-
PDF
- Split View
-
Views
-
Cite
Cite
Ghazanfar Abbas Khan, Evangelia Vogiatzaki, Gaétan Glauser, Yves Poirier, Phosphate Deficiency Induces the Jasmonate Pathway and Enhances Resistance to Insect Herbivory , Plant Physiology, Volume 171, Issue 1, May 2016, Pages 632–644, https://doi.org/10.1104/pp.16.00278
- Share Icon Share
Abstract
During their life cycle, plants are typically confronted by simultaneous biotic and abiotic stresses. Low inorganic phosphate (Pi) is one of the most common nutrient deficiencies limiting plant growth in natural and agricultural ecosystems, while insect herbivory accounts for major losses in plant productivity and impacts ecological and evolutionary changes in plant populations. Here, we report that plants experiencing Pi deficiency induce the jasmonic acid (JA) pathway and enhance their defense against insect herbivory. Pi-deficient Arabidopsis (Arabidopsis thaliana) showed enhanced synthesis of JA and the bioactive conjugate JA-isoleucine, as well as activation of the JA signaling pathway, in both shoots and roots of wild-type plants and in shoots of the Pi-deficient mutant pho1. The kinetics of the induction of the JA signaling pathway by Pi deficiency was influenced by PHOSPHATE STARVATION RESPONSE1, the main transcription factor regulating the expression of Pi starvation-induced genes. Phenotypes of the pho1 mutant typically associated with Pi deficiency, such as high shoot anthocyanin levels and poor shoot growth, were significantly attenuated by blocking the JA biosynthesis or signaling pathway. Wounded pho1 leaves hyperaccumulated JA/JA-isoleucine in comparison with the wild type. The pho1 mutant also showed an increased resistance against the generalist herbivore Spodoptera littoralis that was attenuated in JA biosynthesis and signaling mutants. Pi deficiency also triggered increased resistance to S. littoralis in wild-type Arabidopsis as well as tomato (Solanum lycopersicum) and Nicotiana benthamiana, revealing that the link between Pi deficiency and enhanced herbivory resistance is conserved in a diversity of plants, including crops.
While a large number of studies have examined the adaptation and signaling pathways involved in the response of plants to a single stress, plants grown in nature typically are confronted by simultaneous stresses, both biotic and abiotic. The application of combined stresses often leads to responses that are distinct from those to individual stresses and display deleterious interactions (i.e. the adaptation to one stress leads to enhanced sensitivity to a distinct stress; Mittler and Blumwald, 2010). This is exemplified by the antagonistic interactions between the salicylate and jasmonic acid (JA)/ethylene pathways involved in the response to biotrophic pathogens and herbivory, respectively (Spoel et al., 2003). However, few examples of synergistic interaction between abiotic and biotic stresses have been described, such as the positive effect of short-term potassium deficiency on resistance to thrips (Frankliniella spp.; Armengaud et al., 2010).
The essential element phosphorus is one of the most limiting nutrients restraining plant growth in both natural and agricultural ecosystems (Lynch, 2011; MacDonald et al., 2011). Plants essentially acquire phosphorus through the uptake of soluble inorganic phosphate (Pi) by the roots via the PHT1 Pi transporters (Poirier and Jung, 2015). Although the phosphorus level in soil may be high, the concentration of soluble Pi in most soils is kept very low (low micromolar range) due to the formation of insoluble complexes of Pi with calcium, iron, and aluminum and the assimilation of Pi by the soil microflora. High-yield crop production in a majority of agricultural land is thus dependent on fertilizers based on nonrenewable phosphorus-rich mines (Obersteiner et al., 2013).
Plants respond to Pi-deficient conditions through numerous changes at the biochemical, developmental, and gene expression levels (Chiou and Lin, 2011; Plaxton and Tran, 2011; Zhang et al., 2014). These include reductions in shoot growth and primary root elongation, enhancement of the growth of lateral roots and root hairs, replacement of phospholipids by sulfolipids and galactolipids, secretion of phosphatases to scavenge Pi from organic sources, and accumulation of anthocyanins. At the molecular level, PHOSPHATE STARVATION RESPONSE1 (PHR1) is the main transcription factor responsible for the majority of transcriptional responses triggered by Pi deficiency (Rubio et al., 2001; Bustos et al., 2010). PHR1 controls the expression of miR399, a microRNA that moves from shoots to roots to repress the PHO2 transcript (Lin et al., 2008; Pant et al., 2009). PHO2 encodes a ubiquitin ligase involved in the degradation of PHO1, a Pi exporter expressed in the root xylem parenchyma and involved in the transfer of Pi from roots to shoots (Hamburger et al., 2002; Liu et al., 2012).
Herbivory is a major biotic stress responsible for the loss of a substantial fraction of the biomass produced annually in terrestrial ecosystems (Cyr and Face, 1993). JA is one of the most important hormones involved in the response of plants to herbivory-induced wounding, controlling the majority of insect-regulated genes in Arabidopsis (Arabidopsis thaliana) leaves (Acosta and Farmer, 2010). The synthesis and accumulation of JA and of its conjugate jasmonic acid-isoleucine (JA-Ile) are increased within minutes after wounding (Glauser et al., 2008). The JA signaling pathway involves the JAZ proteins acting as repressors of the MYC2 transcription factor, promoting the transcription of JA-responsive genes (Chini et al., 2007; Thines et al., 2007; Yan et al., 2007). Upon wounding, enhanced JA-Ile levels promote the degradation of JAZ proteins, including JAZ10, via JA-Ile interaction with the COI1 protein, an F-box protein recruiting a SCF-type E3 ubiquitin ligase (Yan et al., 2009). JAZ10 and MYC2 form a negative feedback loop, with JAZ10 degradation triggered by JA/JA-Ile, leading to its transcriptional activation via MYC2 (Chini et al., 2007; Thines et al., 2007; Yan et al., 2007).
