-
PDF
- Split View
-
Views
-
Cite
Cite
Md. Moshiul Islam, Wenxiu Ye, Fahmida Akter, Mohammad Saidur Rhaman, Daiki Matsushima, Shintaro Munemasa, Eiji Okuma, Yoshimasa Nakamura, Md. Sanaullah Biswas, Jun’ichi Mano, Yoshiyuki Murata, Reactive Carbonyl Species Mediate Methyl Jasmonate-Induced Stomatal Closure, Plant and Cell Physiology, Volume 61, Issue 10, October 2020, Pages 1788–1797, https://doi.org/10.1093/pcp/pcaa107
- Share Icon Share
Abstract
Production of reactive oxygen species (ROS) is a key signal event for methyl jasmonate (MeJA)- and abscisic acid (ABA)-induced stomatal closure. We recently showed that reactive carbonyl species (RCS) stimulates stomatal closure as an intermediate downstream of hydrogen peroxide (H2O2) production in the ABA signaling pathway in guard cells of Nicotiana tabacum and Arabidopsis thaliana. In this study, we examined whether RCS functions as an intermediate downstream of H2O2 production in MeJA signaling in guard cells using transgenic tobacco plants overexpressing A. thaliana 2-alkenal reductase (n-alkanal + NAD(P)+ ⇌ 2-alkenal + NAD(P)H + H+) (AER-OE tobacco) and Arabidopsis plants. The stomatal closure induced by MeJA was impaired in the AER-OE tobacco and was inhibited by RCS scavengers, carnosine and pyridoxamine, in the wild-type (WT) tobacco plants and Arabidopsis plants. Application of MeJA significantly induced the accumulation of RCS, including acrolein and 4-hydroxy-(E)-2-nonenal, in the WT tobacco but not in the AER-OE plants. Application of MeJA induced H2O2 production in the WT tobacco and the AER-OE plants and the H2O2 production was not inhibited by the RCS scavengers. These results suggest that RCS functions as an intermediate downstream of ROS production in MeJA signaling and in ABA signaling in guard cells.
Introduction
A tiny opening or a pore called a stoma is present in the epidermis of higher plants, and the pore is surrounded by a pair of guard cells. The guard cells modulate stomatal apertures in response to a variety of abiotic and biotic stresses and, consequently, control gas exchange, transpirational water loss and innate immunity (Murata et al. 2015, Melotto et al. 2017). Methyl jasmonate (MeJA), which mediates various plant defense responses (Turner et al. 2002), induces stomatal closure (Suhita et al. 2004, Munemasa et al. 2007).
Similar to abscisic acid (ABA), MeJA induces the production of reactive oxygen species (ROS), which is mediated by the plasma membrane NAD(P)H oxidases, AtrbohD and AtrbohF, in Arabidopsis thaliana guard cells, and then ROS activates the nonselective Ca2+-permeable cation (ICa) channels in the plasma membrane of the guard cells (Pei et al. 2000, Murata et al. 2001, Kwak et al. 2003, Suhita et al. 2004, Munemasa et al. 2007). The activation of the ICa channels contributes to cytosolic free Ca2+ concentration ([Ca2+]cyt) elevation (Schroeder and Hagiwara 1990, Hamilton et al. 2000), which is crucial for both ABA- and MeJA-induced stomatal closure (McAinsh et al. 1990, Staxen et al. 1999, Munemasa et al. 2007, Islam et al. 2010). Downstream of the [Ca2+]cyt elevation, calcium-dependent protein kinases (CDPKs) are regulators of the Ca2+-dependent guard cell ABA and MeJA signaling (Mori et al. 2006, Zhu et al. 2007, Zou et al. 2010, Geiger et al. 2011, Munemasa et al. 2011). A CDPK, CPK6, functions both in ABA-induced stomatal closure (Mori et al. 2006) and in MeJA-induced stomatal closure (Munemasa et al. 2011). By contrast, CPK3 is involved in ABA-induced stomatal closure but not in MeJA-induced stomatal closure. CORONATINE INSENSITIVE 1 (COI1) is an F-box protein and functions as a jasmonate co-receptor with JASMONATE ZIM DOMAIN (JAZ), which recognizes the bioactive hormone (3R,7S)-jasmonoyl-l-isoleucine with high specificity (Fonseca et al. 2009, Yan et al. 2009). The coi1 mutation impairs MeJA-induced stomatal closure but not ABA-induced stomatal closure (Munemasa et al. 2007). Stomatal closure induced by MeJA is also accompanied by the depletion of intracellular glutathione (GSH) in Arabidopsis guard cells (Akter et al. 2010, Akter et al. 2012), but depletion of GSH does not affect MeJA-induced ROS production in guard cells (Akter et al. 2013). However, it remains to be clarified how the generated ROS signal is transduced into downstream reactions in guard cells.
