-
PDF
- Split View
-
Views
-
Cite
Cite
Leonardo A Arias, Sebastián D’Ippolito, Jésica Frik, Natalia L Amigo, Fernanda Marchetti, Claudia A Casalongué, Gabriela C Pagnussat, Diego F Fiol, The DC1 Domain Protein BINUCLEATE POLLEN is Required for POLLEN Development in Arabidopsis thaliana, Plant and Cell Physiology, Volume 63, Issue 12, December 2022, Pages 1994–2007, https://doi.org/10.1093/pcp/pcac122
- Share Icon Share
Abstract
The development of the male gametophyte is a tightly regulated process that requires the precise control of cell division and gene expression. A relevant aspect to understand the events underlying pollen development regulation constitutes the identification and characterization of the genes required for this process. In this work, we showed that the DC1 domain protein BINUCLEATE POLLEN (BNP) is essential for pollen development and germination. Pollen grains carrying a defective BNP alleles failed to complete mitosis II and exhibited impaired pollen germination. By yeast two-hybrid analysis and bimolecular fluorescence complementation assays, we identified a set of BNP-interacting proteins. Among confirmed interactors, we found the NAC family transcriptional regulators Vascular Plant One‐Zinc Finger 1 (VOZ1) and VOZ2. VOZ1 localization changes during pollen development, moving to the vegetative nucleus at the tricellular stage. We observed that this relocalization requires BNP; in the absence of BNP in pollen from bnp/BNP plants, VOZ1 nuclear localization is impaired. As the voz1voz2 double mutants showed the same developmental defect observed in bnp pollen grains, we propose that BNP requirement to complete microgametogenesis could be linked to its interaction with VOZ1/2 proteins. BNP could have the role of a scaffold protein, recruiting VOZ1/2 to the endosomal system into assemblies that are required for their further translocation to the nucleus, where they act as transcriptional regulators.
Introduction
The development of the male gametophyte of flowering plants (pollen grain) takes place inside the anthers and involves two sequential phases: microsporogenesis and microgametogenesis. Microsporogenesis begins when a diploid microsporocyte undergoes meiotic division to form a tetrad of haploid microspores (Twell 2011). During microgametogenesis, a large vacuole is formed inside the released microspores and positions the nucleus in the periphery of the cell. This stage is followed by an asymmetric division or pollen mitosis I (PMI) that yields a large vegetative cell and a small germ cell. The germ cell is subsequently engulfed within the cytoplasm of the vegetative cell. The germ cell then undergoes a second division, pollen mitosis II (PMII), which results in twin sperm cells and yields the mature tricellular pollen. In species such as Arabidopsis thaliana, PMII takes place inside the pollen grain prior to anthesis. Although transcriptional studies have provided significant advances in the understanding of the molecular mechanisms controlling male gametophyte development, the picture is still far from complete (reviewed in Hafidh et al. 2016). Studies on isolated mutants have also proved to be of great importance to identify novel genes and cellular mechanisms involved in this process (Borg et al. 2009).
Divergent C1 (DC1) domains are cysteine/histidine-rich zinc finger modules found exclusively in the plant kingdom. DC1 domains resemble the C1 domains present in protein kinase C and in over a dozen animal proteins (Colon-Gonzalez and Kazanietz 2006). However, C1 domains are not frequent in plants, as they were only identified in diacylglycerol (DAG) kinases (Escobar-Sepúlveda et al. 2017). C1 domains have the ability to bind the secondary messenger DAG but also participate in protein–protein interactions and in the targeting of proteins to the membrane (Colon-Gonzalez and Kazanietz 2006). Sequence and structure similarities between DC1 and C1 domains suggest that some of the roles played by C1 domains in animal proteins could be fulfilled by DC1 domains in plants (D’Ippólito et al. 2017).
The Arabidopsis genome encodes for over 140 uncharacterized proteins harboring DC1 domains (D’Ippólito et al. 2017). Reports regarding genes coding for DC1 domain proteins were limited to transcriptional responses. In Arabidopsis, At5g17960 was described as responsive to hormones and stress treatments (Bhaskar et al. 2015) and ULI3 (At5g59920) was related to ultraviolet-B light responses (Suesslin and Frohnmeyer 2003). In other plant species, DC1 domain proteins have been also associated with stress and hormone responses (Shinya et al. 2007, Li et al. 2010, Hwang et al. 2014, Gao et al. 2016).
The first functional characterization of a DC1 domain protein, Vacuoleless Gametophytes (VLG), was recently reported (D’Ippólito et al. 2017). VLG localizes to prevacuolar compartments/multivesicular bodies, and it was shown to be essential for the development of female and male gametophytes. A role for VLG was proposed during vacuole biogenesis, likely through the interaction with the vesicle-associated membrane protein PVA12 and the GDSL-motif lipase LTL1 (D’Ippólito et al. 2017).
In this work, we studied one of the closest VLG homologs, encoded by At2g44370, and identified that the DC1 domain protein BINUCLEATE POLLEN (BNP) plays a relevant role during male gametophyte development in Arabidopsis. In addition, we demonstrated that BNP is required for pollen germination and early sporophytic developmental stages. To functionally characterize BNP, we searched for protein interactors and identified transcriptional regulators VOZ1 and VOZ2 as BNP binding proteins, and we showed that VOZ proteins co-localize to BNP, supporting a physiological interaction. We showed that VOZ1 localizes in the cytoplasm at early stages of pollen development but moves to the vegetative nucleus at the tricellular stage. We observed that this relocalization requires BNP; in the absence of BNP in pollen from bnp/BNP plants, VOZ1 nuclear localization is impaired. As voz1voz2 mutants also exhibit pollen developmental defects (Celesnik et al. 2013), we propose that the interaction between BNP and VOZ proteins outside the nucleus might impact further on VOZ1/2 activities, resulting in functional consequences for gene expression. Thus, BNP likely act as a scaffold protein recruiting VOZ1/2, and likely other proteins, to the endosomal system into assemblies that might be required to accomplish VOZ1/2 translocation to the nucleus.
Results
BNP codes for a DC1-domain-containing protein
At2g44370, here named BNP, is a single exon gene that encodes a 250-amino-acid-long protein with three DC1 domains (Fig. 1B). Phylogenetic analysis of Arabidopsis DC1 domain protein family grouped BNP into the most divergent cluster, with At5g40590 and VLG being the closest homologs to BNP (Fig. 1C). Multiple sequence alignments of selected protein sequences of this cluster and DC1 domain proteins from Capsicum annuum, tobacco, wheat and cotton showed amino acid conservation mostly restricted to the residues that define the DC1 signature motif, which might be involved in the Zn2+ coordination and folding of the domain (Fig. 1D). On the other hand, amino acids corresponding to loop regions in the folded domain—which might define its binding capabilities (Rahman and Das 2015)—showed very low similarity, suggesting a diversity of interactors for the aligned proteins.
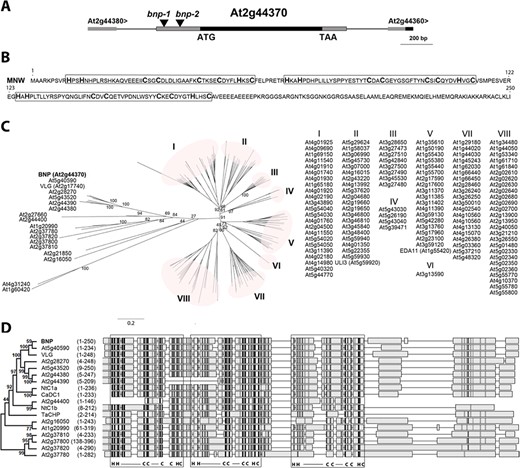
BNP codes for a DC1-domain-containing protein. (A) Schematic view of a region of Arabidopsis chromosome 2 (from 18,323,538 to 18,321,537 bp-TAIR10) showing BNP genomic location and bnp-1 and bnp-2 insertion sites. (B) BNP amino acid sequence with boxed DC1 domains. (C) Phylogenic tree of 140 DC1-domain-containing proteins in Arabidopsis. Bootstrap test results (1,000 replicates) of the major nodes are indicated. IDs of sequences grouped in clusters I–VIII are listed. (D) Alignment of BNP with its closest Arabidopsis homologs and CaDC1 (AEI52549), TaCHP (ACU80555), NtDC1a (BAF80452) and NtDC1b (BAF80453). Phylogenic tree with bootstrap test results (1,000 replicates) for the alignment of the complete 18 sequences is shown. Amino acid region represented for each sequence is indicated. DC1 domains are framed, and signature residues and binding loop regions are indicated (C = Cys, H = His, ― = loop). Higher intensity in gray scale denotes higher sequence similarity.