JA induction and Pi starvation share some common phenotypes, including growth reduction and anthocyanin accumulation, suggesting a potential role of JA in the Pi deficiency response (Shan et al., 2009; Yang et al., 2012). Although numerous transcriptomic studies examining the responses of leaves or whole roots to Pi deficiency have been performed in model plants, such as Arabidopsis (Hammond et al., 2003; Misson et al., 2005; Morcuende et al., 2007; Müller et al., 2007; Bustos et al., 2010; Woo et al., 2012) and rice (Oryza sativa; Wasaki et al., 2006; Li et al., 2010; Secco et al., 2013), only one study (Morcuende et al., 2007) described changes in the expression of JA biosynthetic and signaling genes. A transcriptomic study focusing on Arabidopsis root tips revealed the down-expression of several JA-regulated genes in the low phosphorus insensitive4 (lpi4) mutant, indicating a potential role of JA in primary root elongation under Pi-deficient conditions (Chacón-López et al., 2011). Changes (up- and down-regulation) in the expression of some genes involved in the JA pathway by Pi deficiency also have been described in some transcriptomic studies in legumes, such as common bean (Phaseolus vulgaris; Aparicio-Fabre et al., 2013) and white lupin (Lupinus albus; O’Rourke et al., 2013; Wang et al., 2014). However, the potential role and consequences of the induction of the JA pathway in the response to Pi deficiency remain unknown.
In this work, we show that the JA signaling pathway and the synthesis of JA and JA-Ile are induced by Pi deficiency in both roots and shoots of Arabidopsis. Activation of the JA pathway in the Pi-deficient mutant pho1 leads to a reduction in shoot growth, the accumulation of anthocyanins in leaves, the hyperactivation of the wound response, and an increased resistance to Spodoptera littoralis herbivory. Pi deficiency also increased the resistance of Nicotiana benthamiana and tomato (Solanum lycopersicum) to S. littoralis, indicating that Pi deficiency-mediated herbivory resistance is conserved across several plant species.
RESULTS
Pi Deficiency Induces the JA Biosynthetic and Signaling Pathways
We first analyzed the potential effects of Pi deficiency on the JA pathway by measuring the expression of JAZ10, a gene participating in the JAZ-COI1-MYC2 signaling core and a marker for the activation of the JA signaling pathway (Chini et al., 2007; Thines et al., 2007; Yan et al., 2007). JAZ10 expression was increased 4- to 5-fold in both shoots and roots of wild-type Columbia-0 (Col-0) Arabidopsis plants grown on medium containing low Pi (100 µm) compared with high Pi (1 mm; Fig. 1, A and B). Under the same conditions, the expression of JAZ10 was highly induced in the tips (17-fold) of roots grown on low-Pi medium (Fig. 1C). The expression of JAZ10 in both shoots and roots of plants grown in high or low Pi was strongly reduced in the JA biosynthetic mutant aos lacking allene oxidase activity (Park et al., 2002) and the JA signaling mutant coi1-34 (a fertile allele of coi1; Acosta et al., 2013; Fig. 1, A and B). The expression pattern of two other markers of the JA pathway in leaves, namely VSP2 and LOX2, mirrored the expression of JAZ10 in leaves, being induced in leaves of Pi-deficient plants and this induction being abrogated in the aos and coi1-34 mutants (Fig. 1, D and G). In roots, VSP2 expression was induced in the root tips of Pi-deficient plants but no increase was detected when whole roots were analyzed, indicating that VSP2 expression in Pi-deficient plants is most likely restricted to the root tip (Fig. 1, E and F). LOX2 expression in roots was not affected by Pi deficiency in either whole roots or root tips (Fig. 1, H and I). This is in agreement with previous studies showing that, in contrast to leaves, LOX2 expression remains very low in roots of plants with induced JA biosynthesis following wounding (Grebner et al., 2013; Gasperini et al., 2015).
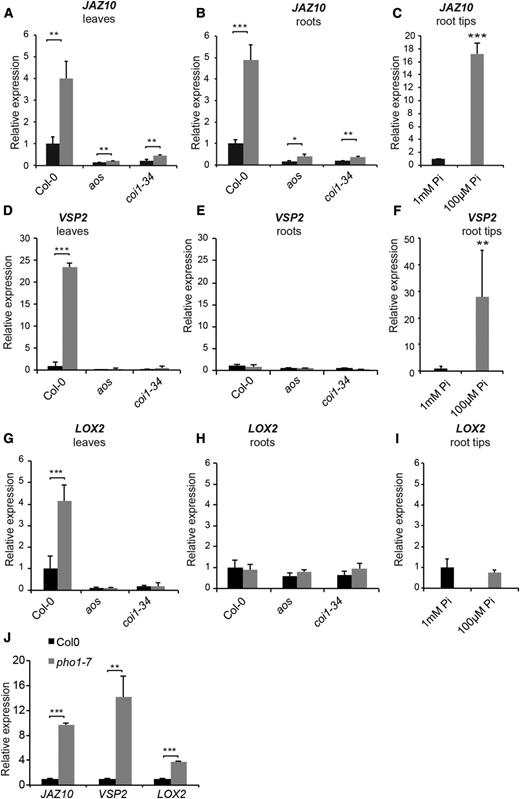
Genes involved in JA biosynthesis and signaling are induced in shoots and roots of Pi-deficient plants. A to I, Expression levels of JAZ10 (A–C), VSP2 (D–F), and LOX2 (G–I) measured in shoots (A, D, and G), whole roots (B, E, and H), and root tips (3 mm; C, F, and I) in Col-0 or the aos and coi1-34 mutants. Plants were grown for 12 d in medium containing 1 mm (black bars) or 100 µm (gray bars) Pi. J, Expression levels of JAZ10, VSP2, and LOX2 measured in shoots of Col-0 (black bars) and pho1-7 (gray bars) grown for 4 weeks in fertilized soil. For A to I, data are means ± sd of three samples obtained from independent plates, with three technical replicates for each sample and each sample consisting of a pool of tissues isolated from 10 plants for shoots, approximately 50 plants for roots, and approximately 150 plants for root tips. For J, data are means ± sd of three samples from plants grown in independent pots and three technical replicates for each sample, with each sample being a pool of three plants. Asterisks denote statistical significance (*, P < 0.05; **, P < 0.01; and ***, P < 0.001) according to Student’s t test. Experiments were performed independently three times with similar results.
The expression pattern of JA-responsive genes in plants grown on low-Pi medium and their dependence on both AOS and COI1 suggested that JA biosynthesis was induced under these conditions. Thus, JA and JA-Ile were quantified using liquid chromatography-mass spectrometry. Both JA and JA-Ile levels increased in shoots (Fig. 2, A and B) and roots (Fig. 2, E and F) of Col-0 plants grown in low-Pi medium. Wounding of leaves resulted in a greater accumulation of JA and JA-Ile compared with Pi deficiency, and the combination of both wounding and Pi deficiency resulted in small but significant increases of JA and JA-Ile compared with wounding alone (Fig. 2, C and D).