Various stresses induce the production of ROS in plants, and accumulating ROS constitutively oxidizes lipids to form lipid peroxides (Mène-Saffrané et al. 2007). Decomposition of lipid peroxides produces an array of substances, including aldehydes, ketones and hydroxy acids (Mueller and Berger 2009). Aldehydes and ketones comprising the α,β-unsaturated bond are termed reactive carbonyl species (RCS) (Mano 2012). They are more potent electrophiles than simple aldehydes or ketones (Esterbauer et al. 1991). In plants, there is a close relationship between levels of lipid peroxide-derived RCS and cellular damage under environmental stress and this damage can be suppressed by RCS scavenging enzymes (Yin et al. 2010, Yamauchi et al. 2011, Mano 2012, Yalcinkaya et al., 2019 and references in the latter two reviews).
RCS functions as an endogenous signaling component downstream of ROS production, to initiate programmed cell death (Biswas and Mano 2015, Biswas and Mano 2016) and to facilitate the auxin action for lateral root formation ( Biswas et al., 2019 ). The NADPH-dependent oxidoreductase 2-alkenal reductase (AER; EC 1.3.1.74) catalyzes the reduction in the α,β-unsaturated bond of RCS and, consequently, AER is involved in the detoxification of RCS in plants. Not only 2-alkenals but also the lipid peroxide-derived oxenes 9-oxo-octadeca-(10E),(12Z)-dienoic acid, 13-oxo-octadeca-(9E),(11Z)-dienoic acid and the potent genotoxin 4-oxo-(2E)-nonenal are recognized as substrates by A. thaliana alkenal reductase (At-AER) (Mano et al. 2002, Mano et al. 2005). To investigate the function of RCS, three independent transgenic tobacco (Nicotiana tabacum) plants P1#11, P1#14 and P1#18 overexpressing A. thaliana 2-alkenal reductase (At5g16970) (AER-OE plants) were generated, which have the 100- to 250-fold higher activity of NADPH-dependent 4-hydroxy-(E)-2-nonenal (HNE) reduction (Mano et al. 2005). Our recent studies showed that AER-OE and scavengers of RCS, carnosine and pyridoxamine, inhibited the ABA- and H2O2-induced stomatal closure whereas they impaired the ABA-induced RCS accumulation but not the ABA-induced H2O2 production in the guard cells and that acrolein and HNE induced stomatal closure in the atrbohD atrbohF double mutant plants (Islam et al. 2016, Islam et al. 2019). We have also presented that RCS activated ICa channels, triggered [Ca2+]cyt elevation and induced stomatal closure in Arabidopsis (Islam et al. 2019, Rhaman et al. 2020). These results indicate that RCS functions as an intermediate downstream of H2O2 production in ABA signaling in guard cells of N. tabacum and A. thaliana. Moreover, RCS enhances GSH depletion in tobacco leaves (Davoine et al. 2006) and RCS-induced stomatal closure is enhanced in the GSH-deficient mutant cad2-1 (Islam et al. 2019). These results suggest that GSH is negatively involved in ABA-induced stomatal closure through the conjugation of RCS. However, it remains to be clarified whether RCS is involved in MeJA-induced stomatal closure.
In this study, we employed AER-OE to examine the effects of MeJA on stomatal movements and the production of H2O2 and RCS and used Arabidopsis plants to examine stomatal movements and cytosolic Ca2+ elevation.
