-
PDF
- Split View
-
Views
-
Cite
Cite
Dugald Reid, Huijun Liu, Simon Kelly, Yasuyuki Kawaharada, Terry Mun, Stig U. Andersen, Guilhem Desbrosses, Jens Stougaard, Dynamics of Ethylene Production in Response to Compatible Nod Factor , Plant Physiology, Volume 176, Issue 2, February 2018, Pages 1764–1772, https://doi.org/10.1104/pp.17.01371
- Share Icon Share
Abstract
Establishment of symbiotic nitrogen-fixation in legumes is regulated by the plant hormone ethylene, but it has remained unclear whether and how its biosynthesis is regulated by the symbiotic pathway. We established a sensitive ethylene detection system for Lotus japonicus and found that ethylene production increased as early as 6 hours after inoculation with Mesorhizobium loti. This ethylene response was dependent on Nod factor production by compatible rhizobia. Analyses of nodulation mutants showed that perception of Nod factor was required for ethylene emission, while downstream transcription factors including CYCLOPS, NIN, and ERN1 were not required for this response. Activation of the nodulation signaling pathway in spontaneously nodulating mutants was also sufficient to elevate ethylene production. Ethylene signaling is controlled by EIN2, which is duplicated in L. japonicus. We obtained a L. japonicus Ljein2a Ljein2b double mutant that exhibits complete ethylene insensitivity and confirms that these two genes act redundantly in ethylene signaling. Consistent with this redundancy, both LjEin2a and LjEin2b are required for negative regulation of nodulation and Ljein2a Ljein2b double mutants are hypernodulating and hyperinfected. We also identified an unexpected role for ethylene in the onset of nitrogen fixation, with the Ljein2a Ljein2b double mutant showing severely reduced nitrogen fixation. These results demonstrate that ethylene production is an early and sustained nodulation response that acts at multiple stages to regulate infection, nodule organogenesis, and nitrogen fixation in L. japonicus.
Legume plants have the capacity to enter into symbiotic relationships with nitrogen-fixing bacteria collectively referred to as rhizobia. The development of a symbiotic nodule is dependent on host-microbe compatibility and is regulated by the plant. Perception of rhizobia-derived lipochitooligosaccharide Nod factors (NF) by the plant is a key determinant of compatibility and initiates host transcriptional and developmental changes. The common symbiotic pathway, so called because of its shared requirement in symbiosis with arbuscular mycorrhizal fungi, is central to these changes (Kistner and Parniske, 2002). Many components of this pathway, and of nodulation specific up- and downstream pathways, have been identified in both model legumes Medicago truncatula and Lotus japonicus, and we name here the genetic components from the latter. Nodulation signaling first requires the NF receptors NFR1 (Radutoiu et al., 2003) and NFR5 (Madsen et al., 2003; Radutoiu et al., 2003) and their interaction with the SYMBIOSIS RECEPTOR KINASE (Stracke et al., 2002). Downstream of these receptors, oscillations (spiking) in nuclear calcium concentrations occur, which are decoded by the CALCIUM/CALMODULIN-DEPENDENT KINASE (CCaMK; Lévy et al., 2004; Tirichine et al., 2006). The nucleoporin, NENA, is also required for normal infection by both arbuscular mycorrhizal fungi and rhizobia (Groth et al., 2010). Transcriptional regulation occurs through interaction of CCaMK with CYCLOPS, which can directly induce expression of downstream targets (Singh et al., 2014). Downstream targets include transcriptional regulators such as NIN (Schauser et al., 1999), which through the heterotrimeric NF-Y complex regulates nodule differentiation (Soyano et al., 2013; Laporte et al., 2014; Baudin et al., 2015; Hossain et al., 2016). Additionally, NIN and the AP2/ERF transcription factor ERN1 (Cerri et al., 2012, 2016, 2017; Kawaharada et al., 2017; Yano et al., 2017) are transcriptionally activated by the GRAS-type transcription factors NSP1 and NSP2 (Kaló et al., 2005; Smit et al., 2005; Heckmann et al., 2006; Murakami et al., 2006; Hirsch et al., 2009). Nodulation signaling also induces cytokinin biosynthesis (Reid et al., 2017), and activation of the cytokinin receptor LHK1 is sufficient to induce nodule organogenesis (Tirichine et al., 2007; Murray et al., 2007).