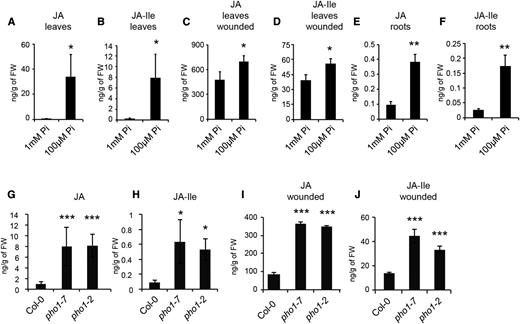
Enhanced levels of JA and JA-Ile in Pi-deficient tissues. A, B, E, and F, JA (A and E) and JA-Ile (B and F) contents in leaves (A and B) and roots (E and F) from plants grown for 12 d in medium containing 1 mm or 100 µm Pi. C and D, JA (C) and JA-Ile (D) contents in shoots from plants grown for 12 d in medium containing 1 mm or 100 µm Pi and 1 h after wounding. G to J, JA (G and I) and JA-Ile (H and J) in shoots of 4-week-old soil-grown Col-0, pho1-7, and pho1-2 plants sampled before wounding (G and H) or 1 h after wounding (I and J). For A to F, data are means ± sd of five samples obtained from independent plates, with each sample consisting of a pool of tissues isolated from 20 plants for leaves and 100 plants for roots. For G to J, data are means ± sd of five samples from plants grown in independent pots, with each sample being a pool of three plants. Asterisks denote statistical significance (*, P < 0.05; **, P < 0.01; and ***, P < 0.001) according to Student’s t test. For G to J, statistical analysis was performed comparing Col-0 with each of the pho1 mutants. Experiments were performed independently one time (I and J), two times (C, D, G, and H), or three times (A, B, E, and F) with similar results. FW, Fresh weight.
The PHO1 gene is involved in the loading of Pi into the root xylem for its transfer to shoots; consequently, pho1 mutants are Pi deficient in shoots (Hamburger et al., 2002). Shoots of pho1 mutants show the typical phenotypes related to Pi deficiency, including reduced growth, anthocyanin accumulation, a replacement of phospholipids with galactolipids and sulfolipids, and induction of Pi deficiency-related genes (Hamburger et al., 2002; Rouached et al., 2011). The pho1 mutant is thus an excellent tool to investigate shoot Pi deficiency and its long-term effects in soil-grown plants. Two alleles of pho1 were used, namely the previously described pho1-2 allele (Hamburger et al., 2002), having a nonsense point mutation in the fifth exon, and a new pho1-7 allele, having a transfer DNA in the second exon (Supplemental Fig. S1).
Quantitative PCR was performed on 4-week-old rosettes of soil-grown Col-0 and pho1-7 and revealed that the expression of JAZ10, VSP2, and LOX2 was induced in pho1-7 rosettes (Fig. 1J). Basal levels of JA and JA-Ile were increased in leaves of both pho1-7 and pho1-2 mutants compared with Col-0 (Fig. 2, G and H). While wounding of Col-0 rosettes resulted in expected increases in JA and JA-Ile levels, similar treatment of the pho1-7 and pho1-2 mutants resulted in 3- to 4-fold hyperaccumulation of both JA and JA-Ile relative to Col-0 (Fig. 2, I and J).
Induction of the JA Pathway by Pi Deficiency Is Partially Controlled by the PHR1 Transcription Factor
The transcription factor PHR1 is known to play an important role in the transcriptional activation of numerous genes by Pi deficiency and acts as a central regulator of the Pi starvation response (Rubio et al., 2001; Bustos et al., 2010). The impact of PHR1 on the activation of the JA pathway by Pi deficiency was assessed by analyzing JAZ10 expression in the phr1 mutant. While JAZ10 expression was enhanced 3-fold in seedlings of Col-0 grown for 7 d on Pi-deficient medium compared with Pi-sufficient medium, phr1-1 mutant seedlings showed no induction (Fig. 3A). However, after 10 d on Pi-deficient medium, both Col-0 and phr1-1 seedlings had a similar 7- to 8-fold induction of JAZ10. In contrast, expression of the IPS1 gene, known to be directly under the control of PHR1 (Rubio et al., 2001; Bustos et al., 2010), remained strongly down-regulated in phr1-1 compared with Col-0 after both 7 and 10 d of Pi deficiency (Fig. 3B). These results indicate that the kinetics of the induction of the JA signaling pathway by Pi deficiency is partially under the control of PHR1.
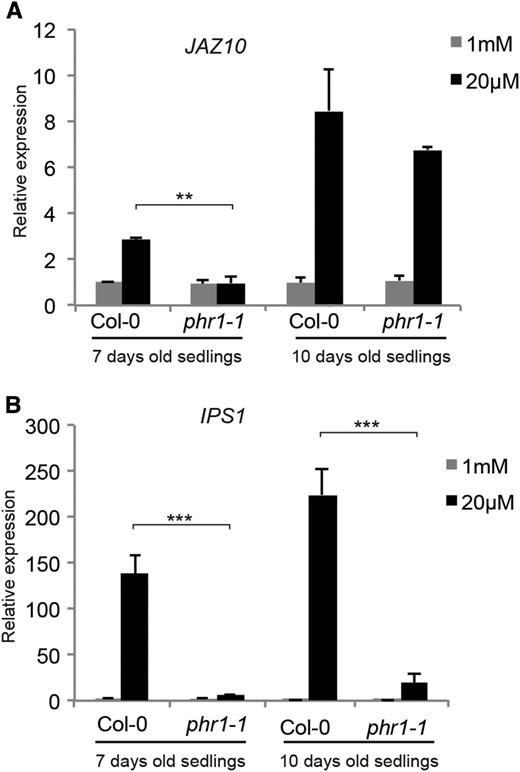
The kinetics of JAZ10 induction by Pi deficiency is influenced by PHR1. JAZ10 (A) and IPS1 (B) expression is shown in Col-0 and phr1-1 seedlings grown for 7 or 10 d in medium containing 1 mm (gray bars) or 20 µm (black bars) Pi. Data are means ± sd of three samples obtained from independent plates, with three technical replicates for each sample and each sample consisting of a pool of tissues isolated from 20 seedlings. Asterisks denote statistical significance (**, P < 0.01 and ***, P < 0.001) according to Student’s t test. Statistical analysis was done comparing Col-0 and phr1-1 within each treatment. Experiments were performed independently three times with similar results.