Bnp is a gametophytic-acting mutation
Two Arabidopsis lines with T-DNA insertions in BNP (SALK_114889—here named bnp-1 and GK-008E01—here named bnp-2) were studied (Fig. 1A). After backcrossing to the Col-0 ecotype, the progeny of self-pollinated plants was genotyped. No homozygous mutant plants were recovered in the offspring from bnp-1/BNP or bnp-2/BNP self-pollinated plants. The ratio of hemizygous to WT plants in the progeny of self-fertilized bnp-1/BNP plants was 1.44 (n = 183). For bnp-2/BNP plants, segregation analysis for sulfadiazine resistance showed a ratio of resistance to sensitive plants of 1.31 (n = 2,634). The proportion of plants harboring the transgene recovered in the progeny of self-fertilized plants was lower than the Mendelian segregation ratio expected for diploid sporophytic (3:1) or embryo lethal (2:1) mutants, suggesting a gametophytic defect. Furthermore, no obvious sporophytic phenotypes were observed in the hemizygous plants, suggesting that bnp is a recessive mutation (Supplementary Fig. S1). The siliques of bnp/BNP plants showed a small percentage of aborted seeds, not different from WT plants (Supplementary Fig. S1).
As no homozygous plants were recovered and siliques from hemizygous plants looked normal, the BNP mutation could be affecting pollen development, germination or early development. To test this hypothesis, we performed reciprocal crosses between bnp/BNP and WT plants and calculated the transmission efficiency of the mutant allele (TE = resistant/WT offspring × 100) (Table 1). Only 15.5% of microgametophytes carrying bnp-1 and 22.0% of the microgametophytes carrying bnp-2 transmitted the insertion to the next generation (P < 0.0001, chi-square test), indicating that bnp affects male gametophyte development or function (Table 1). No significant defects were observed for transmission through the female gametophyte (90.8% and 95.9% for bnp-1 and bnp-2, respectively; Table 1; P-values of 0.51 and 0.66, respectively; chi-square test).
Transmission efficiency of bnp alleles. Transmission efficiency of the bnp-1 and bnp-2 alleles in reciprocal crosses between mutant and WT plants.
Parental genotypes . | Genotype of progenya Number of individuals . | Transmission efficiency of: . | . | ||
---|---|---|---|---|---|
Female . | Male . | WT . | bnp-1/BNP . | bnp-1 . | P-valueb . |
bnp-1/BNP | WT | 98 | 89 | ♀ 90.8% | 0.51 <0.0001 |
WT | bnp-1/BNP | 181 | 28 | ♂ 15.5% | |
WT | bnp-2/BNP | bnp-2 | |||
bnp-2/BNP | WT | 217 | 208 | ♀ 95.9% | 0.66 <0.0001 |
WT | bnp-2/BNP | 254 | 56 | ♂ 22% |
Parental genotypes . | Genotype of progenya Number of individuals . | Transmission efficiency of: . | . | ||
---|---|---|---|---|---|
Female . | Male . | WT . | bnp-1/BNP . | bnp-1 . | P-valueb . |
bnp-1/BNP | WT | 98 | 89 | ♀ 90.8% | 0.51 <0.0001 |
WT | bnp-1/BNP | 181 | 28 | ♂ 15.5% | |
WT | bnp-2/BNP | bnp-2 | |||
bnp-2/BNP | WT | 217 | 208 | ♀ 95.9% | 0.66 <0.0001 |
WT | bnp-2/BNP | 254 | 56 | ♂ 22% |
No homozygous plants were detected;
Chi-square test for a 1:1 segregation hypothesis (degree of freedom = 1).
Transmission efficiency of bnp alleles. Transmission efficiency of the bnp-1 and bnp-2 alleles in reciprocal crosses between mutant and WT plants.
Parental genotypes . | Genotype of progenya Number of individuals . | Transmission efficiency of: . | . | ||
---|---|---|---|---|---|
Female . | Male . | WT . | bnp-1/BNP . | bnp-1 . | P-valueb . |
bnp-1/BNP | WT | 98 | 89 | ♀ 90.8% | 0.51 <0.0001 |
WT | bnp-1/BNP | 181 | 28 | ♂ 15.5% | |
WT | bnp-2/BNP | bnp-2 | |||
bnp-2/BNP | WT | 217 | 208 | ♀ 95.9% | 0.66 <0.0001 |
WT | bnp-2/BNP | 254 | 56 | ♂ 22% |
Parental genotypes . | Genotype of progenya Number of individuals . | Transmission efficiency of: . | . | ||
---|---|---|---|---|---|
Female . | Male . | WT . | bnp-1/BNP . | bnp-1 . | P-valueb . |
bnp-1/BNP | WT | 98 | 89 | ♀ 90.8% | 0.51 <0.0001 |
WT | bnp-1/BNP | 181 | 28 | ♂ 15.5% | |
WT | bnp-2/BNP | bnp-2 | |||
bnp-2/BNP | WT | 217 | 208 | ♀ 95.9% | 0.66 <0.0001 |
WT | bnp-2/BNP | 254 | 56 | ♂ 22% |
No homozygous plants were detected;
Chi-square test for a 1:1 segregation hypothesis (degree of freedom = 1).
Although the insertions in BNP compromised the transmission of the mutation through the male gametophyte, a fraction (about 15–22%) of pollen grains were still able to transmit the insertion to the next generation. However, we did not recover homozygous mutant plants in the self-progeny of hemizygous plants. Thus, we investigated whether embryogenesis or seed germination was affected by the mutation, analyzing pistils and seeds from bnp/BNP plants. No obvious embryo defects were observed in siliques from bnp/BNP plants, as neither developing siliques nor seed sets showed differences from WT siliques (Supplementary Fig. S1). However, seeds from bnp/BNP self-pollinated plants showed reduced germination rates when compared to WT plants (Table 2). Further analysis of the germinated seeds showed that a fraction of the seedlings were arrested soon after germination. Values of seedling lethality obtained were 0.9% for WT (n = 440), 3.4% (n = 754) for seeds from bnp-1/BNP self-pollinated plants and 4.5% (n = 973) for bnp-2/BNP self-pollinated plants (Table 2). Polymerase chain reaction (PCR) genotyping of arrested seedlings showed that 75% (n = 12) corresponded to bnp/bnp homozygous individuals.
Viability of the progeny in bnp mutants. Seed germination and seedling lethality of the progeny of self-pollinated bnp-1/BNP, bnp-2/BNP and WT plants.
. | . | % Unviable progeny . | . | |
---|---|---|---|---|
Parental genotype . | % Viable progeny . | Not germinated . | Seedling lethality . | P-valuea . |
WT | 98.6 (435/441) | 0.2 (1/441) | 1.1 (5/441) | |
bnp-1/BNP | 94.8 (715/754) | 1.7 (13/754) | 3.4 (26/754) | <0.001 |
bnp-2/BNP | 91.4 (688/753) | 2.8 (21/753) | 5.8 (44/753) | <0.00001 |
. | . | % Unviable progeny . | . | |
---|---|---|---|---|
Parental genotype . | % Viable progeny . | Not germinated . | Seedling lethality . | P-valuea . |
WT | 98.6 (435/441) | 0.2 (1/441) | 1.1 (5/441) | |
bnp-1/BNP | 94.8 (715/754) | 1.7 (13/754) | 3.4 (26/754) | <0.001 |
bnp-2/BNP | 91.4 (688/753) | 2.8 (21/753) | 5.8 (44/753) | <0.00001 |
In parentheses, the number of individuals observed over the total analyzed.
Chi-square test for a hypothesis of no relationship between the genotype of the parents and the viability of the progeny (degree of freedom = 1).
Viability of the progeny in bnp mutants. Seed germination and seedling lethality of the progeny of self-pollinated bnp-1/BNP, bnp-2/BNP and WT plants.
