jimian11 were subjected to soil drenching with the SL analog rac-GR24 (3 μM) or Mock control (water containing an equal amount of ethanol) plants for 24 h prior to inoculation with V. dahliae spores. The application of rac-GR24 or Mock control was conducted twice per week during the infection period to maintain the effect of rac-GR24. Plants disease indices were evaluated at 21 dpi, and the results showed that exogenous application of rac-GR24 alleviated V. dahliae-induced leaf wilting and leaf abscission, compared with the Mock control plants (Figure 2A), and that the disease index value of rac-GR24-treated plants was lower than that of the Mock control plants (Figure 2B). In addition, the brown disease associated bands in the vascular tissues of stem sections of rac-GR24-treated plants were smaller and lighter (Figure 2C), and the lesion area percentages in the stem sections of rac-GR24-treated plants were significantly lower than those of Mock control plants (Figure 2D). A fungal renewal cultivation assay showed that the stem sections from rac-GR24-treated plants had less fungal growth than those of Mock control plants (Figure 2E). These results indicated that exogenous application of rac-GR24 enhanced cotton resistance to V. dahliae. Synchronously, we detected the transcript levels of CCD7 and CCD8b in the roots of both susceptible Jimian11 and resistant Hai7124 following treatment with rac-GR24. We found that the transcript levels of these two genes were dramatically reduced at 3 and 6 h post rac-GR24 treatment, and then their transcript levels (GhCCD7 and GhCCD8b) were recovered in the susceptible Jimian11 plants and were even upregulated (GbCCD7 and GbCCD8b) in the resistant Hai7124 plants (Figure 2, F and G).
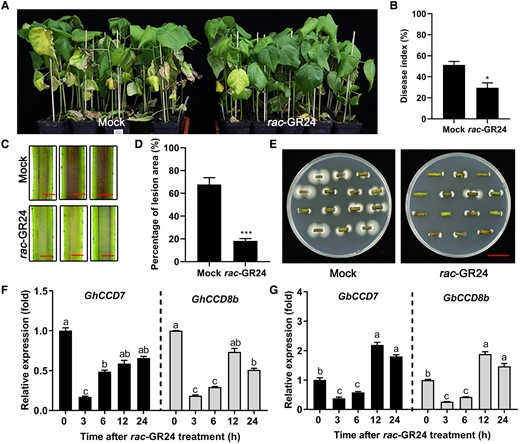
Exogenous application of rac-GR24 enhanced cotton resistance to V. dahliae. A) Disease symptoms of Jimian11 (susceptible cultivar) seedlings treated with 3 µM rac-GR24 and without rac-GR24 (Mock, water control containing equal amount of ethanol) for 24 h, and then exposed to V. dahliae infection for 21 d. The application of rac-GR24 (3 μM) or Mock control was conducted twice per week during the infection period to maintain the effect of rac-GR24. B–E) Disease index (B), stem section anatomy (C), lesion area of stem section (D), and fungal renewal cultivation assay of the stem cuttings (E) from Jimian11 seedlings shown in (A). Data are means ± SD of three biological replicates (n = 3; ≥25 stem cuttings/biological replicate). Bar = 2 mm (C). Bar = 2 cm (E). Asterisks indicate statistically significant differences between Mock and V. dahliae-infected plants as determined by a Student's t-test (*P < 0.05 and ***P < 0.001). F and G) Relative transcript levels of CCD7 and CCD8b in Jimian11 (F) and Hai7124 plants (G) after their treatment with 3 µM rac-GR24 for 0, 3, 6, 12, and 24 h. Data are means ± SD of three biological replicates (n = 3; three independent plants/biological replicate). The expression value at 0 h was normalized as 1. Different letters indicate statistically significant differences between before treatment (0 h) and after treatment (3, 6, 12, 24 h) with rac-GR24 as determined by a one-way ANOVA analysis using the Tukey's HSD test (P < 0.05).
To further investigate whether endogenous SL levels were linked to cotton defense against Verticillium wilt, the SL biosynthesis inhibitor TIS108 was used (Ito et al. 2011). Resistant Hai7124 cotton seedlings with three or four true leaves were soil-drenched with 3 μM TIS108, which resulted in a remarkable downregulation of GbCCD7 and GbCCD8b at the initial time of 1, 3, and 6 h, and then showed a recovery tendency of GbCCD8b or even an upregulation of GbCCD7 (Supplemental Figure S1A), indicating a positive feedback regulation. Subsequently, the germination assay of O. aegyptiaca seeds was carried out to evaluate the SL contents in the cotton root extracts from the resistant Hai7124 cotton plants. Results revealed that the germination percentage of O. aegyptiaca seeds treated with root extracts from plants processed with TIS108 for 3 d was lower than that of O. aegyptiaca seeds treated with root extracts from Mock control plants (Supplemental Figure S1, B and C). These findings indicate that TIS108 treatment inhibited SL biosynthesis in cotton roots. To identify the effect of TIS108 treatment on cotton resistance to V. dahliae, we applied 5 mL of TIS108 (3 μM) solution or the same amount of water (containing an equal amount of ethanol) for 24 h prior to the inoculation with V. dahliae spores. The application of TIS108 or Mock control was conducted twice per week during the infection period to maintain the effect of TIS108. Three weeks after the V. dahliae infection, Hai7124 cotton seedlings showed more severe disease symptoms in plants treated with TIS108 compared with the Mock control plants (Supplemental Figure S1D). The disease index was elevated by ∼20% in TIS108-treated plants compared with the control (Supplemental Figure S1E). Additional assays showed that in comparison with the Mock control plants, the TIS108-treated cotton seedlings had deeper brown spots and higher lesion areas in the vascular tissues of the stems (Supplemental Figure S1, F and G), and their stem sections had more growth of V. dahliae (Supplemental Figure S1H). These results indicate that the inhibition of SL biosynthesis by TIS108 treatment reduced the resistance of cotton plants to Verticillium wilt. Furthermore, data from a disk diffusion assay showed that neither rac-GR24 nor TIS108 directly affected the growth of V. dahliae (Supplemental Figure S1I). In summary, these results demonstrated that the exogenous application of rac-GR24 decreased, while that of SL biosynthesis inhibitor TIS108 increased the susceptibility of cotton plants to V. dahliae infection, implying a positive role of SLs in the regulation of cotton resistance to Verticillium wilt.
Knock-down of GbCCD7 or GbCCD8b decreased endogenous SLs and Verticillium wilt resistance
Because rac-GR24 is a mixture of two isomers and mimic the functions of both SLs and karrikins (KARs) in terms of plant growth and development (De Cuyper et al. 2017), it was necessary to use SL-specific mutants to study the role of SLs in the regulation of cotton resistance to Verticillium wilt. First, the model plant Arabidopsis was selected to confirm the function of SLs in resistance to V. dahliae by using the SL-biosynthetic mutants max3-11 and max4-8, which are defected in the MAX3/AtCCD7 and MAX4/AtCCD8 putative orthologs, respectively. After the application of GFP-labeled V. dahliae (V991-GFP), more severe disease symptoms were observed in the max3-11 and max4-8 mutant plants compared with wild-type (WT) plants (Supplemental Figure S2, A and B). Stronger green fluorescence and more V. dahliae hyphal growth were noted in max3-11 and max4-8 mutant plants than in WT plants (Supplemental Figure S2C). These results illustrated that SL biosynthesis-related genes MAX3/AtCCD7 and MAX4/AtCCD8 play key roles in Arabidopsis resistance to Verticillium wilt.
Next, to assess the function of SLs in cotton resistance to Verticillium wilt, we individually knocked down GbCCD7, GbCCD8a, and GbCCD8b in the resistant Hai7124 cotton plants using the VIGS method. The expression of GbCCD7, GbCCD8a, and GbCCD8b were confirmed to be downregulated in TRV:GbCCD7, TRV:GbCCD8a, and TRV:GbCCD8b plants, respectively (Figure 3, A and B and Supplemental Figure S3A). To confirm whether the SL contents decreased in TRV:GbCCD7, TRV:GbCCD8a, and TRV:GbCCD8b plants, we used the O. aegyptiaca seed germination assay to estimate SL abundance in root extracts from TRV:GbCCD7, TRV:GbCCD8a, and TRV:GbCCD8b cotton plants. The germination percentages of O. aegyptiaca seeds were reduced by treatment with the extracts from TRV:GbCCD7 and TRV:GbCCD8b cotton plants, but not with that from TRV:GbCCD8a plants, relative to that of control TRV:00 plants (Figure 3, C and D and Supplemental Figure S3, B and C). These results indicated that the SL contents decreased in TRV:GbCCD7 and TRV:GbCCD8b cotton plants following the silencing of the respective genes. The CHLOROPLASTOS ALTERADOS (GbCLA) gene was also silenced as a marker to monitor the efficiency of VIGS (Gao et al. 2017), and the albino phenotype of the newly emerged true leaves on the TRV:GbCLA plants indicated that the VIGS system was properly working (Figure 3E and Supplemental Figure S3D).