Results
Expression of At-AER in tobacco guard cells
Arabidopsis AER (EC 1.3.1.74) catalyzes the reduction in the α,β-unsaturated bond of RCS. Three independent AER-OE plants P1#11, P1#14 and P1#18 were generated in which the expression of At-AER was constitutively driven by the strong promoter 35S (Mano et al. 2005). The leaves of AER-OE plants P1#11, P1#14 and P1#18 showed higher levels of At-AER protein than those of wild-type (WT) plants (Mano et al. 2005) did. We examined the accumulation of transcripts of At-AER in isolated tobacco guard cell protoplasts (GCPs) using reverse transcription polymerase chain reaction (RT-PCR). The significant accumulation of At-AER transcript was detected in GCPs of AER-OE plants P1#11, P1#14 and P1#18 but not in GCPs of WT plants (Fig. 1), suggesting that At-AER was much more highly expressed in the guard cells of AER-OE plants P1#11, P1#14 and P1#18 than in those of the WT plants.
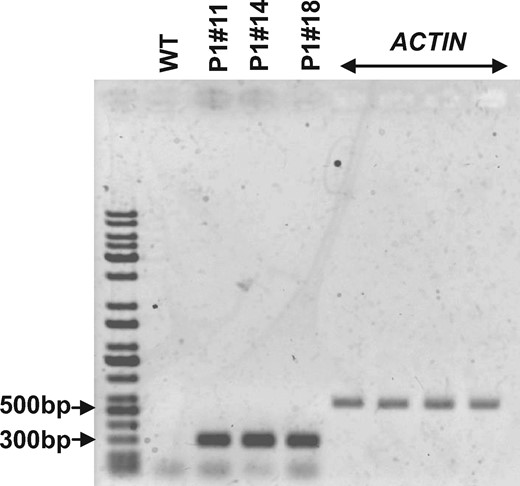
Expression of At-AER in tobacco GCPs. RT-PCR analysis of At-AER transcript accumulation levels in the WT and AER-OE plants P1#11, P1#14 and P1#18 GCPs. Tobacco ACTIN was used as an internal reference for the RT-PCR. The purity of GCPs was >98% on a cell basis.
MeJA-induced stomatal closure in AER-OE
To determine whether RCS mediates MeJA-induced stomatal closure, we analyzed stomatal responses to MeJA in the AER-OE tobacco plants. Treatment with MeJA at 1 and 10 µM induced stomatal closure in WT plants (P < 0.003 at 1 µM, P < 10–3 at 10 µM), but the application of MeJA at 0.1 µM did not induce stomatal closure in WT plants (P = 0.28 at 0.1 µM) (Fig. 2). In AER-OE plants, MeJA treatments did not significantly induce stomatal closure (P1#11: P = 0.08 at 1 µM, P = 0.07 at 10 µM; P1#14: P = 0.17 at 1 µM, P = 0.11 at 10 µM; P1#18: P = 0.59 at 1 µM, P = 0.50 at 10 µM) (Fig. 2). The impairment in the MeJA-induced stomatal closure by AER suggests that RCS are involved in the MeJA signaling for stomatal closure.
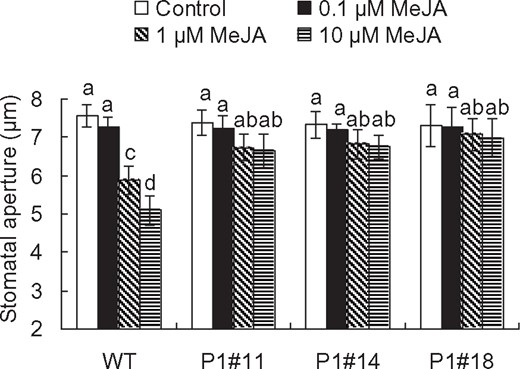
MeJA-induced stomatal closure in tobacco. MeJA-induced stomatal closure in WT and AER-OE plants P1#11, P1#14 and P1#18. Excised leaves were treated with MeJA for 2 h. Averages from three independent experiments (60 total stomata per bar) are shown. Error bars represent standard error of mean. Difference among treatments was analyzed by Tukey’s test: P < 0.05.
