-
PDF
- Split View
-
Views
-
Cite
Cite
G. Alex Mason, Tzitziki Lemus, Christine Queitsch, The Mechanistic Underpinnings of an ago1-Mediated, Environmentally Dependent, and Stochastic Phenotype , Plant Physiology, Volume 170, Issue 4, April 2016, Pages 2420–2431, https://doi.org/10.1104/pp.15.01928
- Share Icon Share
Abstract
The crucial role of microRNAs in plant development is exceedingly well supported; their importance in environmental robustness is studied in less detail. Here, we describe a novel, environmentally dependent phenotype in hypomorphic argonaute1 (ago1) mutants and uncover its mechanistic underpinnings in Arabidopsis (Arabidopsis thaliana). AGO1 is a key player in microRNA-mediated gene regulation. We observed transparent lesions on embryonic leaves of ago1 mutant seedlings. These lesions increased in frequency in full-spectrum light. Notably, the lesion phenotype was most environmentally responsive in ago1-27 mutants. This allele is thought to primarily affect translational repression, which has been linked with the response to environmental perturbation. Using several lines of evidence, we found that these lesions represent dead and dying tissues due to an aberrant hypersensitive response. Although all three canonical defense hormone pathways (salicylic acid, jasmonate, and jasmonate/ethylene pathways) were up-regulated in ago1 mutants, we demonstrate that jasmonate perception drives the lesion phenotype. Double mutants of ago1 and coronatine insensitive1, the jasmonate receptor, showed greatly decreased frequency of affected seedlings. The chaperone HEAT SHOCK PROTEIN 90 (HSP90), which maintains phenotypic robustness in the face of environmental perturbations, is known to facilitate AGO1 function. HSP90 perturbation has been shown previously to up-regulate jasmonate signaling and to increase plant resistance to herbivory. Although single HSP90 mutants showed subtly elevated levels of lesions, double mutant analysis disagreed with a simple epistatic model for HSP90 and AGO1 interaction; rather, both appeared to act nonadditively in producing lesions. In summary, our study identifies AGO1 as a major, largely HSP90-independent, factor in providing environmental robustness to plants.
ARGONAUTE1 (AGO1) is an ancient, highly conserved essential protein. One of 10 functionally distinct AGO proteins in the plant Arabidopsis (Arabidopsis thaliana), AGO1 uses microRNAs (miRNAs) to decrease the expression of target genes. AGO1 and the miRNA pathway are crucial for many regulatory processes throughout development and in response to environmental stimuli. As frequent components of feed-forward loops, miRNAs also play important roles in controlling gene expression noise and maintaining the phenotypic robustness of specific traits in several organisms, including plants (Lempe et al., 2013).
AGO1 and miRNAs also are critical for proper plant responses to abiotic stress. The expression levels of miRNAs respond to heat shock, cold, drought, oxidative stress, UV light exposure, and hypoxia (Sunkar et al., 2012). For example, in response to drought, the expression of miR169 decreases and the expression of its target gene, encoding the transcription factor NFYA5, increases in Arabidopsis (Li et al., 2008). Increased expression of NFYA5 supports plant survival in drought conditions. Transgenic plants overexpressing NYFA5 have drought-tolerant phenotypes; in contrast, plants overexpressing miR169 are drought sensitive (Li et al., 2008).
In addition to orchestrating proper responses to abiotic stress, miRNAs also are implicated in response to biotic factors such as defense against pathogens and herbivores (Pandey et al., 2008; Várallyay et al., 2010; Zhang et al., 2011; Bozorov et al., 2012). There is abundant evidence from expression studies (Navarro et al., 2006; Pandey et al., 2008; Bozorov et al., 2012) that links miRNA expression to disease resistance pathways. For example, MIR393 is up-regulated in response to bacterial flg22, a potent trigger of innate immunity in plants (Nürnberger and Brunner, 2002). miR393 represses the expression of auxin receptor genes, which are involved in plant growth and development (Navarro et al., 2006).
A highly effective plant defense mechanism is the hypersensitive response (HR), which restricts the growth of some pathogens through programmed cell death (Zurbriggen et al., 2010). Pathogen perception triggers complex multifaceted responses, including altered ion fluxes, the initiation of mitogen-activated protein kinase signaling cascades, the accumulation of reactive oxygen species (ROS), the induction of downstream defense genes, and callose deposition at the cell wall, all of which are thought to contain pathogen growth (Kim et al., 2005; Chisholm et al., 2006; Boller and Felix, 2009; Clay et al., 2009). The phytohormones salicylic acid (SA), jasmonate (JA), and ethylene (ET) are of particular importance in HR and are critical for defense against pathogens and/or herbivores (Broekaert et al., 2006; Robert-Seilaniantz et al., 2011). The JA and ET pathways contain several well-characterized miRNA targets (Schommer et al., 2008; Kim et al., 2009). All three hormone pathways interact in a complex manner to coordinate plant defenses in the face of constantly changing environmental challenges in nature (Robert-Seilaniantz et al., 2011).
SA plays a central role in resistance against biotrophic pathogens, such as Pseudomonas syringae and Turnip crinkle virus (Koornneef and Pieterse, 2008). SA and ROS can act synergistically to lead to HR-mediated localized cell death (Shirasu et al., 1997; Coll et al., 2011). JA regulates many processes ranging from plant development (e.g. fertility) to defense against herbivores (Turner et al., 2002; Wasternack, 2007; Balbi and Devoto, 2008; Koo and Howe, 2009; Memelink, 2009; Gfeller et al., 2010). JA modulates ROS production (Overmyer et al., 2000), specifically the production of hydrogen peroxide (Orozco-Cárdenas et al., 2001). JA and SA are often thought to act antagonistically; however, the cross talk between these two hormones is complex (Robert-Seilaniantz et al., 2011).
ET is a positive regulator of ripening and senescence (Bleecker and Kende, 2000). In plant defense, ET potentiates some branches of SA-responsive gene expression (De Vos et al., 2006) but globally represses SA-responsive genes (Chen et al., 2009). ET and JA synergistically promote defense against necrotrophic fungal pathogens through ethylene-responsive transcription factors (ERFs; Lorenzo et al., 2003). However, ET represses JA-dependent herbivory responses (Ballaré, 2011) by antagonizing the transcription factor MYC2 (Anderson et al., 2004; Lorenzo et al., 2004; Song et al., 2014).
Here, we provide evidence that AGO1 maintains phenotypic robustness in the face of an environmental challenge and integrates environmental signals through these canonical defense pathways. We show that ago1 mutant plants develop lesions on embryonic leaves (cotyledons) when grown under full-spectrum light conditions. We demonstrate that these lesions represent localized cell death and originate due to stochastically occurring, aberrant HR. We show that SA, JA, and JA/ET signaling pathways all are significantly up-regulated in full-spectrum light-grown ago1 cotyledons and use genetic analysis to demonstrate that lesions arise primarily due to the JA-dependent pathway.