. | . | % Unviable progeny . | . | |
---|---|---|---|---|
Parental genotype . | % Viable progeny . | Not germinated . | Seedling lethality . | P-valuea . |
WT | 98.6 (435/441) | 0.2 (1/441) | 1.1 (5/441) | |
bnp-1/BNP | 94.8 (715/754) | 1.7 (13/754) | 3.4 (26/754) | <0.001 |
bnp-2/BNP | 91.4 (688/753) | 2.8 (21/753) | 5.8 (44/753) | <0.00001 |
. | . | % Unviable progeny . | . | |
---|---|---|---|---|
Parental genotype . | % Viable progeny . | Not germinated . | Seedling lethality . | P-valuea . |
WT | 98.6 (435/441) | 0.2 (1/441) | 1.1 (5/441) | |
bnp-1/BNP | 94.8 (715/754) | 1.7 (13/754) | 3.4 (26/754) | <0.001 |
bnp-2/BNP | 91.4 (688/753) | 2.8 (21/753) | 5.8 (44/753) | <0.00001 |
In parentheses, the number of individuals observed over the total analyzed.
Chi-square test for a hypothesis of no relationship between the genotype of the parents and the viability of the progeny (degree of freedom = 1).
Altogether these results suggest that the mutation might cause impairment in male gametogenesis or function, reduced seed germination and seedling lethality, which together explain the absence of homozygous mutant plants in the progeny of selfed hemizygous plants.
Pollen development in bnp mutants is arrested at the binuclear stage
The viability of the mature pollen was analyzed in bnp-1/BNP and bnp-2/BNP by means of Alexander staining and fluorescein diacetate (FDA) staining. No differences were observed compared to pollen obtained from WT plants, indicating that the bnp mutation does not affect pollen viability (Supplementary Fig. S2).
We later analyzed the nuclear composition of the mature pollen using 4ʹ,6-diamino-phenylindole (DAPI) staining. Normal mature pollen contains three nuclei: a vegetative nucleus and two sperm nuclei generated by the mitotic division of the germ cell. Mature pollen from WT plants showed 99.2% (n = 772) of trinucleate grains. On the other hand, bnp/BNP plants presented only about 57–59% of trinucleate mature pollen grains [56.9% (n = 1,123) in bnp-1/BNP and 59.0% (n = 981) in bnp-2/BNP] (Table 3). The rest were found at the binucleate stage, suggesting developmental arrest.
Nuclear composition of mature pollen in bnp mutants. Mature pollen from bnp-1/BNP, bnp-2/BNP and WT plants was stained using DAPI.
Genotype . | % Trinucleate pollen . | P-valuea . |
---|---|---|
WT | 99.2 (766/772) | |
bnp-1/BNP | 56.9 (639/1,123) | <0.0001 |
bnp-2/BNP | 59.0 (579/981) | <0.0001 |
Genotype . | % Trinucleate pollen . | P-valuea . |
---|---|---|
WT | 99.2 (766/772) | |
bnp-1/BNP | 56.9 (639/1,123) | <0.0001 |
bnp-2/BNP | 59.0 (579/981) | <0.0001 |
In parentheses, the number of individuals observed over the total analyzed.
The statistical significance was determined by Student’s t-test.
Nuclear composition of mature pollen in bnp mutants. Mature pollen from bnp-1/BNP, bnp-2/BNP and WT plants was stained using DAPI.
Genotype . | % Trinucleate pollen . | P-valuea . |
---|---|---|
WT | 99.2 (766/772) | |
bnp-1/BNP | 56.9 (639/1,123) | <0.0001 |
bnp-2/BNP | 59.0 (579/981) | <0.0001 |
Genotype . | % Trinucleate pollen . | P-valuea . |
---|---|---|
WT | 99.2 (766/772) | |
bnp-1/BNP | 56.9 (639/1,123) | <0.0001 |
bnp-2/BNP | 59.0 (579/981) | <0.0001 |
In parentheses, the number of individuals observed over the total analyzed.
The statistical significance was determined by Student’s t-test.
A genetic complementation assay confirmed that the insertions in BNP are causing the abnormalities observed during pollen development. The bnp-1/BNP hemizygous plants transformed with a ProBNP:BNP:GFP translational fusion construct showed trinucleate mature pollen grains at similar levels of WT plants (Supplementary Fig. S3). This result was verified in T3 plants of five independent complemented lines.
In order to better characterize the defect observed during pollen development, bnp-1/BNP plants were crossed to the qrt1 homozygous plants, which are impaired in a pectin methylesterase responsible for the separation of microspore tetrads (Francis et al. 2006). F2 plants that were hemizygous for the insertion in BNP and homozygous for qrt1 (bnp/BNP qrt1/qrt1) were further analyzed. Mature tetrads were collected and stained with DAPI. Consistently with our previous results, most tetrads (99.1%) of bnp-1/BNP qrt1/qrt1 plants contained at least one microspore and, in most cases, two microspores with an abnormal number of nuclei (Fig. 2A), indicating a failure to complete PMII in about 46% of the pollen grains analyzed.
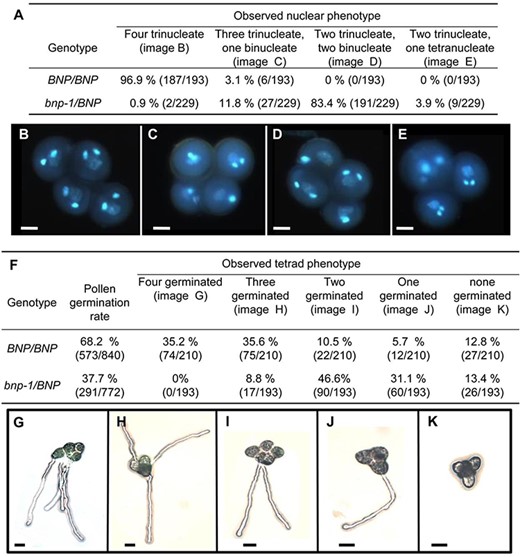
Pollen development and germination is impaired in bnp-1 mutants. (A) Quantification of phenotypes observed in BNP/BNP and bnp-1/BNP plants in the qrt1/qrt1 background. (B–E) Nuclear configuration types revealed by DAPI staining. Bars = 10 µm. (F) Quantification of PT germination in tetrads from BNP/BNP and bnp-1/BNP plants in the qrt1/qrt1 background. (G–K) PT germination in tetrads. Bars = 20 µm.
We next assessed the germination of pollen grains in the tetrads. As shown in Fig. 2, germination rates were 37.7% in pollen collected from the bnp-1/BNP qrt1/qrt1 plants and 68.2% in pollen grains from the BNP/BNP qrt1/qrt1 plants (Fig. 2F). This indicates a reduction of 44.7% in the germination rate in the pollen from the bnp-1/BNP qrt1/qrt1 plants compared to pollen grain tetrads from the BNP/BNP qrt1/qrt1 plants. As the reduction in the rate of germination observed in the hemizygous background bnp-1/BNP was less than 50%, this suggests that although severely affected, a small fraction of bnp pollen grains are still able to germinate.
Analysis of pollen grain germination in bnp-2/BNP plants showed comparable values, as we calculated a decrease in germination rate of about 36% compared to pollen grains from WT plants. Germination rates of 43% (n = 787) and 67% (n = 443) were recorded for pollen grains collected from bnp-2/BNP and WT plants, respectively.
These results show that BNP is required to complete pollen development, specifically to undergo PMII. Arrested pollen grains were viable, but germination was impaired, which explains the reduced transmission of bnp through the male gametophyte (Table 1).
BNP is expressed in pollen and young sporophytic tissues
The expression of BNP was studied in Arabidopsis pollen expressing the BNP–GFP fusion driven by the BNP promoter, which was proved to be functional, as it complemented the phenotype in bnp/BNP plants (Supplementary Fig. S3). BNP–GFP was detected in cytoplasmic speckles following a punctuate pattern at early stages of pollen development (microspore tetrad and released microspore) and in discrete bigger compartments at bicellular and tricellular stages (Fig. 3A, H). The same localization pattern was observed in the pollen tube (PT) of germinating pollen grains (Fig. 3I–J).