To confirm that RCS is involved in the MeJA-induced stomatal closure, we scrutinized the MeJA-induced stomatal closure of WT tobacco plants and Arabidopsis plants using the RCS scavengers, carnosine and pyridoxamine. Both carnosine at 1 mM and pyridoxamine at 0.5 mM inhibited the MeJA-induced stomatal closure in tobacco (carnosine: P < 0.01 for 1 µM MeJA, P < 0.005 for 10 µM MeJA; pyridoxamine: P < 0.03 for 1 µM MeJA, P < 0.006 for 10 µM MeJA) (Fig. 3A, B). Carnosine at 1 mM and pyridoxamine at 0.5 mM also inhibited MeJA-induced stomatal closure in A. thaliana (carnosine: P < 0.04 for 1 µM MeJA, P < 0.04 for 10 µM MeJA; pyridoxamine: P < 0.01 for 1 µM MeJA, P < 0.006 for 10 µM MeJA) (Fig. 3C, D). It should be noted that RCS scavengers did not affect stomatal apertures in the absence of MeJA (tobacco: P = 0.8 for carnosine, P = 0.9 for pyridoxamine; Arabidopsis: P = 0.9 for carnosine, P = 0.9 for pyridoxamine) (Fig. 3A–D). Taken together, these results suggest that RCS is involved in MeJA-induced stomatal closure.
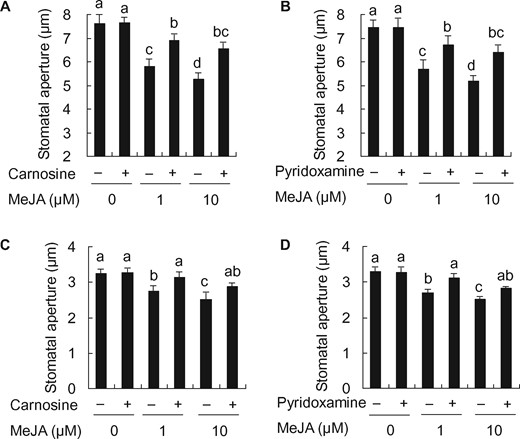
Effects of RCS scavengers, carnosine and pyridoxamine, on MeJA-induced stomatal closure in tobacco and Arabidopsis. Effects of 1 mM carnosine (A) and 0.5 mM pyridoxamine (B) on MeJA-induced stomatal closure in WT tobacco. Effects of 1 mM carnosine (C) and 0.5 mM pyridoxamine (D) on MeJA-induced stomatal closure in Arabidopsis. Averages from three independent experiments (60 stomata per bar) are shown. Error bars represent standard error of mean. Differences among treatments were analyzed by Tukey’s test: P < 0.05.
MeJA-induced production of RCS
To clarify that RCS production occurs downstream of H2O2 production in guard cell MeJA signaling, we quantified RCS production in epidermal tissues of tobacco leaves treated with MeJA for 30 min using a reverse-phase high-performance liquid chromatography (HPLC) after derivatization with 2,4-dinitrophenylhydrazine (DNPH). Typical chromatograms for the epidermal tissues treated with MeJA are shown in Fig. 4A. Treatment with 50 µM MeJA significantly increased the contents of acrolein by 2 nmol/g FW (Fig. 4A, B) and HNE by 5 nmol/g FW (Fig. 4A, C) in the epidermal tissues of WT and also significantly increased the contents of 4-hydroxy-(E)-2-hexenal, (E)-2-pentenal, (E)-2-heptenal, (Z)-3-hexenal, formaldehyde, acetaldehyde, propionaldehyde, n-hexanal, n-heptanal, n-octanal and acetone in the epidermal tissues of WT (Figs. 4A, 5). These results indicate that MeJA induces RCS production in the epidermal tissues of tobacco leaves. The increment in concentrations of acrolein and HNE in the epidermal tissues treated with MeJA can be estimated at approximately 2 and 5 µM, respectively, assuming that 1 g of fresh weight is equivalent to 1 ml. Considering that these values are average concentrations in the epidermal tissues, actual increment in the levels of these aldehydes in guard cells should be >20 µM because MeJA induces detectable ROS production only in guard cells but not in other epidermal cells and because guard cells occupy <10% (v/v) of the epidermal tissue used in this experiment.