We also test the interaction of AGO1 with the molecular chaperone HEAT SHOCK PROTEIN 90 (HSP90), which is known to provide robustness to environmental perturbations in plants. HSP90 plays crucial roles in response to abiotic and biotic factors such as herbivores and pathogens (Lu et al., 2003; Liu et al., 2004; Sangster et al., 2007). Moreover, HSP90 facilitates AGO1 folding and function in a wide range of organisms, including plants (Iki et al., 2010; Iwasaki et al., 2010; Johnston et al., 2010). Consistent with HSP90’s well-established role in altering the penetrance of genetic variation (Sangster et al., 2008a, 2008b; Casanueva et al., 2012; Queitsch et al., 2012), we find that decreased HSP90 levels increase the penetrance of ago1 mutations. Our results indicate that AGO1 and HSP90 act together in maintaining plant robustness and buffering development from environmental perturbations.
RESULTS
ago1 Mutant Seedlings Develop Lesions in Full-Spectrum Light Conditions
In addition to previously characterized ago1 phenotypes (Bohmert et al., 1998; Morel et al., 2002; Baumberger and Baulcombe, 2005; Yang et al., 2006), such as hyponastic cotyledons and developmental delay (Fig. 1), we observed that ago1 mutant seedlings frequently produced white, often raised, lesions that resided primarily on cotyledons (Fig. 1). Wild-type Columbia-0 (Col-0) seedlings showed these lesions at low frequency in both filtered light (yellow long-pass filters, blocking less than 454 nm; Stasinopoulos and Hangarter, 1990) and full-spectrum light (Fig. 1). We grew an allelic series of ago1 mutations in both light conditions and scored the frequency of affected seedlings (Fig. 1). We scored affected seedlings rather than the frequency of lesions because lesions often fused with each other, confounding frequency measures. For the ago1-25, ago1-27, and ago1-46 mutant seedlings (Morel et al., 2002; Smith et al., 2009), the frequency of affected seedlings increased significantly in full-spectrum light compared with the wild type (Fig. 1). We selected the ago1-27 mutant for subsequent investigation of the molecular underpinnings of the lesion phenotype for two reasons. First, we were intrigued by the strong dependency of its lesion phenotype on growth conditions (generalized linear mixed model [GLMM], P < 1 × 10−4). Second, ago1-27 mutants, albeit carrying a stronger allele than ago1-46 mutants, show fewer and less severe unrelated developmental abnormalities than ago1-25 mutant seedlings (Morel et al., 2002).
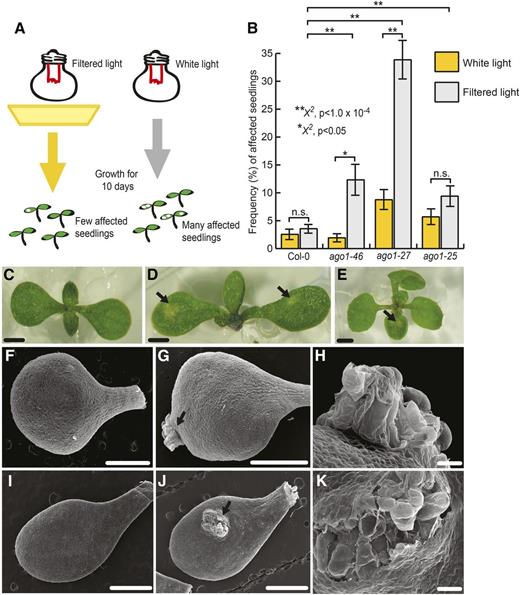
Hypomorphic ago1 mutants showed increased frequency of seemingly stochastic lesions. A, Experimental setup. When grown in yellow, filtered light conditions for 10 d, few ago1 seedlings showed lesions on cotyledons. When grown in full-spectrum light, many more ago1 seedlings developed lesions. B, In full-spectrum light, ago1-46, ago1-27, and ago1-25 mutants showed a significantly increased frequency of affected seedlings compared with the wild type. ago1-27 and ago1-46 mutants showed significantly more affected individuals in unfiltered versus filtered light conditions; a similar, albeit nonsignificant, tendency was observed for seedlings carrying the ago1-25 allele. Error bars indicate se across at least three replicates with n = 25 to 72 seedlings per replicate. Statistical significance was calculated using the χ2 test of significance (**, P < 1 × 10−4 and *, P < 0.05; for all possible P values, see Supplemental Table S1). n.s., Not significant. C to E, Lesions in wild-type and ago1-27 mutant seedlings show distinct morphology. C, Full-spectrum light-grown, 10-d-old, unaffected ago1-27 seedling. D, Full-spectrum light-grown, 10-d-old, affected ago1-27 seedling with lesions denoted by arrows. E, Full-spectrum light-grown, 10-d-old, affected wild-type seedling with lesion denoted by the arrow. Bars = 1 mm. F to K, Scanning electron micrographs of 10-d-old cotyledons grown in full-spectrum light. F, Unaffected wild-type cotyledon. G, Wild-type cotyledon with lesion denoted by the arrow. H, Magnified view of the lesion from G. I, Unaffected ago1-27 cotyledon. J, ago1-27 cotyledon with lesion denoted by the arrow. K, Magnified view of the lesion from I. Bars = 1 mm (F, G, I, and J) and 500 μm (H and K).
The raised, transparent morphology of the ago1-dependent lesions superficially resembled undifferentiated tissue (i.e. ectopic callus tissue; Boutilier et al., 2002). Notably, previous studies described callus-like tissue on cotyledons of hypomorphic serrate mutant plants without experimental follow-up (Grigg et al., 2005). The zinc-finger protein SERRATE is involved in primary miRNA biogenesis; serrate mutants show pleiotropic phenotypes due to perturbed meristem function and leaf axial patterning (Grigg et al., 2005).
We tested the callus hypothesis by analyzing the expression of genes implicated in callus formation (Sugimoto et al., 2010) and by testing the propensity of lesions to facilitate callus formation (Weigel and Glazebrook, 2002). However, genes implicated in callus formation and shoot apical meristem identity showed wild-type expression levels in ago1-27 cotyledons (Supplemental Fig. S1). To test callus formation, we dissected lesions from both ago1-27 and the wild type and tested callus formation on hormone-containing medium. At low hormone dose, ago1-27 lesion tissue performed worse than wild-type or ago1-27 unaffected tissue; at high hormone dose, no difference was observed (Supplemental Fig. S2). We conclude that the observed lesions do not represent undifferentiated, callus-like tissue.