Phytohormones play important roles in regulating symbiotic development and gene expression including common symbiosis pathway genes (Ferguson and Mathesius, 2014). Among these hormones, ethylene has been shown to play a number of specific roles. Treatment with ethylene, or the ethylene precursor ACC, inhibits Ca2+ spiking, symbiotic gene expression, infection thread (IT) development, and nodule organogenesis (Penmetsa and Cook, 1997; Oldroyd et al., 2001). Conversely, application of the ethylene synthesis inhibitor AVG increases nodulation and IT numbers (Nukui et al., 2000; Heckmann et al., 2011). In Medicago, the ethylene insensitive Mtsickle mutant (Penmetsa and Cook, 1997; Penmetsa et al., 2008) has dramatically altered transcriptional responses to Nod factor (Larrainzar et al., 2015) and fails to negatively regulate symbiotic signaling and cytokinin production (van Zeijl et al., 2015). Much of this work has indirectly implied the biosynthesis of ethylene during symbiotic initiation (for review, see Guinel, 2015), and elevated ethylene levels or biosynthesis transcripts have been shown in legumes inoculated with rhizobia (Ligero et al., 1986; Heidstra et al., 1997; Lopez-Gomez et al., 2012). However, the genetics and kinetics of ethylene production during early symbiotic interactions have not been studied with high temporal resolution, nor has its production been detected specifically in response to Nod factor. Ethylene biosynthesis depends on ACC Synthase (ACS) and ACC Oxidase, and gene families encoding these proteins are conserved in legumes (Desbrosses and Stougaard, 2011). ACS is generally rate-limiting, and stabilization of ACS proteins appears to be the major determinant of ethylene biosynthesis (for review, see Chae and Kieber, 2005). ACS stability is regulated by numerous phytohormones including cytokinin and auxin (Chae et al., 2003; Lee et al., 2017).
Studies in Arabidopsis (Arabidopsis thaliana) have revealed that the ethylene signaling pathway is negatively and redundantly regulated by five receptors: ethylene response 1 (ETR1), ETR2, ethylene response sensor 1 (ERS1), ERS2, and ethylene insensitive 4 (EIN4; for review, see Ju and Chang, 2015). In the absence of ethylene, these receptors maintain constitutive triple response 1 (CTR1) in an active form to repress EIN2 function. In response to ethylene, CTR1 is inactivated, allowing cleavage of EIN2 and the nuclear translocation and activity of the C-terminal end of EIN2. This regulates downstream transcription through EIN3 and related transcription factors (Ju et al., 2012).
Ethylene signaling is a key regulator of rhizobia infection and nodule organogenesis, as mutants in Ein2 are hypernodulating and hyperinfected (Penmetsa and Cook, 1997; Penmetsa et al., 2008; Foo et al., 2016). Overexpression of the dominant negative AtETR1-1 allele in L. japonicus is also sufficient to produce ethylene insensitive lines with similar phenotypes (Lohar et al., 2009). In L. japonicus, understanding the role of LjEin2 has been somewhat hindered by the duplication of this gene. Mutant alleles of Ljein2a were identified and named enigma due to their unexpected failure to show increased nodulation (Chan et al., 2013). RNAi studies have indicated that this is likely due to functional redundancy as parallel inhibition of both copies resulted in increased nodulation (Miyata et al., 2013).
Here, we describe the development of a sensitive and widely applicable ethylene detection assay for L. japonicus that allows direct measurements of ethylene production at small time scales (hours) from a small number of seedlings. We also conducted genetic analyses and confirm that both LjEin2a and LjEin2b act redundantly to regulate sensitivity to ethylene in L. japonicus. In addition, we show that L. japonicus produces rhizobia- and NF-dependent ethylene accumulation in the early phases of symbiotic interaction. Genetic analyses indicated that activation of the nodulation signaling pathway was both required and sufficient for this response.
RESULTS
Dynamics of Rhizobia-Induced Ethylene Production
Taking advantage of the high ethylene sensitivity of a laser-based detection system (Cristescu et al., 2013), we designed an assay to study ethylene emission in L. japonicus during early interactions with rhizobia. Growing seedlings on filter paper in 5-mL glass GC vials sealed with synthetic stoppers allowed consistent measurements with low background to be conducted on individual or small numbers of plants. To determine the pattern of ethylene production during the early stages of nodulation, plants were inoculated with M. loti R7A or a mock inoculum and the vials left uncapped until 3 hours before the indicated measurement time. Each measurement point therefore represented the hourly ethylene production rate in the 3 preceding hours. This data showed that M. loti R7A triggers a rapid and significant increase in ethylene production from as early as 6 hpi (Fig. 1). The ethylene production rate of inoculated plants continues to increase until 24 hpi and remained significantly elevated relative to mock treatment throughout the series of measurements.