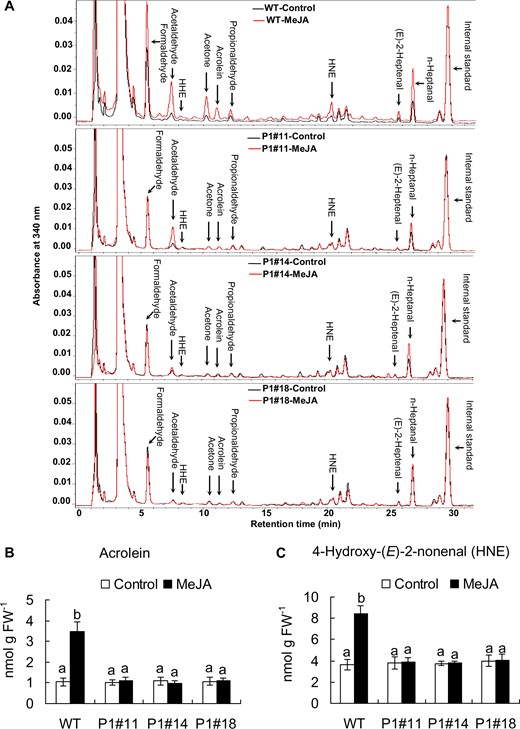
Typical chromatograms for the epidermal tissues treated with or without 50 µM MeJA for 30 min. (A) Typical chromatograms of the DNP derivatives of RCS extracted from control (black lines) and MeJA-treated (red lines) epidermal tissues of WT and AER-OE plants P1#11, P1#14 and P1#18. Identified RCS are labeled at the top of each peak. DNP derivatives of RCS were detected at 340 nm. (B) Contents of acrolein in the epidermal tissues of WT, P1#11, P1#14 and P1#18. (C) Contents of HNE in the epidermal tissues of WT, P1#11, P1#14 and P1#18. Error bars represent standard error of mean (n = 5). Differences among treatments were analyzed by Tukey’s test: P < 0.05.
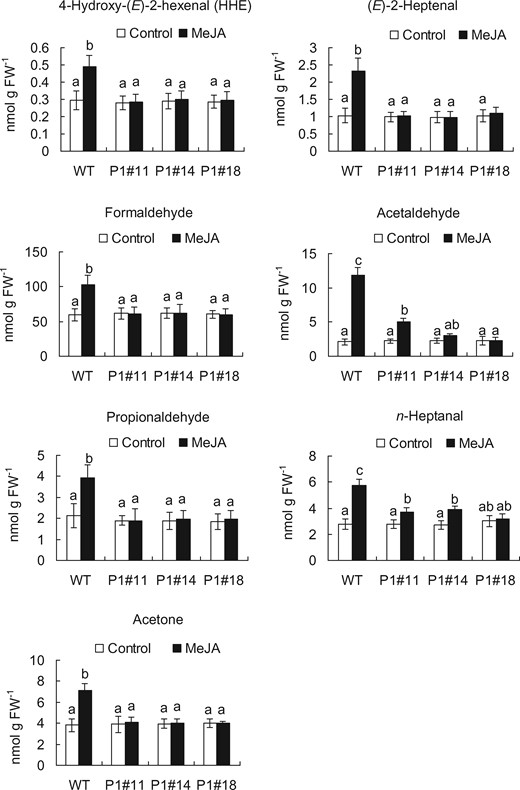
Content of RCS in the epidermal tissues of WT and AER-OE plants P1#11, P1#14 and P1#18 treated with or without 50 µM MeJA for 30 min. Excised leaves of 5- to 7-week-old tobacco plants were blended for 25 s, and epidermal tissues were collected and floated on a stomatal bioassay buffer solution containing 5 mM KCl, 50 µM CaCl2 and 10 mM MES-Tris, pH 6.15, for 2 h in the light followed by the addition of MeJA. Error bars represent standard error of mean (n = 5). Differences among treatments were analyzed by Tukey’s test: P < 0.05.