Contribution of the JA Pathway to Growth Phenotypes of Pi-Deficient Plants
Expression of the JA pathway in Arabidopsis is known to lead to reductions in shoot growth (Zhang and Turner, 2008). Accordingly, under our growth conditions, rosettes of the aos and coi1-1 mutant rosettes were larger than those of Col-0 plants, with an increase of approximately 25% in shoot fresh weight (Fig. 4, A and B). To test whether JA accumulation was involved in the reduction of pho1 shoot growth, double mutants between pho1-7 and aos or coi1-1 were generated. Shoot Pi contents in the double mutants were similar to those of the pho1-7 parent and low compared with Col-0 (Supplemental Fig. S2A). The shoot fresh weight of both pho1-7 aos and pho1-7 coi1-1 mutants was 2-fold higher compared with pho1-7 but remained 3-fold lower than Col-0, while the petiole length of pho1-7 aos and pho1-7 coi1-1 was comparable to Col-0 and 3.5-fold longer than that for pho1-7 (Fig. 4, A and B; Supplemental Fig. S2B). Furthermore, while the pho1 mutant strongly accumulated anthocyanin in rosettes, a phenotype typically associated with Pi deficiency, anthocyanin levels in the double mutants pho1-7 aos and pho1-7 coi1-1 were comparable to those of Col-0 plants (Fig. 4C). pho1-7 aos and pho1-7 coi1-1 double mutants also showed a significantly reduced induction of Pi starvation response genes, such as IPS1 and MGD3 (encoding a monogalactosyl diacylglycerol synthase involved in galactolipd synthesis), in the rosettes compared with the pho1-7 parent (Fig. 4, D and E). However, the increased proportions of sulfolipids and galactolipids relative to phospholipids, a biochemical phenotype typically associated with Pi deficiency and observed in the shoot of the pho1 mutant, were maintained in the pho1-7 aos and pho1-7 coi1-1 plants (Supplemental Fig. S3). JA and JA-Ile levels in the pho1-7 aos mutant were undetectable, and they were greatly reduced in the pho1-7 coi1-1 mutant compared with the pho1-7 parent (Fig. 4, F and G). Grafting experiments between roots and shoots of pho1-7 and pho1-7 aos or pho1-7 coi1-1 showed that perturbation of JA biosynthesis only in the shoot was sufficient to recapitulate the shoot phenotypes of the pho1-7 aos and pho1-7 coi1-1 double mutants (Supplemental Fig. S2C). Together, these results indicate that induction of the JA pathway in the shoot of pho1 is partially responsible for several of the Pi starvation phenotypes associated with this mutant.
![Contribution of the JA pathway to the phenotype of Pi deficiency. A to E, Wild-type and mutant plants grown in fertilized soil for 4 weeks were compared for overall appearance (A), shoot fresh weight (B), shoot anthocyanin content (C), and the expression of the IPS1 (D) and MGD3 (E) genes in shoots. In A, the pots containing Col-0 and pho1-7 plants at left are the same as those shown at right. F and G, JA (F) and Ja-Ile (G) contents in rosette leaves from plants grown for 4 weeks in soil. H, The response of the primary root to low Pi was assessed by growing plants on agar-solidified medium containing 1 mm Pi for 7 d before transferring them to similar medium containing either 1 mm (gray bars) or 10 µm (black bars) Pi for 3 d. Growth of the primary root in the last 3 d was measured. I, Anthocyanin levels in shoots of plants grown for 7 d in agar-solidified medium containing 1 mm Pi followed by transfer to medium containing either 1 mm (gray bars) or 10 µm (black bars) Pi for 5 d. J, Fresh weight of the rosettes of Col-0, aos, and coi1-34 plants grown for 18 d in agar-solidified medium containing 1 mm, 100 µm, or 20 µm Pi. For B, data are means ± sd of 20 plants grown in individual pots. For C, data are means ± sd of five plants grown in independent pots. For D and E, data are means ± sd of three samples from plants grown in independent pots and three technical replicates for each sample, with each sample being a pool of three plants. For F and G, data are means ± sd of five samples from plants grown in independent pots, with each sample being an individual plant for Col-0, coi1-1, and aos and a pool of three plants for pho1-7, pho1-7 coi1-1, and pho1-7 aos. For H, data are means ± sd of 30 individual roots from three independent plates. For I and J, data are means ± sd of 20 to 30 plants grown in five independent plates. For B to G, values marked with lowercase letters were statistically significantly different from those for other groups marked with different letters (P < 0.05, ANOVA with the Tukey-Kramer honestly significant difference [HSD] test). n.d., Not detectable. For H to J, statistical analysis was done comparing Col-0 with the coi1-34 and aos mutants within each treatment, and asterisks denote statistical significance (*, P < 0.05 and **, P < 0.01) according to Student’s t test. Experiments were performed independently one time (F, G, and J), two times (B, H, and I), or three times (C–E) with similar results. FW, Fresh weight.](https://oup.silverchair-cdn.com/oup/backfile/Content_public/Journal/plphys/171/1/10.1104_pp.16.00278/2/m_plphys_v171_1_632_f4.jpeg?Expires=1750316508&Signature=NJs4H46-H~HmaKlSC-NpHeopG~o9sBNdrokAzEZEk2it5J3H7o60hUcd65mQAMtxFx2MS6IbdddeT3jA4pCuX~s6UBBEAmnc~vEmSBjSYg4Uv6EOhPhhd9musXwQljkkOwD0AkezO2O59bktMovkiDBZ6msSIEyFd9hgFStdwYKPlrjQ7vQU1~GoPNfb5lZ5L96KS~7uRA48Za9qT9utGL6wNmba2LATJ6s8sCyYABYBKbtapYaONjZk4pnl0lII7feXU8ltz-wPFZxoRcqsbv9cbkK-rOsMyXdAM4qlNbzmk9Q8~WUbZ7UKUSSVFBPRjaRL7vcdz-Tl7PSBtHOpfg__&Key-Pair-Id=APKAIE5G5CRDK6RD3PGA)