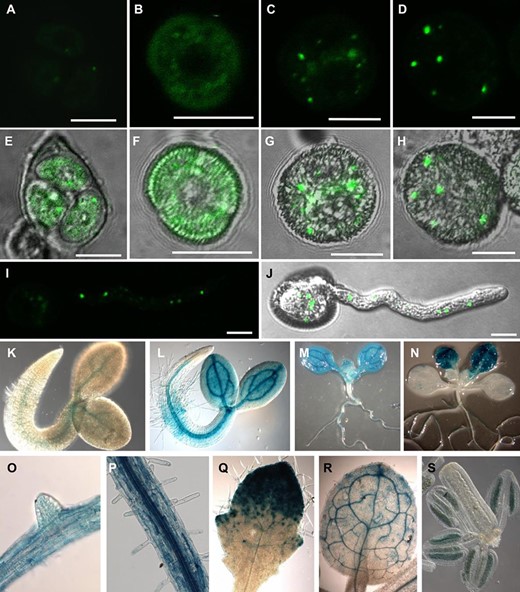
BNP is expressed in pollen and young sporophytic tissues. (A–D) Confocal images showing BNP–GFP through microgametogenesis. (E–H) Overlay between DIC and GFP fluorescence. (A and E) Microspore tetrad, (B and F) released microspore, (C and G) bicellular stage and (D and H) tricellular stage. (I) Image showing a projection along the z-axis of all planes showing GFP fluorescence. (J) Overlay of DIC and GFP fluorescence showing a germinating pollen grain. (K–S) GUS activity in Arabidopsis transgenic plants carrying the pBNP:GUS construct. (K) Seedling 24 h after imbibition, (L) 48 h after imbibition, (M) 6 d post-germination and (N) 12 d post-germination. (O) Root with lateral root primordium, (P) primary root with root hairs, (Q) third leaf 8 d after imbibition and (R) third leaf 14 d after imbibition. (S) GUS signal was detected inside anthers at floral developmental stage 10. Three independent transgenic lines were used for this study. Pictures are representative of the results obtained in all analyzed lines. Bars = 10 µm.
To gain further knowledge of BNP roles in planta, a reporter construction expressing the GUS gene under the control of the BNP promoter was used to analyze BNP expression. GUS activity accumulated at early stages of seedling development, mainly in vascular tissues and developing leaves. It was also observed to be associated with anthers at very early stages of floral development, indicating that the expression of BNP can be detected before meiosis (Fig. 3K–S). Expression data observed were comparable to transcriptional data available in the eFP Browser Data Analysis Tool (https://bar.utoronto.ca/eplant/) (Supplementary Fig. S4). These results also indicate that BNP could be functional not only during pollen development but also at additional developmental stages, such as sporogenesis and germination, and early stages of sporophyte development.
BNP interacts with transcription factors VOZ1 and VOZ2
To gain insight into possible functions of BNP, we carried out a yeast two-hybrid (Y2H) screen to identify potential BNP protein interactors. Two closely related NAC family transcription factors, VOZ1 and VOZ2, were identified among interactors (all interacting clones are presented in Supplementary Table S1). Interestingly, VOZ1 and VOZ2 were reported to bind a pollen-specific promoter element (Mitsuda et al. 2004). BNP was also retrieved in the Y2H screening as a self-interactor (Supplementary Table S1). To confirm BNP–VOZ1, BNP–VOZ2 and BNP–BNP interactions, we performed bimolecular fluorescence complementation (BiFC) assays.
We made constructs to express BNP, and each of the candidate proteins was fused to either the N- or the C-terminal of yellow fluorescent protein (YFP) fragments to generate BNP-N-YFP and VOZ1-C-YFP, VOZ2-C-YFP or BNP-C-YFP fusion constructs. The fusion constructs under the control of the CaMV 35S promoter were used to transiently transform Nicotiana benthamiana leaves, and BiFC signals were analyzed using confocal microscopy (Fig. 4). YFP signal was detected with VOZ1, VOZ2 and BNP, indicating the interaction of the pairs BNP–VOZ1, BNP–VOZ2 and BNP–BNP (Fig. 4). Control N. benthamiana leaves transfected with BNP-N-YFP, VOZ1-C-YFP, VOZ2-C-YFP or BNP-C-YFP did not show any BiFC signal (Fig. 4). An additional control experiment using VLG (as BNP’s closest homolog, sharing 72.0% identity) did not show an interaction with VOZ1, VOZ2 and BNP, indicating the specificity of the observed interactions (Supplementary Fig. S5).
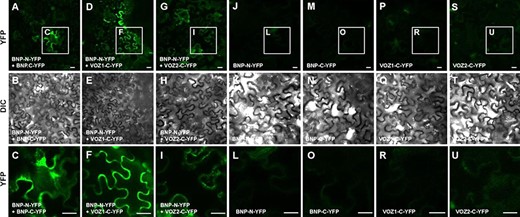
BNP interacts with itself, VOZ1 and VOZ2. BiFC analysis of the interaction of BNP with itself, VOZ1 and VOZ2 in N. benthamiana leaves. Representative confocal microscopy images, showing that BNP interacts with (A–C) itself, (D–F) VOZ1 and (G–I) VOZ2. Reconstituted YFP fluorescence or DIC images of N. benthamiana leaves are shown in epidermal cells co-infiltrated with A. tumefaciens harboring the indicated constructs. Control N. benthamiana leaves transfected with (J–L) BNP-N-YFP, (M–O) BNP-C-YFP, (P–R) VOZ1-C-YFP and (S–U) VOZ2-C-YFP do not show any BiFC signal. The last row (C, F, I, L, O, R and U) corresponds to the magnification of regions of interest (insets) indicated in the first row. Bars = 20 µm.
BNP mainly localizes to Rha1-positive vesicles and co-localizes with VOZ1 and VOZ2
We used the lipophilic dye FM4-64 to gain insight on the subcellular localization of BNP. After internalization, this dye is sequentially distributed to different organelle membranes, following the endocytic pathway (Bolte et al. 2004). We followed FM4-64 in the roots of plants expressing the fusion protein BNP–GFP at different time points. We observed no co-localization of internalized FM4-64 and BNP–GFP at early time points of incubation (10–30 min), discarding the localization of BNP–GFP to early endosomes or to components of the trans-Golgi Network (Supplementary Fig. S6A–E). However, high levels of co-localization were observed at about 120 min of incubation, supporting the localization in late endosomes (Supplementary Fig. S6F–J). In agreement with that, BNP–GFP did not co-localize with Brefeldin bodies caused by the addition of the endosomal recycling inhibitor Brefeldin A to FM4-64-treated roots (Supplementary Fig. S6K–O). In addition, treatment with the PI3K inhibitor LY294002, which was reported to cause swelling and association with late endosomes (Takáč et al. 2013), caused a significant increase in the area of BNP compartments (Supplementary Fig. S7).
To assess BNP localization, we used N. benthamiana to transiently express BNP–GFP together with a late endosome/prevacuolar compartments/multivesicular bodies marker, the Rab5 GTPase Rha1 fused to RFP (Foresti et al. 2010), or with a Golgi apparatus marker, the rat sialyltransferase St fused to RFP (Wee et al. 1998). Representative images shown in Fig. 5 and Pearson and Spearman correlation (PSC) values indicate that BNP–GFP co-localized with Rha1–RFP (Fig. 5A–E) and did not co-localize with St–RFP (Fig. 5F–J), suggesting that BNP is mainly confined to late endosome/prevacuolar compartments/multivesicular bodies’ associated vesicles.