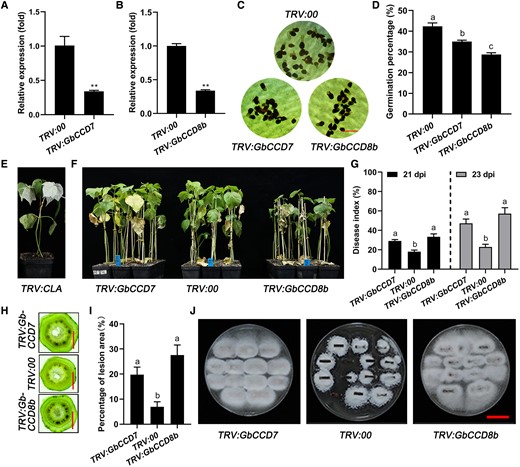
Silencing the SL-biosynthetic genes GbCCD7 and GbCCD8b reduced resistance to V. dahliae in cotton. A and B) Expression of GbCCD7 and GbCCD8b in roots of GbCCD7-silenced (TRV:GbCCD7) and GbCCD8b-silenced (TRV:GbCCD8b) Hai7124 cotton plants. Data are means ± SD of three biological replicates (n = 3; three independent plants/biological replicate). The expression value of TRV:00 was normalized as 1. Asterisks indicate statistically significant differences between TRV:00 and TRV:GbCCD7 or TRV:GbCCD8b plants as determined by a Student's t-test (**P < 0.01). C and D) Representative pictures of O. aegyptiaca seed germination (C) and germination percentages (D) under the treatments with the extracts from roots of TRV:00 (control), TRV:GbCCD7, and TRV:GbCCD8b plants. Bar = 5 mm. Data are means ± SD of three biological replicates (n = 3; ≥30 seeds/biological replicate). E) Albino phenotype of the cotton plants inoculated with TRV:GbCLA vector after 14 d. F) Disease symptoms of TRV:00, TRV:GbCCD7, and TRV:GbCCD8b seedlings at 21 dpi with V. dahliae. G–J) Disease indices (G), stem anatomy (H), lesion area percentages (I), and fungal renewal cultivation levels (J) in TRV:00, TRV:GbCCD7, and TRV:GbCCD8b cotton plants shown in (F). Bar = 2 mm (stem anatomy), Bar = 2 cm (fungal renewal cultivation). Data are means ± SD of three biological replicates (n = 3; ≥25 stem cuttings/biological replicate). Different letters indicate statistical differences among different genotypes as determined by a one-way ANOVA analysis using the Tukey's HSD test (P < 0.05). dpi, day-post-inoculation.
After the inoculation with V. dahliae, both TRV:GbCCD7 and TRV:GbCCD8b, but not TRV:GbCCD8a plants, showed more severe Verticillium wilt-related disease symptoms compared with control TRV:00 plants (Figure 3F and Supplemental Figure S3, E and F). Consistent with their more severe disease symptoms, higher disease index values were recorded for TRV:GbCCD7 and TRV:GbCCD8b plants compared with those of TRV:00 plants (Figure 3G). It is worth noting that the TRV:GbCCD8b plants exhibited more severe wilting, etiolation, and defoliation, as well as a higher disease index value than TRV:GbCCD7 plants, but the differences in disease index were not statistically significant (Figure 3, F and G). Additionally, the TRV:GbCCD7 and TRV:GbCCD8b cotton plants had deeper brown spots and higher lesion areas in the vascular tissues of their stems and more growth of V. dahliae, in comparison with TRV:00 plants (Figure 3, H–J). These results illustrated that silencing either the GbCCD7 or GbCCD8b gene in cotton plants increased their susceptibility to V. dahliae infection.
Overexpression of GbCCD7 or GbCCD8b enhanced cotton and Arabidopsis Verticillium wilt resistance
To further support the functions of GbCCD7 and GbCCD8b in resistance to V. dahliae, first, we generated Arabidopsis transgenic lines ectopically expressing GbCCD7 or GbCCD8b using the CaMV35S promoter. Three independent GbCCD7-overexpressing lines (L14, L31 and L34) and three independent GbCCD8b-overexpressing lines (L1, L12 and L44) were selected for further experiments. The transcript levels and the levels of the proteins (enzymes) encoded by these genes were verified via reverse transcription quantitative real-time polymerase chain reaction (RT-qPCR) and western blotting, respectively (Supplemental Figure S4, A–D), which confirmed the increased expression levels of both genes and accumulation of their corresponding enzymes in the transgenic plants. Disease symptoms in the GbCCD7- and GbCCD8b-overexpressing lines were found to be less severe than in the WT plants at 15 dpi (Supplemental Figure S4, E and F). In addition, by observing the level of green fluorescence after the GFP-labeled V. dahliae V991 infection of the roots, we found that the transgenic lines had lower fungal colonization rates and less fungal hyphal growth than WT plants (Supplemental Figure S4G). These results indicated that ectopic expression of either GbCCD7 or GbCCD8b in Arabidopsis increased Verticillium wilt resistance of transgenic plants, suggesting a positive role for both GbCCD7 and GbCCD8b in response to V. dahliae infection in Arabidopsis.
Because we observed a tendency of more severe wilting of GbCCD8b-silenced than GbCCD7-silenced cotton plants in response to V. dahliae infection (Figure 3, F–J), next, we generated transgenic cotton lines overexpressing GbCCD8b (driven by the CaMV35S promoter) in Baimian1 (a susceptible upland cotton cultivar) to test its effect on cotton resistance to V. dahliae infection. Four T1 transgenic lines (L1, L3, L14, and L15) were verified via PCR (Figure 4A), and the overexpression of GbCCD8b in transgenic plants was confirmed at the transcript level using RT-qPCR and the protein level using western blotting (Figure 4, B and C). Due to the particularly high GbCCD8b expression levels in L1, L14, and L15 plants, these three lines were selected for further phenotypic analyses. To estimate the SL abundance in roots of the transgenic lines, O. aegyptiaca seed germination assay was conducted. Increased germination percentages of O. aegyptiaca seeds by the treatment with the extracts from overexpression transgenic cotton plants were observed relative to that of WT plants (Figure 4, D and E). After V. dahliae infection, disease symptoms were evaluated, and the transgenic plants showed significantly lower disease indices than WT plants (Figure 4, F and G). Stems were dissected to observe the lesion areas at 23 dpi, and the transgenic cotton lines had fewer spots than the WT plants (Figure 4, H and I). These results collectively indicated that overexpression of GbCCD8b in cotton plants enhanced their resistance to V. dahliae.
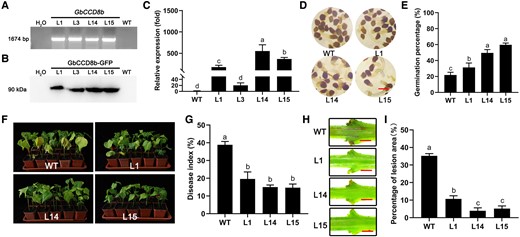
Overexpression of GbCCD8b-GFP enhanced V. dahliae resistance in susceptible upland cotton Baimian1. A) Direct-PCR verification of GbCCD8b-GFP using genomic DNA from WT and overexpression transgenic lines (L1, L14, and L15) plants. H2O represents negative control in the PCR system. B) Western blotting was used to detect GbCCD8b-GFP in WT and transgenic (L1, L14, and L15) plants. Primary antibody of anti-GFP mouse monoclonal antibody and the secondary antibody of hypersensitive response and patho (HRP)-conjugated goat anti-mouse IgG were used. C) The transcript levels of GbCCD8b-GFP in WT and transgenic plants. Data are means ± SD of three biological replicates (n = 3; three independent plants/biological replicate). The expression value of WT was normalized as 1. D and E) Representative pictures of O. aegyptiaca seed germination (D) and germination percentages (E) under the treatments with the extracts from roots of WT and transgenic plants. Data are means ± SD of three biological replicates (n = 3; ≥30 seeds/biological replicate). Bar = 1 mm. F–I) Disease symptoms (F), disease indices (G), browning spots of the dissected stems (H), and lesion area percentages of stem section (I) of the WT and transgenic plants at 23 dpi with V. dahliae. Bar = 2 mm. Data are means ± SD of three biological replicates (n = 3; ≥25 stem cuttings/biological replicate). Different letters indicate statistical differences among different genotypes as determined by a one-way ANOVA analysis using the Tukey's HSD test (P < 0.05). dpi, day-post-inoculation.