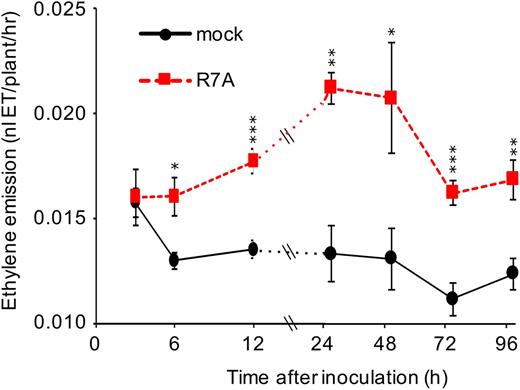
Ethylene emission of L. japonicus during interaction with M. loti. Five-day-old plants were inoculated and grown in unsealed glass vials until capping 3 h prior to each measurement point. Measurements were conducted on independent biological replicates at each time and condition for n = 6. Asterisks represent statistical comparison between mock and R7A by t test at each time: *< 0.05, **< 0.01, ***< 0.001.
The Ethylene Response Depends on the Nodulation Signaling Pathway
Given the close involvement of ethylene in regulating activity of the nodulation signaling pathway, we aimed to determine whether the production of ethylene following rhizobia inoculation was dependent on components of this pathway. Due to the peak of ethylene production occurring at 24 h (Fig. 1), for further experiments, we assayed ethylene production over the first 24 h following inoculation. We measured ethylene in a series of nodulation mutants impaired at different stages of Nod factor-dependent signaling (Fig. 2). Wild-type L. japonicus showed a very significant increase in ethylene production, while mutants in Nod factor perception (nfr1-1 and nfr5-2) showed no response to M. loti R7A inoculation. Similarly, mutants impaired in decoding of calcium spiking (ccamk-13) failed to significantly increase ethylene production. Mutation of the nucleoporin NENA (nena-1) showed a moderate ethylene response to rhizobia. Transcription factors acting downstream of calcium spiking showed moderate (nsp1-1; nsp2-3) or very significant (cyclops-2; nin-2; ern1-2) increases in ethylene production in response to M. loti inoculation.
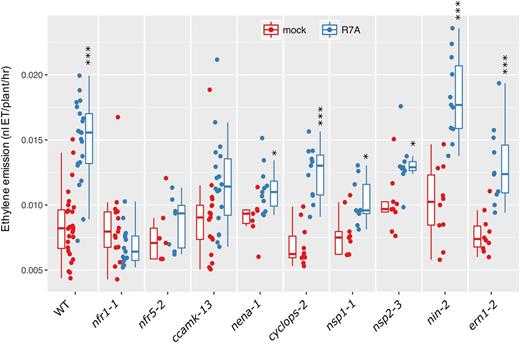
Ethylene emission of L. japonicus nodulation mutants. Ethylene emission over the first 24 h after treatment was determined for the indicated genotypes. Plants were 5 days old at the time of treatment. Asterisks represent statistical comparison between mock and R7A for each genotype using Holm corrected t test: *< 0.05, **< 0.01, ***< 0.001.
Gain-of-function mutations in CCaMK (snf1) and Lhk1 (snf2) are sufficient to induce spontaneous activation of the nodulation signaling pathway and spontaneous nodule organ formation (Tirichine et al., 2006; Tirichine et al., 2007). We measured ethylene production in these mutants and found that in the absence of rhizobia (mock-inoculation), ethylene production is significantly elevated in both mutants relative to wild type under the same conditions (Fig. 3). We did not detect a further enhancement in ethylene production from these mutants following inoculation with rhizobia (Fig. 3).
