-
PDF
- Split View
-
Views
-
Cite
Cite
Akari E Maeda, Norihito Nakamichi, Plant clock modifications for adapting flowering time to local environments, Plant Physiology, Volume 190, Issue 2, October 2022, Pages 952–967, https://doi.org/10.1093/plphys/kiac107
- Share Icon Share
Abstract
During and after the domestication of crops from ancestral wild plants, humans selected cultivars that could change their flowering time in response to seasonal daylength. Continuous selection of this trait eventually allowed the introduction of crops into higher or lower latitudes and different climates from the original regions where domestication initiated. In the past two decades, numerous studies have found the causal genes or alleles that change flowering time and have assisted in adapting crop species such as barley (Hordeum vulgare), wheat (Triticum aestivum L.), rice (Oryza sativa L.), pea (Pisum sativum L.), maize (Zea mays spp. mays), and soybean (Glycine max (L.) Merr.) to new environments. This updated review summarizes the genes or alleles that contributed to crop adaptation in different climatic areas. Many of these genes are putative orthologs of Arabidopsis (Arabidopsis thaliana) core clock genes. We also discuss how knowledge of the clock’s molecular functioning can facilitate molecular breeding in the future.
Introduction
Humans domesticated crops from ancestral wild plants (Zohary et al., 2011). Domestication occurred by reducing several traits, including physical and chemical defenses, seed dehiscence, shoot number and seed dormancy, and increasing germination frequency and seed size. Collectively, these traits are known as the domestication syndrome (Larson et al., 2014). During or after domestication, flowering time suitable for the local climate was optimized. This optimization eventually enabled humans to grow the crops at higher or lower latitudes and different climatic zones. In the last two decades, genetic studies have identified genes responsible for changes in flowering time for many major crops (Bendix et al., 2015; Blumel et al., 2015; Nakamichi, 2015; Millar, 2016; Creux and Harmer, 2019; Gaudinier and Blackman, 2020; McClung, 2021; Steed et al., 2021). In some cases, orthologs of Arabidopsis (Arabidopsis thaliana) core circadian clock genes and light receptor genes were found to control crop flowering times. In addition, plant lineage-specific genes were also found to be key flowering regulators. In this update, we summarize recent comprehensive studies of crop genes and alleles that contributed toward optimizing photoperiodic flowering and permitted crop adaptation into higher or lower latitudes and different climatic regions. For a discussion of vernalization, the long-term cold treatment that breaks dormancy and induces flowering and another important physiological factor that contributed to crop adaptation, readers are referred elsewhere (Gaudinier and Blackman, 2020).
The circadian clock controls photoperiodic flowering time in Arabidopsis
Photoperiodic flowering induction, in which plants flower in response to daylength, was first reported ∼100 years ago (Garner and Allard, 1920). Erwin Bünning proposed that an internal timekeeping mechanism (clock) separates each day into the photophile (light-requiring) and the skotophile (dark-requiring) phases; if light interrupts the skotophile phase, long-day plants will flower and short-day plants will not flower (Bunning, 1960; Song et al., 2014). Pittendrigh and Minis proposed the external coincidence model in which coincidence between light input and the appropriate clock phase triggers flowering initiation (Pittendrigh and Minis, 1964). The activity of Arabidopsis CONSTANS (CO) accounts for the integration of the internal clock phase and external light cues for photoperiodic flowering (Yanovsky and Kay, 2002). CO expression is under the control of the clock, and light controls CO protein stability (Valverde et al., 2004).
The molecular basis for plant clocks has been studied mainly in Arabidopsis. The starting point was a screening for mutants that change the circadian period of genes fused to a luciferase reporter, followed by identification of the causal genes (Millar et al., 1995). In addition to the forward genetic approaches, reverse genetics, functional genetics, biochemical analyses, mathematical modeling, and chemical–biological investigations have revealed the molecular processes underpinning the Arabidopsis circadian clock (Nohales and Kay, 2016; Creux and Harmer, 2019; McClung, 2019; Ono et al., 2019; Saito et al., 2019; Uehara et al., 2019; Webb et al., 2019; Maric and Mas, 2020; Nohales, 2021; Yan et al., 2021). Also, analyses of mutants impaired in photoperiodic flowering identified core clock genes such as EARLY FLOWERING3 (ELF3), ELF4, GIGANTEA (GI), and LATE ELONGATED HYPOCOTYL (LHY) (Hicks et al., 1996; Schaffer et al., 1998; Fowler et al., 1999; Doyle et al., 2002). Most core clock genes encode transcriptional activators or repressors that together form a transcription–translation feedback loop. The transcriptional activators and repressors directly regulate the expression of key flowering-time genes such as CYCLIN DOF FACTORs (CDFs), FLAVIN BINDING, KELCH REPEAT, F-BOX1 (FKF1), CO, and FLOWERING LOCUS T (FT) in leaves (Song et al., 2014; Nakamichi, 2020). Core clock transcription factors that directly regulate key flowering-time genes are known; for example, CIRCADIAN CLOCK-ASSOCIATED1 (CCA1) and LHY repress FKF1 and GI, PSEUDO-RESPONSE REGULATORs (PRRs) repress CDFs, and ELF3, ELF4, and LUX form the evening complex and repress FT (Nakamichi et al., 2012; Liu et al., 2013; Nagel et al., 2015; Kamioka et al., 2016; Liu et al., 2016; Adams et al., 2018; Fukazawa et al., 2021). FT is transcribed under long-day conditions and encodes a flowering hormone that moves from leaves to the shoot apex where FLOWERING LOCUS T (FT) and FD, a basic leucine zipper (bZIP) protein, initiate flowering (Kardailsky et al., 1999; Kobayashi et al., 1999; Abe et al., 2005; Corbesier et al., 2007; Notaguchi et al., 2008).
Virtually all studies to identify molecular details of the clock and photoperiodic flowering time regulation were conducted in laboratories; however, a study showed that FT expression profiles in the field are not identical to those measured in laboratories and pointed out that as yet unknown genes may be responsible for the difference (Song et al., 2018). The genome-wide gene expression profiling for plants grown in the field is an attractive approach to consider the real molecular mechanisms for flowering time. Field transcriptome data have been reported for Arabidopsis, Arabidopsis halleri, and rice (Oryza sativa L.) (Nagano et al., 2012, 2019). In addition, chromatin immunoprecipitation coupled with deep sequencing for A. halleri grown in the field revealed seasonal plasticity and diel stability of histone H3 modifications (H3K27me3 and H3K4me3) in a genomic scale (Nishio et al., 2020). Collectively, it was suggested that fluctuating environmental cues such as temperature and light affect genome-wide gene expression. It is highly possible that temperature and light fluctuations in the field are mediated through light receptors and signaling components (phytochromes and PHOTOPERIODIC CONTROL OF HYPOCOTYL 1) and core clock components (such as ELF3, ELF4, LHY, CCA1, REVELLE4 [RVE4], and RVE8) because temperature and light directly change the activity, localization, or stability of these proteins (Jung et al., 2016, 2020; Legris et al., 2016; Huang et al., 2019; Chen et al., 2020; Silva et al., 2020; Kidokoro et al., 2021). Further studies are needed to fill the gap between our knowledge of molecular mechanisms revealed in the lab and actual mechanism for flowering regulation of plants in nature, although many causal genes for flowering time in crops in the field are orthologs of Arabidopsis core clock genes and photoreceptors.
Wheat (bread wheat, Triticum aestivum L.)
Wheat is a long-day plant that was domesticated in the Fertile Crescent more than 10,000 years ago. Wheat is now grown throughout the temperate, cold, and subtropical zones where climate and temperature are substantially different from the original region. Changing flowering time was one of the key traits allowing wheat to spread to new regions. In the early 20th century, the Italian wheat breeder Strampelli started a breeding project to make his country self-sufficient in wheat production. He crossed the Japanese wheat cultivar “Akakomugi” with European wheat progeny generated by crossing the Dutch cultivar “Wilhelmina Tare” and the Italian cultivar “Riete.” Strampelli found progeny with two useful traits: an early flowering trait that makes wheat escape the hot, dry summer, and the short-straw trait (semi-dwarf trait) that improves the grain/straw ratio (Figure 1; Borojevic and Borojevic, 2005). Further crossing created cultivars such as “San Pastore” that were grown for more than 35 years in Italy and other countries due to their early flowering and semi-dwarf habit (Borojevic and Borojevic, 2005). Progeny from the Strampelli breeding program, with their early flowering and semi-dwarf characteristics, increased wheat productivity more than three times in southern, central, and eastern Europe from 1969 to 1988 (Borojevic and Borojevic, 2005). Eastern European countries continue to grow the early flowering cultivars that are progeny from the Strampelli breeding program (Langer et al., 2014). In contrast, these early flowering varieties did not result in increased yields in the UK and Germany (Worland et al., 1998). Instead, an extended vegetative phase (late flowering) improves wheat yield in these regions and North America.