Mechanistic insights into the influences of rac-GR24 treatment and GbCCD7 deficiency on V. dahliae resistance in cotton roots using transcriptomics
To explore the molecular mechanisms underpinning the influences of rac-GR24 application and role of GbCCD7 on V. dahliae resistance in cotton roots, two sets of RNA sequencing (RNA-seq) experiments were conducted. First, to study the effect of rac-GR24, samples were collected from cotton roots with or without infection with V. dahliae and with or without treatment with rac-GR24 as previously described for phenotypic investigation (Figure 2A). Thus, the following samples rac-GR24-treated V. dahliae-infected roots (GV), rac-GR24-treated uninfected roots (G), Mock V. dahliae-infected roots (V), and Mock uninfected roots (M) were obtained for comparative transcriptome analysis. The data of the transcriptomics are available from the National Center for Biotechnology Information (NCBI) Gene Expression Omnibus under the accession number PRJNA812956, and the results are shown in Supplemental Table S1. Differentially expressed genes (DEGs) were defined as those with more than 1.5-fold change in gene expression (|fold change| > 1.5 and P-value < 0.05), and the lists of identified DEGs are provided in Supplemental Table S2. In the “G vs. M” comparison, 597 genes were upregulated and 433 were downregulated (Figure 5A and Supplemental Table S2, A and B). In the “GV vs. V” comparison, there were 447 upregulated and 393 downregulated genes (Figure 5A and Supplemental Table S2, E and F). There was a total of 413 DEGs that are overlapping between the “GV vs. V” and “GV vs. G” comparisons (Figure 5B and Supplemental Table S2I). Gene ontology (GO) and Kyoto Encyclopedia of Genes and Genomes (KEGG) enrichment analyses were conducted on the DEGs obtained from the “GV vs. V” comparison to understand the role of rac-GR24 on V. dahliae resistance, using the Metascape (http://metascape.org). The upregulated genes were highly enriched in the terms “regulation of phenylpropanoid metabolic process”, “hormone-mediated signaling pathway”, “regulation of hormone levels”, “response to ABA”, and “plant hormone signal transduction” (Figure 5C and Supplemental Table S3A), while the downregulated genes were highly enriched in “JA mediated signaling pathway”, “defense responses to bacteria”, “hormone metabolic process”, and other plant immunity-related processes (Figure 5D and Supplemental Table S3B). Several genes were selected for verification using RT-qPCR (Supplemental Figure S5A). Consistent with the RNA-seq data, ABA-INSENSITIVE 5 (ABI5) and PYRABACTIN RESISTANCE1-LIKE 4 (PYL4), members of the ABA-signaling pathway (Chen et al. 2020), were upregulated in cotton plants treated with rac-GR24 alone or with both rac-GR24 and V. dahliae (Figure 5E). Likewise, the genes CINNAMYL ALCOHOL DEHYDROGENASE 9 (CAD9) and MYELOBLASTOSIS 4 (MYB4), which are involved in phenylpropanoid metabolic processes (Chezem et al. 2017; Muro-Villanueva et al. 2022), were also induced by rac-GR24 alone or when rac-GR24 and V. dahliae were combined in the treatment (Figure 5E). These results demonstrated that ABA signaling and phenylpropanoid metabolic processes were induced by rac-GR24 and are presumably involved in plant immunity.
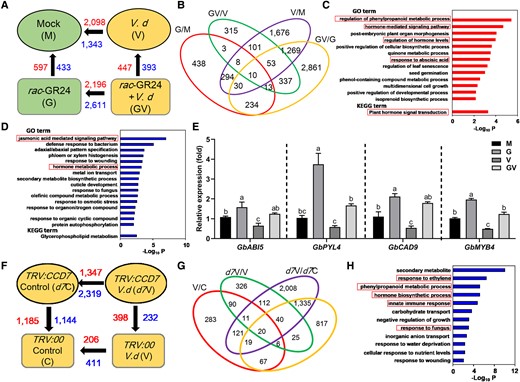
Transcriptome analysis of cotton roots to gain mechanistic insights into SL-mediated V. dahliae resistance. A) Numbers of DEGs of different groups compared in pairs. The red numbers indicate the upregulated DEGs and the blue numbers indicate downregulated DEGs. Mock (M), cotton roots without the applications of V. dahliae and rac-GR24; V. d (V), cotton roots with V. dahliae infection; rac-GR24 (G), cotton roots with rac-GR24 treatment; rac-GR24 + V. d (GV), cotton roots with both V. dahliae and rac-GR24 treatments. B) Venn diagram of DEGs among the groups. Numbers indicate overlapping and non-overlapping DEGs among the groups. C and D) Enrichment analyses of the upregulated (C, 447 genes) and downregulated (D, 393 genes) genes from the “GV vs. V” comparison using GO biological process and KEGG pathway analyses. The X-axis is the accumulative hypergeometric P-values of genes mapped by the terms, representing the abundance of the GO or KEGG terms. E) Relative expression levels of the upregulated (selected from GO terms) in roots of cotton plants treated with or without infection with V. dahliae for 3 d (3 dpi) and with or without treatment with rac-GR24 for 4 d. Data are means ± SD of three biological replicates (n = 3; three independent plants/biological replicate). The expression values of M were normalized as 1. Different letters indicate statistically significant differences in expression levels of each gene among the groups (M, G, V, and GV) as determined by a one-way ANOVA analysis using the Tukey's HSD test (P < 0.05). F) Numbers of DEGs of different groups compared in pairs. TRV:GbCCD7 Control (d7C), TRV:GbCCD7 cotton roots without V. dahliae infection; TRV:00 V. d (V), TRV:00 cotton roots with V. dahliae infection; TRV:00 Control (C), TRV:00 cotton roots without V. dahliae infection; TRV:GbCCD7 V. d (d7V), TRV:GbCCD7 cotton roots with V. dahliae infection. G) Venn diagram of DEGs among the groups. Numbers indicate overlapping and non-overlapping DEGs among the groups. H) GO enrichment analysis of downregulated genes obtained from “d7V vs. V” comparison. Data are means ± SD of three biological replicates (n = 3; three independent plants/biological replicate).
As mentioned earlier, rac-GR24 is usually applied as a mixture of two isomers, which mimic both SL and KAR signals (De Cuyper et al. 2017), we therefore also compared the transcriptomes of roots obtained from TRV:GbCCD7 and TRV:00 plants infected with V. dahliae for 3 dpi to firmly understand SL-mediated resistance of cotton to V. dahliae infection at the molecular level. The comparative transcriptome analysis results are shown in Supplemental Table S4, and the data are accessible under the NCBI Gene Expression Omnibus accession number PRJNA813005. The four groups TRV:GbCCD7 Control (d7C, TRV:GbCCD7 cotton roots without V. dahliae infection), TRV:00 Control (C, TRV:00 cotton roots without V. dahliae infection), TRV:GbCCD7 V. d (d7V, TRV:GbCCD7 cotton roots with V. dahliae infection), and TRV:00V. d (V, TRV:00 cotton roots with V. dahliae infection) were analyzed to identify DEGs (Supplemental Table S5). We found 398 significantly upregulated and 232 downregulated (|fold change| > 2 and P-value < 0.05) genes in “d7V vs. V” roots (Figure 5F and Supplemental Table S5, G and H). There was a total of 91 DEGs that were overlapping between the “d7V vs. V” and “d7C vs. C” comparisons (Figure 5G and Supplemental Table S5I). Functional GO enrichment analysis of 232 downregulated genes indicated that many genes classified by biotic resistance-related terms, such as “phenylpropanoid metabolic process”, “innate immune response”, and “response to fungus”, were regulated by GbCCD7 (Figure 5H and Supplemental Table S6). Additionally, two hormone-related terms, “response to ET” and “hormone biosynthetic process”, were also enriched with downregulated genes (Figure 5H and Supplemental Table S6). Consistent with the RNA-seq data, several genes were selected for verification using RT-qPCR (Supplemental Figure S5B). We found that two MYC2 homologs (GH_D08G1271 and GH_A08G1275) were upregulated in “V vs. C” comparison (Supplemental Table S5B) but not in “d7V vs. d7C” comparison (Supplemental Table S5D), which indicated that MYC2 may play a role in SL-mediated resistance of cotton to V. dahliae infection. These results collectively suggest that SL-associated processes may enhance cotton resistance to V. dahliae infection through the regulation of immune responses and phenylpropanoid metabolic processes, and crosstalk with other hormone signaling pathways.