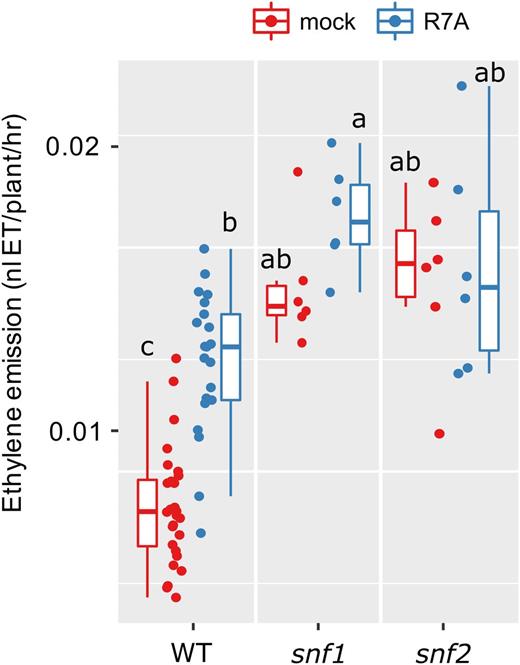
Ethylene emission of L. japonicus spontaneously nodulating mutants. Ethylene emission over the first 24 h after treatment was determined for the indicated genotypes. snf1 is a gain-of-function mutant in LjCCaMK, snf2 is a gain-of-function in LjLhk1. Plants were 5 days old at the time of treatment. Letters represent statistical differences determined by Tukey test at P < 0.05.
Host-Compatible Nod Factor Determines the Ethylene Response
Our measurements of ethylene production in the nodulation mutant series showed that L. japonicus ethylene production is dependent on the nodulation signaling pathway. To determine whether this response is dependent on the production and host specificity of the Nod factor, we inoculated L. japonicus with compatible (M. loti R7A), a Nod factor-deficient compatible (M. loti R7A nodC), and incompatible rhizobia (Sinorhizobium meliloti) strains. This showed that only the wild-type M. loti R7A significantly increases ethylene production, while the Nod factor-defective M. loti nodC mutant and incompatible rhizobia (S. meliloti) did not stimulate ethylene production above mock-treated levels (Fig. 4A). To further confirm the Nod factor specificity, we applied purified M. loti R7A Nod factor and measured ethylene production after 24 h. Nod factor application alone was sufficient to induce a significant increase in ethylene production (Fig. 4B).
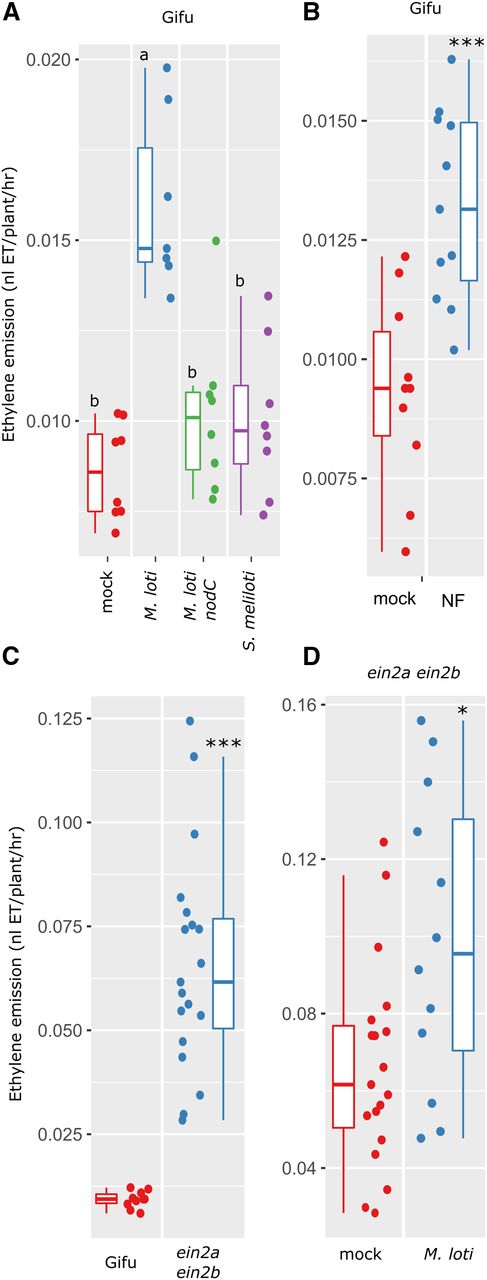
Dependence of L. japonicus ethylene emission on compatible Nod factor. A, Ethylene emission over first 24 h following inoculation of L. japonicus Gifu with M. loti, M. loti nodC, or incompatible S. meliloti as indicated. B, Ethylene emission over first 24 h following treatment with purified M. loti Nod factor. C, Ethylene emission over 24 h for L. japonicus Gifu or the Ljein2a-2 Ljein2b-1 mutant. D, Ethylene emission over 24 h for L. japonicus Ljein2a-2 Ljein2b-1 mutant inoculated with M. loti. Plants were 5 days old at the time of treatment. Letters in A represent statistical differences determined by Tukey test at P < 0.05. Asterisks in B to D represent comparison of the two treatments by t test. *< 0.05, **< 0.01, ***< 0.001.