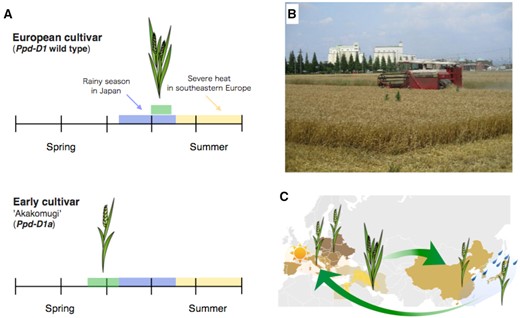
Wheat adaptation to local environments. A, Before the Strampelli breeding program, European cultivars flowered just before the dry summer (upper). The Japanese cultivar “Akakomugi” flowers before the rainy season in May–June (lower). B, The modern Japanese cultivar “Kinuakari” which is used for Japanese noodle (Udon), has grain ready to harvest at the beginning of June near Nagoya, Aichi Prefecture. C, Wheat was introduced into eastern Asia. The early flowering trait that escapes precocious germination on the ear was noted in old Chinese literatures (see text). “Akakomugi” was introduced into Italy by the Strampelli breeding program during the early 20th century.
Not only the Japanese cultivar “Akakomugi” that Strampelli used as a source of earliness and the semi-dwarf phenotype but also most Japanese cultivars must be harvested before the rainy season begins in June (Figure 1). Early flowering helps Japanese farmers escape the problem of precocious germination within the grain head (preharvest sprouting). Early flowering wheat might have already emerged in old China before the wheat was introduced to Japan because the old Chinese literatures such as “Zuo Zhuan” describe the harvesting and eating seasons of wheat ranging between May and September (Liu et al., 2017). Consistently, some Chinese wheat landraces have an early flowering phenotype similar to “Akakomugi” (Yang et al., 2009).
The wheat early flowering trait from “Akakomugi” is caused by a semi-dominant Photoperiod-D1a allele (Ppd-D1a, “a” means photoperiod-insensitive allele) that is found in most Japanese cultivars (Seki et al., 2011). The allele is associated with a 2-kbp deletion upstream from an ortholog of the Arabidopsis PRR7 gene (Beales et al., 2007; Table 1). Deleting the 2-kbp sequence causes misexpression of the PRR gene and also upregulates TaFT1, an Arabidopsis florigen ortholog (Beales et al., 2007). Because the Arabidopsis PRR7 protein acts as a transcriptional repressor (Nakamichi et al., 2010; Liu et al., 2013; Wang et al., 2013), Ppd-D1 may regulate TaFT1 expression via indirectly repressing the expression of some inhibitor genes of TaFT1. It is also possible that Ppd-D1 controls TaFT1 expression by binding transcriptional activators of TaFT1; similar to Arabidopsis PRR proteins binding to CO, a transcriptional activator of FT (Hayama et al., 2017). Ppd-D1a is an allele found on the D genome of the hexaploid bread wheat genome (ABD genome).
Arabidopsis gene . | Crop genes . | Flowering time of mutants . | Adaptation areas . | References . |
---|---|---|---|---|
PhyA | ppd (common bean, SDP) | Early in LD | Long day summer (Mexico, Andean) | Weller et al. (2019) |
E3 (soybean, SDP) | Early in LD | Long day summer | Tsubokura et al. (2014) | |
E4 (soybean, SDP) | Early in LD | Long day summer | Tsubokura et al. (2014) | |
PhyB | Ma3 (sorghum, SDP) | Early in LD | Long day summer (North America) | Childs et al. (1997) |
PhyC | EAM5 (barley, LDP) | Early in LD | Rain before harvesting (Japan) | Nishida et al. (2013b), Pankin et al. (2014) |
Ma5 (sorghum, SDP) | Early in LD | Long day summer (North America) | Yang et al. (2014) | |
LHY | Tof16 (soybean, SDP) | Late in SD | Short day (South America) | Dong et al. (2021) |
PRR7 | ppd-D1a (wheat, LDP) | Early in LD (gain-of-function) | Rain before harvesting (East Asia), dry summer (East Europe) | Beales et al. (2007), Langer et al. (2014) |
ppd-A1, ppd-D1 (wheat, LDP) | Late | Sweden | Shaw et al. (2013), Gauley and Boden (2021) | |
ppd-H1 (barley, LDP) | Late in LD | Long day summer (West Europe, North America) | Turner et al. (2005) | |
Hd2 (rice, SDP) | Early in LD | Long day summer (Northern East Asia, Europe, North America) | Koo et al. (2013), Yan et al. (2013), Guo et al. (2020) | |
Ma1 (sorghum, SDP) | Early in LD | Long day summer (North America) | Murphy et al. (2011) | |
Tof11, Tof12 (soybean, SDP) | Early in LD | During domestication (China) | Lu et al. (2020) | |
BvBTC1 (sugarbeet) | Biennial | Northern Europe | Pin et al. (2012) | |
ELF3 | EAM8 (barley, LDP) | Early in LD | Short growing areas (Scandinavia, Russia), rain before summer (Japan) | Zakhrabekova et al. (2012) |
Hd17 (rice, SDP) | Early (gain-of-function) | Early type is in Northern East Asia | Matsubara et al. (2012), Saito et al. (2012) | |
J (soybean, SDP) | Late in SD | Short day (South America) | Lu et al. (2017) | |
HR (pea, LDP) | Early | Short growing high latitude areas | Weller et al. (2012) | |
PPD (pea, LDP) | Early | Used in laboratory | Rubenach et al. (2017) | |
ELF4 | DNE (pea, LDP) | Early | Used in laboratory | Liew et al. (2009) |
LUX(PCL1) | WPCL (wheat, LDP) | Early | Rain before harvesting (East Asia) | Mizuno et al. (2016) |
EAM10 (barley, LDP) | Early | Short growing areas (Scandinavia) | Campoli et al. (2013) | |
SN (pea, LDP) | Early | Short growing areas (Alaska) | Liew et al. (2014) | |
GI | E2 (soybean, SDP) | Early | Long day summer (Northern East Asia) | Watanabe et al. (2011) |
Late1 (pea, LDP) | Late | Used in laboratory | Hecht et al. (2007) | |
CDF1 | Late2 (pea, LDP) | Late (gain-of-function) | Used in laboratory | Ridge et al. (2016) |
StCDF1 (potato, SDP) | Early (gain-of-function) | Long day | Kloosterman et al. (2013) | |
CO, COL | Hd1 (rice, SDP) | Late in SD, early in LD | Sub-tropical area (Taiwan) | Yano et al. (2000), Wei et al. (2016) |
DTH2 (rice, SDP) | Early in LD (gain-of-function) | East Asia | Wu et al. (2013) | |
COL2 (common bean, SDP) | Early in LD | Long day | Gonzalez et al. (2021) | |
HAP3 | Hd5 (rice, SDP) | Early in LD | Long day summer (North Japan), South Asia | Fujino et al. (2013), Guo et al. (2020) |
CK2 alpha | Hd6 (rice, SDP) | Early in LD | Long day summer (Northern East Asia, Europe, and North America), South Asia | Takahashi et al. (2001), Nemoto et al. (2018), Guo et al. (2020) |
MLK | Hd16 (rice, SDP) | Early in LD | Long day summer | Hori et al. (2013), Nemoto et al. (2018) |
LNK2 | LNK2 (tomato, SDP) | Not known (long circadian period) | Higher latitude | Muller et al. (2018) |
EID1 | EID1 (tomato, SDP) | Late (increased chlorophyll content in LD, phase delay, shorter height) | Higher latitude | Muller et al. (2016) |
FLD | Hd18 (rice, SDP) | Early (gain-of-function) | Japan | Shibaya et al. (2016) |
SOC1 | DTH3 (rice, SDP) | Late | Africa | Bian et al. (2011) |
TFL1 | LF (pea, LDP) | Early | Used in laboratory | Foucher et al. (2003) |
PvTFL1y (common bean, SDP) | Early | During domestication | Repinski et al. (2012), Koinange et al. (1996) | |
Type-B ARR | Ehd1 (rice, SDP) | Late | Sub-tropical area (Taiwan) | Doi et al. (2004), Wei et al. (2016) |