SLs enhanced Verticillium wilt resistance through crosstalk with Ja-, ABA-, and SA-signaling pathways
Next, we found that the transcript levels of JA-signaling-related MYC2 and PDF1.2 genes were upregulated in WT cotton plants treated with rac-GR24 at several time points during the time-course treatment (Figure 6A), while they were downregulated in WT cotton plants treated with TIS108 (Supplemental Figure S6A). Additionally, increased expression levels of MYC2, PDF1.2, and VSP2 were detected in GbCCD8b-overexpressing cotton lines (Figure 6B), but their decreased expression levels were noted in GbCCD7- and GbCCD8b-silenced cotton plants (Supplemental Figure S6B). The expression of SA signaling-related marker genes NPR1 and PR1 (Yan et al. 2016) was suppressed in rac-GR24-treated WT cotton plants and in GbCCD8b-overexpressing cotton lines (Figure 6, A and B), but these genes were upregulated in TIS108-treated WT cotton plants and in both GbCCD7- and GbCCD8b-silenced cotton plants (Supplemental Figure S6, A and B). Furthermore, ABA-signaling-related ABI5 and PYL4 were upregulated in the GbCCD8b-overexpressing cotton lines (Figure 6B). These results suggested that SL-mediated cotton resistance to Verticillium wilt might be associated with the activation of JA and ABA signaling and repression of SA signaling.
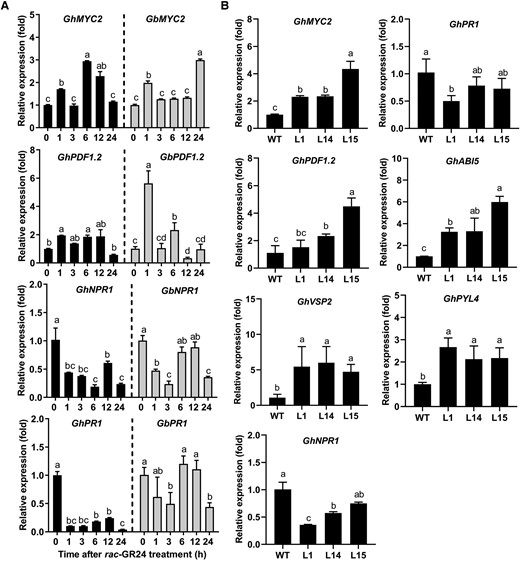
Relative expression levels of several defense-related and hormone-related genes. A) Relative expression levels of studied genes in roots of Jimian11 and Hai7124 plants treated with rac-GR24 for 0, 1, 3, 6, 12, and 24 h. Data are means ± SD of three biological replicates (n = 3; three independent plants/biological replicate). The expression value at 0 h was normalized as 1. Different letters indicate statistically significant differences among different time points after rac-GR24 treatment as determined by a one-way ANOVA analysis using the Tukey's HSD test (P < 0.05). B) Relative expression levels of studied genes in roots of WT and GbCCD8b-overexpressing transgenic lines (L1, L14, and L15). Data are means ± SD of three biological replicates (n = 3; three independent plants/biological replicate). The expression value in WT was normalized as 1. Different letters indicate statistically significant differences among different genotypes as determined by a one-way ANOVA analysis using the Tukey's HSD test (P < 0.05).
To further understand the role of JA, SA, and ABA in SL-mediated resistance of cotton against V. dahliae, we also examined the transcript levels of GbCCD7 and GbCCD8b in cotton plants following their treatments with methyl JA (MeJA), SA, and ABA. Results showed that the expression levels of GbCCD7 and GbCCD8b in cotton roots were reduced by MeJA treatment, but not influenced by SA treatment (Supplemental Figure S6C). Conversely, we observed increased expression levels of GbCCD7 and GbCCD8b in cotton roots after ABA treatment (Supplemental Figure S6C). Importantly, the concentrations of JA and ABA in cotton roots were significantly increased in response to V. dahliae infection (Supplemental Figure S6D), which was in agreement with the RNA-seq data that revealed several upregulated JA biosynthesis-related (e.g. LOX1.4 and LOX1.5) and ABA biosynthesis-related (e.g. AAO) genes in V. dahliae-infected cotton roots (Supplemental Figure S6E). However, the SA content was not influenced by V. dahliae infection (Supplemental Figure S6D). These data together suggest that JA and ABA may affect the homeostasis of SLs in cotton through negative and positive feedback loops, respectively, in response to V. dahliae infection.
Three-dimensional structure and subcellular localization of GbCCD7 and GbCCD8b
To better understand the action of GbCCD7 and GbCCD8b, first their three-dimensional protein structures were predicted using the online website (https://swissmodel.expasy.org/). As shown in Supplemental Figure S7A, both GbCCD7 and GbCCD8b proteins have two kinds of configuration, a monomer and homodimer with a Fe2+ enzyme active center. Next, the subcellular localization of GbCCD7 and GbCCD8b was examined using the GFP-fuzed expression vector for transient expression assay in Nicotiana benthamiana leaves. As shown in Supplemental Figure S7B, the green fluorescent signals were found in the chloroplasts (Supplemental Figure S7C), indicating that GbCCD7 and GbCCD8b are localized in these organelles.
GbMYC2 regulates SL homeostasis by downregulating GbCCD7 and GbCCD8b expression
Considering that exogenous application of MeJA reduced GbCCD7 and GbCCD8b expression levels (Supplemental Figure S6C), we considered whether SL biosynthesis is regulated by MYC2, the key regulator of the JA-signaling pathway. Thus, we first performed an in silico analysis of cis-acting regulatory elements present in the 2-kb promoter regions upstream of GbCCD7 and GbCCD8b genes, and found that their promoter contained many MYB and MYC cis-elements (MYB and MYC recognition sites) (Supplemental Figure S8A). Further analysis showed that there are more cis-elements related to ABA and JA responses than those related to SA response in the 2-kb promoters of GbCCD7, GbCCD8a, and GbCCD8b (Supplemental Figure S8B). Additionally, the distributions of defense-related cis-acting elements in the GbCCD7 and GbCCD8b promoters were highly similar (Supplemental Figure S8C).
To test whether GbMYC2 could bind to the promoter regions of GbCCD7 or GbCCD8b genes, we performed a yeast one-hybrid (Y1H) assay. Self-activation assays of pAbAi-proGbCCD7 and pAbAi-proGbCCD8b showed that Y1HGold reporter strains having the reporter constructs alone were inhibited on the medium synthetic dropout (SD)/-Ura + 700 ng mL−1 AbA (Figure 7A). Yeast reporter strains were then transformed with the pGADT7-GbMYC2 effector plasmid, and obtained transformants could grow on the medium SD/-Ura + 700 ng mL−1 AbA, indicating the interaction of GbMYC2 with the GbCCD7 and GbCCD8b promoters (Figure 7B). These interactions were further verified by a luciferase (LUC) imaging test, using two LUC reporter constructs driven by GbCCD7 and GbCCD8b promoters (ProGbCCD7:LUC and ProGbCCD8b:LUC) (Figure 7C). These two constructs were individually co-infiltrated with the effector Pro35S:MYC2 into N. benthamiana leaves by Agrobacterium tumefaciens injection to conduct a transient expression assay. The results showed that co-transformation of ProGbCCD7/8b:LUC and Pro35S:MYC2 decreased LUC activity compared with co-transformation of ProGbCCD7/8b:LUC and control empty vector (Figure 7D), suggesting that GbMYC2 probably functions as a negative regulator to repress the expression of GbCCD7 and GbCCD8b genes in cotton.
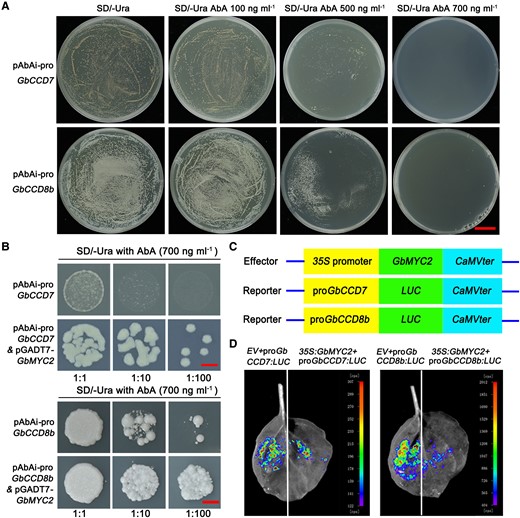
The interaction between GbMYC2 with the promoters of GbCCD7 and GbCCD8b. A) Self-activation assay of pAbAi-proGbCCD7 and pAbAi-proGbCCD8b. Y1HGold reporter strains containing pAbAi-proGbCCD7 or pAbAi-proGbCCD8b reporter construct alone were inhibited on the medium SD (SD)/-Ura + 700 ng mL−1 AbA (aureobasidin A). Bar = 2 cm. B) GbMYC2 binds to the promoters of GbCCD7 and GbCCD8b genes by a yeast one-hybrid (Y1H) assay. Y1HGold reporter strains transformed with pGADT7-GbMYC2 and pAbAi-proGbCCD7/8b plasmid could grow on the medium SD/-Ura + 700 ng mL−1 AbA. Bar = 5 mm. C) Schematic diagram of the LUC reporter gene expression model. D) LUC imaging analysis of the interaction between GbMYC2 and GbCCD7 or GbCCD8b promoter. The LUC signal was collected 2 d after infiltration, and the experiment was repeated three times with three leaves each time. EV, empty vector.