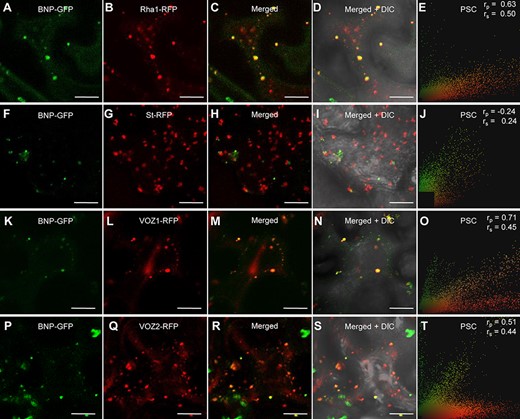
BNP co-localizes with VOZ1 and VOZ2 to Rha1-positive vesicles. (A, F, K and P) Localization of BNP–GFP transiently expressed in N. benthamiana epidermal cells. (B) Fluorescent marker for late endosomes/prevacuolar compartments/multivesicular bodies, RFP–Rha1. (C) Merged image of BNP–GFP and RFP–Rha1. (D) An overlay with the corresponding bright-field image. (E, J, O and T) PSC test calculated using a plugin for ImageJ; r values indicate the level of co-localization ranging from +1 for perfect co-localization to −1 for negative correlation. (G) Fluorescent marker for Golgi apparatus, St–RFP. (H) Merged image of BNP–GFP and St–RFP. (I) An overlay with the corresponding bright-field image. (L) Localization of RFP–VOZ1. (M) Merged image of BNP–GFP and RFP–VOZ1. (N) The corresponding bright-field image of the cells shown in (M). (Q) Localization of RFP–VOZ2. (R) Merged image of BNP–GFP and RFP–VOZ2. (S) Bright-field image of the cells shown in (R). Bars = 20 µm.
To further confirm that BNP and VOZ proteins are indeed localized in the same subcellular compartment, we performed a co-localization study. Nicotiana benthamiana plants were transiently co-transfected with constructions to express BNP–GFP and either VOZ1–RFP or VOZ2–RFP. In these transfected cells, BNP–GFP and VOZ1–RFP or VOZ2–RFP can be found separately; however, a significant shared pixel population indicates partial co-localization, confirmed by moderate correlation scores for Pearson and Spearman tests (Fig. 5K–T).
BNP is required for the nuclear localization of VOZ1 in pollen
We next analyzed VOZ1 localization in pollen at anthesis from BNP/BNP and hemizygous bnp-1/BNP plants (Fig. 6). We observed that in mature trinucleated pollen, VOZ1 localizes to the cytoplasm and to the vegetative nucleus; however, in mature but binucleated pollen from bnp-1/BNP plants, VOZ1 is localized only in the cytoplasm (Fig. 6). This result suggests that the absence of a functional BNP could be affecting the localization of VOZ to the nucleus.
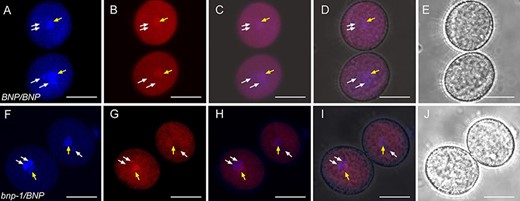
VOZ1 localization in mature pollen from WT and bnp-1/BNP plants. Mature pollen from (A–E) BNP/BNP, VOZ1–RFP and (F–J) bnp-1/BNP, VOZ1–RFP plants was analyzed. Representative z-stack images of confocal micrographs displaying (A, F) DAPI nuclear staining, (B, G) VOZ1–RFP fluorescence and (E, J) bright-field images. (C, H) Merged DAPI and VOZ1–RFP fluorescence images and (D, I) merged DAPI, VOZ1–RFP fluorescence and bright-field images. Arrows indicate the vegetative nucleus (central position) and the generative nuclei (peripheral positions) in each pollen grain. Bars = 10 µm.
To test this, we transformed BNP/BNP and hemizygous bnp-1/BNP plants, both in the qrt1 background, with ProUb10:VOZ1–RFP and analyzed VOZ1–RFP intracellular localization during pollen development (Fig. 7). The introduction of the mutation in the qrt1 background allows to compare and analyze any difference in VOZ1 localization that might arise from an impairment in BNP, as pollen tetrads of the bnp-1/BNP genotype are constituted by two mutant (binucleate) and two WT (trinucleate) pollen cells.
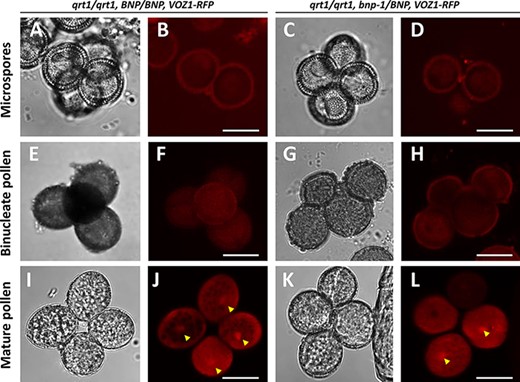
Nuclear localization of VOZ1 is affected in bnp mutants. Pollen tetrads from qrt1/qrt1, BNP/BNP, VOZ1–RFP (A, B, E, F, I and J) and qrt1/qrt1, bnp-1/BNP, VOZ1–RFP (C, D, G, H, K and L) plants were analyzed at microspore (A–D), binucleate (E–H) and mature tricellular (I–L) stages. Representative z-stack images of confocal micrographs displaying VOZ1–RFP fluorescence (B, D, F, H, J and L) and bright-field images (A, C, E, G, I and K) are shown. Arrowheads indicate nuclear localized VOZ1. Bars = 10 µm.
At earlier developmental stages (microspore and bicellular pollen), VOZ1 was detected at the cytoplasm with very low signal intensities, showing no differences between the qrt1/qrt1, BNP/BNP, VOZ1–RFP and qrt1/qrt1, bnp-1/BNP, VOZ1–RFP genotypes (Fig. 7A–H). In mature WT pollen, however, VOZ1–RFP was detected at higher levels, and its localization appeared to be distributed between the cytoplasm and the nuclei (Fig. 7I–L). Most of the pollen tetrads (91.5%) from the qrt1/qrt1, BNP/BNP, VOZ1-RFP plants presented the VOZ1 nuclear localization in the four cells of the tetrad (Table 4). However, most of the tetrads from the qrt1/qrt1, bnp-1/BNP, VOZ1-RFP genotype presented the VOZ1 nuclear localization only in one or two pollen cells (85.2%, Table 4). Nuclear composition of mature pollen from plants expressing VOZ1–RFP (qrt1/qrt1, BNP/BNP, VOZ1–RFP and qrt1/qrt1, bnp-1/BNP, VOZ1–RFP) shown in Table 5 presented comparable values to the results shown in Fig. 2A for qrt1/qrt1, BNP/BNP and qrt1/qrt1, bnp-1/BNP, respectively.
VOZ1 localization in mature pollen in bnp mutants expressing VOZ1. Mature pollen tetrads from the qrt1/qrt1, BNP/BNP, VOZ1–RFP and qrt1/qrt1, bnp-1/BNP, VOZ1–RFP plants.
. | Observed VOZ1 localization . | ||||
---|---|---|---|---|---|
Genotype . | Four nuclear . | Three nuclear and one cytoplasmic . | Two nuclear and two cytoplasmic . | One nuclear and three cytoplasmic . | Four cytoplasmic . |
qrt1/qrt1, BNP/BNP, VOZ1–RFP | 91.5% (65/71) | 7.0% (5/71) | 1.4% (1/71) | 0% (0/71) | 0% (0/71) |
qrt1/qrt1, bnp-1/BNP, VOZ1–RFP | 1.3% (1/76) | 11.8% (9/76) | 57.8% (44/76) | 27.6% (21/76) | 1.3% (1/76) |
. | Observed VOZ1 localization . | ||||
---|---|---|---|---|---|
Genotype . | Four nuclear . | Three nuclear and one cytoplasmic . | Two nuclear and two cytoplasmic . | One nuclear and three cytoplasmic . | Four cytoplasmic . |
qrt1/qrt1, BNP/BNP, VOZ1–RFP | 91.5% (65/71) | 7.0% (5/71) | 1.4% (1/71) | 0% (0/71) | 0% (0/71) |
qrt1/qrt1, bnp-1/BNP, VOZ1–RFP | 1.3% (1/76) | 11.8% (9/76) | 57.8% (44/76) | 27.6% (21/76) | 1.3% (1/76) |
In parentheses, the number of individuals observed over the total analyzed.
VOZ1 localization in mature pollen in bnp mutants expressing VOZ1. Mature pollen tetrads from the qrt1/qrt1, BNP/BNP, VOZ1–RFP and qrt1/qrt1, bnp-1/BNP, VOZ1–RFP plants.