CDK8 | Late3 (pea, LDP) | Late | Used in laboratory | Hasan et al. (2020) |
CYCC1 | Late4 (pea, LDP) | Late | Used in laboratory | Hasan et al. (2020) |
not found, CCT-domain protein | Ghd7 (rice, SDP) | Early | Long day summer (Northern East Asia) | Xue et al. (2008), Koo et al. (2013) |
Ma6 (sorghum, SDP) | Early | Long day summer (North America) | Murphy et al. (2014) | |
ZmCCT (maize, SDP) | Early | Long day summer | Hung et al. (2012), Yang et al. (2013) | |
ZmCCT9 (maize, SDP) | Early | Long day summer | Huang et al. (2018) | |
not found, B3 DNA-binding protein | E1 (soybean, SDP) | Early | Long day summer | Xia et al. (2012) |
not found, SET domain protein | Ma2 (sorghum, SDP) | Early | Long day summer (North America) | Casto et al. (2019) |
FT | Ppd-H2 (barley, LDP) | Late | Cold spring | Kikuchi et al. (2009), Casao et al. (2011) |
Hd3a (rice, SDP) | Late in SD (Nipponbare-type) | Japan | Kojima et al. (2002) | |
GIGAS (pea, LDP) | Late | Used in laboratory | Hecht et al. (2011) | |
ZCN8, ZCN12 (maize, LDP) | Early (gain-of-function, increased expression) | Long day summer | Guo et al. (2018), Castelletti et al. (2020) | |
SP5G (repressor type, tomato, SDP) | Early in LD | Long day | Soyk et al. (2017) |
Arabidopsis gene . | Crop genes . | Flowering time of mutants . | Adaptation areas . | References . |
---|---|---|---|---|
PhyA | ppd (common bean, SDP) | Early in LD | Long day summer (Mexico, Andean) | Weller et al. (2019) |
E3 (soybean, SDP) | Early in LD | Long day summer | Tsubokura et al. (2014) | |
E4 (soybean, SDP) | Early in LD | Long day summer | Tsubokura et al. (2014) | |
PhyB | Ma3 (sorghum, SDP) | Early in LD | Long day summer (North America) | Childs et al. (1997) |
PhyC | EAM5 (barley, LDP) | Early in LD | Rain before harvesting (Japan) | Nishida et al. (2013b), Pankin et al. (2014) |
Ma5 (sorghum, SDP) | Early in LD | Long day summer (North America) | Yang et al. (2014) | |
LHY | Tof16 (soybean, SDP) | Late in SD | Short day (South America) | Dong et al. (2021) |
PRR7 | ppd-D1a (wheat, LDP) | Early in LD (gain-of-function) | Rain before harvesting (East Asia), dry summer (East Europe) | Beales et al. (2007), Langer et al. (2014) |
ppd-A1, ppd-D1 (wheat, LDP) | Late | Sweden | Shaw et al. (2013), Gauley and Boden (2021) | |
ppd-H1 (barley, LDP) | Late in LD | Long day summer (West Europe, North America) | Turner et al. (2005) | |
Hd2 (rice, SDP) | Early in LD | Long day summer (Northern East Asia, Europe, North America) | Koo et al. (2013), Yan et al. (2013), Guo et al. (2020) | |
Ma1 (sorghum, SDP) | Early in LD | Long day summer (North America) | Murphy et al. (2011) | |
Tof11, Tof12 (soybean, SDP) | Early in LD | During domestication (China) | Lu et al. (2020) | |
BvBTC1 (sugarbeet) | Biennial | Northern Europe | Pin et al. (2012) | |
ELF3 | EAM8 (barley, LDP) | Early in LD | Short growing areas (Scandinavia, Russia), rain before summer (Japan) | Zakhrabekova et al. (2012) |
Hd17 (rice, SDP) | Early (gain-of-function) | Early type is in Northern East Asia | Matsubara et al. (2012), Saito et al. (2012) | |
J (soybean, SDP) | Late in SD | Short day (South America) | Lu et al. (2017) | |
HR (pea, LDP) | Early | Short growing high latitude areas | Weller et al. (2012) | |
PPD (pea, LDP) | Early | Used in laboratory | Rubenach et al. (2017) | |
ELF4 | DNE (pea, LDP) | Early | Used in laboratory | Liew et al. (2009) |
LUX(PCL1) | WPCL (wheat, LDP) | Early | Rain before harvesting (East Asia) | Mizuno et al. (2016) |
EAM10 (barley, LDP) | Early | Short growing areas (Scandinavia) | Campoli et al. (2013) | |
SN (pea, LDP) | Early | Short growing areas (Alaska) | Liew et al. (2014) | |
GI | E2 (soybean, SDP) | Early | Long day summer (Northern East Asia) | Watanabe et al. (2011) |
Late1 (pea, LDP) | Late | Used in laboratory | Hecht et al. (2007) | |
CDF1 | Late2 (pea, LDP) | Late (gain-of-function) | Used in laboratory | Ridge et al. (2016) |
StCDF1 (potato, SDP) | Early (gain-of-function) | Long day | Kloosterman et al. (2013) | |
CO, COL | Hd1 (rice, SDP) | Late in SD, early in LD | Sub-tropical area (Taiwan) | Yano et al. (2000), Wei et al. (2016) |
DTH2 (rice, SDP) | Early in LD (gain-of-function) | East Asia | Wu et al. (2013) | |
COL2 (common bean, SDP) | Early in LD | Long day | Gonzalez et al. (2021) | |
HAP3 | Hd5 (rice, SDP) | Early in LD | Long day summer (North Japan), South Asia | Fujino et al. (2013), Guo et al. (2020) |
CK2 alpha | Hd6 (rice, SDP) | Early in LD | Long day summer (Northern East Asia, Europe, and North America), South Asia | Takahashi et al. (2001), Nemoto et al. (2018), Guo et al. (2020) |
MLK | Hd16 (rice, SDP) | Early in LD | Long day summer | Hori et al. (2013), Nemoto et al. (2018) |
LNK2 | LNK2 (tomato, SDP) | Not known (long circadian period) | Higher latitude | Muller et al. (2018) |
EID1 | EID1 (tomato, SDP) | Late (increased chlorophyll content in LD, phase delay, shorter height) | Higher latitude | Muller et al. (2016) |
FLD | Hd18 (rice, SDP) | Early (gain-of-function) | Japan | Shibaya et al. (2016) |
SOC1 | DTH3 (rice, SDP) | Late | Africa | Bian et al. (2011) |
TFL1 | LF (pea, LDP) | Early | Used in laboratory | Foucher et al. (2003) |
PvTFL1y (common bean, SDP) | Early | During domestication | Repinski et al. (2012), Koinange et al. (1996) | |
Type-B ARR | Ehd1 (rice, SDP) | Late | Sub-tropical area (Taiwan) | Doi et al. (2004), Wei et al. (2016) |
CDK8 | Late3 (pea, LDP) | Late | Used in laboratory | Hasan et al. (2020) |
CYCC1 | Late4 (pea, LDP) | Late | Used in laboratory | Hasan et al. (2020) |
not found, CCT-domain protein | Ghd7 (rice, SDP) | Early | Long day summer (Northern East Asia) | Xue et al. (2008), Koo et al. (2013) |
Ma6 (sorghum, SDP) | Early | Long day summer (North America) | Murphy et al. (2014) | |
ZmCCT (maize, SDP) | Early | Long day summer | Hung et al. (2012), Yang et al. (2013) | |
ZmCCT9 (maize, SDP) | Early | Long day summer | Huang et al. (2018) | |
not found, B3 DNA-binding protein | E1 (soybean, SDP) | Early | Long day summer | Xia et al. (2012) |
not found, SET domain protein | Ma2 (sorghum, SDP) | Early | Long day summer (North America) | Casto et al. (2019) |
FT | Ppd-H2 (barley, LDP) | Late | Cold spring | Kikuchi et al. (2009), Casao et al. (2011) |
Hd3a (rice, SDP) | Late in SD (Nipponbare-type) | Japan | Kojima et al. (2002) | |
GIGAS (pea, LDP) | Late | Used in laboratory | Hecht et al. (2011) | |
ZCN8, ZCN12 (maize, LDP) | Early (gain-of-function, increased expression) | Long day summer | Guo et al. (2018), Castelletti et al. (2020) | |
SP5G (repressor type, tomato, SDP) | Early in LD | Long day | Soyk et al. (2017) |
SDP and LDP indicate short-day plants and long-day plants, respectively.