Interestingly, although GbMYC2 negatively regulates the transcription of GbCCD7 and GbCCD8b, GbMYC2 knock-down (TRV:GbMYC2) plants displayed more severe disease symptoms than control plants after 2 wk of inoculation with V. dahliae (Supplemental Figure S9, A–F). The transcript levels of GbMYC2, GbPDF1.2, and GbVSP2 were significantly reduced, while those of GbCCD7 and GbCCD8b increased in TRV:GbMYC2 plants as compared with control plants (Supplemental Figure S9, G–K). These results suggest that GbMYC2 positively regulates cotton resistance to V. dahliae disease by upregulating the expression of GbPDF1.2 and GbVSP2, while downregulating the expression of GbCCD7 and GbCCD8b.
SLs enhance Verticillium wilt resistance by increasing ROS accumulation
ROS play pivotal roles in pattern-triggered immunity and effector-triggered immunity during pathogen infection (Ku et al. 2018). Staining with 3,3′-diaminobenzidine (DAB) was conducted to detect ROS accumulation in the leaves of GbCCD7-silenced and GbCCD8b-silenced cotton plants. The DAB staining assay showed lower ROS accumulation in the leaves of TRV:GbCCD7 and TRV:GbCCD8b cotton plants than in TRV:00 control plants, 2 dpi with V. dahliae (Supplemental Figure S10A). Additionally, the contents of hydrogen peroxide (H2O2) significantly decreased in TRV:GbCCD7 and TRV:GbCCD8b plants compared with TRV:00 control plants (Supplemental Figure S10B). These results indicate a weaker ROS burst, and thus lower ROS accumulation due to SL deficiency in GbCCD7-silenced and GbCCD8b-silenced cotton plants. Furthermore, we detected more cell death in the TRV:GbCCD7 and TRV:GbCCD8b plants than in control plants using the trypan blue (TB) staining assay, where the leaves of TRV:GbCCD7 and TRV:GbCCD8b plants were more highly stained than the leaves of TRV:00 cotton plants after infection with V. dahliae (Supplemental Figure S10C). This result suggested that SLs may promote ROS accumulation to increase resistance to V. dahliae in cotton.
Discussion
Previous investigations indicated that SLs regulate various developmental processes, and resistance to abiotic and biotic stresses in various plant species (Gomez-Roldan et al. 2008; Kapulnik et al. 2011b; de Jong et al. 2014; Ha et al. 2014; Torres-Vera et al. 2014; Ueda and Kusaba 2015; Decker et al. 2017; Lahari et al. 2019; Nasir et al. 2019; Li et al. 2020; Xiong et al. 2020; Aquino et al. 2021). However, the detailed roles and mechanisms modulated by SLs in response to V. dahliae infection were still unclear in the important crop cotton. In the present study, we provide several lines of evidence that SLs positively regulate V. dahliae resistance in cotton. First, exogenous application of the SL analog rac-GR24 enhanced the resistance of cotton to V. dahliae (Figure 2), whereas application of the SL biosynthesis inhibitor TIS108 reduced resistance (Supplemental Figure S1). Second, knock-down of GbCCD7 or GbCCD8b decreased SL content in cotton plants and reduced their Verticillium wilt resistance (Figure 3), whereas overexpression of GbCCD7 in Arabidopsis and GbCCD8b in both cotton and Arabidopsis increased SL content (measured in cotton transgenic plants) and the resistance of both transgenic plants (Figure 4 and Supplemental Figure S4). Furthermore, we observed that the expression levels of GbCCD7 were increased after the application of TIS108 for 12 h (Supplemental Figure S1A), indicating a feedback regulation of SL biosynthesis, at least through GbCCD7, in cotton. Similar regulatory processes were also observed in Arabidopsis and rice (Ito et al. 2013, 2022).
To prevent V. dahliae infection, plants have evolved several resistant mechanisms (Song et al. 2020), and phytohormones play pivotal roles in balancing plant growth and stress responses (Mauch-Mani and Mauch 2005; Torres-Vera et al. 2014; Zhang et al. 2018; Yang et al. 2019; Song et al. 2020). In this study, we found that the JA-signaling-related MYC2 and PDF1.2 genes were upregulated, whereas SA signaling-related NPR1 and PR1 genes were downregulated in cotton plants after their treatment with rac-GR24 (Figure 6A). Additionally, we found that silencing the GbMYC2 in cotton plants reduced their resistance to V. dahliae, and downregulated the expression of the JA-responsive genes GbPDF1.2 and GbVSP2 (Supplemental Figure S9) that are known to be involved in pathogen resistance (Xiong et al. 2020). These data suggest that SLs might enhance JA-signaling through GbMYC2, and its downstream genes GbPDF1.2 and GbVSP2, while suppressing SA signaling, thereby resulting in induced V. dahliae resistance in cotton (Figure 8).
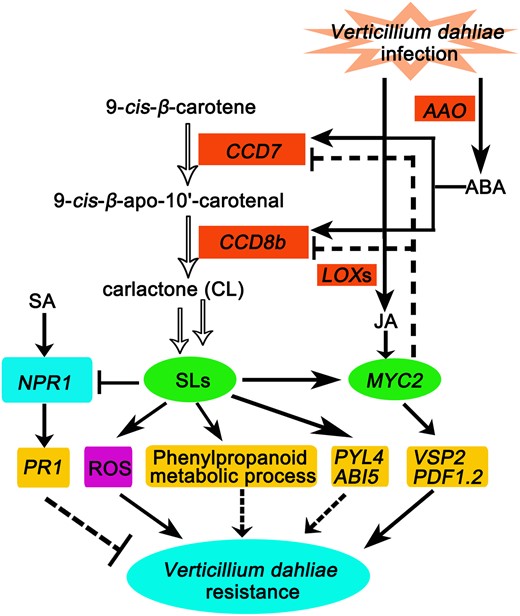
A proposed model for the role of SLs in enhancing cotton resistance to V. dahliae via crosstalk with JA-, SA-, and ABA-signaling pathways, and promoting ROS accumulation and phenylpropanoid metabolic process. V. dahliae infection induces the expression of CCD7 and CCD8b, resulting in SL accumulation and consequently improving V. dahliae resistance by promoting the expression of several defense-related genes involved in JA-signaling, including MYC2, PDF1.2, and VSP2. Additionally, SLs also increase ROS accumulation and enhance ABA-signaling-related genes PYL4 and ABI5 expression, while suppressing the expression of SA signaling-related genes NPR1 and PR1 genes to enhance V. dahliae resistance. The phenylpropanoid metabolic process, which is induced by SLs, may also be involved in improvement of V. dahliae resistance. V. dahliae infection triggers accumulations of ABA and JA, probably by inducing ABA biosynthesis-related AAO gene and JA biosynthesis-related LOX genes, respectively. V. dahliae infection-induced ABA upregulates the expression of CCD7 and CCD8b, resulting in increased SL content in cotton roots. V. dahliae infection-induced JA upregulates MYC2 expression, which leads to repression of the expression of CCD7 and CCD8b, consequently decreased SL content in cotton roots. Such positive feedback loop by ABA and negative feedback loop by JA in regulation of SL homeostasis result in a balance among these three hormones, leading to improved cotton disease resistance to V. dahliae infection. Hollow arrows indicate biosynthesis and black arrows indicate stimulation. Black blunt bars indicate suppression, dotted arrows indicate uncertain stimulation, and dotted blunt bar indicate uncertain suppression.
