-
PDF
- Split View
-
Views
-
Cite
Cite
Ying Wang, Brenda C. Salasini, Madiha Khan, Bhaswati Devi, Michael Bush, Rajagopal Subramaniam, Shelley R. Hepworth, Clade I TGACG-Motif Binding Basic Leucine Zipper Transcription Factors Mediate BLADE-ON-PETIOLE-Dependent Regulation of Development, Plant Physiology, Volume 180, Issue 2, June 2019, Pages 937–951, https://doi.org/10.1104/pp.18.00805
- Share Icon Share
Abstract
Lateral organs formed by the shoot apical meristem (SAM) are separated from surrounding stem cells by regions of low growth called boundaries. Arabidopsis (Arabidopsis thaliana) BLADE-ON-PETIOLE1 (BOP1) and BOP2 represent a class of genes important for boundary patterning in land plants. Members of this family lack a DNA-binding domain and interact with TGACG-motif binding (TGA) basic Leu zipper (bZIP) transcription factors for recruitment to DNA. Here, we show that clade I bZIP transcription factors TGA1 and TGA4, previously associated with plant defense, are essential cofactors in BOP-dependent regulation of development. TGA1 and TGA4 are expressed at organ boundaries and function in the same genetic pathways as BOP1 and BOP2 required for SAM maintenance, flowering, and inflorescence architecture. Further, we show that clade I TGAs interact constitutively with BOP1 and BOP2, contributing to activation of ARABIDOPSIS THALIANA HOMEOBOX GENE1, which is needed for boundary establishment. These studies expand the functional repertoire of clade I TGA factors in development and defense.
The shoot apical meristem (SAM) provides all of the aerial structures in a plant. This primary meristem is a constant source of cells for the production of leaves, stems, branches, and flowers throughout the life cycle (Aichinger et al., 2012). Lateral organs formed by the SAM are separated from the stem cell domain by low-growth regions called boundaries. Impairment of boundaries leads to SAM termination and/or fusion of organs (Aida and Tasaka, 2006a, 2006b). As organs enlarge, boundaries define the base of shoots and control specific aspects of plant architecture. Boundaries provide axillary meristems essential for flower formation and branching. Boundaries are also sites for the regulated detachment of organs from the plant body, which is essential for abscission and seed dispersal (Hepworth and Pautot, 2015).
BLADE-ON-PETIOLE (BOP) genes, first described in Arabidopsis (Arabidopsis thaliana), are regulators of boundary patterning in land plants (Khan et al., 2014). BOP1 and BOP2 encode closely related transcriptional coactivators characterized by an N-terminal Broad-Complex, Tramtrack, and Bric-a-brac/POX virus and zinc finger (BTB/POZ) domain located upstream of an ankyrin repeat domain (Ha et al., 2004; Hepworth et al., 2005; Norberg et al., 2005). The BTB/POZ domain interacts with CULLIN3-RING E3 ubiquitin ligase to target transcription factors for degradation (Fu et al., 2012; Zhang et al., 2017; Chahtane et al., 2018). The ankyrin repeats interact with TGACG-motif binding (TGA) basic Leu zipper (bZIP) transcription factors for recruitment to DNA (Zhang et al., 1999; Després et al., 2000; Zhou et al., 2000; Hepworth et al., 2005; Xu et al., 2010). In Arabidopsis, BOP1 and BOP2 are part of a larger family of six BTB-ankyrin proteins. This family contains two phylogenetic subclades: a primary subclade comprised of NONEXPRESSOR OF PATHOGENESIS-RELATED GENES1 (NPR1) and three close homologs (NPR2, NPR3, and NPR4), with roles in plant defense; and a secondary subclade comprised of BOP1 and BOP2, with roles in plant development (Khan et al., 2014). NPR1 is essential for a broad-spectrum defense response called systemic acquired resistance (SAR) mediated by salicylic acid (SA). NPR3 and NPR4 also play a role in this response (Cao et al., 1997; Fu et al., 2012; Wu et al., 2012b; Yan and Dong, 2014; Ding et al., 2018). By contrast, BOP1 and BOP2 are global regulators of plant architecture. In aerial parts of the plant, they act at lateral organ boundaries to regulate abscission and the morphogenesis of leaves, inflorescences, fruits, and flowers (Khan et al., 2014; Hepworth and Pautot, 2015). BOP1 and BOP2 are also expressed in primary and secondary vasculature tissues of roots and promote symbiotic nodule organ identity in legumes (Couzigou et al., 2012; Liebsch et al., 2014; Woerlen et al., 2017). Despite these functional differences, NPR1, BOP1 and BOP2 share a highly conserved domain structure, suggesting a similar mode of action (Khan et al., 2014).
In particular, BTB-ankyrin proteins lack a DNA-binding domain and interact with transcription factors for recruitment to DNA. Yeast two-hybrid screens have been used to identify TGA bZIP transcription factors important for NPR1 function (Zhang et al., 1999; Després et al., 2000, 2003; Zhou et al., 2000; Boyle et al., 2009). TGA transcription factors in Arabidopsis are divided into five subclades (clade I: TGA1 and TGA4; clade II: TGA2, TGA5, and TGA6; clade III: TGA3 and TGA7; clade IV: TGA9 and TGA10; and clade V: TGA8, also known as PERIANTHIA/PAN; Gatz, 2013). Genetic studies show highly overlapping roles for TGA factors in plant defense, stress responses, and/or development (Gatz, 2013; Noshi et al., 2016). NPR1 constitutive interaction with clade II TGAs plays a major role since SAR is abolished in tga2 tga5 tga6 triple mutants, resulting in an npr1-like phenotype (Cao et al., 1994; Zhang et al., 2003). NPR1 also interacts with clade III TGA3 (Choi et al., 2010) and in a redox-dependent manner with clade I TGA1 and TGA4 to impart its function (Després et al., 2003; Sun et al., 2018).
In previous work, we showed that BOP1 and BOP2 interact with clade V TGA8/PERIANTHIA (PAN). Loss-of-function bop1 bop2 and pan mutants have a similar pentamerous arrangement of first-whorl floral organs and extra petals, showing that BOP-PAN interactions regulate floral patterning (Hepworth et al., 2005; Xu et al., 2010). However, inactivation of TGA8/PAN captures only a subset of bop1 bop2 loss-of-function phenotypes, suggesting the involvement of other TGA family members (Running and Meyerowitz, 1996; Ha et al., 2004; Hepworth et al., 2005; Norberg et al., 2005; McKim et al., 2008; Xu et al., 2010).
Here, we identify a role for clade I TGAs in BOP1 and BOP2-dependent regulation of plant architecture. These TGA factors are coexpressed at organ boundaries and function in the same genetic pathways as BOP1 and BOP2 that are important for meristem integrity, flowering, and inflorescence architecture. Distinct from NPR1, TGA1 and TGA4 interact constitutively with BOP1 and BOP2 in yeast (Saccharomyces cerevisiae) cells. Further, clade I TGAs and BOP1 colocalize to A-box-containing sites in the promoter of boundary gene ARABIDOPSIS THALIANA HOMEOBOX GENE1 (ATH1), contributing to its activation. These results highlight a dual role for clade I TGAs in development and defense through differential interaction with BTB-ankyrin proteins.
RESULTS
Transcript Profiling Identifies Clade I TGAs Upregulated by BOP1
TGA bZIP transcription factors are required for the nuclear functions of BOP1 and BOP2 (Khan et al., 2014). The TGA family contains 10 members (Gatz, 2013). To identity potential partners, we used the transcriptome of BOP1-overexpressing plants to determine coregulated TGA bZIP genes (Khan et al., 2015). Within our microarray data set, TGA4 transcripts were upregulated 3.1-fold relative to transcripts in wildtype (Supplemental Table S1). These data were confirmed by reverse transcription quantitative PCR (RT-qPCR) experiments showing that both clade I TGA1 and TGA4 transcripts were significantly increased in the stem of bop1-6D transgenic plants that overexpress BOP1 (Fig. 1A). Despite this increase, these genes are not direct targets of BOP1 (Kahn, 2013). Loss-of-function bop1 bop2 mutations disrupt organ boundary patterning, resulting in loss of abscission, leafy petioles, fused organs, and characteristic defects in floral patterning (Khan et al., 2014). Consistent with previous reports, no such boundary-related defects have been observed in tga1, tga4, or tga1 tga4 mutants (Kesarwani et al., 2007; Shearer et al., 2012; Alvarez et al., 2014). The only obvious similarity to bop1 bop2 mutants is the “pinwheel” curvature of the leaves in tga1 and tga1 tga4 double mutants (Fig. 1, B–G; see also Shearer et al., 2012).