Arabidopsis gene . | Crop genes . | Flowering time of mutants . | Adaptation areas . | References . |
---|---|---|---|---|
PhyA | ppd (common bean, SDP) | Early in LD | Long day summer (Mexico, Andean) | Weller et al. (2019) |
E3 (soybean, SDP) | Early in LD | Long day summer | Tsubokura et al. (2014) | |
E4 (soybean, SDP) | Early in LD | Long day summer | Tsubokura et al. (2014) | |
PhyB | Ma3 (sorghum, SDP) | Early in LD | Long day summer (North America) | Childs et al. (1997) |
PhyC | EAM5 (barley, LDP) | Early in LD | Rain before harvesting (Japan) | Nishida et al. (2013b), Pankin et al. (2014) |
Ma5 (sorghum, SDP) | Early in LD | Long day summer (North America) | Yang et al. (2014) | |
LHY | Tof16 (soybean, SDP) | Late in SD | Short day (South America) | Dong et al. (2021) |
PRR7 | ppd-D1a (wheat, LDP) | Early in LD (gain-of-function) | Rain before harvesting (East Asia), dry summer (East Europe) | Beales et al. (2007), Langer et al. (2014) |
ppd-A1, ppd-D1 (wheat, LDP) | Late | Sweden | Shaw et al. (2013), Gauley and Boden (2021) | |
ppd-H1 (barley, LDP) | Late in LD | Long day summer (West Europe, North America) | Turner et al. (2005) | |
Hd2 (rice, SDP) | Early in LD | Long day summer (Northern East Asia, Europe, North America) | Koo et al. (2013), Yan et al. (2013), Guo et al. (2020) | |
Ma1 (sorghum, SDP) | Early in LD | Long day summer (North America) | Murphy et al. (2011) | |
Tof11, Tof12 (soybean, SDP) | Early in LD | During domestication (China) | Lu et al. (2020) | |
BvBTC1 (sugarbeet) | Biennial | Northern Europe | Pin et al. (2012) | |
ELF3 | EAM8 (barley, LDP) | Early in LD | Short growing areas (Scandinavia, Russia), rain before summer (Japan) | Zakhrabekova et al. (2012) |
Hd17 (rice, SDP) | Early (gain-of-function) | Early type is in Northern East Asia | Matsubara et al. (2012), Saito et al. (2012) | |
J (soybean, SDP) | Late in SD | Short day (South America) | Lu et al. (2017) | |
HR (pea, LDP) | Early | Short growing high latitude areas | Weller et al. (2012) | |
PPD (pea, LDP) | Early | Used in laboratory | Rubenach et al. (2017) | |
ELF4 | DNE (pea, LDP) | Early | Used in laboratory | Liew et al. (2009) |
LUX(PCL1) | WPCL (wheat, LDP) | Early | Rain before harvesting (East Asia) | Mizuno et al. (2016) |
EAM10 (barley, LDP) | Early | Short growing areas (Scandinavia) | Campoli et al. (2013) | |
SN (pea, LDP) | Early | Short growing areas (Alaska) | Liew et al. (2014) | |
GI | E2 (soybean, SDP) | Early | Long day summer (Northern East Asia) | Watanabe et al. (2011) |
Late1 (pea, LDP) | Late | Used in laboratory | Hecht et al. (2007) | |
CDF1 | Late2 (pea, LDP) | Late (gain-of-function) | Used in laboratory | Ridge et al. (2016) |
StCDF1 (potato, SDP) | Early (gain-of-function) | Long day | Kloosterman et al. (2013) | |
CO, COL | Hd1 (rice, SDP) | Late in SD, early in LD | Sub-tropical area (Taiwan) | Yano et al. (2000), Wei et al. (2016) |
DTH2 (rice, SDP) | Early in LD (gain-of-function) | East Asia | Wu et al. (2013) | |
COL2 (common bean, SDP) | Early in LD | Long day | Gonzalez et al. (2021) | |
HAP3 | Hd5 (rice, SDP) | Early in LD | Long day summer (North Japan), South Asia | Fujino et al. (2013), Guo et al. (2020) |
CK2 alpha | Hd6 (rice, SDP) | Early in LD | Long day summer (Northern East Asia, Europe, and North America), South Asia | Takahashi et al. (2001), Nemoto et al. (2018), Guo et al. (2020) |
MLK | Hd16 (rice, SDP) | Early in LD | Long day summer | Hori et al. (2013), Nemoto et al. (2018) |
LNK2 | LNK2 (tomato, SDP) | Not known (long circadian period) | Higher latitude | Muller et al. (2018) |
EID1 | EID1 (tomato, SDP) | Late (increased chlorophyll content in LD, phase delay, shorter height) | Higher latitude | Muller et al. (2016) |
FLD | Hd18 (rice, SDP) | Early (gain-of-function) | Japan | Shibaya et al. (2016) |
SOC1 | DTH3 (rice, SDP) | Late | Africa | Bian et al. (2011) |
TFL1 | LF (pea, LDP) | Early | Used in laboratory | Foucher et al. (2003) |
PvTFL1y (common bean, SDP) | Early | During domestication | Repinski et al. (2012), Koinange et al. (1996) | |
Type-B ARR | Ehd1 (rice, SDP) | Late | Sub-tropical area (Taiwan) | Doi et al. (2004), Wei et al. (2016) |
CDK8 | Late3 (pea, LDP) | Late | Used in laboratory | Hasan et al. (2020) |
CYCC1 | Late4 (pea, LDP) | Late | Used in laboratory | Hasan et al. (2020) |
not found, CCT-domain protein | Ghd7 (rice, SDP) | Early | Long day summer (Northern East Asia) | Xue et al. (2008), Koo et al. (2013) |
Ma6 (sorghum, SDP) | Early | Long day summer (North America) | Murphy et al. (2014) | |
ZmCCT (maize, SDP) | Early | Long day summer | Hung et al. (2012), Yang et al. (2013) | |
ZmCCT9 (maize, SDP) | Early | Long day summer | Huang et al. (2018) | |
not found, B3 DNA-binding protein | E1 (soybean, SDP) | Early | Long day summer | Xia et al. (2012) |
not found, SET domain protein | Ma2 (sorghum, SDP) | Early | Long day summer (North America) | Casto et al. (2019) |
FT | Ppd-H2 (barley, LDP) | Late | Cold spring | Kikuchi et al. (2009), Casao et al. (2011) |
Hd3a (rice, SDP) | Late in SD (Nipponbare-type) | Japan | Kojima et al. (2002) | |
GIGAS (pea, LDP) | Late | Used in laboratory | Hecht et al. (2011) | |
ZCN8, ZCN12 (maize, LDP) | Early (gain-of-function, increased expression) | Long day summer | Guo et al. (2018), Castelletti et al. (2020) | |
SP5G (repressor type, tomato, SDP) | Early in LD | Long day | Soyk et al. (2017) |
Arabidopsis gene . | Crop genes . | Flowering time of mutants . | Adaptation areas . | References . |
---|---|---|---|---|
PhyA | ppd (common bean, SDP) | Early in LD | Long day summer (Mexico, Andean) | Weller et al. (2019) |
E3 (soybean, SDP) | Early in LD | Long day summer | Tsubokura et al. (2014) | |
E4 (soybean, SDP) | Early in LD | Long day summer | Tsubokura et al. (2014) | |
PhyB | Ma3 (sorghum, SDP) | Early in LD | Long day summer (North America) | Childs et al. (1997) |
PhyC | EAM5 (barley, LDP) | Early in LD | Rain before harvesting (Japan) | Nishida et al. (2013b), Pankin et al. (2014) |
Ma5 (sorghum, SDP) | Early in LD | Long day summer (North America) | Yang et al. (2014) | |
LHY | Tof16 (soybean, SDP) | Late in SD | Short day (South America) | Dong et al. (2021) |
PRR7 | ppd-D1a (wheat, LDP) | Early in LD (gain-of-function) | Rain before harvesting (East Asia), dry summer (East Europe) | Beales et al. (2007), Langer et al. (2014) |
ppd-A1, ppd-D1 (wheat, LDP) | Late | Sweden | Shaw et al. (2013), Gauley and Boden (2021) | |
ppd-H1 (barley, LDP) | Late in LD | Long day summer (West Europe, North America) | Turner et al. (2005) | |
Hd2 (rice, SDP) | Early in LD | Long day summer (Northern East Asia, Europe, North America) | Koo et al. (2013), Yan et al. (2013), Guo et al. (2020) | |
Ma1 (sorghum, SDP) | Early in LD | Long day summer (North America) | Murphy et al. (2011) | |
Tof11, Tof12 (soybean, SDP) | Early in LD | During domestication (China) | Lu et al. (2020) | |
BvBTC1 (sugarbeet) | Biennial | Northern Europe | Pin et al. (2012) | |
ELF3 | EAM8 (barley, LDP) | Early in LD | Short growing areas (Scandinavia, Russia), rain before summer (Japan) | Zakhrabekova et al. (2012) |
Hd17 (rice, SDP) | Early (gain-of-function) | Early type is in Northern East Asia | Matsubara et al. (2012), Saito et al. (2012) | |
J (soybean, SDP) | Late in SD | Short day (South America) | Lu et al. (2017) | |
HR (pea, LDP) | Early | Short growing high latitude areas | Weller et al. (2012) | |
PPD (pea, LDP) | Early | Used in laboratory | Rubenach et al. (2017) | |