. | Observed VOZ1 localization . | ||||
---|---|---|---|---|---|
Genotype . | Four nuclear . | Three nuclear and one cytoplasmic . | Two nuclear and two cytoplasmic . | One nuclear and three cytoplasmic . | Four cytoplasmic . |
qrt1/qrt1, BNP/BNP, VOZ1–RFP | 91.5% (65/71) | 7.0% (5/71) | 1.4% (1/71) | 0% (0/71) | 0% (0/71) |
qrt1/qrt1, bnp-1/BNP, VOZ1–RFP | 1.3% (1/76) | 11.8% (9/76) | 57.8% (44/76) | 27.6% (21/76) | 1.3% (1/76) |
. | Observed VOZ1 localization . | ||||
---|---|---|---|---|---|
Genotype . | Four nuclear . | Three nuclear and one cytoplasmic . | Two nuclear and two cytoplasmic . | One nuclear and three cytoplasmic . | Four cytoplasmic . |
qrt1/qrt1, BNP/BNP, VOZ1–RFP | 91.5% (65/71) | 7.0% (5/71) | 1.4% (1/71) | 0% (0/71) | 0% (0/71) |
qrt1/qrt1, bnp-1/BNP, VOZ1–RFP | 1.3% (1/76) | 11.8% (9/76) | 57.8% (44/76) | 27.6% (21/76) | 1.3% (1/76) |
In parentheses, the number of individuals observed over the total analyzed.
Nuclear composition of mature pollen in bnp mutants expressing VOZ1. Mature pollen tetrads from qrt1/qrt1, BNP/BNP, VOZ1–RFP and qrt1/qrt1, bnp-1/BNP, VOZ1–RFP plants.
. | Observed nuclear phenotype . | |||
---|---|---|---|---|
Genotype . | Four trinucleate . | Three trinucleate and one binucleate . | Two trinucleate and two binucleate . | Two binucleate and one tetranucleate . |
qrt1/qrt1, BNP/BNP, VOZ1–RFP | 97.3% (71/73) | 2.7% (2/73) | 0% (0/73) | 0% (0/73) |
qrt1/qrt1, bnp-1/BNP, VOZ1–RFP | 3.6% (4/110) | 18.2% (20/110) | 77.3% (85/110) | 0.9% (1/110) |
. | Observed nuclear phenotype . | |||
---|---|---|---|---|
Genotype . | Four trinucleate . | Three trinucleate and one binucleate . | Two trinucleate and two binucleate . | Two binucleate and one tetranucleate . |
qrt1/qrt1, BNP/BNP, VOZ1–RFP | 97.3% (71/73) | 2.7% (2/73) | 0% (0/73) | 0% (0/73) |
qrt1/qrt1, bnp-1/BNP, VOZ1–RFP | 3.6% (4/110) | 18.2% (20/110) | 77.3% (85/110) | 0.9% (1/110) |
In parentheses, the number of individuals observed over the total analyzed.
Nuclear composition of mature pollen in bnp mutants expressing VOZ1. Mature pollen tetrads from qrt1/qrt1, BNP/BNP, VOZ1–RFP and qrt1/qrt1, bnp-1/BNP, VOZ1–RFP plants.
. | Observed nuclear phenotype . | |||
---|---|---|---|---|
Genotype . | Four trinucleate . | Three trinucleate and one binucleate . | Two trinucleate and two binucleate . | Two binucleate and one tetranucleate . |
qrt1/qrt1, BNP/BNP, VOZ1–RFP | 97.3% (71/73) | 2.7% (2/73) | 0% (0/73) | 0% (0/73) |
qrt1/qrt1, bnp-1/BNP, VOZ1–RFP | 3.6% (4/110) | 18.2% (20/110) | 77.3% (85/110) | 0.9% (1/110) |
. | Observed nuclear phenotype . | |||
---|---|---|---|---|
Genotype . | Four trinucleate . | Three trinucleate and one binucleate . | Two trinucleate and two binucleate . | Two binucleate and one tetranucleate . |
qrt1/qrt1, BNP/BNP, VOZ1–RFP | 97.3% (71/73) | 2.7% (2/73) | 0% (0/73) | 0% (0/73) |
qrt1/qrt1, bnp-1/BNP, VOZ1–RFP | 3.6% (4/110) | 18.2% (20/110) | 77.3% (85/110) | 0.9% (1/110) |
In parentheses, the number of individuals observed over the total analyzed.
Next, we analyzed if the lack of a functional BNP could also affect the levels of VOZ1 in pollen. We quantified VOZ1–RFP by measuring red fluorescence intensity in mature pollen cells of the qrt1/qrt1, BNP/BNP, VOZ1–RFP and qrt1/qrt1, bnp-2/BNP, VOZ1-RFP plants (Supplementary Fig. S8). Interestingly, lower red fluorescence levels were recorded in the qrt1/qrt1, bnp-2/BNP, VOZ1-RFP plants, suggesting that BNP could have a role in the promotion of VOZ1 stabilization. Altogether, these results strongly suggest that BNP is involved in VOZ1 localization, as its nuclear localization relies on the presence of a functional BNP.
We then analyzed BNP localization during pollen development using WT plants harboring stable BNP–GFP and VOZ1–RFP constructs. We observed that both VOZ1 and BNP are localized in the cytoplasm at early developmental stages. In contrast to VOZ1, no nuclear localization was recorded for BNP (Supplementary Fig. S9). Partial co-localization was detected in areas in the cytosol where BNP and VOZ1 might interact at the microspore and bicellular stages. This was not observed in mature pollen, where VOZ1 shows both nuclear and cytoplasmic localization. As BNP remained in the cytosol at every developmental stage, forming speckles during the binucleate and mature pollen stages, any role related to VOZ proteins translocation/function would be performed outside the nucleus and before pollen maturation.
Discussion
In this study, we identified BNP, a gene coding for a DC1 domain protein that is required for pollen development and germination. Analysis of the hemizygous bnp/BNP plants showed that transmission through the male gametophyte is severely impaired; about half of the pollen grains from bnp/BNP plants were arrested by the binucleate stage, and their germination was significantly reduced.
By means of Y2H and BiFC experiments, we identified and confirmed VOZ1 and VOZ2 as BNP-interacting proteins. VOZ1 and VOZ2 are transcription factors that belong to the NAC transcription factor family and were reported to be involved in the control of flowering time (Celesnik et al. 2013, Yasui and Kohchi 2014), either through the binding of PhyB (Yasui et al. 2012), Flowering Locus C (Mimida et al. 2011) or Constans (Koguchi et al. 2017, Kumar et al. 2018). VOZ proteins are also involved in biotic and abiotic stress responses (Neill et al. 2002, Nakai et al. 2013, Song et al. 2018, Schwarzenbacher et al. 2020). VOZ1 and VOZ2 were proved to be functionally redundant, as single mutants for each gene showed normal phenotypes, but the voz1voz2 double mutants displayed phenotypic defects (Yasui et al. 2012, Celesnik et al. 2013, Nakai et al. 2013, Kumar et al. 2018). Interestingly, voz1voz2 plants presented the same pollen developmental defect observed in bnp/BNP plants, as a fraction of their pollen grains contained only two nuclei—the vegetative nucleus and a single reproductive nucleus (Celesnik et al. 2013). This fact supports a physiological role for the interaction between BNP and VOZ proteins during pollen development.
BNP was localized mainly in Rha1-positive vesicles, which are defined as late endosomes/prevacuolar compartments/multivesicular bodies. VOZ proteins are functional in the nucleus, but they were initially identified in cytoplasmic speckles and a cytoplasmic–nuclear translocation was proposed to modulate VOZ activity as transcriptional regulators (Yasui et al. 2012, Koguchi et al. 2017). VOZ1 and VOZ2 do not contain either a transmembrane region or localization signals such as a nuclear localization signal (NLS) or nuclear export signal (Jensen Michael et al. 2010). This suggests that VOZ1 and VOZ2 nuclear/cytoplasmic localization must rely on another mechanism. Endocytic proteins have been previously shown to participate in the spatiotemporal regulation of transcription factors (Palfy et al. 2012, Wu et al. 2012, Serrano et al. 2016). They may facilitate the assembly of multi-molecular complexes through protein–protein interaction domains and act as regulators (Pyrzynska et al. 2009). As can be concluded from the analysis shown in Figs. 6, 7 and Table 3, a similar situation could be taking place between the pairs BNP–VOZ1, where VOZ1 is required to interact with BNP to reach the nuclear import machinery.