We measured RCS contents in the epidermal tissues from AER-OE leaves. Treatment with MeJA did not significantly increase the contents of most species of RCS, including acrolein and HNE, in the epidermal tissues from AER-OE leaves (P1#11, P1#14 and P1#18) (Figs. 4, 5). These results along with the stomatal assay data (Figs. 2, 3) suggest that MeJA-induced stomatal closure is accompanied by RCS production.
MeJA-induced H2O2 production
We examined MeJA-induced H2O2 production in the tobacco AER-OE guard cells using an H2O2-sensitive fluorescence dye, 2′,7′-dichlorodihydrofluorescein diacetate (H2DCF-DA). Treatment with 50 µM MeJA induced H2O2 production in the guard cells of WT (P < 10−3) and the three AER-OE plants, P1#11 (P < 10−3), P1#14 (P < 10−3) and P1#18 (P < 10−3) (Fig. 6), indicating that scavenging RCS by AER did not affect MeJA-induced H2O2 production. Taken together with the result that MeJA-induced stomatal closure was inhibited in the AER-OE plants (Fig. 2), these results suggest that RCS is generated downstream of ROS production in the MeJA signal pathway in the guard cells.
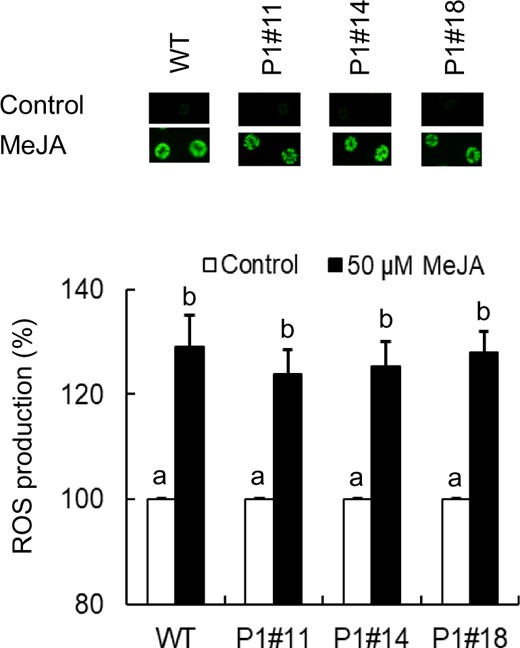
MeJA-induced H2O2 production in guard cells of WT and AER-OE plants P1#11, P1#14 and P1#18. Effects of 50 µM MeJA on H2O2 production in guard cells of WT, P1#11, P1#14 and P1#18. The vertical scale represents the percentage of H2DCF-DA fluorescent levels when the fluorescent intensities obtained from MeJA-treated cells are normalized to control value taken as 100% (white bars). Bars indicate averages of three independent experiments (at least 60 guard cells). Error bars represent standard error of mean. Note that the error bars for control are smaller than the vertical columns. Difference among treatments was analyzed by Tukey’s test: P < 0.05.
To further clarify that RCS is generated downstream of ROS production in the MeJA signal pathway in the guard cells, we tested the effects of RCS scavengers on MeJA-induced H2O2 production in WT tobacco and Arabidopsis guard cells. Neither carnosine nor pyridoxamine suppressed the MeJA-induced H2O2 production in the guard cells of WT tobacco (P < 10−3 for carnosine, P < 10−3 for pyridoxamine) and Arabidopsis (P < 10−3 for carnosine, P < 10−3 for pyridoxamine) (Fig. 7).