ELF4 | DNE (pea, LDP) | Early | Used in laboratory | Liew et al. (2009) |
LUX(PCL1) | WPCL (wheat, LDP) | Early | Rain before harvesting (East Asia) | Mizuno et al. (2016) |
EAM10 (barley, LDP) | Early | Short growing areas (Scandinavia) | Campoli et al. (2013) | |
SN (pea, LDP) | Early | Short growing areas (Alaska) | Liew et al. (2014) | |
GI | E2 (soybean, SDP) | Early | Long day summer (Northern East Asia) | Watanabe et al. (2011) |
Late1 (pea, LDP) | Late | Used in laboratory | Hecht et al. (2007) | |
CDF1 | Late2 (pea, LDP) | Late (gain-of-function) | Used in laboratory | Ridge et al. (2016) |
StCDF1 (potato, SDP) | Early (gain-of-function) | Long day | Kloosterman et al. (2013) | |
CO, COL | Hd1 (rice, SDP) | Late in SD, early in LD | Sub-tropical area (Taiwan) | Yano et al. (2000), Wei et al. (2016) |
DTH2 (rice, SDP) | Early in LD (gain-of-function) | East Asia | Wu et al. (2013) | |
COL2 (common bean, SDP) | Early in LD | Long day | Gonzalez et al. (2021) | |
HAP3 | Hd5 (rice, SDP) | Early in LD | Long day summer (North Japan), South Asia | Fujino et al. (2013), Guo et al. (2020) |
CK2 alpha | Hd6 (rice, SDP) | Early in LD | Long day summer (Northern East Asia, Europe, and North America), South Asia | Takahashi et al. (2001), Nemoto et al. (2018), Guo et al. (2020) |
MLK | Hd16 (rice, SDP) | Early in LD | Long day summer | Hori et al. (2013), Nemoto et al. (2018) |
LNK2 | LNK2 (tomato, SDP) | Not known (long circadian period) | Higher latitude | Muller et al. (2018) |
EID1 | EID1 (tomato, SDP) | Late (increased chlorophyll content in LD, phase delay, shorter height) | Higher latitude | Muller et al. (2016) |
FLD | Hd18 (rice, SDP) | Early (gain-of-function) | Japan | Shibaya et al. (2016) |
SOC1 | DTH3 (rice, SDP) | Late | Africa | Bian et al. (2011) |
TFL1 | LF (pea, LDP) | Early | Used in laboratory | Foucher et al. (2003) |
PvTFL1y (common bean, SDP) | Early | During domestication | Repinski et al. (2012), Koinange et al. (1996) | |
Type-B ARR | Ehd1 (rice, SDP) | Late | Sub-tropical area (Taiwan) | Doi et al. (2004), Wei et al. (2016) |
CDK8 | Late3 (pea, LDP) | Late | Used in laboratory | Hasan et al. (2020) |
CYCC1 | Late4 (pea, LDP) | Late | Used in laboratory | Hasan et al. (2020) |
not found, CCT-domain protein | Ghd7 (rice, SDP) | Early | Long day summer (Northern East Asia) | Xue et al. (2008), Koo et al. (2013) |
Ma6 (sorghum, SDP) | Early | Long day summer (North America) | Murphy et al. (2014) | |
ZmCCT (maize, SDP) | Early | Long day summer | Hung et al. (2012), Yang et al. (2013) | |
ZmCCT9 (maize, SDP) | Early | Long day summer | Huang et al. (2018) | |
not found, B3 DNA-binding protein | E1 (soybean, SDP) | Early | Long day summer | Xia et al. (2012) |
not found, SET domain protein | Ma2 (sorghum, SDP) | Early | Long day summer (North America) | Casto et al. (2019) |
FT | Ppd-H2 (barley, LDP) | Late | Cold spring | Kikuchi et al. (2009), Casao et al. (2011) |
Hd3a (rice, SDP) | Late in SD (Nipponbare-type) | Japan | Kojima et al. (2002) | |
GIGAS (pea, LDP) | Late | Used in laboratory | Hecht et al. (2011) | |
ZCN8, ZCN12 (maize, LDP) | Early (gain-of-function, increased expression) | Long day summer | Guo et al. (2018), Castelletti et al. (2020) | |
SP5G (repressor type, tomato, SDP) | Early in LD | Long day | Soyk et al. (2017) |
SDP and LDP indicate short-day plants and long-day plants, respectively.
Another PPD insensitiveness source, Ppd-B1a, is caused by an increased copy number of the Ppd-B1 gene in the B genome (Diaz et al., 2012). Early flowering is also achieved by deleting the putative promoter region of the Ppd-B1 (Nishida et al., 2013b). Ppd-A1a alleles have a deletion in the 5′-upstream region of PRR in the A genome of bread and durum (tetraploid) wheat (Wilhelm et al., 2009; Nishida et al., 2013b). The Ppd-A1a and Ppd-B1a alleles are not common in wheat cultivars, so their contribution to wheat adaption to local areas in the past is unclear (Seki et al., 2013; Nishida et al., 2013b).
Triple loss-of-function mutations in Ppd-A1, Ppd-B1, and Ppd-D1 result in late flowering (Shaw et al., 2013). Loss-of-function alleles of Ppd-D1 are frequently found in wheat varieties in the A.E. Watkins collection and the Gediflux collection (Wingen et al., 2014), mainly gathered from northern and western Europe and Australia; the loss-of-function allele of Ppd-A1 is frequently found in Sweden (Shaw et al., 2013). Late flowering results in an increased spikelet number (Gauley and Boden, 2021), suggesting that loss-of-function of Ppd-A1 and/or Ppd-D1 may contribute to grain yield in certain climatic regions.
The super-early flowering Japanese cultivar “Chogukuwase” was found to have Ppd-D1a plus additional mutations (loss-of-function mutations in three putative orthologs of the Arabidopsis LUX/PHYTOCLOCK1 genes, WPCL-A1, WPCL-B1, and WPCL-D1) that cause PPD insensitiveness (Mizuno et al., 2016). Expression of the Ppd-A1 and Ppd-B1 genes is upregulated in “Chogukuwase,” suggesting that WPCL represses these Ppd-1 genes. This hypothesis is based on a molecular linkage between the LUX protein and the PRR9 and PRR7 genes in Arabidopsis (Helfer et al., 2011; Ezer et al., 2017). The earliness per se D1 (Eps-D1) allele has contributed to the fine tuning of flowering time. A deletion in the genomic region that includes the ELF3 gene corresponds to the Esp-D1 early flowering allele (Zikhali et al., 2016). The flowering phenotype controlled by Eps-D1 is pronounced at lower temperatures (Ochagavia et al., 2019), suggesting an interaction between ELF3 and temperature in wheat, similar to that reported in Arabidopsis (Jung et al., 2020). The Eps-Am gene in einkorn wheat is very likely an ortholog of ELF3 (Alvarez et al., 2016).
Barley (Hordeum vulgare)
Barley is a long-day plant that was also domesticated in the Fertile Crescent and has spread throughout the world. Changing the vernalization requirement and/or the photoperiodic flowering time response enabled barley to expand into divergent latitudes and local areas with climates different from the Fertile Crescent. PPD-H1 and PPD-H2 are regarded as major determinants for photoperiodic flowering in barley (Laurie et al., 1995; Fernandez-Calleja et al., 2021). Ppd-H1 encodes a PRR (Turner et al., 2005; Table 1). Spring barley varieties in western Europe and North America have a recessive ppd-H1 allele, causing late flowering and extension of the vegetative growth phase that eventually increases yields (Figure 2; Turner et al., 2005). Although Ppd-H1 confers early flowering in general, Ppd-H1 can also delay flowering in a specific environment or genetic background (Fernandez-Calleja et al., 2021). Phenotypes of Arabidopsis prr7 mutants are also conditional or genetic background dependent. For example, the long period of prr7 prr9 is diminished at a lower temperature (Salome et al., 2010), the period length of prr7 is light dependent (Farre et al., 2005), prr7 mutations shorten the period in the prr5 mutant background (Nakamichi et al., 2005b), and flowering in prr7 is delayed in long days but accelerated in short days (Nakamichi et al., 2005a). In addition, PRR7 mRNA expression is temperature dependent (Mizuno et al., 2014), and photosynthetic sugar represses PRR7 expression (Haydon et al., 2013; Seki et al., 2017). The interaction between PRR and environmental, metabolic, and genetic factors may be responsible for the phenotype’s complexity.