The involvement of the endomembrane system in nuclear translocation has been previously reported, as several membrane receptors undergo endocytosis and are transported to the nucleus via endosomal trafficking (Mukherjee et al. 2006, Wang et al. 2010, 2020). However, the mechanisms of the process are still under investigation, and several mechanisms are proposed to allow the cargo to reach the nuclear import machinery, including retrograde movement of vesicles through the Golgi and endoplasmic reticulum, the release of the cargo in the cytoplasm or the exposing of an NLS that is recognized by importin-β (Ek-Ramos et al. 2014).
Implications of the VOZ1/2 nuclear translocation may be related to the role of VOZ1/2 as a transcriptional regulator, with such regulation occurring in the nucleus (Yasui et al. 2012). Interestingly, the nuclear localization of VOZ proteins was rarely detected, and the addition of NLS signals was usually required to place it in the nucleus (Yasui et al. 2012, Schwarzenbacher et al. 2020). However, it was reported that the degradation of VOZ1/2 also takes place in the nucleus, facilitated by the E3 ubiquitin ligase BRUTUS (Selote et al. 2018). Thus, BNP could be involved in the function and/or in the degradation of VOZ1/2.
Our results also indicated that BNP could be essential not only during pollen development but also at additional developmental stages, such as germination and early stages of sporophyte development. In agreement with this, BNP expression was detected very early during seedling development, in particular in vascular tissues (Fig. 3). The expression pattern of BNP also suggests that it could play roles in other sporophytic tissues, either through the interaction with VOZ TFs, that are also ubiquitously expressed through the plant (Yasui et al. 2012), or through the interaction with other proteins. Potential BNP-interacting proteins identified by Y2H experiments (Supplementary Table S1) provide a promising list of candidates that might be involved with BNP in other plant tissues.
Only one additional DC1 domain protein, VLG, has been characterized so far and was likewise proven to be required for pollen development. However, VLG deficiency caused the arrest of microgametogenesis at PMI, an earlier stage, yielding unviable uninucleate pollen grains (D’Ippólito et al. 2017). On the other hand, VLG was also essential for female gametogenesis (D’Ippólito et al. 2017).
Notably, the Arabidopsis genome encodes over 140 uncharacterized proteins harboring DC1 domains. Multiple sequence alignments of DC1 domain proteins from different species showed amino acid conservation restricted to the residues that define the DC1 signature motif, but divergence in the amino acids corresponding to the folded domain—which might define binding capabilities (Rahman and Das 2015)—suggesting a diversity of interactors. Thus, DC1 domain proteins emerge as a novel family of highly specialized modular scaffold proteins, are capable of promoting the recruitment of binding targets and affect their localization and functionality.
Altogether, our results support a role for BNP acting as a scaffold protein recruiting VOZ1 and VOZ2 to the endomembrane system. This recruitment appears to facilitate VOZ1—and likely VOZ2—translocation to the nucleus, promoting their activity as transcriptional regulators during pollen development. In addition, a role for BNP in the regulation of the levels VOZ proteins could not be discarded, as lower levels of these proteins were present in mature pollen lacking a functional BNP.
Materials and Methods
Plant materials and growth conditions
Arabidopsis (Columbia [Col-0] ecotype) lines SALK_114889 (bnp-1, with T-DNA insertion located in chr2 TAIR10 18323075) and GK-008E01 (bnp-2, with T-DNA insertion located in chr2 TAIR10 18323371) were obtained from Arabidopsis Biological Resource Center (ABRC) (Ohio State University, OH, USA) (Alonso et al. 2003). Both lines were backcrossed twice to the WT Columbia prior to their use. The presence of a single insertion in At2g44370 and all genotypes was confirmed by PCR-based genotyping and segregation analysis. When indicated, seeds were sterilized in 20% (v/v) sodium hypochlorite, washed with sterile water and plated on Murashige and Skoog (MS) plates with 50 μg/ml of kanamycin or 7.5 μg/ml of sulfadiazine, stratified at 4°C for 48 h and placed at 23°C, in 16 h light and 8 h dark. Resistant seedlings were then transferred onto soil and grown under the conditions described above.
Molecular characterization of insertional lines
For bnp-1, the left border–genomic sequence junction was determined by PCR in plants, showing kanamycin resistance using the T-DNA-specific primer LBb1 combined with the genomic sequence-specific primers bnp-1 RP and bnp-1 LP (Supplementary Table S2). For bnp-2, the left border–genomic sequence junction was determined by PCR in plants, showing sulfadiazine resistance using the T-DNA-specific primer GKGT8474 combined with the genomic-sequence-specific primers bnp-2 RP and bnp-2 LP (Supplementary Table S2).
Segregation Analysis
For self-fertilization analysis, the progeny of self-pollinated hemizygous plants was germinated on a selective growth medium containing 50 µg/ml of kanamycin for bnp-1 or 7.5 µ/ml of sulfadiazine for bnp-2, and the ratio of resistant to sensitive plants was scored. Reciprocal crosses were performed as described previously (Pagnussat et al. 2005).
Generation of vectors for plant transformation
The genomic DNA was extracted from rosette leaves or whole seedlings as described (León et al. 2007). To generate ProBNP:BNP–GFP translational fusion, the region including BNP open reading frame (ORF) and putative promoter (672 upstream of the ATG codon) was amplified by PCR using the primer combination listed in Supplementary Table S2. The amplicon was cloned using pENTR™ Directional TOPO® Cloning Kit (Invitrogen, Carlsbad, CA, USA) using Gateway® technology, and the sequence was verified. The resulting plasmid pENTR-ProBNP-BNP was subjected to the attL × attR-recombinational reaction using the destination vector pMDC107 (Curtis and Grossniklaus 2003). The ProBNP:GUS construct was generated, amplifying the BNP putative promoter region (672 upstream of the ATG codon) by PCR using the primer combination listed in Supplementary Table S2. The amplicon was cloned into pENTR™/TOPO (Invitrogen), and its sequence was verified. The resulting plasmid pENTR-ProBNP was subjected to the LR reaction using the destination vector pMDC162 (Curtis and Grossniklaus 2003). For Pro35S:BNP–GFP construct, BNP ORF was PCR-amplified using as template clone DKLAT2G44370 obtained from ABRC (Ohio State University, OH, USA) using the primer combination listed in Supplementary Table S2. The amplicon was cloned into pENTR™/TOPO (Invitrogen), and its sequence was verified. The resulting plasmid pENTR-BNP was subjected to the LR reaction using the destination vector pMDC83 (Curtis and Grossniklaus 2003). For ProUb10:VOZ1–RFP and ProUb10:VOZ2–RFP constructions, respective ORFs were PCR-amplified using primers listed in Supplementary Table S2, cloned in pENTR™/TOPO (Invitrogen) and sequence-verified. The resulting plasmids pENTR-VOZ1 and pENTR-VOZ2 were subjected to the LR reaction using the destination vector pUBN-RFP-DEST (Grefen et al. 2010).
Obtaining bnp/BNP plants in the qrt1 background
To obtain bnp mutants in qrt1 background, bnp-1/BNP was crossed with the qrt1 homozygous plants. Kanamycin-resistant plants from the progeny were selected and allowed to self-fertilize. Homozygous qrt1 plants harboring bnp mutations were selected for further analysis (Johnson-Brousseau and McCormick 2004).
In a similar way, the qrt1/qrt1-bnp-2/BNP–VOZ1–RFP plants were obtained crossing bnp-2/BNP plants expressing VOZ1–RFP with the qrt1 homozygous plants.
Transformation of Agrobacterium tumefaciens and Arabidopsis
Vectors were introduced into Agrobacterium strain GV3101 by electroporation. Arabidopsis WT plants were transformed using the floral dip method (Clough and Bent 1998). Transformant plants were selected based on their ability to survive in MS medium with 15 mg l−1 hygromycin. Resistant plants (green seedlings with true leaves) was then transferred to soil and grown under the conditions described above.