Contribution of the JA pathway to the phenotype of Pi deficiency. A to E, Wild-type and mutant plants grown in fertilized soil for 4 weeks were compared for overall appearance (A), shoot fresh weight (B), shoot anthocyanin content (C), and the expression of the IPS1 (D) and MGD3 (E) genes in shoots. In A, the pots containing Col-0 and pho1-7 plants at left are the same as those shown at right. F and G, JA (F) and Ja-Ile (G) contents in rosette leaves from plants grown for 4 weeks in soil. H, The response of the primary root to low Pi was assessed by growing plants on agar-solidified medium containing 1 mm Pi for 7 d before transferring them to similar medium containing either 1 mm (gray bars) or 10 µm (black bars) Pi for 3 d. Growth of the primary root in the last 3 d was measured. I, Anthocyanin levels in shoots of plants grown for 7 d in agar-solidified medium containing 1 mm Pi followed by transfer to medium containing either 1 mm (gray bars) or 10 µm (black bars) Pi for 5 d. J, Fresh weight of the rosettes of Col-0, aos, and coi1-34 plants grown for 18 d in agar-solidified medium containing 1 mm, 100 µm, or 20 µm Pi. For B, data are means ± sd of 20 plants grown in individual pots. For C, data are means ± sd of five plants grown in independent pots. For D and E, data are means ± sd of three samples from plants grown in independent pots and three technical replicates for each sample, with each sample being a pool of three plants. For F and G, data are means ± sd of five samples from plants grown in independent pots, with each sample being an individual plant for Col-0, coi1-1, and aos and a pool of three plants for pho1-7, pho1-7 coi1-1, and pho1-7 aos. For H, data are means ± sd of 30 individual roots from three independent plates. For I and J, data are means ± sd of 20 to 30 plants grown in five independent plates. For B to G, values marked with lowercase letters were statistically significantly different from those for other groups marked with different letters (P < 0.05, ANOVA with the Tukey-Kramer honestly significant difference [HSD] test). n.d., Not detectable. For H to J, statistical analysis was done comparing Col-0 with the coi1-34 and aos mutants within each treatment, and asterisks denote statistical significance (*, P < 0.05 and **, P < 0.01) according to Student’s t test. Experiments were performed independently one time (F, G, and J), two times (B, H, and I), or three times (C–E) with similar results. FW, Fresh weight.
While shoots of the pho1 mutant are Pi deficient, its roots are Pi sufficient (Poirier et al., 1991). Since soil Pi deficiency is first perceived by the root system, the effect of JA accumulation was examined in plants experiencing Pi deficiency in both roots and shoots. Pi deficiency leads to reduced growth of the primary root (Williamson et al., 2001; López-Bucio et al., 2002). Strong reduction of primary root elongation by Pi deficiency was essentially maintained in the aos and coi1-34 mutants, with only a small but statistically significant difference observed compared with Col-0 (Fig. 4H). At the shoot level, Pi-deficient aos and coi1-34 showed 2- to 3-fold reductions in anthocyanin accumulation relative to Col-0 (Fig. 4I). Shoot fresh weight of the Arabidopsis aos and coi1-34 mutants was approximately 15% to 30% higher compared with Col-0 plants, irrespective of whether plants were grown in high (1 mm) or low (100 or 20 µm) Pi (Fig. 4J).
Pi Deficiency Leads to Increased Resistance of Plants to Insect Herbivory
To investigate whether JA accumulation in pho1 shoots had an effect on herbivory, we exposed plants to the generalist insect larvae of S. littoralis. Only 30% of the caterpillars feeding on pho1-7 survived after 12 d, while the survival rate on Col-0, aos, and coi1-1, as well as the double mutants pho1-7 aos and pho1-7 coi1-1, was 90% to 100% (Fig. 5A). Surviving caterpillars feeding on pho1-7 showed a 3.5-fold reduction in fresh weight compared with insects feeding on Col-0 (Fig. 5B). As expected, caterpillars feeding on the JA-deficient aos and coi1-1 mutants had higher fresh weight compared with Col-0 (Fig. 5B; Schlaeppi et al., 2008). The higher resistance of pho1 to herbivory was greatly reduced in the pho1-7 aos and pho1-7 coi1-1 double mutants (Fig. 5B). However, caterpillars feeding on pho1-7 coi1-1 or pho1-7 aos double mutants showed a slightly reduced (1.4-fold) weight when compared with the parental controls coi1-1 or aos. These data indicate that activation of the JA pathway is an important determinant affecting S. littoralis survival rate and larvae weight in the pho1 mutant but that JA-independent factors also influence the weight of larvae feeding on pho1.
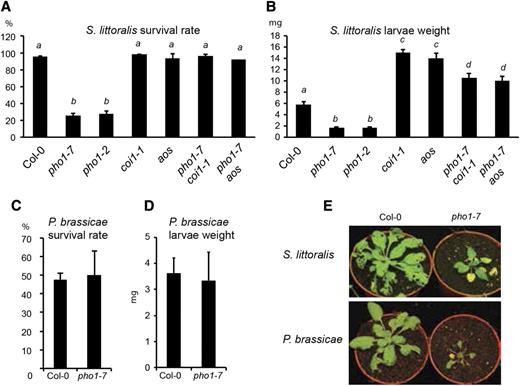
The pho1 mutant is resistant to the generalist herbivore S. littoralis but not the specialist herbivore P. brassicae. A and B, Survival rate (A) and larvae weight (B) of S. littoralis caterpillars after 12 d of feeding on 4-week-old plants grown in fertilized soil. C and D, Survival rate (C) and larvae weight (D) of P. brassicae caterpillars after 7 d of feeding on 4-week-old plants grown in fertilized soil. E, Representative images of Col-0 and pho1-7 plants after feeding on S. littoralis (top) and P. brassicae (bottom). Data are means ± sd of 20 to 30 caterpillars for A and B and 10 to 20 caterpillars for C and D feeding on individually grown plants. Values in A and B marked with lowercase letters were statistically significantly different from those for other groups marked with different letters (P < 0.05, ANOVA with the Tukey-Kramer HSD test). For C and D, Student’s t test was used. Experiments were performed independently two times (C and D) or three times (A and B) with similar results.