LjEin2a and LjEin2b Act Redundantly in Ethylene Signaling
Duplication of Ein2 in L. japonicus has hindered the functional study of its product, which has therefore relied on analyses of Ljein2a mutants (Chan et al., 2013) or on simultaneous RNAi inhibition of both genes (Miyata et al., 2013). We isolated exonic LORE1 insertion mutants in LjEin2a (Lj1g3v4590690.1) and LjEin2b (Lj5g3v0659810.1) and obtained a double mutant with the Ljein2a-2 Ljein2b-1 LORE1 alleles (allele details listed in Supplemental Table S1). The severe phenotypes of the Ljein2a Ljein2b mutant are reminiscent of the M. truncatula sickle (Penmetsa et al., 2008) and Arabidopsis ein2 (Alonso et al., 1999) mutants, suggesting complete ethylene insensitivity of the double mutant. To quantify this, we analyzed hypocotyl elongation of the Ljein2a Ljein2b double mutant, which is one of the classical triple response tests. Wild-type L. japonicus Gifu plants are severely affected by ACC application, displaying progressively reduced hypocotyl length at increased ACC concentrations, while the Ljein2a Ljein2b double mutant was completely insensitive to ACC application with long and unaltered hypocotyls at all tested concentrations (Fig. 5, A and B).
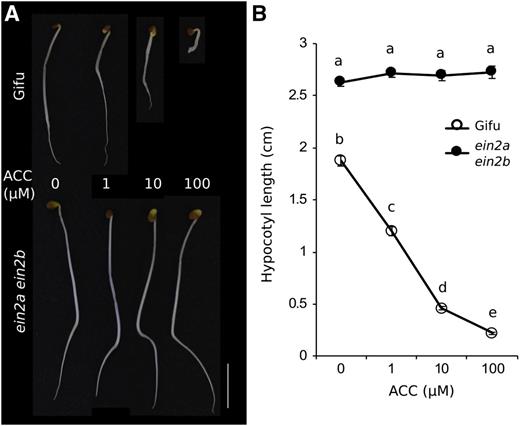
Ethylene sensitivity of Ljein2a Ljein2b mutants. A, Hypocotyl elongation test of Gifu and ein2a ein2b plants germinated in the dark in the presence of the indicated ACC concentrations. Scale bar = 1 cm. B, Quantification of hypocotyl length of Gifu and Ljein2a Ljein2b in the elongation test. Plants were germinated in the dark for 7 days. Different letters indicate significant difference as determined using Tukey posthoc testing with P < 0.01 and n = 33 to 51.
Analyses of nodulation phenotypes indicated that single Ljein2 mutants did not exhibit large effects, with only Ljein2b mutants showing a small but significant increase in nodulation (Fig. 6, A and C). Conversely, the Ljein2a Ljein2b double mutant displayed a significant hypernodulation phenotype (Fig. 6, A and C). The double mutant was also hyperinfected, with infection threads forming over the length of the root (Fig. 6B). Quantification of infection thread density showed that the Ljein2a Ljein2b double mutant is significantly hyperinfected relative to the wild type and both single mutants (Fig. 6E). Among single mutants, the Ljein2b mutant has significantly increased infection relative to wild type (Fig. 6E). In our conditions, we did not detect reduced infection thread density of the Ljein2a mutant that was previously reported in EMS derived mutants (Chan et al., 2013). We observed that, despite the hypernodulation phenotype, the Ljein2a Ljein2b mutant appeared to be nitrogen starved and nodules remained white or light pink in color (Fig. 6A). An acetylene reduction assay was conducted to quantify the nitrogen fixation in these plants, which revealed a significantly reduced nitrogen fixation capacity 2 weeks after inoculation (Fig. 6D). Sectioning of nodules from wild type and Ljein2a Ljein2b did not reveal obvious colonization defects in the mutant (Supplemental Fig. S1).