Reversely, we found that the expression levels of the SL biosynthesis-related GbCCD7 and GbCCD8b genes were decreased and increased by exogenous applications of MeJA and ABA, respectively, in cotton roots (Supplemental Figure S6C). These results suggested that JA and ABA might affect the homeostasis of SLs in cotton, and thus influence the function of SLs in cotton resistance to V. dahliae infection. In support of our findings, previous studies showed that SLs biosynthesis might be promoted in plants through the actions of some other hormones, such as ABA, auxin, and ET that upregulate several SL biosynthesis-related genes (Ha et al. 2014; Li et al. 2018b; Omoarelojie et al. 2019; Faizan et al. 2020; Wu et al. 2022). Furthermore, we also found that V. dahliae infection significantly increased the expression of several JA biosynthesis-related and ABA biosynthesis-related genes, and thereby the JA and ABA contents in cotton roots (Supplemental Figure S6, D–E), which may result in the change in the expression of the GbCCD7 and GbCCD8b, thereby SL content in infected cotton roots (Figure 8). The result that GbMYC2 directly binds to the promoters of GbCCD7 and GbCCD8b genes and represses their expression (Figure 7) supports the idea that JA potentially downregulates the expression of GbCCD7 and GbCCD8b through a negative feedback loop. Thus, MYC2 may have dual transcriptional regulatory activities in plant responses to pathogens, which was also supported by previous investigations (Lorenzo et al. 2004; Sasaki-Sekimoto et al. 2013). The dual functions of TFs in repression or activation of various target genes through their direct physical interaction is an interesting phenomenon that has been reported previously, such as for ABI4 (Huang et al. 2017) and MYB70 (Wan et al. 2021). Additionally, our transcriptome analysis showed that ABA-signaling-related genes, such as ABI5 and PYL4, were upregulated in cotton plants by either rac-GR24 treatment or overexpression of GbCCD8b (Figs. 5 and 6), suggesting a positive association between S, L, and ABA responsiveness. We proposed that SLs might enhance cotton resistance to V. dahliae infection through enhancing ABA responses in cotton roots during the early invasive stage, which was supported by the positive role of ABI5 and ABA signaling during early post-invasive resistance in Arabidopsis plants (Ton et al. 2009; Ku et al. 2018). These findings collectively suggest the existence of a negative feedback loop and a positive feedback loop to maintain SL homeostasis in cotton by JA and ABA, respectively, in response to V. dahliae infection. The balance among these three hormones may lead to improved cotton disease resistance to V. dahliae infection (Figure 8).
One of the main reasons for pathogenesis of V. dahliae infection is xylem vessel blockage (Luo et al. 2014; Shaban et al. 2018). To prevent such blockage, plants trigger various processes, such as lignin synthesis through phenylpropanoid metabolic pathway and callose deposition, which result in cell wall modifications to block pathogens from entering the roots during the early stages of infection and xylem vessels at later stages (Shi et al. 2012; Yang et al. 2017). Our transcriptome data indicated that the phenylpropanoid metabolic pathway is involved in SL- and V. dahliae-induced cotton Verticillium wilt resistance as evidenced by, for example, the induced expression of the GbCAD9 and GbMYB4 genes in cotton roots by rac-GR24 (Figure 5E). This observation suggests that SLs might enhance V. dahliae resistance through lignification of xylem vessels, which is supported by the function of GhMYB4 as a positive regulator of cotton resistance to V. dahliae (Xiao et al. 2021). In contrast to the upregulation of genes related to the phenylpropanoid metabolic process in rac-GR24-treated plants, many genes classified into the GO term “regulation of phenylpropanoid metabolic process” were downregulated in TRV:GbCCD7-silenced plants (Figure 5H). These results further indicated that SLs enhance Verticillium wilt resistance through promoting phenylpropanoid metabolic process (Figure 8).
In addition, we also found that ROS accumulation was reduced in TRV:GbCCD7 and TRV:GbCCD8b cotton plants, compared with control plants, demonstrating the involvement of ROS in SL-mediated Verticillium wilt resistance (Supplemental Figure S10, A and B). ROS accumulation has also been reported to be regulated by the JA- and ABA-signaling pathways (Ku et al. 2018; Boba et al. 2022), and the accumulation of ROS has generally found to be important in regulation of systemic disease resistance in plants as a signaling molecule after pathogen invasion, including the activation of Ca2+ signaling, kinase cascades, and hormone signaling (Choudhury et al. 2013; Suzuki and Katano 2018). These results supported the view that SLs also enhance Verticillium wilt resistance through increasing ROS accumulation (Figure 8).
In conclusion, our results demonstrated that SLs positively regulate Verticillium wilt resistance in cotton plants through crosstalk with the JA-, SA-, and ABA-signaling pathways and by increasing ROS accumulation and promoting phenylpropanoid metabolic processes. SLs promote the expression of JA-signaling-related PDF1.2 and MYC2 genes, suppress SA-signaling-related NPR1 and PR1 gene expression, and promote the expression of the ABA-signaling-related ABI5 and PYL4 genes (Figure 8). In addition, JA likely regulates SL homeostasis through GbMYC2 in a negative feedback loop, and ABA appears to promote SL biosynthesis through upregulation of the CCD7 and CCD8 genes in cotton roots (Figure 8). Maintaining the balance among ABA, JA, and SLs is important for cotton disease resistance to V. dahliae infection. Collectively, our results provide insights that could aid in enhancing Verticillium wilt resistance in cotton plants through genetic modification of SL-biosynthetic pathway components.
Materials and methods
Plant materials and growth conditions
The island cotton (G. barbadense) variety Hai7124 and the upland cotton (G. hirsutum) varieties Jimian11 and Baimian1 (a hybrid variety from Bainong 8602 and Xin3313, Henan Institute of Science and Technology, China) were used in this study. Cotton seeds were imbibed in water for 8 h, and then incubated at 28 °C in the dark for germination. Three days after germination, the seedlings were transferred to soil and grown under 8/16 h light/dark photoperiod (22/25 °C). After being grown in soil for 10 d, seedlings were used for VIGS-infiltration experiments. Transgenic lines overexpressing GbCCD8b were obtained in Baimian1 background by plant tissue culture technology using the vector of pEarleyGate 103 (BioVector, Beijing, China), and T1 generation seeds were used for Verticillium wilt resistance verification. For Arabidopsis (A. thaliana), the Columbia-0 (Col-0) accession was used as the WT, and the Arabidopsis transgenic lines ectopically expressing GbCCD7 or GbCCD8b were obtained using the vector of pCAMBIA2300-GFP (BioVector, Beijing, China). Seeds of max3-11 (SALK_023975) and max4-8 (SALK_072750) in Col-0 background were obtained from a previous study (Umehara et al. 2008).
Phylogenetic analysis and gene exon/intron architecture construction
GbCCD7 and 8a/b protein sequences were aligned using MUSCLE in MEGA 7 (Kumar et al. 2016). An unrooted phylogenetic tree was constructed using the neighbor-joining (NJ) method, 1000 bootstrap replicates, and the Jones–Taylor–Thornton model. The phylogenetic tree was further visualized and annotated using Evolview (https://www.evolgenius.info; Balakrishnan et al. 2019). The gene exon/intron architecture was determined with the Gene Structure Display Server (http://gsds.cbi.pku.edu.cn/) based on the coding sequence (CDS) and corresponding genomic sequences (Li et al. 2014). For cis-acting element analysis, the 2-kb promoter regions upstream of the ATG codon of each sequence were analyzed using PlantCARE (Lescot et al. 2002).
Cultivation of V. dahliae strain V991
The highly aggressive defoliating V. dahliae strain V991 was stored in 30% (v/v) glycerol at −80 °C. For activation, 100 µL conidial suspension was cultured on potato dextrose agar (PDA) plates for 7 d at 25 °C in the dark. Fungi collected from the plates were then inoculated in Czapek's medium (Gao et al. 2013) and cultured at 25 °C for 3−4 d with 150 rpm shaking until the concentration reached ∼108 to 109 spores mL−1. Fungal conidia were collected by filtering the culture through gauze, followed by dilution to an approximate density of 107 conidia mL−1 with sterile water before use.
Gene cloning and VIGS vector construction
The CDSs for GbCCD7, GbCCD8a/b, and GbMYC2 were obtained from the cotton database (https://cottonfgd.org/sequenceserver/) (GB_A12G1178_ZJU-AD2 _v1.1.a1, GB_A06G1044_ZJU-AD2_v1.1.a1, GB_A07G0258_ZJU-AD2_v1.1.a1, and GB_A08G1925_ZJU-AD2_v1.1.a1, respectively). Primers were designed using Premier 6.0 (http://www.premierbiosoft.com/) to amplify the GbCCD7, GbCCD8a/b, and GbMYC2 sequences from Hai7124 cDNA. The three genes were cloned into the 007vs cloning vector (TsingKe Biotech, Beijing, China), then Escherichia coli DH5α cells were separately transformed with each of the plasmids. Constructs were verified by sequencing and stored at −80 °C until use. The GbCCD7, GbCCD8a/b, and GbMYC2 fragments were amplified by PCR using adaptor primers having restriction sites of BamHI and KpnI to allow one-step cloning and vector digestion (Supplemental Table S7A). The PCR products of GbCCD7, GbCCD8a/b, and GbMYC2 with the adaptors were cloned into the pTRV2 vector to produce the recombinant plasmids TRV:GbCCD7, TRV:GbCCD8a, TRV:GbCCD8b, and TRV:GbMYC2. A. tumefaciens strain GV3101 was transformed with the recombinant plasmids and stored at −80 °C before use.