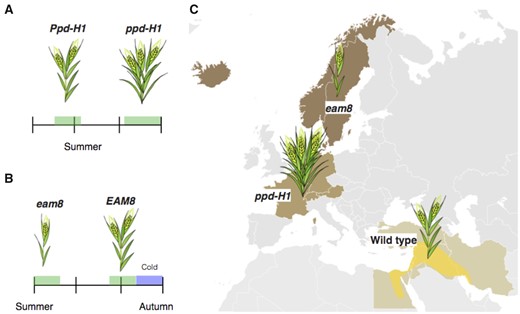
Barley adaptation to local environments. A, In western Europe, ppd-H1 alleles flower later than Ppd-H1 alleles, providing additional leaves that increase grain yield if the area is suitable for barley growth. B, eam8 (mat-a) mutations cause early flowering, enabling grain harvest before the onset of the cold season in northern Europe. C, Geographic distribution of barley cultivars.
PPD-H2 was known as a locus affecting flowering time under short-day conditions when barley flowers late. PPD-H2 is HvFT3, an FT ortholog (Kikuchi et al., 2009). HvFT1 triggers flowering under long days, whereas HvFT3 is expressed more abundantly during short days than long days. A loss-of-function allele that exhibits late flowering, ppd-h2 is preferentially found in plants growing in high-latitude regions (over 44˚N in Europe; Casao et al., 2011). Flowering induction under short-day conditions such as early spring was likely unfavorable in these regions due to damage from cold stress (Casao et al., 2011).
The Swedish Seed Association selected early-flowering barley varieties from ionizing irradiation-treated mutants (Zakhrabekova et al., 2012). The praematurum-a (mat-a) was such a mutant and was allelic to erectoides-o.16 and early maturity 8 (eam8) mutants (Zakhrabekova et al., 2012). Early flowering barley is now used in regions with short growing seasons, such as Scandinavia and Iceland (Figure 2). Early flowering cultivars are also used near the equator to harvest grains several times per year (Zakhrabekova et al., 2012). The causal gene for the early flowering cultivars is an ortholog of Arabidopsis ELF3, a component of the EC (Faure et al., 2012; Zakhrabekova et al., 2012). The causal gene for eam10 was identified as an ortholog of Arabidopsis LUX, a component of the EC (Campoli et al., 2013). The causal gene for eam5 was determined to be a Phytochrome C (PhyC) (Pankin et al., 2014). In parallel, the early flowering of “Hayakiso 2” (HK2) was found to be due to a mutation in PhyC (Nishida et al., 2013a). Both HK2 and eam5 mutations result in the same nonsynonymous amino acid substitution. The mutant HK2/eam5-type phyC may be hyperactive because the mutant-type phyC complemented the flowering time of a rice phyA phyC double mutant (Nishida et al., 2013a).
The eam6/eps2/mat-c mutation results in early flowering and is frequently found in winter barley varieties from the Mediterranean. Early flowering helps barley escape from droughts that cause low yields. The causal gene for eam6/eps2/mat-c is an ortholog of Arabidopsis TERMINAL FLOWER 1 (TFL1; Comadran et al., 2012) that suppresses flowering by competing with FT (Zhu et al., 2020).
Rice (O. sativa L.)
Flowering time in rice (heading) has been extensively studied by molecular genetic studies, such as the identification of a quantitative trait locus (QTL) controlling heading time (Itoh and Izawa, 2013; Matsubara et al., 2014; Izawa, 2021). Identification of several QTL responsible for differences in flowering time (heading date [Hd]) between two rice cultivars, japonica rice “Nipponbare” and indica rice “Kasalath,” suggested that rice employs a similar but distinct mechanism for regulating flowering time compared to Arabidopsis (Yano et al., 2000; Takahashi et al., 2001; Kojima et al., 2002; Tamaki et al., 2007; Taoka et al., 2011; Matsubara et al., 2012; Saito et al., 2012; Fujino et al., 2013; Hori et al., 2013; Koo et al., 2013; Shibaya et al., 2016; Nemoto et al., 2018; Table 1). QTL studies using other parental rice cultivars identified RICEFT1, earlyHd1 (Ehd1), Ghd7 (for grain number, plant height, andHd), for days to heading on chromosome2 (DTH2), and DTH3 (Doi et al., 2004; Xue et al., 2008; Bian et al., 2011; Ogiso-Tanaka et al., 2013; Wu et al., 2013; Table 1). The rice photoperiodic pathway is somewhat similar to that of Arabidopsis but also contains genes not found in Arabidopsis. Clock-CO(Hd1)-FT(Hd3a) is a conserved pathway; however, CO activates FT in Arabidopsis, whereas Hd1 represses Hd3a in rice under long-day conditions (Hayama et al., 2003). This difference explains Hd3a induction during short days rather than during long days in rice. The orthologous rice Ghd7-Ehd1 module is not found in Arabidopsis but is conserved in short-day monocots (Matsubara et al., 2014). The Ghd7-Ehd1 module is required to precisely determine day length (Itoh et al., 2010).
Asian rice, including japonica and indica rice, was domesticated near the Yangtze River in China and is now widely grown throughout Asia. Rice was originally a short-day plant and was not suitable for harvesting in the north where the day length is long during summer (Izawa, 2007). The northern limit of ancestral rice is estimated at around 31°N (Izawa, 2007); however, even in high latitude areas such as Europe, North America, the far-eastern parts of Russia, northern China, and northern Japan (Hokkaido Island), farmers can now grow and harvest modern rice cultivars. Selection of elite rice cultivars in which the photoperiodic response is reduced means that rice flowers in such northern areas even under noninductive long-day conditions in the summer (Koo et al., 2013). Double mutations in OsPRR73/Hd2/Ghd7.1 and Ghd7 cause rice to flower under natural long-day conditions and contribute to the adaptation toward northern areas over 40˚N (Figure 3; Koo et al., 2013; Yan et al., 2013). Diverse photoperiod sensitivity and flowering time traits have been optimized through on-demand selection in local areas and regions (Koo et al., 2013). Analysis of the geographic distribution of 3,010 rice accessions revealed that hd2 hd6 mutants are predominantly found in northern areas such as northeastern Asia, Europe, and North America, whereas the hd5 hd6 mutants are enriched in South Asia (Guo et al., 2020). For adaptation to Taiwan, a sub-tropical region with warm weather and a narrow photoperiod range (10.8–13.3 h), double mutations of Hd1 and Ehd1 that result in late-flowering occurred (Wei et al., 2016). Late flowering has resulted in extended periods of vegetative growth and additional photosynthetic organs, eventually resulting in higher yields. Collectively, humans have expanded the suitable growing areas for rice by selecting a variety of mutants in the photoperiodic pathway.
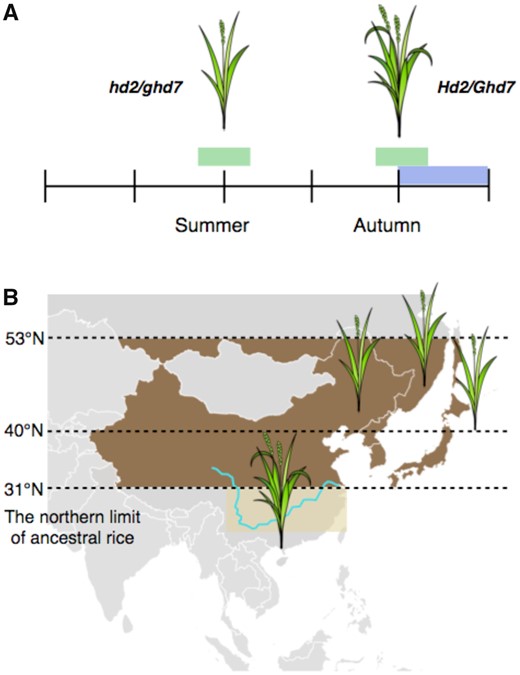
Rice adaptation into northern latitudes. A, Rice flowering is inhibited by long days in the summer at higher latitudes. hd2/ghd7 double mutants flower even under long days, enabling grain maturation before the cold season. B, The early flowering cultivars are now grown beyond the northern limits of ancient rice cultivation.