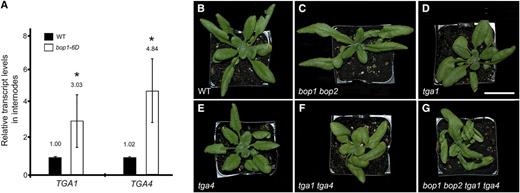
Clade I TGA transcript analysisand phenotype of tga1 tga4 double mutants. A, TGA1 and TGA4 transcript levels were measured in the internodes of wild-type (WT) and transgenic plants overexpressing BOP1 (bop1-6D) using RT-qPCR. Data are means ± sd of three biological replicates. Asterisks indicate asignificant difference compared to the wild type (Student's t test, *P < 0.01). B to G, Inactivation of TGA1 causes petiole curvature. Plants were grown under long-day conditions. Photos show a wild-type plant (B), bop1 bop2 (C), and tga1 (D) mutants, with helical growth of leaves, tga4 mutant (E), with leaves similar to wild type, tga1 tga4 double mutant (F), and bop1 bop2 tga1 tga4 quadruple mutant (G), with strong helical growth of leaves. Scale bar = 1 cm.
TGA1/TGA4 Are Required by BOP1 and BOP2 to Exert Changes in Inflorescence Architecture
To reveal a potential role for clade I TGAs, we studied the impact of tga1 and tga4 loss-of-function mutations on the phenotype of 35S:BOP2 transgenic plants. Constitutive overexpression of BOP1 or BOP2 inhibits stem elongation, resulting in short plants (Norberg et al., 2005; Khan et al., 2012b, 2015). Previously, we showed that this dwarf phenotype is reversed by inactivating boundary genes such as ATH1 or KNOTTED1-LIKE FROM ARABIDOPSIS THALIANA6 (KNAT6), which function genetically downstream of BOP1 and BOP2 (Norberg et al., 2005; Khan et al., 2012a, 2012b). To test if clade I TGAs are part of this module, 35S:BOP2 plants were crossed with wild-type control plants, tga1 or tga4 single mutants, and tga1 tga4 double mutants. The F1 progeny of 35S:BOP2 plants crossed to wild-type and tga1 or tga4 single-mutant plants were dwarf. By contrast, the F1 progenies of 35S:BOP2 plants crossed to tga1 tga4 double-mutant plants showed recovery of stem elongation. Approximately 82% of plants in this population (155/190) were tall (≥12 cm high; Fig. 2). These data suggest that TGA1 and TGA4 are required for BOP1- and BOP2-dependent changes in inflorescence architecture.
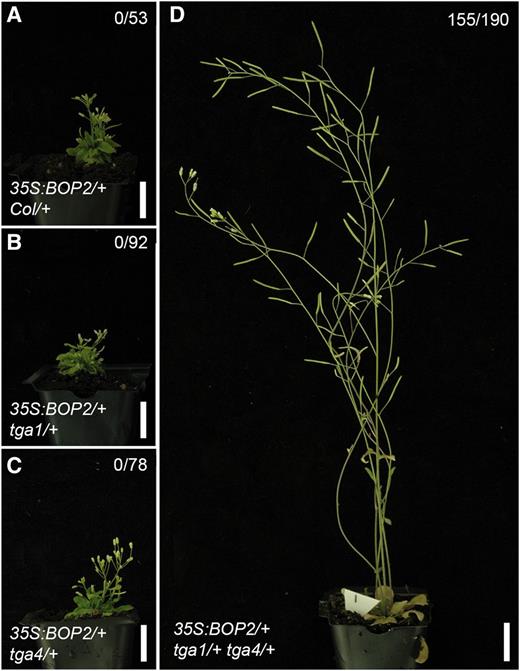
TGA1 and TGA4 are required for BOP-dependent regulation of inflorescence architecture. Plants homozygous for a 35S:BOP2 transgene were crossed to wild-type Col plants or homozygous tga1, tga4, or tga1 tga4 mutants. Representative F1 progenies are shown. The number of plants with restored stem elongation (>12 cm tall) in the population is given at the top right of images. A, 35S:BOP2/+ Col/+ control plants are dwarf. B, 35S:BOP2/+ tga1/+ plants are similar to the control plant. C, 35S:BOP2/+ tga4/+ plants are similar to the control plant. D, 35S:BOP2/+ tga1/+ tga4/+ plants show restored stem elongation. Scale bars = 2 cm.
Clade I TGAs Function in the Same Genetic Pathways as BOP1 and BOP2
SAM integrity relies on a precise balance between maintenance of the stem cell population and organogenesis (Aichinger et al., 2012). Two closely related BELL homeodomain proteins, PENNYWISE (PNY) and POUND-FOOLISH (PNF), required for meristem integrity, enable SAM maintenance and flowering by spatially regulating boundary genes, such as BOP1 and BOP2, in the shoot apex and stem (Smith et al., 2004; Khan et al., 2012b, 2015; Bencivenga et al., 2016). In pny mutants, apical dominance is partially lost and irregular stem elongation results in inflorescences with clusters of flowers/siliques instead of even spacing (Byrne et al., 2003; Smith and Hake, 2003). In pny pnf double mutants, the SAM is narrow and terminates prematurely in a small majority of seedlings (Ung et al., 2011; Ung and Smith, 2011). Further, these mutants are incapable of elongating a stem and fail to produce flowers (Smith et al., 2004). Inactivation of BOP1 andBOP2 or genes acting downstream of BOP1 and BOP2 complements pny and pny pnf mutant phenotypes (Khan et al., 2012a, 2012b, 2015). This complementation occurs because repression of boundary genes by PNY and PNF preserves organized division of cells in the SAM required for meristem function, including the production of stem and flowers (Ung et al., 2011; Andrés et al., 2015; Bencivenga et al., 2016).
To examine if clade I TGAs function in the same genetic pathway, we crossed pny and pny pnf mutants with tga1, tga4, and tga1 tga4 mutants and analyzed the phenotypes of the resulting higher-order mutants. SAM termination defects are a characteristic of pny pnf mutations. Figure 3 shows that ∼48.2% of pny pnf mutants stopped making leaves, indicating failed meristem activity. No such arrest was observed in tga1 pny pnf, tga4 pny pnf, and tga1 tga4 pny pnf mutants, which indicates that inactivation of clade I TGAs restores meristem function. We also tested if pny and pny pnf defects in flowering and inflorescence architecture could be rescued. Figure 4 shows that pny silique clustering defects were partially rescued by tga1 and completely rescued by tga1 tga4 mutations. Further, flowering in pny pnf was restored by tga1, tga4, and tga1 tga4 mutations. Quantitative phenotypic analyses showed that parameters of inflorescence architecture and flowering time in rescued genotypes were similar to those in the wild type (Supplemental Fig. S1). These collective data suggest that clade I TGAs function as boundary genes acting in the same genetic pathway as BOP1 and BOP2 to control meristem function and inflorescence architecture.
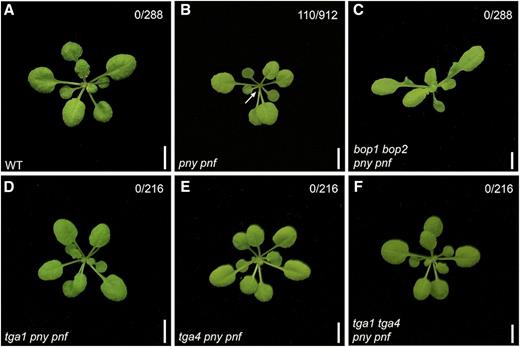
Inactivation of BOP1, BOP2, TGA1, and TGA4 corrects pny pnf meristem arrest. Shown are 25-d-old plants grown under short-day conditions. The number of plants showing a meristem arrest is indicated at the top right of each image. A, A wild-type plant. B, A pny pnf mutant with an arrested SAM (the arrow indicates no new leaf growth). C to E, No meristem arrest is observed in bop1 bop2 pny pnf (C), tga1 pny pnf (D), tga4 pny pnf (E), and tga1 tga4 pny pnf (F) mutants. Scale bars = 5 mm.