A bioassay was performed using the specialist herbivore caterpillars of Pieris brassicae (Reymond et al., 2004). Glucosinolates are the characteristic herbivory defense metabolites of plants of the Brassicaceae family, to which Arabidopsis belongs, and these metabolites are induced through the activity of the JA pathway (Mikkelsen et al., 2003). P. brassicae is equipped to detoxify glucosinolates and, thus, shows similar feeding behavior on Arabidopsis regardless of a plant’s JA status (Wittstock et al., 2004; Schlaeppi et al., 2008). P. brassicae caterpillars showed similar feeding behavior on pho1-7 and Col-0, with no significant difference observed in the fresh weight and survival rate of caterpillars feeding on these plants (Fig. 5, C–E).
The effect of Pi deficiency on S. littoralis herbivory was assessed in wild-type Arabidopsis as well as in two crop plants, N. benthamiana and tomato. For all plants, both caterpillar weight and survival rate were reduced considerably in plants grown under Pi-deficient conditions compared with plants grown on Pi-sufficient conditions (Fig. 6). These results show that shoot Pi deficiency leads to increased resistance to herbivory in a diversity of plants, including economically important crops.
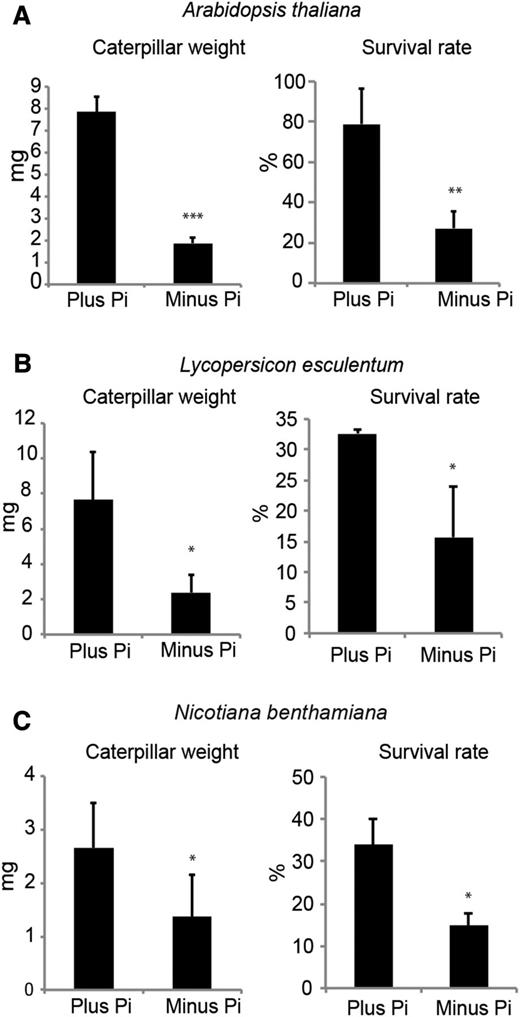
Long-term Pi deficiency induces resistance to S. littoralis herbivory. Plants were grown from seeds on a soilless aeroponic system supplemented with Hoagland nutrient solution containing either 1 mm Pi or no added Pi. Weight (left) and survival rate (right) of S. littoralis caterpillars were measured after either 12 d of feeding on 4-week-old Arabidopsis (A) or 12 d of feeding on 3-week-old tomato (B) or N. benthamiana (C). Data are means ± sd of 10 to 20 caterpillars feeding on individually grown plants. Student’s t test was performed, and asterisks denote statistical significance (*, P < 0.05; **, P < 0.01; and ***, P < 0.001). Experiments were performed independently two times (B and C) or three times (A) with similar results.
DISCUSSION
Several phytohormones play important roles in the adaptation of plants to Pi deficiency, with most studies focusing on changes in the root system architecture (RSA; Niu et al., 2013). Under Pi deficiency, primary root growth is inhibited, while the initiation and growth of both lateral roots and root hairs are stimulated. Changes in the synthesis, distribution, or sensitivity of the signaling pathway to various hormones are known to act on the RSA (Rubio et al., 2009). For example, Pi deficiency is associated with increased sensitivity to auxin via the overexpression of the auxin receptor TIR1 (Pérez-Torres et al., 2008), cytokinin synthesis and signaling via CRE1 are decreased (Franco-Zorrilla et al., 2002), and the synthesis of both brassinosteroids and gibberellins is decreased while the synthesis of ethylene and strigolactones is increased (Ma et al., 2003; Jiang et al., 2007; Mayzlish-Gati et al., 2012; Singh et al., 2014). This work demonstrates that Pi deficiency leads to an increase in the synthesis of JA/JA-Ile and to an activation of the JA signaling pathway in Arabidopsis. This was shown for both roots and shoots of wild-type plants grown in medium with low Pi and in shoots of the pho1 mutant that is chronically Pi deficient due to a block in the transfer of Pi from roots to shoots.
Although the absolute level in JA/JA-Ile present in Pi-deficient pho1 leaves remained relatively low compared with wounded plants (approximately 40- to 70-fold lower), pho1 had a robust hyperaccumulation of JA/JA-Ile following wounding compared with the wild type and an enhanced resistance to herbivory. This is analogous to defense priming, which is defined as enhanced activation of the defense response to insect or pathogen attack by a previous stimulation (Conrath et al., 2006; Frost et al., 2008). Priming of the herbivory defense response has been associated with distinct signaling cues, such as the release of volatiles from neighboring wounded plants (Frost et al., 2008) or the association of roots with mycorrhizal fungi (Jung et al., 2012).
This work shows that Pi-deficient plants have increased resistance to S. littoralis herbivory. This was shown for both the Pi-deficient pho1 mutant and Pi-deficient wild-type Arabidopsis, N. benthamiana, and tomato. The observation that the weights of larvae feeding on pho1-7 coi1-1 and pho1-7 aos double mutants are lower than those of larvae feeding on coi1-1 and aos single mutants shows the involvement of JA-independent factors affecting larvae weight on the pho1 mutant, indicating that the pathway(s) implicated in herbivory resistance in pho1 and coi1/aos may not be the same. However, the larger difference in larvae weight between pho1 and the wild type (3.5-fold) compared with coi1-1 or aos and the respective pho1-7 coi1-1 and pho1-7 aos mutants (1.4-fold) suggests that the increased resistance of pho1 to herbivory is impacted by increased JA levels. Considering that induction of the JA pathway following wounding provides a major contribution to the resistance of plants to numerous herbivorous insects, including S. littoralis (Acosta and Farmer, 2010), the fact that wounded pho1 plants accumulate 3- to 4-fold more JA-Ile and JA compared with wounded wild-type plants is likely to contribute to the impact of the pho1 mutation on herbivory resistance and larvae weight.