Sorghum (Sorghum bicolor)
Sorghum is a short-day plant but is thought to have acquired mutations by which flowering is induced under long-day conditions during the early stages of domestication in East Africa (Craufurd et al., 1999; Larson et al., 2014). Sorghum is used for two distinct purposes. One use is as a food crop (grain sorghum), and the other use is as an energy crop to provide lignocellulosic-based biofuels (sweet sorghum) (Murphy et al., 2011). Several genetic loci in sorghum are involved in regulating flowering time or maturity, Ma1 to Ma6 (Murphy et al., 2011). Between 1900 and 1940, an early flowering recessive allele Ma1 was selected by breeders in the USA. The early flowering Ma1 allele can flower under natural long-day conditions and has been used in breeding grain sorghum (Murphy et al., 2011). The Ma1, Ma2, Ma3, and Ma6 loci were identified as SbPRR37, a SET domain gene, PhyB, and Ghd7 ortholog, respectively (Childs et al., 1997; Murphy et al., 2011, 2014; Casto et al., 2019). Sequence analysis suggests that Ma5 is probably a PhyC ortholog (Yang et al., 2014).
The double-recessive mutations of Ma1 (SbPRR37) and Ma6 (SbGhd7) contributed to the birth of varieties with weak photoperiodism and the ability to flower during a noninductive photoperiod, such as long days over 12.5 h in the summer in the USA (Murphy et al., 2014). The relationship among double mutations of PRR37 and Ghd7, early flowering under long days, and adaptation to northern regions are common features in the adaptation of rice and sorghum to new environments (Koo et al., 2013; Murphy et al., 2014).
Maize (Zea mays spp. mays)
Approximately 9,000 years ago, maize (Z.mays spp. mays) was domesticated from teosinte, Z.mays ssp. parviglumis, a wild plant that grows in southwestern Mexico (Matsuoka et al., 2002). The southwestern Amazon is recognized as a secondary center for maize improvement post domestication (Kistler et al., 2018). Teosinte is a short-day plant and flowers very late or not at all when day lengths are greater than 13 h, whereas most modern maize cultivars can flower in long days (Hung et al., 2012). Genetic analyses using maize and teosinte as parental plants revealed that mutations in ZmCCT, an ortholog of Ghd7, impaired photoperiod sensitivity and caused the plant to flower under long-day conditions during the growing season at high latitudes (Hung et al., 2012; Yang et al., 2013). A mutation in another Ghd7 ortholog, ZmCCT9, was implicated in the spreading of maize throughout the North and South American continents (Huang et al., 2018). Furthermore, alternations in cis-regulatory regions for ZEA CENTRORADIALIS 8 (ZCN8) that encodes the maize florigen (Lazakis et al., 2011; Meng et al., 2011) conferred flowering under long-day conditions in the summer at high latitudes (Guo et al., 2018). Expression changes in another FT ortholog, ZCN12, are also implicated in the photoperiod insensitivity of maize compared to teosinte (Castelletti et al., 2020). The expression of ZCN8 is suppressed in teosinte, a short-day plant, under long-day conditions, whereas ZCN8 expression in day-neutral maize is not influenced by day length (Lazakis et al., 2011; Meng et al., 2011). Two ZmCCT genes in teosinte suppress ZCN8 expression under long-day conditions (Yang et al., 2013; Huang et al., 2018). Teosinte had not grown at higher latitudes due to long daylength in the summer, but invaded Europe in last century. The invaded teosinte flowers even under long-day conditions, due to the introgression of the genomic regions containing maize ZCN8(Le Corre et al., 2020).
Soybean (Glycine max (L.) Merr.)
Soybean (G.e max (L.) Merr.) is a short-day plant and was domesticated in China, within latitudes 32˚N–40˚N (Li et al., 2008). By selecting photoperiodic insensitive mutations that flower under long-day conditions, soybeans were adapted to higher latitudes. Soybean cultivars grown at high latitudes either have mutations in e1, e2, e3, e4, or multiple mutations thereof (Tsubokura et al., 2014). E1 encodes a protein that has a B3 DNA binding domain, a protein sequence found in plant-specific transcription factors (Xia et al., 2012). E2, E3, and E4 encode GI orthologue, photoreceptor PhyA3, and PhyA2, respectively (Liu et al., 2008; Watanabe et al., 2009, 2011). Of the e1 alleles, e1fr and e1nl showed very early flowering under long-day conditions and are found in the Swedish cultivar “Fiskeby V,” Japanese cultivars “Sakamotowase” and “Toyosuzu,” and northern Chinese cultivars “Hejian1” and “Heihe28” (Xia et al., 2012).
A recent study found that early flowering was a key trait for domesticating soybean from the ancestral wild species G. soja (Sieb. and Zucc.) (Lu et al., 2020). Genome-wide association study (GWAS) and QTL analyses of wild, landrace, and cultivated plants revealed that stepwise mutations in two PRR genes belonging to the PRR7/PRR3 sub-group (Tof11 and Tof12) occurred during the domestication process (Lu et al., 2020). The soybean PRR represses CCA1/LHY gene expression, showing that PRRs act as the core clock transcription repressors. Tof11 and Tof12 control flowering time via modulation of CCA1/LHY, E1, and FT expression in the leaf.
Soybeans were introduced to South America in the early 20th century; however, due to the short daylength throughout the year in these regions, soybeans flowered too early and yields were low. In the 1970s, the long-juvenile (LJ) trait that delays flowering and increases yield was found in Peruvian landrace PI 159925 (Lu et al., 2017). Incorporating the LJ trait increased soybean yield substantially in South America. A major locus of LJ (J) is an Arabidopsis ELF3 ortholog, and late flowering J mutants show de-repression of E1 expression (Lu et al., 2017). J acts as a clock component that is the basis for daylength measurement (Lu et al., 2017). A recent study found that a mutation in Tof16, an ortholog of LHY, results in late flowering and improves yield under short-day conditions (Dong et al., 2021). More than 80% of the accessions growing in low latitudes, such as Brazil and India, have tof16 and j mutations, suggesting a highly selected history for the late-flowering alleles in these regions (Dong et al., 2021).
Pea (Pisum sativum L.)
Pea is a long-day plant that originated in the Fertile Crescent of the Middle East. By selecting varieties with different photoperiodic responses, peas have spread to divergent latitudes and climates. HR (high response), SN (sterile nodes), LF (late flowering), and E (early initiating) have long been known as causal loci that change pea flowering time (Weller and Ortega, 2015). The hr mutations that allow the plants to flower earlier, even in short days, enabling the plants to grow at higher latitudes where growing seasons are limited (Weller et al., 2012). The early-flowering sn mutations are common in varieties used in Alaska (Liew et al., 2014). HR, SN, and LF are Arabidopsis ELF3-, LUX-, and TFL1-orthologs, respectively (Foucher et al., 2003; Weller et al., 2012; Liew et al., 2014). Artificially induced mutations revealed the molecular controls for pea flowering (Weller and Ortega, 2015). Early-flowering loci DIE NEUTRALIS (DNE) and PPD, and late-flowering loci LATE BLOOMER 1 (LATE1), LATE2, LATE3, LATE4, and GIGAS were identified. DNE and PPD encode elf4 and elf3b orthologs, respectively (Liew et al., 2009; Rubenach et al., 2017). The function of HR (ELF3a), PPD (ELF3b), SN (LUX), and DNE (ELF4) for flowering time in pea implies that these proteins act in a manner similar to the Arabidopsis EC, consisting of ELF3, ELF4, and LUX, that negatively regulates FT expression (Nusinow et al., 2011; Fukazawa et al., 2021). LATE1, LATE2, LATE3, LATE4, and GIGAS encode GI-, CDF-, CYCLIN DEPENDENT KINASE8 (CDK8)-, CYCLIN C1 (CYCC1)-, and FT-orthologs, respectively (Hecht et al., 2007, 2011; Ridge et al., 2016; Hasan et al., 2020). The late2 is a dominant mutation of PsCDFc1, by which the PsCDFc1 protein is not recognized by PsFKF1, a possible ubiquitin E3 ligase (Ridge et al., 2016). In Arabidopsis, clock transcription factors CCA1 and LHY control the transcription of FKF1, and FKF1 degrades CDF proteins in blue light (Imaizumi et al., 2005). Transient expression reporter assays indicate that PsCDFc1 can suppress FT promoter activity (Ridge et al., 2016). PsCDK8 and PsCYCC1 genetically and physically interact, strongly suggesting that PsCYCC1 is a possible cyclin partner of PsCDK8. Mutations of either PsCDK8 or PsCYCC1 reduce FT gene expression (Hasan et al., 2020).