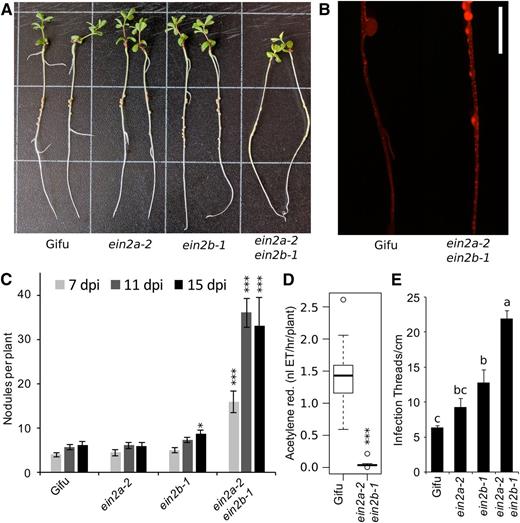
Nodulation phenotypes of Ljein2 mutants. A, Nodulation phenotypes of wild-type Gifu, Ljein2a-2, and Ljein2b-1 single and double mutants grown for 15 d after inoculation with M. loti R7A. B, Infection phenotype of wild-type Gifu and Ljein2a-2 Ljein2b-1 inoculated with M. loti MAFF303099 DsRED. C, Quantification of nodule numbers at the indicated time-points after inoculation with M. loti R7A. D, Acetylene reduction activity of whole roots 14 d after inoculation with M. loti R7A. E, Quantification of infection threads 10 d after inoculation with M. loti R7A. Grid squares = 3 cm in A, scale bar = 5 mm in B. Asterisks represent statistical difference to wild-type Gifu at each time-point as determined using posthoc Dunnett test in C and Wilcox rank-sum test in D. Letters in E represent statistically significant differences determined by Tukey test. n = 19 to 30 in C, 10 in D, and 5 to 8 in E. *< 0.05, **< 0.01, ***< 0.001.
To determine whether the ethylene signaling pathway is required for rhizobia-dependent ethylene production, we also conducted ethylene measurements on the Ljein2a Ljein2b double mutant. These measurements showed that the ethylene production baseline is greatly elevated in Ljein2a Ljein2b mutants (Fig. 4C), while a further elevation of ethylene in a rhizobia dependent manner is still maintained (Fig. 4D). However, unlike the wild type, treatment of Ljein2a Ljein2b mutants with purified NF did not significantly increase ethylene production (Supplemental Fig. S2).
DISCUSSION
We developed a sensitive laser-based assay for determining ethylene emission from L. japonicus that allowed detection of ethylene production at high resolution in the early phases of rhizobial interactions. This allowed us to verify that rapid induction of ethylene biosynthesis genes (Larrainzar et al., 2015) leads to a rapid rhizobia-dependent increase in ethylene production. The clear phenotypes of ethylene-insensitive mutants (Penmetsa and Cook, 1997) and rapid transcriptional up-regulation of ethylene responsive genes (Larrainzar et al., 2015) has previously indicated that this was likely to be the case. The substantial interaction of ethylene with early Nod factor signaling highlights the importance of this early stimulation of ethylene production in regulating nodulation events. The sensitivity of the assay we have developed will allow further investigation of a number of proposed interactions between rhizobial symbiosis and ethylene. This includes crosstalk of other hormones with identified relationships to nodulation and ethylene signaling such as gibberellin (Ferguson et al., 2011), jasmonic acid (Sun et al., 2006), abscisic acid (Ding et al., 2008), and cytokinin (Lorteau et al., 2001; Ferguson et al., 2005; van Zeijl et al., 2015). Relationships between ethylene and the autoregulation (Penmetsa et al., 2003; Gresshoff et al., 2009) or nitrate regulation (Ligero et al., 1991) of nodulation may also be characterized in greater detail. Ethylene has also been shown to positively regulate nodulation that occurs via intercellular “crack entry” infection in Sesbania (D’Haeze et al., 2003). Investigation of ethylene responses to rhizobia that infect in this manner in Lotus spp. (Acosta-Jurado et al., 2016) may help to identify if this regulation is common to this type of infection.
It is well established that ethylene production is stimulated by pathogen-associated molecular patterns (Felix et al., 1999), a response that is conserved in L. japonicus treated with Flg22 peptide (Lopez-Gomez et al., 2012). The ability of purified Nod factor to stimulate ethylene production, together with the absence of any response to incompatible, or Nod factor-deficient, rhizobia shows that the early responses we detected are symbiotic rather than a moderated pathogenic response. The timing of ethylene production we observed is consistent with transcriptional profiles reported in M. truncatula, where ethylene-dependent signaling was identified from 3 to 6 h (Larrainzar et al., 2015). Ethylene then remained elevated over the period of our experiment following a peak at 24 h. Our system is limited to the early stages of nodulation as plants outgrow the measurement system. Modifications to extend the system to later times, when nodules emerge, could answer whether ethylene remains elevated over periods of weeks.