To explore the origin and identity of the lesion tissue, we employed scanning electron microscopy (SEM; Fig. 1). SEM revealed that both wild-type and ago1-27 lesions erupt from beneath the epidermis, suggesting that mesophyll cells are primarily affected (Fig. 1). Cells within lesions appeared to be ruptured, suggesting that at least some lesions represented dead or dying tissue (van Doorn et al., 2011).
To exclude the possibility that ruptured cells were an artifact of SEM sample preparation, we used vital dye staining to validate our findings. Localized cell death is induced in plants as part of the HR upon pathogen attack. We hypothesized that the ago1 lesions were a result of stochastically occurring, aberrant HR that was enhanced in ago1 seedlings by exposure to full-spectrum light. Localized cell death is examined by staining with vital dyes such as Trypan Blue, which stains dead and dying tissue dark blue (Weigel and Glazebrook, 2002). We performed Trypan Blue staining of unaffected and affected cotyledon tissue (Fig. 2). Cotyledons exhibiting lesions retained the dye, with lesions exhibiting dark blue staining indicative of ruptured cell walls (Fig. 2). In fact, even unaffected ago1-27 cotyledons showed deeper staining than wild-type cotyledons, suggesting increased susceptibility to cell wall rupture. To confirm these results, we performed 3,3′-diaminobenzidine (DAB) staining (Fig. 2). DAB staining indicates the presence of ROS, specifically hydrogen peroxide, which is a reliable marker of HR-mediated localized cell death (Weigel and Glazebrook, 2002). Indeed, we observed DAB staining coinciding with lesions (Fig. 2). Based on both Trypan Blue and DAB staining, we concluded that ago1-27 cotyledons show lesions that resemble HR-mediated localized cell death.
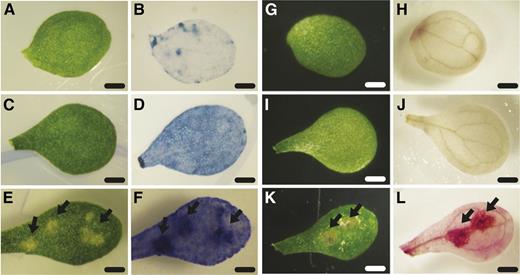
Vital staining of unaffected and affected tissue from wild-type and ago1-27 cotyledons. A and G, Unaffected wild-type cotyledons prior to staining. B, Unaffected wild-type cotyledon after Trypan Blue staining showed little dye accumulation. H, Unaffected wild-type cotyledon after DAB staining showed little dye polymerization. C and I, Unaffected ago1-27 cotyledons prior to staining. D, Unaffected ago1-27 cotyledon after Trypan Blue staining showed some dye accumulation. J, Unaffected ago1-27 cotyledon after DAB staining showed no dye polymerization. E and K, Affected ago1-27 cotyledons prior to staining. F, Affected ago1-27 cotyledon after Trypan Blue staining showed strong accumulation of dye in lesions (arrows). L, Affected ago1-27 cotyledon after DAB staining showed strong dye polymerization in lesions (arrows). Bars = 1 mm.
ago1-27 Cotyledons Overexpress Canonical SA, JA, and JA/ET Signaling Markers in Full-Spectrum Light
Phytohormones mediate all major aspects of a plant’s response to environmental perturbations, including defense against biotic stresses. The three phytohormones SA, ET, and JA are the canonical defense hormones. As previous results link light conditions (UV-B) with defense hormone response (A-H-Mackerness et al., 1999; Mazza et al., 1999), we set out to examine whether the SA, JA, and JA/ET pathways were responsible for the lesions on ago1 cotyledons. We measured the expression of marker genes for SA, JA, or JA/ET signaling in 10-d-old cotyledons of wild-type, ago1-46, ago1-27, and ago1-25 mutant seedlings grown in the lesion-inducing, full-spectrum light condition. Specifically, we assessed the expression of PATHOGENESIS-RELATED GENE1 (PR1), VEGETATIVE STORAGE PROTEIN1 (VSP1), and PLANT DEFENSIN1.2 (PDF1.2), which are up-regulated in defense-triggered SA, JA, and JA/ET signaling, respectively (Benedetti et al., 1995; Boter et al., 2004; Lorenzo et al., 2004; Mur et al., 2006; van Loon et al., 2006; Memelink, 2009). All three marker genes were significantly up-regulated in ago1 cotyledons compared with the wild type (Fig. 3); hence, more detailed analysis was required to identify the involved pathway(s).
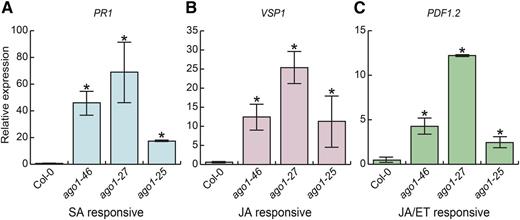
Marker genes of all three canonical defense hormone pathways were up-regulated in ago1 cotyledons. PR1 (SA responsive; A), VSP1 (JA responsive; B), and PDF1.2 (JA/ET responsive; C) expression were dramatically up-regulated in 10-d-old ago1-46, ago1-27, and ago1-25 cotyledons in full-spectrum light. Expression was measured by quantitative reverse transcription (RT)-PCR; all assayed genes were normalized to UBC21 (UBIQUITIN-CONJUGATING ENZYME 21) expression. Error bars indicate se across at least two replicates of cotyledons harvested from 18 pooled seedlings.
ago1 plants are susceptible to virus infection, implying a direct link between SA-mediated defense and AGO1 activity (Morel et al., 2002; Ruiz-Ferrer and Voinnet, 2009). As the SA marker gene PR1 was most strongly up-regulated in ago1 cotyledons, we first examined the expression of additional genes in SA signaling. Although there are no known miRNA targets in the SA signaling pathway, altered light conditions change the expression of many miRNAs (Zhou et al., 2007). Based on previous studies, we selected three SA signaling genes. The first, NONEXPRESSOR OF PATHOGENESIS-RELATED GENES1 (NPR1), was selected because it contributes to the activation of PR genes after SA perception (Mukhtar et al., 2009). NPR1 is also a key modulator in SA-JA cross talk (Ballaré, 2011). The second, SUPPRESSOR OF NPR1-1 (SNC1), was reported previously to be up-regulated in ago1-36 mutants (Yi and Richards, 2007). The third, ENHANCED DISEASE SUSCEPTIBILITY1 (EDS1), is a component of R gene-mediated disease resistance in Arabidopsis (Falk et al., 1999); EDS1 also is required for the production of SA in some conditions involving pathogen challenge (Rustérucci et al., 2001). All three genes were significantly up-regulated in ago1-27 cotyledons (Fig. 4).