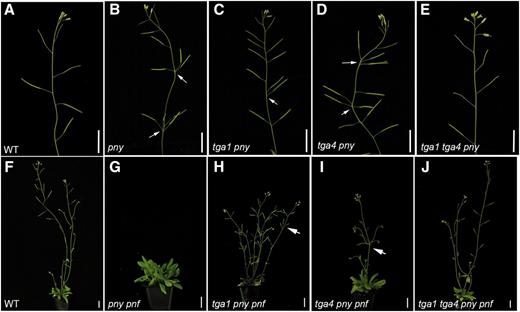
Inactivation of TGA1 and/or TGA4 corrects pny and pny pnf defects in inflorescence architecture and flowering. Shown are 6-week-old plants grown under continuous light. A, A wild-type inflorescence apex. B, A pny mutant with clustered siliques (arrows). C, A tga1 pny mutant showing partial rescue of clustering (arrow). D, A tga4 pny mutant showing little or no rescue of clustering (arrows). E, A tga1 tga4 pny mutant showing no clusters, similar to wild typeF, A wild-type flowering plant. G, A nonflowering pny pnf mutant. H, A tga1 pny pnf mutant showing restored flowering and partial rescue of clustered siliques (arrow). I, A tga4 pny pnf mutant showing restored flowering and little or no rescue of clustered siliques (arrow). J, A tga1 tga4 pny pnf mutant showing restored flowering and no clusters, similar to wild type. Scale bars = 1.5 cm.
TGA1 and TGA4 Expression Is Enriched at Organ Boundaries
BOP1 and BOP2 expression is absent from the SAM and enriched at organ boundaries throughout the life cycle (Ha et al., 2004; Norberg et al., 2005; Xu et al., 2010; Khan et al., 2012b, 2015; Andrés et al., 2015). To examine if TGA1 and TGA4 are also expressed in organ boundaries, we used promoter fusions to GUS (Jefferson et al., 1987). Analysis of stained tissue showed that TGA1 and TGA4 were expressed in roots, leaves, stems, and flowers (Fig. 5). This distribution matches in silico gene expression profiling data from AtGenExpress (Schmid et al., 2005; Waese et al., 2017). Within aerial tissues, TGA1 and TGA4 expression was enriched in the vasculature of leaves and stems. Cross sections of the primary stem showed that TGA4 was expressed in phloem and both genes were expressed in xylem, whereas BOP1 and BOP2 expression was largely absent from internodes (Fig. 5). Comparing to BOP1 and BOP2, strong overlap with TGA1 and TGA4 expression was observed at the base of young leaves and in vasculature at the base of mature rosette leaves. Strong overlap in expression was also observed at shoot and organ boundaries in the inflorescence, including nodes where floral pedicels join to the stem, the base of young floral organs where abscission zones form, and the base of mature fruits where a protective layer is deposited postabscission (Fig. 5). These data indicate a boundary-enriched expression pattern for clade I TGAs that partially overlaps with BOP1 and BOP2. Similar to other boundary genes, no TGA1 or TGA4 expression was detected in the SAM (Supplemental Fig. S2).
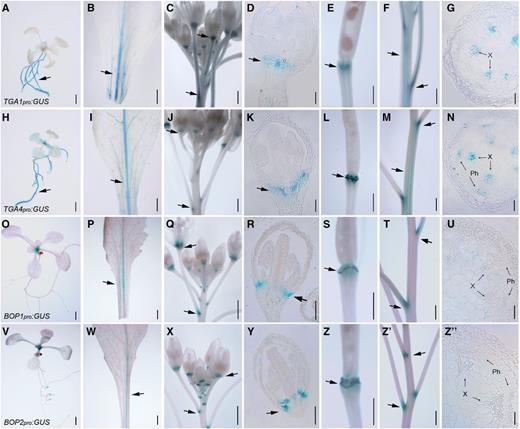
TGA1 and TGA4 expression is enriched at boundaries in the inflorescence in a pattern that overlaps with BOP1 and BOP2. Arrows denote enriched areas of expression. A to G, Shown are TGA1pro:GUS expression patterns in a 10-d-old seedling (A),rosette leaf petiole (B), inflorescence apex (C), longitudinal section of a young flower (D), abscission zone of a mature fruit (E), inflorescence stem (F), and stem cross section (G). H to N, Shown are TGA4pro:GUS expression patterns in a 10-d-old seedling (H), rosette leaf petiole (I), inflorescence apex (J), longitudinal section of a young flower (K), abscission zone of a mature fruit (L), inflorescence stem (M), and stem cross section (N). O to U, Shown are BOP1pro:GUS expression patterns in a 10-d-old seedling (O), rosette leafpetiole (P), inflorescence apex (Q), longitudinal section of a young flower (R), abscission zone of a mature fruit (S), inflorescence stem (T), and stem cross section (U). V to Z", Shown areBOP2pro:GUS expression patterns in a 10-d-old seedling (V), rosette leaf and petiole (W), inflorescence apex (X), longitudinal section of a young flower (Y), abscission zone of a mature fruit (Z), inflorescence stem (Z′), and stem cross section (Z″). X, Xylem; Ph, phloem. Scale bars = 1 mm (A–C, E, F, H– J, L, M, O–Q, S, T, V–X, Z, and Z′) and 50 µm (D, G, K, N, R, U, Y, and Z″).
PNY and PNF Repress Clade I TGA Transcription Factor Gene Expression
PNY, and presumably PNF, directly repress boundary genes in the SAM and stem to preserve the organized division of cells required for pattern formation (Andrés et al., 2015; Bencivenga et al., 2016). Boundary genes including BOP1, BOP2, ATH1, KNAT2, and KNAT6 have an expanded domain of expression in the stem and apices of pny and pny pnf mutants (Ragni et al., 2008; Khan et al., 2012a, 2012b, 2015; Andrés et al., 2015). This prompted us to test if TGA1 and/or TGA4 expression domains are likewise expanded in pny and pny pnf mutants. In pny and pny pnf/+ mutants, both genes showed strongly upregulated expression in the stem, particularly around nodes with clustered flowers (Fig. 6). This pattern was similar to misexpression of BOP1 and BOP2 in pny stems (Khan et al., 2012b). We did not observe misexpression of either gene in the SAM or inflorescence meristem of pny pnf mutants, suggesting that control elements for expression might be missing (Supplemental Fig. S2). Overall, these data confirm that, similar to other boundary genes, PNY and PNF spatially control clade I TGA expression.
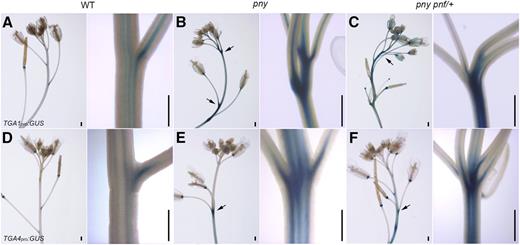
Expression pattern of TGA1 and TGA4 in wild-type and mutant inflorescences . A, TGA1pro:GUS in wild type shows expression at the base of pedicels, flowers, fruits, and stem vasculature (close-up). B and C, TGA1pro:GUS inpny (B) and pny pnf/+ (C) mutants shows strong up-regulation in the stem (arrow) concentrated at the nodes (close-ups). D, TGA4pro:GUS in wild type shows an expression pattern similar to TGA1pro:GUS (A). E and F, TGA4pro:GUS in pny (E) and pny pnf/+ (F) mutants shows strong up-regulation in the stem (arrows) concentrated at the nodes (close-ups). Scale bars = 0.5 mm.
