-
PDF
- Split View
-
Views
-
Cite
Cite
Priya Ramakrishna, Grain scans: fast X-ray fluorescence microscopy for high-throughput elemental mapping of rice seeds, Plant Physiology, Volume 191, Issue 3, March 2023, Pages 1465–1467, https://doi.org/10.1093/plphys/kiac598
- Share Icon Share
Rice (Oryza sativa) is a staple food for over half of the global population. The rice grain has been targeted for biofortification to enhance its nutritional value and to prevent the accumulation of toxic elements. Therefore, understanding the regulatory mechanisms that control the amount and spatial distribution of essential and toxic elements in the seeds is essential. Several analytical techniques have been developed for in situ imaging of elements in plants, including histochemical staining, metal-sensitive fluorescent probes, and mass spectrometry or X-ray spectroscopy-based techniques (Conn and Gilliham, 2010; Lombi et al., 2011; Zhao et al., 2014; Kopittke et al., 2018). The method often depends on the questions being asked and the required level of throughput, sensitivity, and resolution.
Inductively Coupled Plasma—Mass Spectrometry (ICP-MS), for instance, is an analytical technique that provides a snapshot of the bulk elemental status of a sample (Salt et al., 2008). ICP-MS has been used for high-throughput elemental analysis of Arabidopsis (Arabidopsis thaliana) seeds from a diverse set of natural accessions, identifying several accessions with interesting extreme elemental phenotypes (Campos et al., 2021). In addition to being destructive, ICP-MS also lacks the visual component and spatial detail, whereas elemental imaging enables associating the distribution of an element with its functions or alteration of these functions. High-throughput elemental imaging techniques that can non-destructively screen and quantify elemental distribution patterns in seeds are necessary.
X-ray fluorescence microscopy (XRF) is an in vivo non-destructive imaging technique that allows quantitative mapping of elemental distribution in plants (Punshon et al., 2009; Donner et al., 2013; Kopittke et al., 2018). It has become a favorite method to quantify metals in seeds of hyperaccumulator plants (van der Ent et al., 2022), Arabidopsis (Kim et al., 2019), and rice (Takahashi et al., 2009). However, XRF-based techniques are often not synonymous with high-throughput. For instance, a previous study reported an acquisition time of 7.5 h for an elemental map of a longitudinal grain section with a step size of 25 µm (Lombi et al., 2009). The development of an advanced Maia fluorescence detector system that enables faster acquisition time has advanced the high-throughput of XRF-based techniques and opened-up new possibilities (Ryan et al., 2010).
In this issue of Plant Physiology, Ren et al., (2022) present the use of synchrotron-based fast XRF (µ-XRF) as a high-throughput, non-destructive phenotyping tool for elemental distribution in rice seeds. The authors reported a scan time of ∼39 h for 4,190 mutagenized seeds at 30 µm resolution (average rice grain size from the varieties in the study was ∼5 mm). They quantified the concentration and elemental distribution in the endosperm, embryo, and aleurone layer of 4,190 EMS-mutagenized rice seeds and a panel of 533 diverse rice accessions (Figure 1A). They also used a laboratory-based µ-XRF system to image grain half-section for detail on the aleurone layer and presented a script to semi-automate the extraction of elemental data from the µ-XRF images using ImageJ.
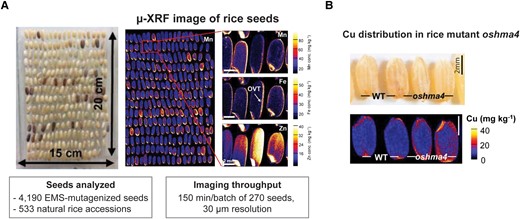
µ-XRF-based high-throughput elemental imaging of rice seeds. A, A representative image of a batch of rice seeds prepared for analysis and a µ-XRF image of the general elemental concentration in the seeds. B, A µ-XRF elemental map of copper (Cu) in the rice mutant oshma4 known for having increased Cu levels in the embryo and endosperm. OVT, ovular vascular trace. Modified from Ren et al. (2022).
The authors first tested the pipeline on three previously characterized rice mutants: heavy metal atpase 2 (oshma2) (low Zn), metal transporter natural resistance-associated macrophage proteins (osnramp5) (low Mn), and heavy metal atpase 4 (oshma4) (high Cu). The µ-XRF images supported the known elemental phenotypes and provided spatial details on differences in their distribution in the seed. For instance, in oshma4 the reported higher Cu levels corresponded to significantly increased levels (172%–190%) in the embryo and endosperm of the mutant (Figure 1B), raising many interesting questions—Does the endosperm become the dumping site when there is excess Cu? Is this pattern due to defective nutrient transport? What is the functional relevance of the redistribution?
Next, to screen for potential mutants with unusual elemental phenotypes, the authors performed a principle component analysis (PCA) on the µ-XRF elemental data of the two seed libraries. Among the EMS-mutagenized rice seeds, they identified individuals with outlier seed shapes (107) and elemental phenotypes (692). From the natural rice accessions, they distinguished individuals with strong elemental differences in the whole seed as well as selected tissues (embryo, aleurone layer, or endosperm). They found strong and expected correlations between the chemical analogs K and Rb, or Ca and Sr. They also reported strong associations between the elements Mn, Fe, Cu, and Zn in the seed. Interestingly, a similar pattern has been reported for these elements in Arabidopsis seeds (Campos et al., 2021), which shows a recurrent pattern across species. The results suggest these elements behave in an inter-dependent manner, and studying them in combination may uncover potential shared transport pathways or proteins that regulate their spatial distribution.
The authors also performed a genome-wide association study on a sub-population of the natural rice accessions using seed shape and the spatial elemental details of the seeds from the µ-XRF analysis. They identified 11 significant SNPs linked to seed shape and 42 loci associated with elemental accumulation in the embryo (11 loci), endosperm (18), and aleurone layer (13). These findings constitute an interesting starting point for functional studies on elemental distribution in the seed and their substructures.
This work demonstrates that synchrotron-based fast X-ray fluorescence microscopy (µ-XRF) can be an effective and powerful elemental screening tool for seeds. µ-XRF can be used to detect toxic metals (arsenic, silicon, or cadmium) in grains, study effects of grain polishing or test heritability of nutrient traits for breeding. The synchrotron-based imaging set-up is often part of advanced research facilities available at select locations in the world. Routine screening would be reliant on beamtime availability and the high costs of synchrotron light. The advanced detectors greatly increase the speed of data acquisition and the feasibility of the method for screening. With continued progress in the beam sources and speed of acquisition, there is even scope for in situ time-resolved imaging in the future. Access to synchrotron-based systems could limit researchers, but the lab-based XRF used in this study could present an alternate, intermediate accessible set-up that enables spatial details with niche applications. We may foresee that rice grains may not be the last agronomically important seed to be screened by µ-XRF!
References
Author notes
Conflict of interest statement. None declared.