Common bean (Phaseolus vulgaris L.)
The wild ancestor of the common bean was indeterminate and had short-day induced photoperiodic flowering. Mesoamerica is considered as the region of origin for wild beans. Wild beans then spread to the Andean region of South America about 100,000 years ago, forming two different wild gene pools (Schmutz et al., 2014; Rendon-Anaya et al., 2017). About 8,000 years ago, common bean was domesticated from two separate wild populations in Mexico and the Andean region. To spread common beans to high latitude areas, varieties that flower in long days with determinate growth habits were selected (Kwak et al., 2008; Weller et al., 2019). Loss of PvTFL1y, an ortholog of Arabidopsis TFL1, results in a determinate growth habit (Koinange et al., 1996; Repinski et al., 2012). The PPD insensitive early flowering ppd alleles were selected independently in Mexico and the Andean region. The causal gene for ppd is phyA3 (Weller et al., 2019). Loss-of-function of phyA3 does not result in changing photomorphogenic responses and circadian rhythms, which are regulated by phytochrome red-light signaling in Arabidopsis (Weller et al., 2019). This finding suggests that the common bean phyA3 has a specific role in flowering control other than the physiological events associated with red-light signaling. The mutations in CONSTANS LIKE 2 (COL2) result in early flowering, and contributed to common bean’s adaptation to long days (Gonzalez et al., 2021). Expression of FT was elevated in early flowering col2 mutants under long-day conditions, suggesting that COL2 represses FT expression (Gonzalez et al., 2021).
Tomato (Solanum lycopersicum L.)
Tomato is generally known to be a day-natural plant that flowers regardless of PPD; however, ancestral wild plants of tomato are short-day plants (Soyk et al., 2017). Tomato was domesticated in the Andean region of South America near the equator and introduced into Europe. The loss of photoperiod sensitivity was indispensable for tomato cultivation in Europe and was achieved by selecting the allele attenuating long-day induced expression of flowering repressor SELF-PRUNING 5G (SP5G; Soyk et al., 2017). SP5G is structurally similar to FT, but its activity for flowering is the opposite. SP5G suppresses tomato FT (SFT) expression. Compared to their wild ancestors, it was indicated that major tomato cultivars have a lengthened circadian period (Muller et al., 2016). The slowdown of the pace is associated with higher growth performance under long-day conditions. Mutations in two genes are known to cause period slowing in tomato cultivars: one is an ortholog of Arabidopsis EID1 encoding an E3 ubiquitin ligase (Dieterle et al., 2001; Muller et al., 2016), and the other is an LNK2 ortholog (Rugnone et al., 2013; Muller et al., 2018) Both genes are involved in phytochrome signaling.
Potato (Solanum tuberosum L.)
Potatoes were domesticated in the Andean region near the equator. Wild potatoes form tubers under short-day conditions but not long days. Modern potato cultivars develop tubers even under long days due to a loss of photopeiod sensitivity. A genetic study found that mutation of an Arabidopsis CDF1 ortholog (StCDF1) caused tuber formation under long days by controlling the expression of StSP6A that encodes a mobile signal to initiate tuber formation (Navarro et al., 2011; Kloosterman et al., 2013). Day length-insensitive potatoes have StCDF1 alleles that lack the C-terminal region bound by StFKF1, an E3 ligase for degradation of StCDF1.
Sugar beet (Beta vulgaris ssp. vulgaris)
Sugar beet is an important source of sugar production throughout the world. The ancestral plant of sugar beet, wild sea beet (B.vulgaris ssp. maritima), has an annual habit and induces flowering in long days, whereas sugar beets (ssp. vulgaris) in northern Europe have a biennial habit (Pin et al., 2012). BOLTING TIME CONTROL 1 (BvBTC1) is a major determinant of this annual–biennial regulation. BvBTC1 encodes a PRR in the PRR7/PRR3 clade (Pin et al., 2012). BvBTC1 controls the annual–biennial trait through BvFT expression but does not regulate the clock.
Conclusion and perspectives
Thanks to the great effort of modern plant genetics, genes controlling photoperiodic flowering in crops have been identified and characterized. There are both common (e.g. ELF3, LUX, PRR73, and PHY) and specific genes (Ghd7 in monocots and E1 in legumes) in the plant lineages. Given that direct target genes of Arabidopsis core clock transcription factors in flowering time regulation were revealed, it is possible to assume the target genes of Arabidopsis core clock orthologs; however, direct target genes of monocot specific Ghd7 and Ehd1 and legume specific E1 are not fully identified (see Outstanding Questions).
The knowledge about molecular mechanisms for flowering time regulation will assist us in creating new cultivars for use in a broader range of latitudes and climatic areas that will eventually contribute to human adaptation to global warming. Naturally occurring alleles resulted in weakened or complete loss of photoperiod sensitivity (e.g. flowering under short-day conditions or late flowering under long-day conditions of long-day plants). Increasing photoperiod sensitivity by generating an absolute photoperiodic reaction from a facultative one may be potentially valuable. Canonical breeding relied on allelic variation, but improved genome editing technology offers to fine-tune flowering time. Plants are enriched in genetic redundancy; however, dominant mutations such as misexpression of wheat Ppd-D1 and stable forms of potato StCDF1 and pea PsCDFc1 have overcome genetic redundancy (Figure 4). Dominant mutations are frequently found that disrupt negative regulatory events, such as transcriptional repression and protein degradation. Mutations in negative regulatory processes by genome editing are promising targets for overcoming genetic redundancy (see Outstanding Questions and Figure 4). Current and further understanding of clock systems controlling flowering time will provide a compelling rationale for such genome editing.

Alleles that alter the flowering time beyond genetic redundancy. A, Modern potato cultivars carry StCDF1.2 or StCDF1.3 alleles in which StCDF1 proteins are less recognized by an ubiquitin E3 ligase StFKF1. Similarly, there are pea mutants in which PsCDF1c is less bound by PsFKF1, thereby altering flowering time. B, Wheat Ppd-D1a allele carries 2.0-kbp deletion upstream Ppd-D1 gene. The deleted region probably contains the cis-regulatory motif implicated in repression of Ppd-D1 transcription. Ppd-D1a and StCDF1.2, StCDF1.3 are dominant alleles compared to the original alleles. C, Possible targets for modulating flowering time by genome editing. Mutations impairing or weakening interactions between the transcription repressor and its binding DNA element result in upregulation of the target gene and modulate flowering time, even if the target gene is genetically redundant. EC consists of ELF3, ELF4, and LUX. E1 is a specific regulator in legumes. Ghd7 and Ehd1 are photoperiodic flowering regulators only found in short-day monocots.
Decreasing the photoperiodic response enables crops to adapt to different latitudes and climates.
Genetic studies such as QTL and GWAS have revealed genes involved in photoperiodic flowering in major crops.
Genetic loci implicated in changing flowering time through florigen expression and activity have been commonly used for adaptation, e.g. phytochrome red light signaling, core circadian clock genes (ELF3, ELF4, LUX, and PRR37), a legume-specific E1, and a short-day monocot-specific Ghd7.
Clock gene activities for flowering are opposite in long-day plants and short-day plants.
How do Arabidopsis molecular models obtained from laboratory studies account for flowering time regulation in the field?
What are the genome-wide direct target genes for Ghd7 and Ehd1, monocot key transcription factors, and E1, a legume-specific transcription factor?
Can we synthesize attractive alleles to fine-tune flowering time?
Is it possible to pinpoint mutations that change clock function substantially by overcoming genetic redundancy?
N.N. conceived the manuscript. A.E M. and N.N. wrote the manuscript. A.E M. drew the figures.
The author responsible for distribution of materials integral to the findings presented in this article in accordance with the policy described in the Instructions for Authors (https://dbpia.nl.go.kr/plphys/pages/general-instructions) is Norihito Nakamichi ([email protected]).
Acknowledgments
We thank Shihori Oda (Ministry of Agriculture, Forestry, and Fishers, Aichi Prefecture) for sharing the photo used in Figure 1.
Funding
This work was supported by Grant-in-Aid for Scientific Research on Innovative Areas 20H05411 and 21H05656 to NN; Japan Society for the Promotion of Science 18H02136 and 20K21272; Takeda Science Foundation to NN. AEM is supported by Graduate Program of Transformative Chem-Bio Research.
Conflict of interest statement. None declared.
References
Author notes
Senior author