Clade I bZIP TGAs Are Required for Activation of ATH1 Expression
BOP1 and BOP2 directly activate boundary genes to control leaf patterning and inflorescence architecture (Jun et al., 2010; Khan et al., 2015). Experiments using a steroid-inducible form of BOP1 fused to the hormone-binding domain of the rat (Rattus norvegicus) glucocorticoid receptor (BOP1-GR) have shown that BOP1 directly activates ATH1, a homeobox gene important for boundary patterning (Gómez-Mena and Sablowski, 2008; Khan et al., 2015). Analysis identified a 2.0-kb fragment of the ATH1 promoter fused to GUS that is responsive to BOP1 induction. This reporter gene is up-regulated in D35S:BOP1-GR plants after 4 h of dexamethasone (DEX) treatment. Stronger induction occurs after 24 h of sustained DEX treatment (Khan et al., 2015).
To test if ATH1 expression is likewise dependent on clade I TGAs, we first monitored ATH1 transcript levels in bop1 bop2 and tga1 tga4 double and bop1 bop2 tga1 tga4 quadruple mutants compared to wild-type control plants (Fig. 7, A and B). In seedlings and inflorescence apices, ATH1 transcripts were significantly reduced in bop1 bop2, tga1 tga4, and bop1 bop2 tga1 tga4 mutants compared to the wild type, which is consistent with these genes functioning in the same genetic pathway. To further test if BOP1 requires TGA1/TGA4 transcription factors to activate ATH1 expression, D35S:BOP1-GR transgenic plants were used to monitor the DEX induction of a 2.0-kb ATH1pro:GUS reporter gene in wild-type versus tga1 tga4 mutant plants (Fig. 7, C–G). Reporter gene expression was diminished in tga1 tga4 mutant plants compared to the wild type, and no significant induction was observed in most lines after long-term treatment with DEX. DEX-treated D35S:BOP1-GR tga1 tga4 plants were also significantly taller than D35S:BOP1-GR DEX-treated control plants, consistent with a requirement for ATH1 in BOP1-dependent restriction of stem elongation (Fig. 7H; Khan et al., 2012a). Collectively, these data suggest that clade I TGAs are positive regulators of ATH1 expression.
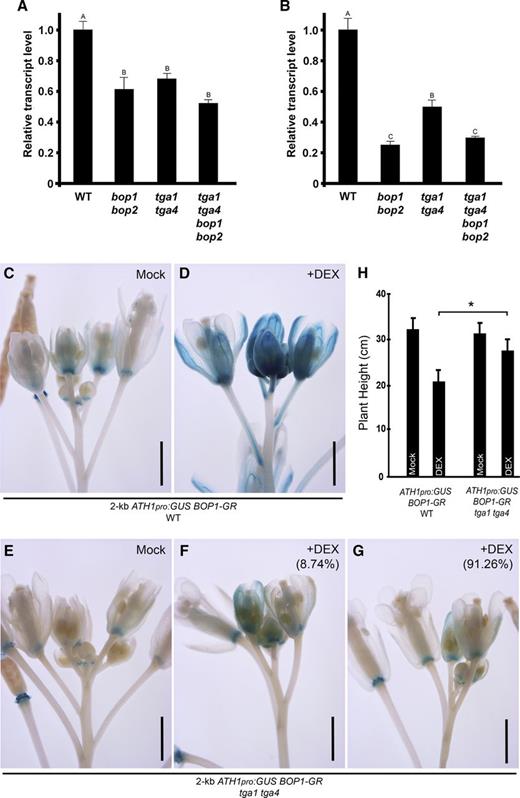
Clade I TGAs are required for BOP1 induction of ATH1 expression. A and B, ATH1 transcript levels were measured in wild type (WT) and mutants using RT-qPCR. Shown are data for 10-d-old seedlings (A) and inflorescence apices (B). Data are the means ± se from three independent experiments, with the same letter indicating no significant difference at P ≤ 0.05 (ANOVA with Duncan's posthoc test). C to G, Induction of 2-kb ATH1pro:GUS by D35S:BOP1-GR was disrupted by inactivation of TGA1 and TGA4. C and D, 2-kb ATH1pro:GUS D35S:BOP1-GR in wild-type Col-0 background. Images are representative. C, In the inflorescence apex from a mock treated plant, ATH1 expression was mainly at the base of floral organs. D, In the inflorescence apex from a 10 µm DEX-treated plant, ATH1 expression was induced in flowers, pedicels, and pedicel axils. E to G, 2-kb ATH1pro:GUS D35S:BOP1-GR in a tga1 tga4 mutant. E, In the inflorescence apex from a mock treated plant, ATH1 expression pattern was similar to that in mock Col-0 plants (C). F and G, In the inflorescence apices from 10 µm DEX-treated plants, there was intermediate induction (F; 8.74% of apices) and weak induction (G; 91.26% of apices) compared to DEX-treated Col-0 plants (n = 87 for wild type; n = 183 for tga1 tga4). Scale bars = 1 mm. H, Measurement of plant height for DEX-induced plants (n = 18 for wild-type background plants; n > 36 for tga1 tga4 background plants). Data are means ± sd. Asterisk, significant difference between DEX-treated wild-type and tga1 tga4 background plants (Student’s t test, *P < 0.01).
Clade I TGA Factors Interact Constitutively with BOP1 and BOP2
TGA bZIP transcription factors selectively interact with BTB-ankyrin proteins, which can function as transcriptional activators when localized to DNA (Després et al., 2000; Hepworth et al., 2005; Xu et al., 2010; Wu et al., 2012a). BOP1 and BOP2 interactions with clade I TGA1 and TGA4 were previously tested in yeast, but the results were inconclusive (Hepworth et al., 2005). On the other hand, NPR1 selectively interacts with TGA1 in planta under reducing conditions triggered by exposure to SA (Després et al., 2003). This exposure is thought to alter the redox status of TGA1 Cys residues, creating a conformational change that brings about interaction with NPR1. mTGA1-C260N/C266S mutants (and equivalent mutants of TGA4) are thought to interact constitutively with NPR1, because formation of an inhibitory intramolecular disulphide bridge is abolished (Després et al., 2003).
The yeast two-hybrid assay is highly sensitive for detecting protein-protein interactions. Therefore, we used this method to reassess BOP interactions with clade I TGA factors using PAN as a positive control. BOP1 and BOP2 fused to the DNA-binding domain of the yeast transcription activator protein GAL4 were used as bait. Wild-type or Cys mutant forms of TGA1 and TGA4 fused to the activation domain of GAL4 were used as prey. Figure 8A shows that BOP1 and BOP2 preferentially interact with wild-type TGA1 and TGA4 in yeast. Interestingly, redox mimic mutations that promote NPR1 interaction with TGA1 and TGA4 reduce interactions with BOP1 and BOP2. As a further test, we used bimolecular fluorescence complementation (BiFC) to examine BOP1 and BOP2 interaction with TGA1 and TGA4 in planta. Figure 8B shows reconstitution of yellow fluorescent protein (YFP) fluorescence in nuclei of transformed Nicotiana benthamiana leaf cells, indicating that BOP1 and BOP2 form a complex with TGA1 and TGA4 in plant cells.
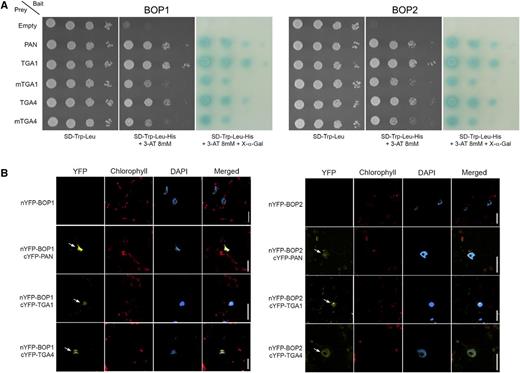
Biochemical evidence of BOP1 and BOP2 interaction with TGA1 and TGA4 is shown. A, Pairwise yeast two-hybrid assays showing BOP1 and BOP2 interaction with wild-type and Cys mutant forms of TGA1 and TGA4. BOP1 and BOP2 baits were fused to the DNA-binding domain of yeast GAL4. TGA preys were fused to the transcriptional activation domain of yeast GAL4. Plasmid constructs were cotransformed into yeast AH109 strain before serial dilutions (10−1, 10−2, 10−3, 10−4) were plated onto SD/−Trp/−Leu medium with or without His + 8 mm 3-AT. Growth on SD/−Trp/−Leu/−His/+3-AT above background confirms a protein-protein interaction. Dilutions were spotted in replicate onto SD/−Trp/−Leu/−His/+3-AT medium supplemented with 20 mg L−1 X-α-gal for assessment of α-galactosidase reporter activity. Photos on SD/−Trp/−Leu were taken 2 d after plating. Photos on SD/−Trp/−Leu/–His/+3-AT with or without X-α-gal were taken after 3 d. For all assays, interaction with PAN was used as a positive control and an empty prey vector was used as a negative control. B, BOP1 and BOP2 interaction with TGA1 and TGA4 in nuclei of transiently transformed N. benthamiana leaves. BiFC was used to examine BOP1 and BOP2 interactions with TGA1 and TGA4 in planta. The N terminus of YFP (nYFP) was fused in frame at the N terminus BOP1 or BOP2. The C terminus of YFP (cYFP) was fused in frame at the N terminus of PAN, TGA1, or TGA4. Pairs of constructs were transiently expressed in N. benthamiana leaves. Reconstitution of YFP fluorescence was examined by confocal microscopy 4 d after infiltration. Yellow fluorescence in the nucleus was detected for BOP1 and BOP2 paired with PAN, TGA1, and TGA4 (arrows). Red fluorescence, chlorophyll a autofluorescence. Blue fluorescence, DAPI-stained nuclei. Scale bars = 20 µm.