VIGS transient expression and pathogen infection assays
The VIGS transient expression assay was performed as previously described (Gao et al. 2011). All VIGS-infiltrated Hai7124 seedlings, including TRV:GbCLA, TRV:GbCCD7, TRV:GbCCD8a, TRV:GbCCD8b, TRV:GbMYC2, and TRV:00, were grown for 2 wk until the albino phenotype appeared in the TRV:GbCLA seedlings. True leaves of these cotton seedlings with the exception of TRV:GbCLA were harvested and stored at −80 °C to quantify interference efficiency. TRV:00, TRV:GbCCD7, TRV:GbCCD8a, TRV:GbMYC2, and TRV:GbCCD8b cotton seedlings were then inoculated with V. dahliae V991 spore suspension (1 × 107 spores mL−1) and water (control) through injured roots for 1 min. These V. dahliae-treated and water-treated seedlings were transplanted to their original pots and incubated in a growth chamber at 23 °C with a 16-h light and 8-h dark cycle and 80% humidity. The experiments were performed with at least 30 seedlings per treatment and three independent treatments were performed. The proportion of diseased plants and the disease index were calculated as previously described (Yan et al. 2016). The degree of wilt plants was evaluated and divided into five grades from 0 to 4 based on their disease severity, and the disease index was calculated with the following formula: disease index = [(∑disease grades × number of infected plants)/(total checked plants × 4)] × 100.
For Arabidopsis inoculations, 4-wk-old seedling roots were rinsed in water and inoculated with the GFP-labeled V. dahliae V991 spore suspension (1 × 107 spores mL−1) through injured roots for 1 min, and the plants were then replanted in fresh soil. After incubation for 0, 6, 12, and 24 h at 23 °C, the inoculated roots were observed with a confocal laser scanning microscope (ZeissLSM710, Oberkochen, Germany), experimental setup (argon ion laser, eGFP, 488 nm, gains 60 dB).
Section anatomy in stems and fungal renewal assay
Stems of cotton plants were manually cut into cross sections and observed with a stereo microscope (OLYMPUS, Tokyo, Japan). The fungal renewal culture of V. dahliae required for the stem sections was sterilized with 0.1% HgCl2 for 5 min and washed six times with sterilized water. The stem sections from V. dahliae-infected cotton plants were laid on PDA medium and incubated at 25 °C for 1 wk before observation of the V. dahliae growth states.
RNA isolation and RT-qPCR analyses
Total RNA was extracted from cotton leaves using the FastPure Plant Total RNA isolation kit (Nanjing Vazyme Biotech Co., Ltd, China). First-strand cDNA was reverse transcribed using a HiScript II Q RT SuperMix kit for RT-qPCR (Nanjing Vazyme Biotech Co., Ltd). RT-qPCR was used to quantify the expression levels of relevant genes in plant tissues. RT-qPCR was performed on an ABI 7500 real-time PCR system (Applied Biosystems, Waltham, MA, USA) with SYBR green (Bio-Rad, Hercules, CA, USA). Relative gene expression levels were calculated using the 2−ΔΔCt method (Rieu and Powers 2009) with the cotton UBQ7 gene (GhUB7, Accession: DQ116441) as the internal control. Gene-specific primers used for RT-qPCR are shown in Supplemental Table S7B. The reaction procedure was 40 cycles of 95 °C for 20 s, 95 °C for 3 s, and then 60 °C for 30 s. All reactions were performed with three biological and three technical replicates.
Western blot analysis
Total proteins were extracted from the transgenic lines of cotton and Arabidopsis as previously described (Zhang et al. 2016), and used for Western blotting. The total proteins were separated by 12% sodium dodecyl sulfate-polyacrylamide gel electrophoresis and transferred onto a polyvinylidene fluoride membrane (Roche Applied Science, Mannheim, Germany). The membrane was orderly blotted with the primary antibody of anti-GFP mouse monoclonal antibody and the secondary antibody of HRP-conjugated AffiniPure goat anti-mouse IgG (Thermo, Waltham, Massachusetts). The color was developed using chemiluminescence imaging system Fusion-FX (Vilber, Paris, France).
Hormone treatments
Each of the following hormones rac-GR24, SA, ABA, and MeJA was dissolved in ethanol to make 10 mM stock solution. The working solutions were generated by diluting stock solutions to 10 μM with distilled water, and an equivalent concentration of ethanol diluted in distilled water was used as the control. When the cotton seedlings had three to four true leaves, the seedlings were treated by foliar spraying with SA, ABA, and MeJA, or soil drenching with rac-GR24. Leaf or root samples were collected at different time points after the treatments. Plant materials were collected into storage bags and frozen in liquid nitrogen, then used immediately or stored at −80 °C. Each treatment had three biological replicates.
Germination assay of O. aegyptiaca seeds
The O. aegyptiaca seed germination assay was modified from a previous report (Ma et al. 2012). Briefly, O. aegyptiaca seeds were surface-sterilized by immersion in 1% sodium hypochlorite for 3 min with mild shaking, and then rinsed with water for 5 times. The sterilized seeds were directly used for the germination assay, which involved spraying seeds with water on 8-mm disks of glass fiber filter paper (Whatman, GE Healthcare UK Ltd., Buckingham shire, UK) placed in Petri dishes. The disks with seeds were briefly blotted with filter paper to remove excess water before the treatments with the extracts. To obtain aqueous extracts from cotton roots, root samples (100 mg per sample) were ground to a powder and sonicated for 30 min in 1.5 mL water. The aqueous extracts from the cotton roots were centrifuged and the supernatants were assayed immediately by applying 15 µL of each extract solution to the O. aegyptiaca seeds on the disks in Petri dishes. The imbibed seeds were incubated in the dark at 25 °C and germination percentages were examined 8 d later. The experimental groups of root extracts from Mock, V. dahliae-infected, TIS108-treated, and gene-silenced and overexpression transgenic plants were used to test O. aegyptiaca seed germination. Three water-treated disks (negative control) with conditioned O. aegyptiaca seeds were performed parallelly.
UPLC-MS/MS analysis of hormone contents
Cotton roots from V. dahliae-infected and -uninfected plants were harvested after 2 h of inoculation and frozen in liquid nitrogen, then stored at −80 °C. Phytohormone extraction and analysis were performed as previously described (Wang et al. 2016a). Briefly, root samples (0.5 g) were ground in mortar using liquid nitrogen and extracted with 2 mL of ethyl acetate in 5 mL micro-centrifuge tubes. Samples were vortexed and sonicated for 15 min in an Elmasonic S150 bath (Elma Ultrasonic, Singen, Germany), and then centrifuged for 10 min at 2500 × g. The supernatant was re-extracted and the ethyl acetate was evaporated to dry under vacuum conditions. The dried fractions were re-suspended in 150 µL of acetonitrile:water (25:75, v/v) solution, filtered, and subjected to ultra performance liquid chromatography (UPLC)-MS/MS analysis on an Agilent 1290 infinity HPLC system (Agilent Technologies, Palo Alto, USA).
Subcellular localization
The GbCCD7 and GbCCD8b genes were amplified using primers with adaptor sequences attB1 and attB2 added to the 5′ end. The PCR products were cloned into the pK7FWG2.0 vector (http://gateway.psb.ugent.be/search) with BP and LR (attB + attP; attL + attR) reactions. The fuzed expression vectors p35S-GFP:GbCCD7 and p35S-GFP:GbCCD8b were obtained and subsequently transformed into A. tumefaciens strain GV3101, and empty pK7FWG2.0 was used as a control. N. benthamiana leaves were infiltrated with the Agrobacterium culture suspensions for both target genes and the control using a 2 mL needless syringe. After incubation in the dark for 2 to 3 d at 25 °C, segments of infiltrated leaves were observed with a confocal laser scanning microscope (ZeissLSM710, Oberkochen, Germany), experimental setup (argon ion laser, eGFP (enhanced green fluorescent protein), 488 nm, gains 60 dB).