We found that the nodulation pathway is a key determinant of ethylene responsiveness. Ethylene emission of L. japonicus treated with M. loti deficient in Nod factor production was indistinguishable from mock-treated plants. This was further supported by the requirement for NFR1 and NFR5 in this response and the ability of purified Nod factor to initiate ethylene production. Downstream of Nod factor perception, there is likely some redundancy or branching in the common symbiotic pathway as CCaMK is required, while NENA, CYCLOPS, NIN, and ERN1 are not required, or are only partially required, for stimulation of ethylene. NSP1 and NSP2 may play a role in activation of ethylene as mutants in these show a significant, albeit reduced, M. loti-dependent increase. It is known that cytokinin can stimulate ethylene biosynthesis, at least in part by increasing the stability of ACS (Chae et al., 2003). Our finding that the snf2 gain-of-function mutation in the L. japonicus Lhk1 cytokinin receptor increases ethylene is consistent with this well-established cytokinin-ethylene crosstalk. The elevated ethylene in snf1 may suggest that elevated cytokinin levels exist in this mutant, further highlighting the role of the nodulation signaling pathway in the induction of cytokinin production (Reid et al., 2017).
Our analyses of a double mutant confirmed the redundancy of LjEin2a and LjEin2b in regulating ethylene signaling in both symbiotic and nonsymbiotic contexts. This clarified previous work that had used either single mutants (Chan et al., 2013) or RNAi approaches (Miyata et al., 2013) to study these genes. The significant hypernodulation and hyperinfection phenotypes of the Ljein2a Ljein2b double mutant demonstrates that ethylene signaling acts to negatively regulate both infection and organogenesis pathways in legumes forming both determinate (L. japonicus, this study) and indeterminate (M. truncatula, Penmetsa et al., 2008) nodules. Interestingly, the Ljein2a Ljein2b double mutant does not show acetylene reduction activity 2 weeks after inoculation, despite apparently normal nodule infection. The fact that the nodules are colonized may indicate that the onset of nitrogen fixation is delayed or reduced rather than completely lost. In her review, Guinel (2015) indicates ethylene regulation of bacteroid differentiation as a likely determinant of nodule function, which may account for our observation in Ljein2a Ljein2b mutants. We have previously reported that mutants in Ljckx3, which have elevated levels of cytokinin, also have reduced nitrogen fixation (Reid et al., 2016). Mtsickle also has greatly increased cytokinin following Nod factor treatment (van Zeijl et al., 2015). It is therefore possible the reduced nitrogen fixation is a result of elevated cytokinin in Ljein2a Ljein2b mutants rather than a direct effect of ethylene. It remains a possibility that ethylene exerts a direct effect on rhizobia signaling, although ethylene perception and signaling is not defined in bacteria.
The massive stimulation of ethylene biosynthesis in the Ljein2a Ljein2b mutant indicates the presence of negative feedback mechanisms on the ethylene biosynthesis pathway. It has previously been shown in Arabidopsis that Atein2 mutants also overproduce ethylene (Guzmán and Ecker, 1990). We have shown that the rhizobia induced increase in ethylene production is maintained in Ljein2a Ljein2b, demonstrating that despite the high levels, further activation of biosynthesis is possible. This stimulation, however, results in a relatively lower fold-induction compared to that in wild type. The greater effect of rhizobia compared to purified NF and reduced relative induction in Ljein2a Ljein2b mutants may account for the absence of NF stimulation of ethylene in this mutant. The high levels of ethylene in Ljein2a Ljein2b and a complete insensitivity in the hypocotyl elongation assay suggests that this mutant is completely insensitive to ethylene. The complete insensitivity of the mutant to ethylene shows a functional ethylene signaling pathway is not required for Nod factor signaling, infection thread development, or nodule organogenesis but is required for initiation of nitrogen fixation and negative regulation of nodulation.
In conclusion, we show that ethylene production is rapidly induced by nodulation signaling in a Nod factor-dependent manner. The nodulation signaling pathway is both required and sufficient to induce this ethylene accumulation. Mutant studies showed that Ein2 in L. japonicus is duplicated and that the two copies are functionally redundant, with mutants exhibiting complete ethylene insensitivity. Ethylene therefore negatively regulates infection and organogenesis in both determinate and indeterminate nodulating species via Ein2.