Activation of the JA pathway by Pi deficiency had effects on some traits associated with Pi deficiency. Thus, in pho1-7 plants, blocking JA biosynthesis or signaling via the aos or coi1-1 mutant, respectively, resulted in the elongation of petioles or the reduction of leaf anthocyanin production. At the biochemical level, changes in lipid composition typically triggered by Pi deficiency and observed in the pho1 mutant were not influenced by the JA pathway. Thus, the decrease in MGD3 expression observed in the pho1-7 coi1-1 and pho1-7 aos double mutants was not sufficient to impact membrane lipid composition. Previous analysis of gene expression in roots of the lpi4 mutant, which is insensitive to the inhibitory effect of Pi deficiency on primary root growth, revealed a down-regulation of several genes involved in JA biosynthesis, suggesting that JA may contribute to RSA (Chacón-López et al., 2011). Our data show that the expression of JAZ10 and VSP2 is robustly up-regulated in Pi-deficient root tips, indicating that the increase in JA and JA-Ile levels observed in Pi-deficient whole roots likely applies also to the root tip. Primary root elongation of the aos and coi1-34 mutants was still strongly reduced under Pi deficiency, with only a small but statistically significant difference compared with wild-type plants, indicating a marginal contribution of the JA pathway to this root phenotype. While blocking the JA pathway had a larger beneficial impact on pho1 shoot growth than on the wild type, it did not make the growth of pho1 comparable to that of Pi-sufficient wild-type plants. These results indicate that, while JA levels in pho1 negatively affect shoot growth, other factors associated with Pi deficiency have larger contributions to this phenotype. In contrast to pho1, blocking the JA pathway in plants experiencing Pi deficiency in both roots and shoots had only marginal effects on shoot growth. This may indicate that root-to-shoot long-distance signaling occurring in plants experiencing both root and shoot Pi deficiency may limit plant growth even in the absence of JA synthesis or perception. However, reduction of shoot anthocyanin still occurred in aos and coi1-1 plants with low root and shoot Pi. This effect is likely mediated, in part, by the interaction of the JAZ repressor with MYB75, a transcription factor activating the anthocyanin biosynthetic pathway (Qi et al., 2011), as well as through the interaction between the JAZ and DELLA proteins (Jiang et al., 2007; Hou et al., 2010).
The pathway linking Pi deficiency to JA induction remains to be elucidated. While the kinetics of the activation of the JA signaling pathway by Pi deficiency in the phr1 mutant was delayed, activation of the pathway was not abolished, indicating that other signaling components in addition to PHR1 are participating. Interestingly, Arabidopsis mutants in genes involved in inositol polyphosphate synthesis show alterations in the JA-mediated defense response to herbivorous insects (Mosblech et al., 2008, 2011; Laha et al., 2015). The inositol polyphosphates InsP5 and InsP8 are known to bind to the COI1-JAZ coreceptor complex and likely act as coactivators (Sheard et al., 2010; Laha et al., 2015). The ipk1-1 mutant deficient in InsP6 synthesis also is affected in the Pi signaling cascade (Stevensons-Paulik et al., 2005; Kuo et al., 2014). It is thus possible that Pi deficiency influences both Pi and JA signaling pathways via the modulation of the inositol polyphosphate pool. Unfortunately, no studies have specifically measured changes in the inositol polyphosphate pools in response to Pi deficiency in plants.
A few other examples of interactions between nutrients and the JA pathway have been described. The addition of silicon was shown to trigger priming of the JA-mediated herbivory defense in rice (Ye et al., 2013). Potassium deficiency in Arabidopsis increased the expression of several JA-responsive genes, enhanced the synthesis of several oxylipins in leaves, including JA, and was associated with reduced damage by thrips (Armengaud et al., 2004, 2010; Troufflard et al., 2010). Sulfur deficiency resulted in an enhanced expression of several genes involved in JA synthesis, and mutation affecting sulfur metabolism influenced basal JA levels (Hirai et al., 2003; Maruyama-Nakashita et al., 2003; Nikiforova et al., 2003; Rodríguez et al., 2010).
Insect herbivory and Pi deficiency are two stresses that individually have negative impacts on plant fitness and seed yield and that are prevalent in both natural plant ecosystems and agriculture. Furthermore, insect herbivory has been shown to impact ecological and evolutionary changes in plant populations, even over a few generations (Maron and Crone, 2006; Agrawal et al., 2012). As the JA-mediated wound response is implicated in the resistance to herbivory not only by insects but also by detritivorous crustaceans and vertebrate herbivores (Farmer and Dubugnon, 2009; Mafli et al., 2012), the activation of the JA pathway by Pi deficiency may have broad ecological and evolutionary consequence on plant populations. Our work provides an insight into how nutrient supply can impact the growth and defense of plants, an issue of global importance, since Pi-based fertilizers and plant pest protection are the two major inputs of modern-day agriculture.
MATERIALS AND METHODS
Plant Material and Growth Conditions
All Arabidopsis (Arabidopsis thaliana) plants used in this study, including mutants and transgenic plants, were in the Col-0 ecotype. The aos, coi1-1, coi1-34, and pho1-2 mutants were described previously (Feys et al., 1994; Hamburger et al., 2002; Park et al., 2002; Acosta et al., 2013). The gl1 mutation present in the original coi1-1 mutant (Feys et al., 1994) was removed by backcrossing and was used in all experiments (provided by Jane Glazebrook, University of Minnesota). The pho1-7 mutant is a transfer DNA mutant from the Salk collection and was obtained from the European Arabidopsis Stock Centre (http://arabidopsis.info/). For in vitro experiments, plants were grown in one-half-strength Murashige and Skoog (MS) salts (Duchefa) containing 1% (w/v) Suc and 0.7% (w/v) agar. For Pi-deficient medium, MS salts without Pi (Caisson) and purified agar (Conda) were used. Phosphate buffer at pH 5.7 (93.5% KH2PO4 and 6.5% K2HPO4) was used to adjust Pi concentration in medium. In the Pi-deficient medium, phosphate buffer was replaced by equimolar amounts of KCl. Growth chamber conditions were 22°C, 60% humidity, 100 μE m−2 s−1 white light, and a photoperiod of either 16 h of light/8 h of dark (long days) or 10 h of light/14 h of dark (short days).