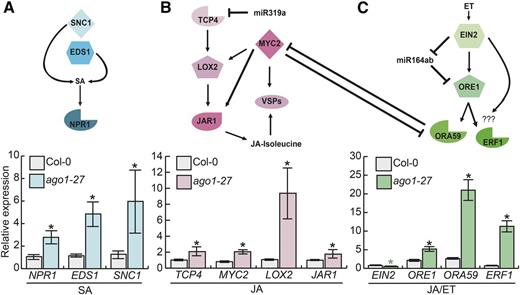
Genes in all three defense hormone pathways, including miRNA target genes, were up-regulated in ago1-27 cotyledons. A, Top, pathway diagram indicates the pathway connection of three tested SA genes, none of which are miRNA targets. Bottom, NPR1, EDS1, and SNC1 were up-regulated in ago1-27 seedlings relative to the wild type. B, Top, pathway diagram indicates the pathway connection of four tested JA genes, the most upstream of which, TCP4, is an miRNA target gene. Bottom, the canonical jasmonate signaling and biosynthesis genes TCP4, LOX2, MYC2, VSP2, and JAR1 were strongly up-regulated. C, Top, pathway diagram indicates the pathway connection of four tested JA/ET genes. The most upstream gene, EIN2, acts in an incoherent feed-forward loop with mir164ab and its target gene ORE1. ET and JA pathways show cross-regulation via ORA59 and MYC2. Bottom, the JA/ET markers ORE1, ORA59, and ERF1 were significantly up-regulated in ago1-27 cotyledons relative to the wild type; in contrast, EIN2 was significantly down-regulated in ago1-27 cotyledons. All assayed genes are normalized to UBC21 (UBIQUITIN-CONJUGATING ENZYME 21) expression. Error bars indicate se across three to four replicates of cotyledons harvested from 18 pooled seedlings. Black asterisks represent significant up-regulation in ago1-27 cotyledons; the gray asterisk (EIN2) represents significant down-regulation in ago1-27 cotyledons.
We next investigated additional marker genes in JA signaling. In Arabidopsis, miR319a represses specific members of the TEOSINTE BRANCHED/CYCLOIDEA/PCF (TCP) family of transcription factors, which positively regulate JA biosynthesis (Danisman et al., 2012). TCP4 promotes JA biosynthesis by activating the expression of LIPOXYGENASE2 (LOX2; Schommer et al., 2008; Danisman et al., 2012). JASMONATE INSENSITIVE1/MYC2, a master regulator of the response to herbivores, also activates the expression of LOX genes (Boter et al., 2004; Lorenzo et al., 2004). TCP4-regulated LOX2 catalyzes an early step of JA biosynthesis (Gfeller et al., 2010). Lastly, JASMONATE RESISTANT1 (JAR1) functions in the synthesis of biologically active JA-Ile (Guranowski et al., 2007). All four tested genes (TCP4, LOX2, MYC2, and JAR1) were significantly up-regulated in ago1-27 cotyledons (Fig. 4).
Lastly, we examined additional genes known to function in JA/ET signaling. We selected ETHLYLENE INSENSITIVE2 (EIN2) because it is the key integrator of JA/ET stress responses (Alonso et al., 1999) and is required for PDF1.2 expression (Alonso et al., 1999). We also examined the transcription factor ORESARA1 (ORE1), which is a direct target of miR164ab and which, together with EIN2, regulates ET signaling via a trifurcate feed-forward loop (Kim et al., 2009). JA and ET also activate the expression of AP2/ERF-domain transcription factors (Nakano et al., 2006) such as OCTADECANOID-RESPONSIVE ARABIDOPSIS AP2/ERF59 (ORA59) and ERF1 (Lorenzo et al., 2003; Pré et al., 2008). Overexpression of ERF1 (Berrocal-Lobo et al., 2002) or ORA59 (Pré et al., 2008) enhances resistance against fungal pathogens. Overexpression of ORA59 also suppresses MYC-dependent signaling in the defense against herbivores, making plants more susceptible to herbivores (Verhage et al., 2011).
All but one gene were significantly up-regulated in ago1-27 cotyledons (Fig. 4). The outlier, EIN2, was significantly down-regulated at about half of wild-type levels. Given the strong up-regulation of PDF1.2, this specific result is likely a consequence of extensive feedback relationships. In summary, all three stress response pathways were up-regulated, suggesting that ago1 mutants no longer interpret environmental signals correctly and may be overly sensitive to the subtle stress of full-spectrum light (Stasinopoulos and Hangarter, 1990).
Exogenous JA and ET Treatments, But Not SA Treatment, Suffice to Induce Lesions in the Wild Type
The consistent up-regulation of all three candidate pathways necessitated a different approach to identify the molecular origin(s) of the lesions in ago1 cotyledons. We applied all three phytohormones separately and in combination and assayed the frequency of affected ago1-27 and wild-type seedlings. Specifically, we sprayed 5-d-old seedlings grown in full-spectrum light with increasing concentrations of phytohormones (Genoud et al., 2002; Weigel and Glazebrook, 2002; Sangster et al., 2007) and scored affected seedlings at day 10. As SA signaling marker genes were up-regulated, we predicted that exogenous SA treatment would increase the number of affected individuals in both ago1-27 and the wild type. However, the frequency of affected ago1-27 seedlings decreased; there was no significant response in the wild type (Fig. 5). This result excludes SA as the cause of the observed lesions.
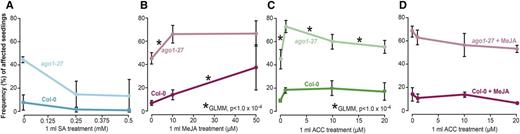
Methyl jasmonate (MeJA) treatment was sufficient to induce lesions. A, Under high-SA conditions, fewer seedlings were affected for both the wild type and ago1-27 in full-spectrum light. B, At 10 μm MeJA, ago1-27 was significantly more responsive compared with the wild type (GLMM, P < 1 × 10−4), whereas at the 50 μm MeJA treatment, the wild type was more responsive (GLMM, P < 1 × 10−4). C, At 1 μm ACC (ET precursor molecule), ago1-27 was more responsive than the wild type (GLMM, P < 1 × 10−4), reaching a frequency of affected seedlings similar to ago1-27 seedlings at 10 μm MeJA. At higher doses, ACC repressed the lesion phenotype in ago1-27 (GLMM, P < 1 × 10−4) and had no further effect on the wild type. D, Combining 10 μm MeJA with varying ACC concentrations yielded no significant change, albeit the frequencies of affected seedlings declined. n = 72 to 144 individuals per replicate, with two to five replicates each. The difference in responsiveness between genotypes was modeled using GLMM, testing for interaction between genotype and treatment, with replicate and number of seedlings set as random variables (*, P < 1 × 10−4; for all possible P values, see Supplemental Table S4).