Clade I bZIP TGAs Directly Bind to the ATH1 Promoter
An interaction between BOP1 and BOP2 and clade I TGAs in nuclei suggests that these proteins might colocalize to the promoter of target genes to activate transcription. The ATH1 promoter contains abundant motifs that match or closely match consensus TGA binding sites (Khan et al., 2015). Direct association of BOP1 at two TGA-binding sites in the ATH1 promoter region was identified by chromatin immunoprecipitation (ChIP; Khan et al., 2015). Leaf material collected from BOP1pro:BOP1-GR bop1 bop2 flowering plants was probed using an anti-GR antibody. Eight sites with TGA motifs spanning a 2,178-base pair region upstream of the ATH1 transcription start site were tested. Site IV (nucleotides −2686 to −2577) with a 1.77-fold enrichment of BOP1 protein after DEX treatment was identified (Khan et al., 2015). Site VII (nucleotides −1529 to −1416) was identified as a second potential binding site at lower confidence. Both of these sites contain an A-box motif, which is a strong binding site for TGA1 (Izawa et al., 1993).
To test if TGA1 and TGA4 were enriched at these sites, we first generated tga1 tga4 transgenic plants harboring TGA1pro:TGA1 and TGA4pro:TGA4 translational fusions with GFP so we could use commercially available GFP antibody for the ChIP experiments. GFP fusions were expressed from the same native promoters that were used for GUS lines (Fig. 5). TGA1-GFP and TGA4-GFP fusion proteins localized in the nuclei of transgenic plants (Fig. 9A). The activity of fusion proteins was further monitored by complementation of tga1 tga4 sensitivity to tunicamycin-induced endoplasmic reticulum stress (Wang and Fobert, 2013). Two TGA1pro:TGA1-GFP tga1 tga4 and TGA4pro:TGA4-GFP tga1 tga4 lines showing complementation of this defect were used for ChIP assays (Fig. 9B). The ChIP assay showed significant enrichment of TGA1 at site IV and site VII in the ATH1 promoter, whereas TGA4 interacted preferentially with site IV (Fig. 9, D and E). These results are consistent with the model that BOP1 and BOP2 interact with clade I TGAs for recruitment to the promoter of target genes.
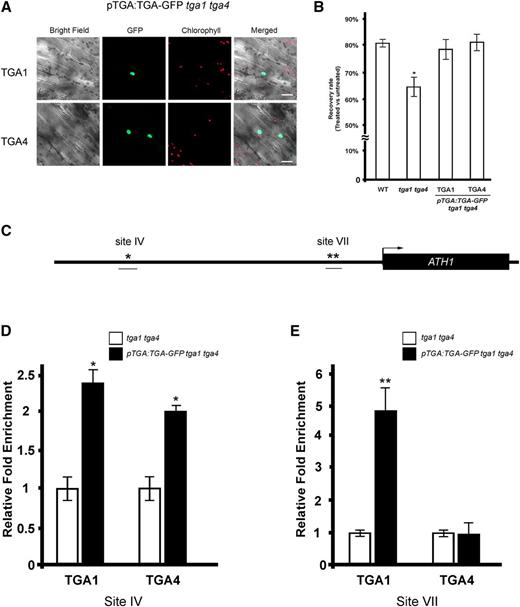
TGA1 and TGA4 bind to the same cis elements as BOP1 and BOP2 in the ATH1 promoter based on ChIP assays. A, Confocal microscope images of TGA1pro:TGA1-GFP tga1 tga4 and TGA4pro:TGA4-GFP tga1 tga4 seedlings show nuclear localization of TGA1-GFP and TGA4-GFP. B, TGA1pro:TGA1-GFP tga1 tga4 and TGA4pro:TGA4-GFP tga1 tga4 lines for ChIP are complemented for TM sensitivity. Five-day-old seedlings (n = 5 per treatment group, 5–6 replicates) were submerged in 0.5× liquid MS media with or without 0.8 µg mL−1 TM for 6 h. The fresh weight of TM- versus mock-treated seedlings after 5 d of recovery on TM-free media is shown. Data are means ± sd. Asterisk, significant difference compared to the wild type (Student’s t test, P < 0.05). C, Map of the ATH1 promoter and 5′ untranslated region. The black arrow marks the transcription start site. Predicted consensus A-box binding sites for TGA bZIP factors are marked with asterisks. ChIP-qPCR amplified fragments are underlined. D and E, ChIP-qPCR assay showing that TGA1 and TGA4 bind to the ATH1 promoter. Apices of 6-week-old plants were harvested for ChIP using anti GFP-antibody. qPCR was performed to calculate the abundance of specific regions of genomic DNA. Each ChIP-qPCR histogram indicates the mean ± se of three biological replicates, except in D, where only two biological replicates were quantified for TGA4. Enrichment values were normalized to input DNA. For each biological replicate, three technical qPCR reactions were performed. Asterisks, significant difference compared to control (Student’s t test *P < 0.05; **P < 0.01).
DISCUSSION
Arabidopsis BTB-ankyrin proteins BOP1 and BOP2 control plant architecture by means of their activity at organ boundaries. These proteins are part of a larger family of NPR1-like proteins with roles in plant immunity. Despite these different functions, all proteins in this family have a similar domain structure and rely on interaction with bZIP TGA transcription factors for recruitment to DNA and activation of target genes. We show here that clade I TGA transcription factors, previously associated with plant defense, function broadly in BOP-dependent regulation of plant development. This work reveals a role for clade I TGAs at boundaries and hints at possible additional roles for this module in plant defense.
Clade I TGA bZIPs Have Dual Functions in Development and Defense
Clade I TGA-like factors were originally identified biochemically in Nicotiana tabacum nuclear extracts, based on their ability to bind to an activating sequence 1 (as-1) element in the Cauliflower mosaic virus 35S promoter (Katagiri et al., 1989; Lam et al., 1989). The tandem TGACG motifs comprising this element drive expression of the 35S promoter in roots (Benfey and Chua, 1990) and show responsiveness to signals like SA, jasmonic acid, the auxin-related herbicide 2,4-dichlorophenoxyacetic acid, and hydrogen peroxide associated with biotic and abiotic stress (Liu and Lam, 1994; Qin et al., 1994; Xiang et al., 1996). Compatible with these findings, inactivation of TGA1 and TGA4 genes impairs innate immunity and, to a lesser extent, NPR1-dependent SAR (Després et al., 2003; Kesarwani et al., 2007; Wang and Fobert, 2013; Sun et al., 2018). Clade I TGAs are required for the full induction of SYSTEMIC ACQUIRED RESISTANCE1 (SARD1) and CALMODULIN-BINDING PROTEIN 60g (CBP60g) genes needed for biosynthesis of SA and pipecolic acid during both types of immune response (Sun et al., 2018). Clade I TGAs are also reported to have developmental roles. tga1 tga4 double mutants have altered root architecture under low nitrate conditions (Alvarez et al., 2014; Canales et al., 2017) and curved petioles (Shearer et al., 2012). Biochemical experiments show that TGA4 interacts with CONSTANS and binds to the FLOWERING LOCUS T promoter, suggesting formation of a complex that regulates flowering (Song et al., 2008).