An original pho1-7 coi1-1 double homozygous mutant was identified through genotyping for the pho1-7 and coi1-1 alleles and the corresponding wild-type alleles (a list of primers used in this work is found in Supplemental Table S1). Genotyping for coi1-1 includes sequencing of the PCR fragment to identify the point mutation. Since the pho1-7 coi1-1 double homozygous mutant is male sterile, it was crossed with pollen from fertile pho1-7/pho1-7 coi1-1/COI1 plants, resulting in the generation of a seed stock that is 50% pho1-7/pho1-7 coi1-1/COI1 and 50% pho1-7/pho1-7 coi1-1/coi1-1. In all experiments involving the pho1-7 coi1-1 double homozygous mutant, plants from this mixed seed stock were first grown and assayed for various parameters (herbivory test, growth parameters, anthocyanin quantification, etc.) and were subsequently genotyped after sampling to identify plants that were pho1-7 coi1-1 double homozygous.
Quantitative Reverse Transcription-PCR
Plant material was immediately frozen in liquid nitrogen and ground using a mortar and pestle. Approximately 100 to 150 mg of powder was used to extract the RNA using the RNeasy plant mini kit (Qiagen). Samples were treated with DNase on columns during RNA extraction using the RNase-free DNase kit (Qiagen). Approximately 500 ng of RNA per sample was used for reverse transcription using SuperScript II reverse transcriptase (Invitrogen). Quantitative PCR was performed using SYBR select master mix (Invitrogen) and Stratagene thermocycler Mx3005p. Primers used for quantitative PCR are described in Supplemental Table S1.
Anthocyanin and Lipid Quantification
Anthocyanin quantification was essentially performed as described previously (Mita et al., 1997). In brief, 25 µL of methanol containing 1% (v/v) HCl was added per 1 mg of leaf fresh weight. Leaves were then homogenized in the solution overnight at 4°C. Samples were centrifuged, and the absorbance of the supernatant was measured at 657 and 530 nm. Anthocyanin was calculated according to the formula A 530 − (0.25 × A 657), to compensate for the contribution of chlorophyll and its degradation products. Analysis of diacylglycerides was performed by the Kansas Lipidomics Research Center (www.k-state.edu/lipid/lipidomics/).
Insect Bioassays
Bioassays with Spodoptera littoralis and Pieris brassicae were performed as described previously (Bodenhausen and Reymond, 2007). In brief, plants were placed in cages fitted with windows on both sides or in nylon tents (Bugdorm; http://shop.bugdorm.com), and freshly hatched insects were placed directly on leaves. S. littoralis and P. brassicae caterpillars were left to feed for 12 and 7 d, respectively. Insects were weighed on the Mettler-Toledo MT5 precision balance. S. littoralis eggs were obtained from Syngenta, while P. brassicae were reared in house as described previously (Schlaeppi et al., 2008).
Pi Measurement
Pi measurement was performed as described previously (Rouached et al., 2011). In brief, 50 µL of water was added per 1 mg fresh weight of material. Material was frozen at −20°C and then thawed at ambient temperature before boiling at 80°C for 1 h. Supernatant was taken for Pi measurement after centrifugation. Pi measurement was done using the molybdate assay as described previously (Ames, 1966).
Hormone Quantification
JA and JA-Ile quantification was performed as described previously (Glauser et al., 2014). In brief, plant material was frozen in liquid nitrogen immediately after harvesting. Samples were then ground using a mortar and pestle, and approximately 100 mg of the powder was weighed using a precision balance (Mettler Toledo AG245). JA and JA-Ile were extracted in a mixture of ethylacetate:formic acid (99.5:0.5, v/v) containing d5-JA (1 ng) and [13C6]JA-Ile (1 ng) as internal standards. The solution was then evaporated to dryness, and the residue was reconstituted in methanol:water (70:30, v/v). Five microliters of extract was injected into an ultra-high-pressure liquid chromatography system coupled to a tandem mass spectrometer. Quantification was achieved using a five-point calibration curve ranging from 0.5 to 200 ng mL−1 JA and JA-Ile and containing 1 ng of labeled internal standards.
Root Assay
Seedlings were grown vertically in one-half-strength MS salts containing 1% (w/v) Suc and 0.7% (w/v) agar for 7 d. Seedlings were then transferred to plates containing one-half-strength MS salts with either 1 mm Pi medium (high Pi) or 10 µm Pi medium (low Pi). Root length after the transfer was measured 3 d later using ImageJ software.
Statistical Analysis
Data were generated from material grown in different Petri dishes and individual pots at different times and are reported as means with sd. Statistical significance was evaluated using either Student’s t test or ANOVA with the Tukey-Kramer HSD test. Experiments also were independently reproduced with a gap of several months, with consistent results, as indicated in the figure legends.
Supplemental Data
The following supplemental materials are available.
Supplemental Figure S1. The pho1 alleles.
Supplemental Figure S2. Contribution of the JA pathway to the phenotype of Pi deficiency.
Supplemental Figure S3. Lipid profile of leaves of plants grown in soil.
Supplemental Table S1. List of oligonucleotides.
ACKNOWLEDGMENTS
We thank Philippe Reymond and Edward Farmer for useful discussions and critical reading of the article, Christelle Bonnet and Steve Lassueur for help on caterpillar bioassays, and Javier Paz-Ares for providing seeds of the phr1 mutant.
Glossary
- JA
jasmonic acid
- JA-Ile
jasmonic acid-isoleucine
- Pi
inorganic phosphate
- Col-0
Columbia-0
- RSA
root system architecture
- MS
Murashige and Skoog
- HSD
honestly significant difference
LITERATURE CITED
Author notes
This work was supported by the Swiss National Foundation (grant nos. 31003A–138339 and 31003A–159998).
Address correspondence to [email protected].
The author responsible for distribution of materials integral to the findings presented in this article in accordance with the policy described in the Instructions for Authors (www.plantphysiol.org) is: Yves Poirier ([email protected]).
G.A.K. and Y.P. designed experiments and wrote the article, and Y.P. supervised the whole project; G.A.K. performed all experiments, except grafting experiments, which were performed by E.V.; G.G. provided technical and analytical support for jasmonic acid measurements.
Articles can be viewed without a subscription.