We next tested whether the application of MeJA would increase the frequency of affected seedlings and whether we could detect differences in the responses of ago1-27 and the wild type. In the wild type, the frequency of affected individuals increased dramatically at 50 μm MeJA. Thus, JA treatment suffices to cause lesions (Fig. 5). In ago1-27 seedlings, the number of affected individuals increased significantly at low MeJA dosage (10 μm) but did not increase further at high MeJA dosage (50 μm). This result suggests that ago1-27 is hypersensitive to JA at low concentrations but its response plateaus at higher concentrations (Fig. 5). We compared the responses of the wild type and ago1-27 to MeJA using a generalized linear model with genotype and treatment as fixed effects and replicate as a random effect. Indeed, ago1-27 mutants were significantly more responsive to MeJA at 10 μm (GLMM, P < 1 × 10−4), whereas at 50 μm, the wild type was more responsive (GLMM, P < 1 × 10−4), indicating that ago1-27 mutants have a lower threshold for JA perception and saturation.
Lastly, we tested whether ET treatment could increase the number of affected seedlings using the ET precursor 1-aminocyclopropane-1-carboxylic acid (ACC; Fig. 5). At the lowest dose of ACC (1 μm), the frequency of affected ago1-27 seedlings increased sharply. Comparing ago1-27 and the wild-type response to ACC, we found that ago1-27 mutants were significantly more responsive at 1 μm (GLMM, P < 1 × 10−4). However, with increasing ACC doses, the frequency of affected seedlings decreased subtly but significantly in ago1-27 and remained unchanged in the wild type (GLMM, P < 1 × 10−4). Thus, ET treatment does increase the number of affected ago1-27 individuals at low doses and decreases the frequency of affected ago1-27 seedlings at high doses, but it fails to fully mimic the mutant phenotype in the wild type.
As both JA and ET treatment produced increases in affected seedlings, we wanted to test the effect of both hormones in combination. We performed these experiments with 10 μm MeJA, the highly responsive dose for ago1-27 mutant seedlings, and at various concentrations of ACC. The presence of ACC did not significantly change the frequency of affected ago1-27 and wild-type individuals observed at 10 μm MeJA (Fig. 5). Thus, we conclude that JA, but not ET or SA, is likely the main driver of the environmentally sensitive lesion phenotype in ago1-27.
Disruption of JA Perception Dramatically Reduces Lesions in ago1-27 Mutant Seedlings
Thus far, our expression results and exogenous hormone treatments point to JA as the key hormone underlying the lesion phenotype in ago1-27 mutant seedlings. To test whether JA causes the lesion phenotype, we created a double mutant of ago1-27 and coronatine insensitive1 (coi1-1) to block JA perception. COI1 encodes the main receptor of biologically active JA; the well-characterized coi1-1 mutant carries an amino acid substitution in the F-box motif of COI1 (Feys et al., 1994; Yan et al., 2009). The coi1-1 homozygous seedlings showed significantly fewer individuals with lesions than the wild type (Fig. 6). The frequency of affected seedlings in the ago1-27;coi1-1 double mutant was dramatically reduced compared with the ago1-27 single mutant, albeit higher than in the wild type (Fig. 6). The disruption of JA perception and signaling appears to strongly suppress the environmentally sensitive lesions in both ago1-27 and the wild type.
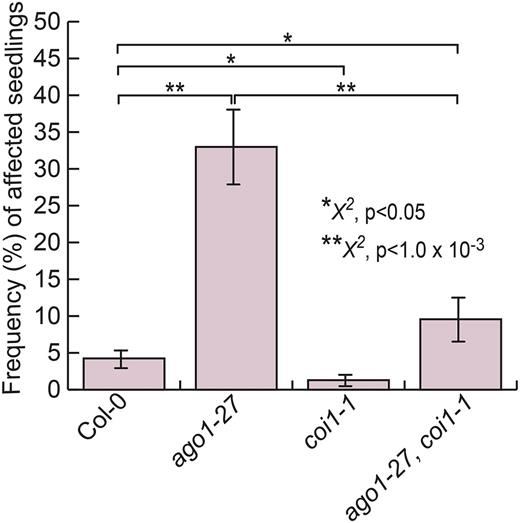
Disruption of JA perception suppresses lesion phenotype. coi1-1 mutant seedlings showed significantly fewer affected seedlings than the wild type (P < 0.05). ago1-27;coi1-1 double mutant seedlings showed dramatically fewer affected seedlings than ago1-27 single mutant seedlings (P < 1 × 10−3); however, the frequency of affected seedlings was significantly higher than in the wild type (P < 0.05). Statistical significance was calculated using the χ2 test of significance (**, P < 1 × 10−3 and *, P < 0.05; for all reported P values, see Supplemental Table S4). n = 72 to 144 individuals per replicate, with four replicates each. Error bars represent se across biological replicates.
To explore the possible interplay of JA and ET perception and signaling genetically, we created triple mutants of ago1-27 with coi1-1 and ein2-1. EIN2 is a membrane-bound transport protein; ein2-1 mutants carry an early stop mutation resulting in a truncated protein (Alonso et al., 1999). Consistent with our expression data for EIN2 in ago1-27 mutant seedlings, the ein2-1 homozygous seedlings produced significantly more lesions than the wild type and were statistically similar to ago1-27 mutant seedlings (Supplemental Fig. S3). Thus, loss of ET perception increases rather than decreases the lesion phenotype. Double mutants of ago1-27 and ein2-1 resembled ago1-27 single mutants (Supplemental Fig. S3), suggesting that lesions in both mutant genotypes arise through the same pathway. The coi1-1 mutation was epistatic in both the double mutant with ein2-1 and the triple mutant ago1-27;coi1-1;ein2-1 (Supplemental Fig. S3), identifying JA perception as causal for lesions in single ago1-27 and ein2 mutants. We conclude that the observed environmentally sensitive, ago1-27-dependent lesions are primarily driven by JA and that the effects of exogenous ET are likely due to the known cross-regulation of both pathways.