Morphological and histological analyses
Pollen grain viability was assessed using Alexander’s staining (Alexander 1969) and FDA staining (Heslop-Harrison and Heslop-Harrison 1970). For pollen development analysis, successive buds in the same inflorescence were collected (Lalanne and Twell 2002), fixed in ethanol:acetic acid 3:1 until discoloration and stored at room temperature. Anthers were washed, dissected and mounted on microscopy slides with DAPI staining solution. For PT germination, pollen grains were incubated overnight in a dark moisture chamber at 22°C in 0.01% boric acid, 5 mM CaCl2, 5 mM KCl, 1 mM MgSO4, 10% sucrose pH 7.5 and 1.5% agarose, as described previously (Boavida and McCormick 2007). For GUS staining, tissues from ProBNP:GUS carrying plants were collected and incubated in GUS staining solution as already described (Pagnussat et al. 2007). Gametophytes were observed on a Zeiss Axio Imager A2 microscope using differential interference contrast (DIC) optics. Seedlings were observed on a Nikon SMZ800 Stereomicroscope, and images were captured with a Canon DS126431 camera.
Yeast two-hybrid screen
The Y2H screening was performed by Hybrigenics Services S.A.S. (Paris, France). A DNA fragment encoding Arabidopsis At2g44370 (amino acids 1–250) was PCR-amplified and cloned into pB66 as the C-terminal fusion to the Gal4 DNA-binding domain (Gal4-At2g44370) and used as a bait to screen Universal Arabidopsis Normalized library containing 3.2 million of independent clones in pGADT7-RecAB vector. pB66 was derived from the original pAS2ΔΔ (Fromont-Racine et al. 1997). For the Gal4 bait construct, 35 million clones were screened using a mating approach with YHGX13 (Y187 ade2-101::loxP-kanMX-loxP, matα) and L40∆Gal4 (mata) yeast strains as previously described (Fromont-Racine et al. 1997). Overall, 158 His+ colonies were selected on a medium lacking tryptophan, leucine and histidine. The prey fragments of the positive clones were amplified by PCR and sequenced at their 5ʹ and 3ʹ junctions. The resulting sequences were used to identify the corresponding interacting proteins in the GenBank database [National Center for Biotechnology Information (NCBI)] using a fully automated procedure, and in total, 23 different clones were identified (Supplementary Table S1). A confidence score (Predicted Biological Score) was attributed to each interaction as previously described (Formstecher et al. 2005).
Co-localization experiments in N. benthamiana
Agrobacterium tumefaciens strain GV3101 carrying Pro35S:BNP–GFP was co-infiltrated with A. tumefaciens cells carrying either the late endosome marker RFP–Rha1 or the Golgi apparatus marker St–RFP, in leaf epidermal cells of N. benthamiana plants. Infiltrated leaves were analyzed 48–72 h after infiltration on a confocal microscope (Nikon Eclipse C1 Plus) using EZ-C1 3.80 imaging software and Ti-Control. PSC plugin for ImageJ was used to calculate correlation coefficients from a minimum of 400 individual regions of interest corresponding with punctate structures that were manually selected from at least 10 independent cell images (French et al. 2008).
Co-localization experiments in Arabidopsis
Arabidopsis root cells expressing a 35S:BNP–GFP construction were used to analyze the co-localization between BNP and the endocytic tracer FM4-64. Roots were incubated for the indicated times with 6 µM FM4-64 solution, washed twice and then incubated in a liquid medium or with the indicated inhibitors. For Brefeldin A treatments, incubation was performed in 50 µM Brefeldin A solution for 15 min. For LY294002 treatments, incubation was performed in 100 µM LY294002 solution for 120 min. Confocal microscopy images were obtained at the indicated times with a confocal microscope (Nikon Eclipse C1 Plus) using EZ-C1 3.80 imaging software and Ti-Control. PSC plugin for ImageJ was used to calculate the correlation coefficients from a minimum of 400 individual regions of interest from at least 10 independent cell images (French et al. 2008).
BiFC analysis
The cDNA sequences of At2g44370 (BNP), At1g28520 (VOZ1), At2g42400 (VOZ2) and At2g17740 (VLG) were PCR-amplified using the primers listed in Supplementary Table S2. The PCR products were cloned into pENTR™/TOPO (Invitrogen), sequenced and recombined through BP reaction into BiFC destination plasmids pUBN-YN and pUBN-YC (Grefen et al. 2010). The binary plasmids were then transformed into A. tumefaciens strain GV3101 by electroporation. Split nYFP- and cYFP-tagged protein pairs and the gene-silencing suppressor p19 were co-expressed in N. benthamiana leaves by A. tumefaciens-mediated inoculation. Plant leaves were examined 48–72 h post-infiltration with a confocal microscope (Nikon Eclipse C1 Plus) using a Super Fluor 40.0×/1.30/0.22 Oil objective, numerical aperture 1.300. Acquisition was performed using EZ-C1 3.80 imaging software and Ti-Control. All pictures were acquired with the same settings.
Quantification of VOZ1–RFP in mature pollen
High-quality images of mature pollen grains from plants with the genotypes qrt1/qrt1, BNP/BNP, VOZ1–RFP and qrt1/qrt1, bnp-1/BNP, VOZ1–RFP were acquired, and red fluorescence was quantified. Red fluorescence background of mature pollen grains from plants not expressing VOZ1–RFP was subtracted. Fluorescence was expressed in arbitrary units relative to the calculated cell area of each pollen grain. Data points plotted on a violin plot using GraphPad, median (blue line) and quartiles (black lines) are indicated. Box: mean values ± SD and number of data points (n) are indicated. A significant difference of the means was indicated using unpaired t-test.
Bioinformatics and phylogenetic analysis
Sequences from the DC1 domain family were identified using the Basic Local Alignment Search Tool from NCBI using initially BNP as a reference and retrieved sequences in iterative searches. Protein sequence alignments were performed using MEGA7 (version 7.0.14) (Kumar et al. 2016). Phylogenetic trees were constructed using the neighbor-joining method and the default settings of MEGA7 (version 7.0.14) (Kumar et al. 2016). The evolutionary distances were computed using the Poisson correction method (Zuckerkandl and Pauling 1965) and are in the units of the number of amino acid substitutions per site. The trees were drawn using FigTree (version 1.4.3) (http://tree.bio.ed.ac.uk/). Graphic display of identities was visualized using Geneious (version 9.1.4) (http://www.geneious.com) based on an identity matrix (Kearse et al. 2012).
Supplementary Data
Supplementary data are available at PCP online.
Data Availability
All newly generated sequences are available for peer review and registered in public repositories. Sequence data from this article can be found in the Arabidopsis Genome Initiative database under accession numbers BNP (At2g44370), VOZ1 (At1g28520), VOZ2 (At2g42400) and VLG (At2g17740).
Funding
Agencia Nacional de Promoción Cientifica y Técnica Argentina (PICT2013-1524 and PICT2017-0232 to D.F.F.); Consejo Nacional de Investigaciones Cientificas y Técnicas (PIP-0200); Comisión de Investigaciones Cientificas; Universidad Nacional de Mar del Plata; Howard Hughes Medical Institute (International Early Career Scientist award 55007430 to G.C.P.).
Acknowledgements
We would like to thank Michael Blatt and Christopher Grefen (Glasgow University, UK) for providing pUB-Destination vectors, Jurgen Denecke (University of Leeds, UK) for providing the constructs St–RFP and Rha–RFP, Nicolas Setzes for assistance with statistical analysis and Daniela Villamonte for technical assistance with confocal microscopy. L.A.A., J.F. and F.M. are fellows of Consejo Nacional de Investigaciones Cientificas y Técnicas (CONICET); N.L.A. is a fellow of ANPCyT; D.F.F., G.C.P., C.A.C. and S.D. are researchers from CONICET.
Author Contributions
D.F.F. conceived the original screening and research plans; D.F.F., G.C.P. and C.A.C. supervised the experiments; L.A.A., S.D., J.F., N.L.A and F.M. performed most of the experiments; D.F.F., G.C.P and L.A.A. designed the experiments and analyzed the data; D.F.F. conceived the project and wrote the article with contributions of all the authors; D.F.F. agrees to serve as the author responsible for contact and ensures communication.
Disclosures
The authors have no conflicts of interest to declare.
References
Author notes
contributed equally to this work.