Here, we characterize a role for clade I TGA1 and TGA4 transcription factors at organ boundaries. Both genes are strongly expressed in mature organ boundaries, including the base and petiole of leaves, axil of floral pedicels, and the base of floral organs. Despite this pattern, tga1 tga4 mutants have no obvious boundary-related defects, presumably due to redundancies. Transcripts for TGA3 and TGA9 are also upregulated in BOP1-overexpressing transgenic plants (Khan et al., 2015; Supplemental Table S1) and may contribute. This suggestion is supported by studies in maize (Zea mays) showing that LIGULELESS2 (LG2, most related to AtTGA9) contributes to establishment of the leaf blade-sheath boundary. Ligule and auricle structures formed at this junction are missing in lg2 mutants, causing leaf blades to be more upright as they bend away from the stem (Walsh et al., 1998; Tian et al., 2011). lg2 mutants are also delayed in flowering and have fewer, more upright, tassel branches (Walsh and Freeling, 1999).
To investigate a boundary function for clade I TGAs, we studied the impact of loss-of-function mutations on the phenotype of transgenic plants constitutively expressing BOP1 or BOP2. Constitutive expression of BOP1 and BOP2 inhibits stem elongation and flowering (Norberg et al., 2005; Khan et al., 2012b, 2015). Depletion of TGA1 and TGA4 activities in 35S:BOP2 plants restored internode elongation in the majority of F1 progeny, suggesting that these factors contribute to BOP2 activity. To further examine the role of TGA1 and TGA4 in boundaries, we investigated their interaction with PNY and PNF. Ectopic expression of boundary genes in the shoot apex of pny and pny pnf mutants impairs SAM maintenance, flowering, and stem elongation (Andrés et al., 2015; Khan et al., 2015). These defects are corrected by bop1 bop2 (Khan et al., 2012b, 2015) and were also suppressed by tga1, tga4, or tga1 tga4 mutations consistent with functions in the same genetic pathway. Similar onset of flowering in tga1 pny pnf and tga4 pny pnf compared to tga1 tga4 pny pnf mutants suggests that TGA1 and TGA4 have interchangeable functions in the shoot apex. This contrasts with the stem, where TGA1 inactivation had a greater impact on rescue of pny and pny pnf defects in silique clustering. Both TGA1 and TGA4 reporter genes showed an expanded pattern of expression in pny and pny pnf/+ stems, suggesting that they are under the same regulatory control as other boundary genes. PNY directly represses BOP1 and BOP2 in the shoot apex and stem to maintain organized patterns of cell division required for meristem activity, flowering, and stem elongation (Andrés et al., 2015; Bencivenga et al., 2016). Genome-wide analysis of PNY binding sites shows PNY interacting with the genomic DNA of numerous boundary genes, including ATH1 and KNAT6, which function in the same genetic pathway as BOP1 and BOP2. The same study identifies TGA1 and TGA4 as having high-confidence binding sites for PNY, suggesting direct repression (Bencivenga et al., 2016).
Clade I TGAs Are Required for BOP-Dependent Activation of a Boundary Gene
BOP1 and BOP2 fulfill their function in part by promoting the expression of boundary genes, both directly and indirectly (Jun et al., 2010; Khan et al., 2012a, 2015; Kahn, 2013). Our data provide evidence that clade I TGA factors are directly required for BOP-dependent activation of ATH1, encoding a BELL-type homeobox gene that functions in boundary patterning. Loss-of-function ath1 mutations cause ectopic elongation of the region below the shoot apex in seedlings, fusion of floral organs, and partial defects in floral organ abscission (Gómez-Mena and Sablowski, 2008). In young seedlings, ATH1 transcripts were reduced similarly by bop1 bop2 and tga1 tga4 mutations. In inflorescence apices, ATH1 transcripts were partially reduced in tga1 tga4 mutants compared to bop1 bop2, suggesting increased redundancy during the reproductive stage. A 2-kb region of the ATH1 promoter fused to GUS is responsive to BOP1 induction (Khan et al., 2015). In a tga1 tga4 mutant background, BOP1 induction of this GUS reporter was markedly reduced, also suggesting that clade I TGAs are ATH1 positive regulators. ChIP assays have demonstrated BOP1 binding to site VII within this 2-kb promoter and site IV, which is slightly upstream (Khan et al., 2015). These sites contain A-box motifs (TAC/GTA) whose two palindromic half sites have been shown biochemically to recruit TGA1 (Schindler et al., 1992; Izawa et al., 1993; Khan et al., 2015). Compatible with these data, we show that site IV is a binding site for TGA1 and TGA4, whereas site VII selectively binds TGA1. Nucleotides flanking the AC/GT core greatly influence the degree of binding (Schindler et al., 1992). These differences likely reflect unique binding requirements for TGA4, whose preference for flanking residues has not been determined biochemically. Collectively, these studies demonstrate a requirement for clade I TGAs in BOP1-dependent activation of ATH1. Genome-wide analysis of BOP1 and BOP2 binding sites is an important future direction in determining if this mechanism applies widely to the induction of genes important for boundary function.
Clade I TGAs Interact Differentially with BOP1 and BOP2 and NPR1
Clade I TGA recruitment of BOP1 and BOP2 to the promoter of ATH1 is additionally supported by a physical interaction between BOPs and TGA1 and TGA4 detectable in yeast cells. An interaction is also observed in nuclei of N. benthamiana leaves using BiFC assays. Preferential interaction of BOP1 and BOP2 with native TGA1 and TGA4 contrasts with NPR1, whose interaction with clade I TGAs is redox regulated. Interaction of TGA1 with NPR1 is stimulated in planta in the presence of SA, which changes the conformation of NPR1 and redox status of TGA1 and TGA4 (Després et al., 2003; Wu et al., 2012b). Mutations of specific Cys residues in TGA1 (C260/C266) and equivalent residues in TGA4 (C256/C262) bring about interaction with NPR1 in yeast (Després et al., 2003), suggesting that SA-induced reduction of an inhibitory disulphide bond promotes complex formation. This interaction also occurs in SA-treated leaves but is not critical for SAR (Després et al., 2003; Kesarwani et al., 2007). Clade I TGAs function primarily in innate immunity based on transcriptome studies and backed by phenotypic data (Kesarwani et al., 2007; Shearer et al., 2012; Wang and Fobert, 2013; Sun et al., 2018). Interestingly, redox mimic mutations that promote NPR1 interaction with TGA1 and TGA4 (Després et al., 2003) reduce the interaction with BOP1 and BOP2 in yeast cells. Thus, selective recruitment of BTB-ankyrin proteins by clade I TGAs might depend on redox status. CC-type glutaredoxins (named ROXYs in Arabidopsis) are candidates for redox control. ROXYs interact physically and genetically with TGA bZIP factors in regulating both development and defense (Ndamukong et al., 2007; Zander et al., 2012; Gutsche et al., 2015; Uhrig et al., 2017). ROXY1 interacts with TGA8/PAN, whose redox-sensitive DNA-binding activity determines petal number in flowers (Li et al., 2009; Gutsche and Zachgo, 2016). Similarly, ROXY1 and ROXY2 interact with TGA9 and TGA10, whose functions are redundantly required for anther and pollen development (Murmu et al., 2010). ROXY1 weakly interacts with TGA1 and TGA4 in yeast, but the biological significance is untested (Li et al., 2009). Redox modification by nitrosylation increases the DNA-binding activity of TGA1 to target sites in vitro (Lindermayr et al., 2010). Alternatively, ROXY19/GLUTAREDOXIN480 interacts with TGA2 and TOPLESS-type transcriptional corepressors in yeast, possibly functioning as a redox-regulated adaptor for establishing TGA-dependent repression of target genes (Uhrig et al., 2017). During SAR, activated NPR1 enters the nucleus and interacts with TGA2, thereby masking its repression domain and providing an activation domain for induction of defense genes (Boyle et al., 2009). Clade I TGA recruitment of BOP1 and BOP2 may serve as a similar switch for activation of target genes involved in development or defense. Elevated basal levels of pathogenesis-related (PR) defense genes, including PR1, PR2, and PR5, in tga1 tga4 mutants is consistent with at least a subset of genes being under negative regulation (Lindermayr et al., 2010; Shearer et al., 2012). Future experiments will investigate this model.