AGO1 and HSP90 Interact Genetically in Producing the Lesion Phenotype
Recent studies in both animals (Iwasaki et al., 2010; Johnston et al., 2010) and plants (Iki et al., 2010) demonstrate that the molecular chaperone HSP90 is required for different aspects of AGO function. HSP90-reduced plants show an overexpression of genes involved in JA biosynthesis and are more resistant to herbivores than wild-type plants (Sangster et al., 2007). Therefore, we wanted to explore if the observed environmentally and JA-dependent lesion phenotype was affected by perturbing HSP90. To do so, we created a double mutant of ago1-27 with a previously published RNA interference (RNAi) line, RNAi-A1 (Sangster et al., 2007). This line primarily affects levels of the environmentally responsive, heat-inducible HSP90.1 paralog, which could be plausibly involved in the environmentally dependent lesion phenotype. We refrained from using highly specific inhibitors of the chaperone, as these compounds are highly sensitive to full-spectrum light (Queitsch et al., 2002). Indeed, we observed a subtle but significant increase in affected seedlings in single HSP90 mutants (HSP90 RNAi-A1) compared with wild-type seedlings (Fig. 7). This result is consistent with the previously described role of HSP90 in JA signaling (Sangster et al., 2007). However, HSP90 perturbation produced far fewer affected seedlings than AGO1 perturbation, inconsistent with the simple epistasis of both genes. Assuming that the HSP90 RNAi-A1 lesion phenotype arises as the result of an AGO1-HSP90 interaction, there should be no further increase in the frequency of affected seedlings in the double mutant. If, however, HSP90 and AGO1 act independently, we should observe additive effects. These simple assumptions are complicated by the fact that both ago1-27 and HSP90 RNAi-A1 are weak, partial loss-of-function mutants. Our result deviated subtly but significantly from additive expectations (GLMM, P < 1 × 10−4; Fig. 7), suggesting a nonadditive relationship between the chaperone and AGO1 in this phenotype. Nonadditivity is supported by our expression analysis of HSP90 across all three mutant backgrounds (Supplemental Fig. S4). In full-spectrum light conditions, HSP90 RNAi-A1 mutants showed strong down-regulation of the chaperone and significantly more seedlings with lesions than the wild type; the ago1-27 mutants showed significant up-regulation of HSP90 compared with the wild type, consistent with experiencing stress, and many more affected seedlings than both the wild type and single HSP90 mutants; finally, the double mutant showed reduced HSP90 levels compared with the wild type, albeit to a lesser extent than in the single HSP90 RNAi-A1 mutants, and the highest frequency of seedlings with lesions. The nonadditive higher incidence of affected seedlings in this double mutant is consistent with previous observations that HSP90 perturbation increases the penetrance of genetic variants (Queitsch et al., 2012; Lempe et al., 2013). In summary, our results indicate that although AGO1 and HSP90 appear to be interdependent with regard to responding properly to environmental perturbations, neither contribution is fully explained by the other. Furthermore, AGO1 appears to be the principal integrator of environmental signaling for the proper repression of lesion formation, with a much reduced role for the environmentally responsive HSP90.1 paralog.
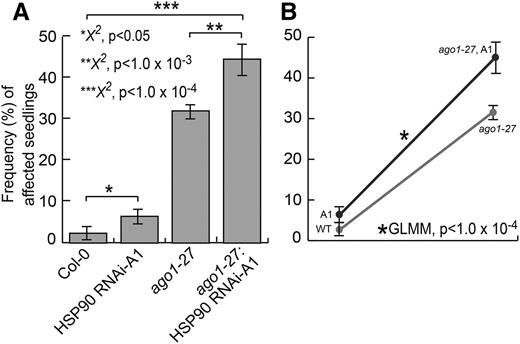
AGO1 and HSP90 interact genetically. A, HSP90 RNAi-A1 seedlings showed significantly more affected seedlings than the wild type (P < 0.05). ago1-27;HSP90 RNAi-A1 double mutant seedlings showed significantly more affected seedlings than ago1-27 single mutant seedlings (P < 1 × 10−4). Error bars represent se across two replicates of 108 seedlings. B, The ago1-27 mutation showed higher penetrance in the presence of HSP90 RNAi-A1 (ago1-27; A1) in full-spectrum light (GLMM, P < 1 × 10−4). WT, Wild type. Statistical significance was calculated using the χ2 test of significance (***, P < 1 × 10−4; **, P < 1 × 10−3; and *, P < 0.05; for all possible P values, see Supplemental Table S4). n = 72 to 144 individuals per replicate, with two replicates each. Error bars represent se across biological replicates.
DISCUSSION
Here, we present a previously undescribed phenotype in ago1 mutant plants, apparently stochastic lesions in cotyledons, and uncover its molecular underpinnings. Our initial observation and subsequent investigation of the lesion phenotype identify AGO1 as a key integrator of environmental signals and hormone signaling, thereby providing robustness to microenvironmental challenges as was observed previously for the molecular chaperone HSP90. The spontaneous lesions that we observed in ago1 seedlings, especially in response to full-spectrum light, appear to be dead and dying tissue bearing the hallmarks of HR due to up-regulated JA/ET signaling. In the wild type, functional AGO1 appears to play a key role in properly deploying HR only upon pathogen attack.
Previous studies have implicated AGO1 in different plant defense pathways through gene expression (Kurihara et al., 2009; Zhang et al., 2011) and genetic studies (Yi and Richards, 2007). It is tempting to speculate that the observed lesions in ago1 mutants involve misregulation of R genes (Yi and Richards, 2007), although empirical evidence is lacking thus far. In contrast, the role of AGO1 in defense against viruses is exceedingly well studied; viruses employ several strategies to counteract AGO1 upon infection, including translational repression and protein degradation (Chapman et al., 2004; Lakatos et al., 2006; Zhang et al., 2006; Azevedo et al., 2010; Chiu et al., 2010; Giner et al., 2010).
Notably, ago1-27 mutants were far more responsive to full-spectrum light than other ago1 mutants, presumably due to the functional differences between mutant alleles. The AGO1-dependent regulation of gene activity occurs through two principal mechanisms, mRNA cleavage (Voinnet, 2009) and translational repression (Iwakawa and Tomari, 2013). In plants, AGO1 was thought previously to function primarily through RNA cleavage, because of the nearly perfect base pairing of plant miRNAs with their target sequences (Rhoades et al., 2002). More recently, however, several studies have provided evidence for translational repression (Aukerman and Sakai, 2003; Bari et al., 2006; Brodersen et al., 2008), in particular with regard to the heat stress-induced down-regulation of SPL transcription factors (Gandikota et al., 2007; Stief et al., 2014). Translational repression, which is reversible, has been proposed as a mechanism for facilitating recovery after environmental stress (Sunkar et al., 2007; Voinnet, 2008). In fact, the environmentally responsive ago1-27 allele (Morel et al., 2002) is thought to affect primarily translational repression (Brodersen et al., 2008; Voinnet, 2009). Our ago1-27 results are consistent with a recent report demonstrating that ago1-27 seedlings are more responsive to heat stress compared with ago1-25 seedlings (Stief et al., 2014). Similarly, as we show, translational repression appears to be relevant for the response to the subtle stress of full-spectrum light.
Our study also links light conditions to JA signaling, which is supported by several previous studies. Specifically, solar UV-B radiation renders plants more resistant to herbivory, primarily due to up-regulation of the JA pathway (Ballare et al., 1996; Mazza et al., 1999; Demkura et al., 2010). Consistent with these prior findings, the ago1-dependent lesions were much less frequent in filtered light conditions that exclude UV-B radiation (Stasinopoulos and Hangarter, 1990).