Dual-LUC reporter (DLR) assay
The interaction of GbMYC2 with GbCCD7 and GbCCD8b promoters was examined using a dual-LUC reporter (DLR) assay. The reporter plasmid pGreenII 0800-LUC (Hellens et al. 2005) contained the LUC gene, controlled by GbCCD7 and GbCCD8b promoters with G-box binding elements upstream. The Renilla LUC-encoding gene driven by the 35S promoter was used as an internal control. To construct the effector plasmid, the CDS of GbMYC2 was introduced into the overexpression vector pGreenII 62-SK to generate p35S-GbMYC2. Subsequently, A. tumefaciens strain GV3101 cultures were transformed with the pCCD-LUC reporter or p35S-GbMYC2 effector plasmid, or with the empty vector used as a control. Equal amounts of A. tumefaciens strain GV3101 cultures containing pCCD-LUC and p35S-GbMYC2 constructs were mixed and co-infiltrated into N. benthamiana leaves. After 12 h in the dark, plants were returned to normal growth conditions for 24 h, after which leaves were uniformly sprayed with 0.2 mg mL−1 LUC substrate. The fluorescence signal was detected after 8 min in darkness, and the LUC assay was conducted using the DLR assay system and a GloMax 20-20 luminometer (Promega, Madison, WI, USA).
Y1H assay
A Y1H assay was performed using the Matchmaker Gold Yeast One-Hybrid Library Screening System (Clontech, Dalian, China). The tested promoter fragments were inserted into the yeast reporter plasmid pAbAi. The bait constructs were linearized with endonuclease BstBI (New England Biolabs, Ipswich, MA, USA), and inserted into the genome of Y1HGold yeast strain by homologous recombination to generate a bait-specific reporter strain. The prey construct pGADT7-GbMYC2 was introduced into the bait-specific yeast reporter strain to further confirm the interaction between pCCD7/8b and GbMYC2. The empty vector pGADT7 was used as a control. Transformed yeast clones were cultured at 30 °C on SD/-Ura or SD/-Leu medium with different concentrations of aureobasidin A (AbA) to inhibit self-activation.
Histochemical assay and H2O2 measurement
Total H2O2 accumulation was examined by staining with DAB. True cotton leaves were incubated overnight in 1 mg mL−1 DAB-HCl at pH 3.8 in the dark, then exposed to a vacuum for 10 min. The staining solution was drained off and absolute ethanol added before tissue immersion in a boiling water bath for 10 min to remove chlorophyl. H2O2 was observed in the leaves as a reddish-brown precipitate. The content of H2O2 was determined using the method previously described (Wang et al. 2019). Fresh cotton roots (0.1 g) were homogenized in 1 mL of pre-cold acetone. Then, H2O2 content was determined using a H2O2 assay kit (Solarbio, China) and its absorbance was measured at 415 nm. Data are represented as the amount of H2O2 per gram roots (μmol g−1). All analyses had three biological replicates.
TB staining
Cotton leaves were boiled for 5 to 8 min and stained with solution of TB for 4 to 6 h at room temperature. After 95% ethanol decolorization treatment, the distribution of damage in the leaf tissues was observed. Three leaves were treated per experiment.
RNA-seq
Cotton plants were grown in vermiculite for 3 wk and treated with rac-GR24 24 h prior to infection with V. dahliae. Uninfected plants were used as the control. The TRV:00 and TRV:GbCCD7 plants were prepared in the same way as previously described in pathogen infection assays. Then, the roots of these V. dahliae-treated and water-treated seedlings TRV:00 and TRV:GbCCD7 were collected. Total RNA was isolated from roots collected 3 dpi and libraries were prepared for sequencing. Sequencing was performed on the HiSeq2000 platform (Illumina, San Diego, CA, USA). After preprocessing the RNA-seq data with Trimmomatic software (Bolger et al. 2014), the clean reads were mapped to the G. hirsutum ZJU assembly genome using Hisat2 with default parameters (Kim et al. 2015). Htseq-count (Simon et al. 2015) software was used to count the number of reads per protein-coding gene in each sample. DESeq2 (Love et al. 2014) was used to standardize the counted number of genes in each sample (BaseMean value was used to estimate the expression level), the multiple difference was calculated, and a negative binomial distribution test was used to test the significance of differences. DEGs for rac-GR24 treatment experiment were defined as those with P-value < 0.05 and |fold change| > 1.5, and DEGs obtained from comparison of TRV:GbCCD7 and TRV:00 roots were defined as those with P-value < 0.05 and |fold change| > 2. GO and KEGG analysis of enriched biological process terms among the DEGs was conducted using AgriGO (Du et al. 2010). All samples had four biological replicates.
Accession numbers
All the RNA-seq data produced in this study were deposited at NCBI Gene Expression Omnibus under the accession numbers (PRJNA812956 and PRJNA813005). The major genes/proteins sequence mentioned in this study is shown in Supplemental Figure S8 and can be obtained from the online cotton database (https://cottonfgd.org/sequenceserver/).
Acknowledgments
We thank Professor Yongqing Ma for providing O. aegyptiaca seeds and technology for checking seed germination.
Supplemental data
The following materials are available in the online version of this article.
Supplemental Figure S1. Inhibition of SL biosynthesis reduced cotton resistance against V. dahliae.
Supplemental Figure S2. SL-deficient A. thaliana mutant plants are more sensitive than WT plants to V. dahliae infection.
Supplemental Figure S3. Response of GbCCD8a-silenced (TRV:GbCCD8a) Hai7124 plants to V. dahliae infection.
Supplemental Figure S4. Overexpressing GbCCD7 and GbCCD8b in A. thaliana enhanced the resistance of transgenic plants to V. dahliae infection.
Supplemental Figure S5. Fold changes in the expression of genes in different comparison as determined by RNA-seq and RT-qPCR.
Supplemental Figure S6. Relative transcript levels of studied genes in various experimental conditions.
Supplemental Figure S7. Three-dimensional structure and subcellular localization of GbCCD7 and GbCCD8b.
Supplemental Figure S8. Classification of putative cis-acting elements in the promoters of the GbCCD7 and GbCCD8a/b genes.
Supplemental Figure S9. Silencing GbMYC2 in Hai7124 plants reduced V. dahliae resistance.
Supplemental Figure S10. Accumulation of ROS and cell death in GbCCD7- and GbCCD8b-silenced Hai7124 cotton plants after inoculation with V. dahliae.
Supplemental Table S1. Gene expression levels (Fragments Per Kilobase of exon model per Million mapped fragments, FPKM values) and fold changes in cotton roots with and without (mock) rac-GR24-treated under the V. dahliae infected and uninfected conditions.
Supplemental Table S2. List of up- and downregulated genes in different comparison in rac-GR24-treated under the V. dahliae infected and uninfected conditions.
Supplemental Table S3. Enrichment analysis of DEG in rac-GR24-treated under the V. dahliae infected and uninfected conditions using both GO and KEGG analyses.
Supplemental Table S4. Gene expression levels (FPKM values) and fold changes in cotton roots of TRV:00 and TRV:GbCCD7 plants under infection and no-infection of V. dahliae conditions.
Supplemental Table S5. List of up- and downregulated genes in TRV:00 and TRV:GbCCD7 plants under infection and no-infection of V. dahliae conditions.
Supplemental Table S6. Enrichment analysis of DEGs in TRV:00 and TRV:GbCCD7 plants under infection and no-infection of V. dahliae conditions using both GO and KEGG analyses.
Supplemental Table S7. List of primers used in RT-qPCR analysis and vector construction.
Supplemental Table S8. Major genes/proteins used in this study.
Funding
This work was supported by the National Key Research and Development Program of China (No. 2022YFD1200303), the Strategic Priority Research Program of the Chinese Academy of Sciences (Grant No. XDA28110100), the Natural Science Foundation of China (NSFC; Grant No. 32070262; U1704104), Henan Natural Science Foundation (No. 222300420404), Innovation and Talent Introduction Program on Crop Stress Biology (111 Project), and National Key R&D Programme of China (#2018YFE0194000) from Ministry of Science and Technology of the People’s Republic of China.
References
Author notes
Y.F.C., F.F.Y., and W.Q.L. conceived the experiment content and research plans; F.F.Y. and A.S.S. performed most of the experiments; X.B.Z., K.C., and W.Q.L. provided technical assistance; J.L.L., L.L.S., S.W., C.X.W., Pi.W., A.M.C., and Z.Y.C. analyzed the data with input from D.J.B., L.-S.P.T., and L.H.-E.; Pe.W., J.X.Z., Z.L.L., C.W.C., J.X., and Y.C. conceived part of the project; D.J.B. critically reviewed and edited the manuscript. L.H.-E., L.-S.P.T., W.Q.L., and Y.F.C. supervised and complemented the writing.
The author responsible for distribution of materials integral to the findings presented in this article in accordance with the policy described in the Instructions for Authors (https://dbpia.nl.go.kr/plphys/pages/General-Instructions) is Feifei Yi.
Conflict of interest statement: The authors declared that there is no any competing interests.