MATERIALS AND METHODS
Plant Materials and Growth Conditions
Seeds were germinated in vitro on minimal media (Haughn and Somerville, 1986). Seedlings were transferred to sterilized soil (Promix BX Black, Premier Horticulture) supplemented with a 0.1% (w/v) solution of 20-20-20 fertilizer. Plants were grown to maturity in growth chambers at 21°C with light intensity of 110–130 µmol m−2 s−1 under short days (8 h light/16 h dark), long days (16 h light/8 h dark), or continuous light (24 h light), as required. The wild type in this study was the Col-0 ecotype of Arabidopsis (Arabidopsis thaliana). 35S:BOP2 and bop1-6D overexpression lines were described previously (Norberg et al., 2005). Mutant lines were obtained from the Arabidopsis Biological Resource Center (https://abrc.osu.edu/). The bop1-3 (SALK_012994), bop2-1 (SALK_075879), tga1-1 (SALK_028212), tga4-1 (SALK_127923), pny-40126 (SALK_40126), and pnf-96116 (SALK_96116) alleles have been described previously (Smith and Hake, 2003; Smith et al., 2004; Hepworth et al., 2005; Kesarwani et al., 2007). All mutant combinations were generated by crossing and confirmed by genotyping (Smith and Hake, 2003; Smith et al., 2004; Hepworth et al., 2005; Devi, 2014). BOP1pro:GUS and BOP2pro:GUS reporter lines have been previously described (McKim et al., 2008; Xu et al., 2010). Primers used in this study are listed in Supplemental Table S2. Constructs were used to transform plants by floral dipping (Clough and Bent, 1998) using the Agrobacterium tumefaciens strain C58C1 GV3101 pMP90 (Koncz and Schell, 1986).
Construction of GUS Reporter Gene Lines and Staining
To create TGA1pro:GUS and TGA4pro:GUS reporter genes, the 5′ untranslated region up to and including the start codon for TGA1 (nucleotides −3969 to +3) and TGA4 (nucleotides −3950 to +3) were amplified from genomic DNA as template (bacterial artificial chromosome [BAC] M1QN23 for TGA1 and BAC T31P16 for TGA4) using iProof DNA polymerase (Bio-Rad). Primers incorporating BamHI and NcoI restriction sites at the 5′ ends were used to facilitate directional cloning. Products were cloned into pCR-BluntII-TOPO (Invitrogen) and sequenced. Inserts were released by digestion with BamHI and NcoI and ligated into the corresponding sites of pGCO:GUS to create translational fusions with the GUS gene uidA (Hepworth et al., 2002). A. tumefaciens was cotransformed with pSOUP and introduced into wild-type plants (Hellens et al., 2000). Glufosinate-ammonium resistant primary transformants were selected on soil. Homozygous lines were isolated for downstream experiments. Tissues were stained and/or embedded and sectioned for GUS activity as described (Woerlen et al., 2017). Low-resolution images were collected under bright field using a stereomicroscope (SteRIO Discover V20, Carl Zeiss). A compound microscope (Axio Imager M2, Carl Zeiss) was used for high-resolution images.
The construction of 2-kb ATH1pro:GUS D35S:BOP1-GR transgenic plants was previously described. This line contains a steroid-inducible form of BOP1 that upregulates expression of a 2-kb ATH1pro:GUS reporter gene in the presence of DEX (Khan et al., 2015). Transgenes were introduced by crossing into tga1 tga4 double mutant background. F1 and F2 progenies were grown on minimal media using 25 µg mL−1 hygromycin B and 10 µg mL−1 phosphinothricin for selection of D35S:BOP1-GR and 2-kb ATH1pro:GUS, respectively. Mutants homozygous for tga1 and tga4 were identified by genotyping in the F2 generation (Shearer et al., 2012).
For analysis of GUS activity, homozygous 2-kb ATH1pro:GUS D35S:BOP1-GR tga1 tga4 plants were germinated on minimal selection media containing 3 µm DEX under continuous light. After a week, seedlings were moved to soil and treated daily with a 10 µm DEX solution (prepared from a 30 mm stock solution in ethanol). A 0.12% (v/v) ethanol solution was used for mock treatment. The inflorescence apices from 30-d-old plants were harvested and stained for GUS activity.
Yeast Two-Hybrid Assay
Protein-protein interactions were assayed using a Matchmaker GAL4-based yeast two-hybrid system (Clontech) and Gateway-compatible pGBKT7-DEST (bait) and pGADT7-DEST (prey) plasmids modified from pGBKT7 and pGADT7-Res vectors, respectively (Lu et al., 2010). BOP1 and BOP2 proteins fused to the DNA-binding domain of yeast GAL4 were used as bait. TGA1, TGA4, and TGA8/PAN proteins fused to the transcriptional activation domain of yeast GAL4 were used as prey. To prepare Gateway entry vectors, the full-length coding sequences of BOP1, BOP2, PAN, TGA1, and TGA4 were cloned into pCR8/GW/TOPO-TA (Thermo Fisher Scientific). Template plasmids containing mutated forms of TGA1 (mTGA1-C260N/C266S) and TGA4 (mTGA4-C256S/C262S) were supplied by Charles Després (Després et al., 2003). The full-length coding sequences of mTGA1 and mTGA4 were cloned into pENTR/D-TOPO (Thermo Fisher Scientific). All entry vector inserts were sequenced to confirm authenticity. To make the final plasmids, recombination reactions were performed using Gateway LR Clonase II enzyme mix according to the manufacturer’s instructions (Invitrogen). Bait and prey plasmid were cotransformed into yeast AH109 strain using a high-efficiency lithium acetate method (Gietz and Schiestl, 2007). Yeast transformed with bait and prey plasmids were identified by selection on synthetic defined (SD) media plates lacking Leu and Trp (SD/−Leu/−Trp). Positive colonies were cultured in fresh SD/−Leu/−Trp liquid medium. Dilution series were spotted onto SD/−Leu/−Trp medium or SD medium lacking Leu, Trp, and His plus 8 mm 3-amino-1,2,4-triazole (3-AT; Sigma-Aldrich; SD/−Leu/−Trp/−His/+3-AT) for assessment of His reporter gene activity. A 3-AT concentration (8 mm) sufficient to distinguish positive growth from background was determined empirically. Dilution series were spotted in replicate onto SD/−Trp/−Leu/−His/+3-AT media supplemented with 20 mg L−1 of X-α-Gal (Clontech) for assessment of α-galactosidase reporter gene activity.
BiFC Assay
Protein-protein interactions in planta were analyzed using a Gateway-compatible BiFC system (Tsai and Gazzarrini, 2012). Donor vectors for yeast two-hybrid were used for LR recombination into BiFC vectors to obtain nYFP-BOP1, nYFP-BOP2, cYFP-PAN, cYFP-TGA1, and cYFP-TGA4 fusions, respectively. BiFC constructs were introduced into A. tumefaciens GV3101 by electroporation. Leaves of 6-week-old Nicotiana benthamiana plants were infiltrated as described previously (Li et al., 2009). Samples were imaged using a Carl Zeiss LSM800 Airyscan confocal laser scanning microscope 4 d after infiltration. Nuclei were stained by incubating leaf discs in distilled water containing 5 µg mL−1 of the reagent 4′,6-diamidino-2-phenylindole (DAPI; Sigma-Aldrich). Afterward, leaf discs were mounted on a microscope slide for observation through the abaxial side. For visualization of YFP, chlorophyll a, and DAPI fluorescence, excitation lasers of 488 nm, 488 nm, and 405 nm, respectively, were used. Emission bands of 410–605 nm for YFP, 650–700 nm for chlorophyll a, and 410–605 nm for DAPI were acquired using gallium-arsenide-phosphide detectors and a Plan-Apo 40×/1.4 oil objective. Images were processed using Zen 2.3 software (Carl Zeiss).