Similarly, HSP90 perturbation is known to increase JA signaling, and it yields greater resistance to herbivores (Sangster et al., 2007). As expected, mutants with reduced HSP90 levels show subtly but significantly greater frequency of affected seedlings. HSP90 plays important roles in plant defense, especially in defense against microbial pathogens (Hubert et al., 2003; Takahashi et al., 2003; Sangster and Queitsch, 2005; Zhang et al., 2015). HSP90 also is implicated in chaperoning AGO1 (Iki et al., 2010). Our double mutant and expression analyses, however, suggest a synergistic, rather than a simple epistatic or additive, relationship. ago1-27 single mutants show many more affected seedlings than HSP90 RNAi-A1 seedlings. Although HSP90 expression levels in the double mutant are considerably higher than in the single HSP90 mutant, the number of affected seedlings increases further in double mutants beyond additive expectations. Note that the interpretation of this double mutant result is complicated by the fact that both mutants represent weak partial mutants of essential genes. The increased penetrance of the ago1-27 mutation upon HSP90 perturbation is consistent with the notion that HSP90 is critical for buffering deleterious mutations (Queitsch et al., 2012). Our results further suggest that fluctuations in environmental conditions are buffered by both AGO1 and HSP90.
In our gene expression studies, we observed that SA marker genes, like JA and ET markers, also were highly up-regulated. We conclude that ago1 plants generally up-regulate these hormone pathways, presumably in part due to complex cross-regulation. However, as our exogenous hormone treatments and genetic analyses demonstrate, JA is the main driver of the lesion phenotype. In summary, AGO1 and the miRNA pathway appear to minimize variation in phenotype among isogenic seedlings and suppress the aberrant misregulation of plant defense pathways, similar to previous observations for the phenotypic capacitor HSP90.
MATERIALS AND METHODS
Plant Growth Conditions
Arabidopsis (Arabidopsis thaliana) Col-0 was used as the wild-type reference. ago1-46, ago1-27, ago1-25, HSP90 RNAi-A1, coi1-1, and ein2-1 were in the Col-0 background (Supplemental Table S2). For experiments, seeds were sterilized with ethanol and plated onto 1× Murashige and Skoog (MS) basal salt medium supplemented with 1× MS vitamins, 1% (w/w) Suc, 0.05% (w/w) MES, and 0.24% (w/w) phytagel. After stratification in the dark at 4°C for 5 d, plates were transferred to an incubator (Conviron) that was set to long days (16 h of light/8 h of dark at 22°C/20°C), with light supplied at 100 μmol m−2 s−1 by cool-white fluorescent bulbs; for control plates (filtered light), a long-pass yellow filter that blocks 454-nm light was placed in front of the bulbs. Seedlings were then scored for lesions at day 10.
Genotyping and Gene Expression Analyses
RNA Extraction and Quantitative Real-Time PCR
Total RNA was extracted from 30 mg of frozen tissue using the SV Total RNA Isolation System (Promega). Subsequently, 2 μg of RNA was subjected to DNase treatment using the Ambion Turbo DNA-Free Kit (Applied Biosystems). RNA integrity and purity were checked with an Agilent Bioanalyzer using the RNA 6000 Nano Kit (Agilent Technologies). For complementary DNA synthesis, 200 ng of DNase-treated RNA was reverse transcribed using the Transcriptor First Strand cDNA Synthesis Kit (Roche) and oligo(dT) primers. Transcript abundance was determined by real-time quantitative PCR using the LightCycler 480 system (Roche), with LightCycler 480 SYBR Green I Master (Roche) and the following PCR conditions: 5 min at 95°C, followed by 35 cycles of 15 s at 95°C, 20 s at 55°C, and 20 s at 72°C. To ensure that PCR products were unique, a melting-curve analysis was performed after the amplification. UBC21 (UBIQUITIN-CONJUGATING ENZYME 21, AT5G25760) was used as a reference. All quantitative RT-PCR primers were designed with Primer3Plus software (http://www.primer3plus.com/). Sequences for real-time PCR primers are shown in Supplemental Table S4. Relative quantification was determined with the ƊƊCT method (Fryer et al., 2011). Error was calculated using se across at least three biological replicates.
DNA Extraction and Genotyping
Arabidopsis genomic DNA was extracted for genotyping analysis by PCR using the cetyl-trimethyl-ammonium bromide method (Weigel and Glazebrook, 2002). The RNAi-A1, ago1-27, coi1-1, and ein2-1 alleles were genotyped using the primers listed in Supplemental Table S3. PCR conditions for ago1-27 genotyping were as follows: 5 min at 94°C, followed by 35 cycles of 30 s at 94°C, 30 s at 55°C, and 1 min at 72°C. PCR product was then digested with Bsp1286I at 37°C, which cuts the wild-type sequence. PCR conditions for coi1-1 genotyping were as follows: 5 min at 94°C, followed by 35 cycles of 30 s at 94°C, 30 s at 55.7°C, and 1 min at 72°C. PCR product was then digested with XcmI, which cuts the wild-type sequence. PCR conditions for ein2-1 genotyping were as follows: 5 min at 94°C, followed by 35 cycles of 30 s at 94°C, 30 s at 63.2°C, and 2 min at 72°C. PCR product was then digested with BsrBI, which cuts the wild-type sequence. HSP90 RNAi-A1 plants were genotyped using two PCR assays. For RNAi construct identification, the Lm1 and Rc3 primers (Supplemental Table S3) were used with the following parameters: 3 min at 95°C, followed by 30 cycles of 30 s at 95°C, 30 s at 55°C, and 1 min, 15 s at 72°C. For wild-type sequence identification, the Lw1 and Rc3 primers (Supplemental Table S3) were used with the previously described PCR conditions.
Hormone Response Assays
MeJA
Seedlings were grown as described in “Plant Growth Conditions” under full-spectrum light conditions. Five days post germination, seedlings in groups of 36 were sprayed with 1 mL of water, 1 mL of 10 μm MeJA solution (Sigma-Aldrich), or 1 mL of 50 μm MeJA solution and returned to the incubator. Seedlings were then scored for lesions at day 10.
SA
Seedlings were grown as described in “Plant Growth Conditions” under full-spectrum light conditions. Five days post germination, seedlings in groups of 36 were sprayed with 1 mL of 1× PBTx, 1 mL of 10 μm SA in 1× PBTx, or 1 mL of 100 μm SA in 1× PBTx and returned to the incubator. PBTx buffer was prepared as follows: Bovine serum albumin 0.2% (w/v) and Triton X-100 0.5% (v/v) dissolved in 1× phosphate buffered saline (PBS). Seedlings were then scored for lesions at day 10.