MATERIALS AND METHODS
Plant and Bacterial Genotypes and Growth Conditions
Lotus japonicus ecotype Gifu was used in all experiments (Handberg and Stougaard, 1992). Homozygous LORE1 inserts were genotyped with allele-specific primers in combination with the P2 internal LORE1 primer as described (Urbański et al., 2012). Primer sequences were obtained from the LORE1 resource page (Małolepszy et al., 2016; Mun et al., 2016) or designed in the same region of the reverse primer if amplification was unsuccessful (primers listed in Supplemental Table S1). Sinorhizobium meliloti 1021 (Meade et al., 1982), Medicago loti R7A (Sullivan et al., 1995), and the NF defective M. loti R7A nodC variant (Rodpothong et al., 2009) were diluted to an inoculum density of OD600 = 0.01. For infection thread quantification, an M. loti R7A DsRed strain was used. For nonquantitative infection phenotyping, the M. loti MAFF303099 strain carrying chromosomal DsRed insertion was used (Maekawa et al., 2009). For phenotyping, 3-d-old seedlings were transferred to square petri plates with 1.4% agar slants covered with filter paper. The agar slants contained nitrate free quarter-strength B&D nutrients (Broughton and Dilworth, 1971), and light was blocked from reaching the roots with metal grids.
Ethylene Measurements
For ethylene measurements, two 3-d-old seedlings were transferred to 5-mL glass GC vials containing filter paper cut to fit and 750 µL nitrate-free quarter-strength B&D nutrients. The seedlings were acclimatized in the vials for 2 d before inoculation with 250 µL rhizobia (OD600 = 0.01), directly applied to the roots. The GC vials were capped after inoculation using synthetic stoppers from Vacuette Z No-Additive tubes (Greiner Bio-One, product no. 455001). Ethylene accumulation over the following 24 h was then measured. For the time-series, vials were left uncapped until 3 h prior to the indicated measurement times. Each biological replicate refers to the two seedlings in independent vials. Ethylene measurements were conducted using an ETD-300 laser-based detection system (SensorSense). Measurements were conducted in sampling mode with a flow rate of 2.5 L/min and 6-min sample period. Ethylene emission data were calculated as nL ethylene evolved/plant/hour the vial was capped. Ethylene evolved in the acetylene reduction assay was quantified on the ETD-300 in the same manner as previously described (Reid et al., 2016).
Statistical Analyses
Statistical analyses were carried out using R software (R Core Team, 2015). Comparison of multiple groups included ANOVA followed by Tukey posthoc testing to determine statistical significance indicated by different letter annotations. Students t-test or Wilcoxon rank-sum test was used as indicated depending on sample size when making single comparisons. Biological replicates in ethylene measurements are plotted as individual points with summary box plots for each condition. Nodulation assays with larger numbers of replicates are plotted as mean with SE for the indicated number of biological replicates.
Accession Numbers
Sequence data from this article can be found in the Lotus Base (lotus.au.dk) data libraries under accession numbers Lj1g3v4590690.1 (LjEin2a) and Lj5g3v0659810.1 (LjEin2b).
Supplemental Data
The following supplemental materials are available.
Supplemental Table S1. List of gene identifiers and oligonucleotides.
Supplemental Figure S1. Nodule sections of L. japonicus Gifu and Ljein2a-2 Ljein2b-1 double mutant.
Supplemental Figure S2. Ethylene emission of Ljein2a Ljein2b in response to purified M. loti Nod factor.
ACKNOWLEDGMENTS
We thank Finn Pedersen and Karina Kristensen for glasshouse assistance; Clive Ronson, John Sullivan, and Nicolai Maolanon for purified Nod factor; and Krzysztof Szczyglowski, Mandana Miri, and Brett Ferguson for helpful discussion.
LITERATURE CITED
Author notes
This work was supported by the Danish National Research Foundation grant no. DNRF79; ENSA, Engineering Nitrogen Symbiosis for Africa; Chinese Scholarship Council fellowship to H.L.
Current address: Department of Plant BioSciences, Faculty of Agriculture, Iwate University, 3-18-8 Ueda, Morioka, Iwate, Japan.
Address correspondence to [email protected].
The author responsible for distribution of materials integral to the findings presented in this article in accordance with the policy described in the Instructions for Authors (www.plantphysiol.org) is: Jens Stougaard ([email protected]).
D.R., S.K., Y.K., S.U.A., and J.S. conceived the research plan; D.R. and H.L. conducted experiments; G.D., S.K., and D.R. developed methodology, H.L., D.R., T.M. identified mutants; D.R. prepared the figures and wrote the manuscript with input from all authors.
Articles can be viewed without a subscription.