Construction of TGA1pro:TGA1-GFP and TGA4pro:TGA4-GFP tga1 tga4 Transgenic Plants
ChIP-qPCR assays were used to evaluate binding of TGA1 and TGA4 proteins to A-box sites in the ATH1 promoter, which was previously identified as enriched for BOP1 (Khan et al., 2015). For this, translational fusions to GFP were generated to allow use of commercially available anti-GFP antibody for ChIP. Translational fusions were expressed behind the same promoters as used for GUS lines. To create TGA1pro:TGA1-GFP, a genomic DNA fragment including the 5′ untranslated and coding region of TGA1 without a stop codon (nucleotides −4122 to +1716) was amplified from BAC DNA using iProof as the polymerase (BioRad). The resulting fragment was cloned into Gateway-compatible entry vector pENTR/D-TOPO (Invitrogen). The insert was sequenced to ensure fidelity. Translational fusion with GFP was achieved by moving the TGA1pro:TGA1 insert into destination vector pMDC107 (Curtis and Grossniklaus, 2003) using LR Clonase (Invitrogen). After LR recombination, the junction between TGA1 and GFP was sequenced to ensure the fusion was in frame. To create TGA4pro:TGA4-GFP, DNA sequences containing the putative TGA4 promoter and coding sequences of TGA4 and GFP were joined together in the binary vector pBAR1 (Holt et al., 2002) by Gibson assembly (Gibson et al., 2009). The 5′ untranslated sequence of TGA4 (nucleotides −3949 to +0) was amplified from BAC DNA. The coding sequence of TGA4 without a stop codon was amplified from cloned complementary DNA (cDNA) template. The GFP coding sequence was amplified from pMDC107. TGA4 and GFP coding sequences were first assembled in pBAR1 linearized with XbaI using In-Fusion (Clontech). The resulting plasmid was linearized with BamHI for incorporation of the TGA4pro sequence resulting in the final construct. The assembled inserts were sequenced to ensure fidelity.
Constructs were introduced into tga1 tga4 mutant plants. TGA1pro:TGA1-GFP primary transformants were selected on agar plates containing 25 mg mL−1 hygromycin B. TGA4pro:TGA4-GFP primary transformants were selected on agar plates containing 10 mg mL−1 phosphinothricin. Homozygous lines were generated for downstream experiments.
To monitor the subcellular localization of fusion proteins, transgenic seedlings were imaged under a LSM800 Airyscan confocal laser scanning microscope (Carl Zeiss). For visualization of GFP and chlorophyll a, excitation lasers of 488 nm were used. Emission bands of 500–617 nm for GFP and 645–700 nm for chlorophyll a were acquired using gallium-arsenide-phosphide detectors and a Fluor 20×/0.75 M27 objective. Images were processed with ZEN 2.3 software (Carl Zeiss).
Tunicamycin Assays
Surface-sterilized seeds were germinated on one-half strength Murashige and Skoog (MS) medium supplemented with 1% (w/v) Suc. Five-day-old seedlings were immersed in liquid one-half strength MS media with or without 0.8 µg mL−1 tunicamycin (TM; Sigma Aldrich) for 6 h. After treatment, the seedlings were rinsed three times with liquid one-half strength MS media and then recovered for 5 d on fresh one-half strength MS plates with 1% (w/v) Suc. Fresh weight of seedlings (n = 5 per treatment group, 5–6 replicates) were measured and quantified essentially as described (Wang and Fobert, 2013). The experiment was repeated twice with similar results.
ChIP Assay
The ChIP assay was performed according to a previously described protocol (Gendrel et al., 2005) using an anti-GFP antibody (Ab290; Abcam). Homozygous TGA1pro:TGA1-GFP tga1 tga4, TGA4pro:TGA4-GFP tga1 tga4, and tga1 tga4 seeds were germinated on minimal media under long days and transferred to soil for continued growth. Apex tissue was collected from 6-week-old flowering plants for analysis. Quantification of immunoprecipitated DNA by qPCR was performed. The input percentage of each primer set was first obtained by comparing cycle threshold (Ct) value with input DNA. The fold enrichment was then calculated using input percentage of GFP-immunoprecipitated samples against no-antibody control samples. The fold enrichment value of the UBIQUITIN5 primer set (Boyle et al., 2009) was used to normalize data. Final results were shown relative to the tga1 tga4 genetic control. Primers used for ChIP-qPCR are listed in Supplemental Table S2.
RT-qPCR
Total RNA was isolated from seedlings, apices, or internodes using Trizol reagent (Invitrogen). Plant seeds were germinated on minimal media plates under long days. Ten-day-old seedlings (25–30 per replicate) were harvested for total RNA isolation. For apices, 5-d-old seedlings were transferred onto soil for continued growth under long days. Dissected apices (10–15 per replicate) were <0.5 cm tall, with the majority of flowers under stage 13. For internodes, a fresh razor blade was used to harvest tissue from the primary and secondary inflorescences of 5 to 6 plants per replicate, starting above the first silique from the bottom and all the way to where the internodes were too small (<0.2 cm long) to collect.
For internodes, cDNA was synthesized using 1 µg of RNA and RT-qPCR was performed as previously described (Khan et al., 2012b). For seedlings and apices, RNA samples were treated with Turbo DNase I (Ambion) at 37°C for 30 min followed by treatment with DNase Inactivation Reagent (Ambion) according to the manufacturer’s instructions. Reverse transcription was performed using 1 µg of total RNA and SuperScript III Reverse Transcriptase (Invitrogen) with Oligo d(T) according to the manufacturer’s instructions. Quantitative PCR was carried out using 2 µL of 10-fold-diluted cDNA, gene specific primers (Supplemental Table S2), and Power SYBR Green PCR MasterMix (Applied Biosystems) on a StepOnePlus Thermocycler (Applied Biosystems). Results were calculated using the ∆Ct method (Pfaffl, 2001). The arithmetic mean of GLYCERALDEHYDE-3-PHOSPHATE DEHYDROGENASE C SUBUNIT (GAPC), TUBULIN BETA CHAIN 4 (TUB4), and EUKARYOTIC TRANSLATION INITIATION FACTOR 4A1 (EIF4A1) were used as normalization controls. At least two technical replicates were performed for each sample. Data shown are the average of three independent biological replicates. Error bars represent the se.
Accession numbers
Sequence data from this article can be found in the EMBL/GenBank data libraries under accession numbers At5g02030 (PNY), At2g27990 (PNF), At3g57130 (BOP1), At2g41370 (BOP2), At1g68640 (PAN), At5g65210 (TGA1), At5g10030 (TGA4), At4g32980 (ATH1), At3g04120 (GAPC), At5g44340 (TUB4), At3g13920 (EIF4A1), and At3g62250 (UBQ5).
Supplemental Data
The following supplemental materials are available.
Supplemental Figure S1. Quantitative analyses of pny and pny pnf phenotypic rescue by tga1 and/or tga4.
Supplemental Figure S2. TGA1pro:GUS and TGA4pro:GUS expression in wild-type and pny pnf shoot apices.
Supplemental Table S1. List of differentially expressed TGA genes.
Supplemental Table S2. List of primers.
ACKNOWLEDGMENTS
We thank Thearany Lay for microscopy images of GUS-stained stem and inflorescence apex sections, Denise Chabot and Keith Hubbard at Agriculture and Agri-Food Canada for confocal microscopy support, and Véronique Pautot for critical reading of the manuscript.
LITERATURE CITED
Author notes
This work was supported by the Natural Sciences and Engineering Research Council (NSERC grant no. 327195 to S.R.H.). B.C.S. was supported by a Douglas Anglin Scholarship. Y.W. was supported by Ontario Graduate Scholarships in 2017–2018 and 2018–2019.
Present address: Biological Sciences Department, University of Zambia, Lusaka, Zambia 32379.
Present address: Department of Cell and Systems Biology, University of Toronto, Toronto, Ontario, Canada M5S 3B2.
Author for contact: [email protected].
Senior author.
The author responsible for distribution of materials integral to the findings presented in this article in accordance with the policy described in the Instructions for Authors (www.plantphysiol.org) is: Shelley R. Hepworth ([email protected]).
All authors made essential contributions to the project. Y.W. conducted most of the experiments and co-wrote the article. M.K. and M.B. initiated the project and generated preliminary data. B.C.S. and B.D. provided additional data. R.S. contributed expertise and access to confocal microscopy. S.R.H. conceived the project, supervised the experiments, and co-wrote the article.
Articles can be viewed without a subscription.