ACC (ET Precursor)
Seedlings were grown as described in “Plant Growth Conditions” under full-spectrum light conditions. Five days post germination, seedlings in groups of 36 were sprayed with 1 mL of water, 1 mL of 1 μm ACC, 1 mL of 10 μm ACC, or 1 mL of 20 μm ACC in water and returned to the incubator. Seedlings were then scored for lesions at day 10.
Callus Induction
To test for callus-hormone responsivity, plants were grown for 10 d under full-spectrum light conditions. Affected and unaffected tissue was then excised using a Pasteur pipette tip and plated on medium with varying levels of callus-inducing hormones. Medium with the low concentration of callus-inducing hormones was as follows: 250 μL of 2,000× 2,4-dichlorophenoxyacetic acid (final concentration of 0.25 mg L−1), 250 μL of 2,000× kinetin (final concentration of 0.025 mg L−1), 1× Gamborg’s B5 salts, 20 g of Glc, and 0.5 g of MES, pH 5.7. Medium with the high concentration of callus-inducing hormones was as follows: 500 μL of 2,000× 2,4-dichlorophenoxyacetic acid (final concentration of 0.5 mg L−1), 500 μL of 2,000× kinetin (final concentration of 0.05 mg L−1), 1× Gamborg’s B5 salts, 20 g of Glc, and 0.5 g of MES, pH 5.7. Plates were then placed under yellow filter light conditions (to maintain the quality of hormones) and scored at 10 d post callus induction using the scoring index described in Supplemental Figure S2.
HR Assays
Trypan Blue Staining
Fresh affected and unaffected cotyledons were collected and then treated with Trypan Blue staining solution. Trypan Blue staining solution was prepared as follows. Trypan Blue (Sigma-Aldrich) was added to lactophenol (10 mL of lactic acid, 10 mL of glycerol, 10 mL of phenol, and 10 mL of water) to a concentration of 2.5 mg mL−1. Two volumes of ethanol were then added to the Trypan Blue/lactophenol solution. Staining solution was added to fresh tissue and boiled for 1 min (100°C). Tissue was then allowed to incubate in staining solution for 5 to 10 min at room temperature while rotating. Stain solution was removed by washing with lactophenol/ethanol solution. Samples were then mounted on glass slides with 70% (w/v) glycerol and photographed with a Canon Power Shot S5 IS camera. Images were edited using Adobe Photoshop CS3.
DAB
Fresh affected and unaffected cotyledons were collected and then treated with 1 mg mL−1 DAB (pH 3.8; Sigma-Aldrich) staining solution for 8 h. Tissue was then destained with 100% (v/v) ethanol. Samples were mounted on glass slides with 70% (w/v) glycerol and photographed with a Canon Power Shot S5 IS camera. Images were edited using Adobe Photoshop CS3.
Phenotyping Assays
MeJA Screening
Ten-day-old seedlings were sprayed with 50 to 100 μm MeJA (Sigma-Aldrich) solution in water. Seedlings were incubated for an additional 3 d and then screened for response to JA (i.e. anthocyanin accumulation)
ACC Screening
For these experiments, seeds were sterilized with ethanol and plated onto 1× MS basal salt medium supplemented with 1× MS vitamins, 1% (w/w) Suc, 0.05% (w/w) MES, and 0.24% (w/w) phytagel. Experimental plates were made to 10 μm ACC. After stratification in the dark at 4°C for 5 d, plates were transferred to an incubator (Conviron) that was set to long days (16 h of light/8 h of dark at 22°C/20°C), with light supplied at 100 mmol m−2 s−1 by cool-white fluorescent bulbs. Control and experimental plates were exposed to filtered light for 3 h and then wrapped in aluminum foil. Plates were incubated for 7 d and then scored for the triple response.
Statistical Analysis
All statistical analyses were performed in R version i386 3.1.2. Comparisons of seedling lesion frequencies were performed with the χ2 test. Seedling lesion frequency was modeled with binomial regression using a mixed model from the MASS package’s glmmPQL function (Ripley et al., 2015). Replicate and individual within a replicate were designated as random effects, whereas genotype and treatment were designated as fixed effects.
The accession numbers for the genes analyzed in this work are listed in Supplemental Table S2.
Supplemental Data
The following supplemental materials are available.
Supplemental Figure S1. Plant stem cell marker genes are not up-regulated in full-spectrum light-grown ago1-27 seedlings.
Supplemental Figure S2. ago1-27 lesion tissue did not show enhanced callus formation.
Supplemental Figure S3. The disruption of JA perception in coi1-1 is epistatic to both ago1-27- and ein2-1-dependent lesion phenotypes.
Supplemental Figure S4. HSP90 expression is reduced by RNAi in ago1-27 seedlings.
Supplemental Table S1. Reported P values.
Supplemental Table S2. Accession numbers of the genes analyzed.
Supplemental Table S3. Genotyping primers.
Supplemental Table S4. Quantitative RT-PCR primers.
ACKNOWLEDGMENTS
We thank Maximilian Press, Pauline Rival, Jennifer Lachowiec, and Elizabeth Morton for helpful discussions and article editing.
Glossary
- miRNA
microRNA
- HR
hypersensitive response
- ROS
reactive oxygen species
- SA
salicylic acid
- JA
jasmonate
- ET
ethylene
- Col-0
Columbia-0
- GLMM
generalized linear mixed model
- SEM
scanning electron microscopy
- DAB
3,3′-diaminobenzidine
- MeJA
methyl jasmonate
- ACC
1-aminocyclopropane-1-carboxylic acid
- RNAi
RNA interference
- MS
Murashige and Skoog
- RT
reverse transcription
LITERATURE CITED
Author notes
This work was supported by the National Institutes of Health (new innovator award no. DP2OD008371 to C.Q.), the National Science Foundation (graduate fellowship no. DGE–1256082 to G.A.M.), and the National Human Genome Research Institute (genome training grant no. T32 HG00035 to G.A.M.).
Present address: University of California Los Angeles, David Geffen School of Medicine, Department of Human Genetics, Gonda (Goldschmied) Neuroscience and Genetics Research Center, 695 Charles E. Young Drive South, Box 708822, Los Angeles, CA 90095.
Address correspondence to [email protected].
The author responsible for distribution of materials integral to the findings presented in this article in accordance with the policy described in the Instructions for Authors (www.plantphysiol.org) is: Christine Queitsch ([email protected]).
T.L. noted ago1-dependent lesions; G.A.M., T.L., and C.Q. conceived experiments; G.A.M. and T.L. performed experiments; G.A.M. performed data analysis; G.A.M. and C.Q. wrote the article; all authors read, commented on, and improved the article.
Articles can be viewed without a subscription.