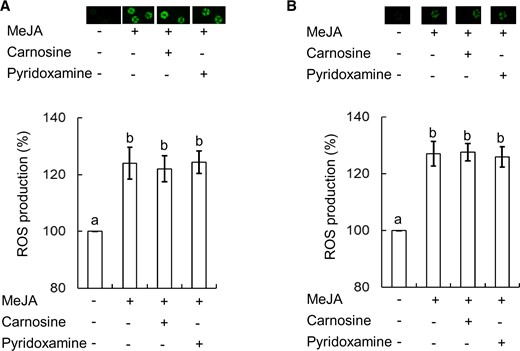
Effects of RCS scavengers on MeJA-induced H2O2 production in tobacco and Arabidopsis. (A) Effects of RCS scavengers, carnosine and pyridoxamine, on MeJA-induced H2O2 production in WT tobacco guard cells. (B) Effects of RCS scavengers, carnosine and pyridoxamine, on MeJA-induced H2O2 production in Arabidopsis guard cells. The vertical scale represents the percentage of H2DCF-DA fluorescent levels when fluorescent intensities of MeJA-treated cells are normalized to control value taken as 100%. Bars indicate averages of three independent experiments (at least 60 guard cells). Error bars represent standard error of mean. Note that the error bar for control is smaller than the vertical column. Differences among treatments were analyzed by Tukey’s test: P < 0.05.
Impairment of MeJA-induced [Ca2+]cyt elevation by RCS scavengers in guard cells
The generation of ROS is proposed to trigger the [Ca2+]cyt elevation (Pei et al. 2000, Murata et al. 2001), which is essential for MeJA-induced stomatal closure (Munemasa et al. 2007, Islam et al. 2010). To elucidate the RCS action in the MeJA-induced stomatal closure, we examined the effects of the RCS scavengers, carnosine and pyridoxamine, on MeJA-induced [Ca2+]cyt elevation in Yellow Cameleon 3.6 (YC 3.6)-expressing Arabidopsis guard cells. When guard cells were treated with 10 μM MeJA in the absence of carnosine and pyridoxamine, 68.2% (n = 22) of the guard cells showed [Ca2+]cyt elevations (Fig. 8A, B, G). MeJA at 10 μM induced the [Ca2+]cyt elevation in 54.6% (n = 22) of the guard cells in the presence of 1 mM carnosine (Fig. 8C, G) and the [Ca2+]cyt elevation in 40% (n = 20) of the guard cells in the presence of 0.5 mM pyridoxamine (Fig. 8D, G). These data indicate that carnosine (P < 0.03) and pyridoxiamine (P < 0.02) significantly suppressed MeJA-induced [Ca2+]cyt elevation. Note that spontaneous [Ca2+]cyt elevation was observed in the guard cells treated with carnosine (35%; n = 20; Fig. 8E, G) or pyridoxiamine (22.8%; n = 22; Fig. 8F, G), as well as in the untreated guard cells (control) (27.3%; n = 22; Fig. 8A, G). These results also suggest that RCS functions in the MeJA signaling pathway in guard cells.
![Effects of RCS scavengers on MeJA-induced [Ca2+]cyt elevations in Arabidopsis guard cells. Representative yellow cameleon 3.6 fluorescence emission ratios (535 nm/480 nm) showing [Ca2+]cyt elevations in Arabidopsis guard cells in response to the indicated treatments: (A) 0.1% ethanol (n = 22), (B) 10 µM MeJA (n = 22), (C) 10 µM MeJA + 1 mM carnosine (n = 22), (D) 10 µM MeJA + 0.5 mM pyridoxamine (n = 20), (E) 1 mM carnosine (n = 20) and (F) 0.5 mM pyridoxamine (n = 22). (G) Percentage bar chart showing the number of control (0.1% Ethanol)-, MeJA-, MeJA + carnosine-, MeJA + pyridoxamine-, carnosine- and pyridoxamine-induced [Ca2+]cyt elevations in the Arabidopsis guard cells. The [Ca2+]cyt transient elevations were counted when changes in [Ca2+]cyt ratios were ≥0.1 units from the baseline.](https://oup.silverchair-cdn.com/oup/backfile/Content_public/Journal/pcp/61/10/10.1093_pcp_pcaa107/3/m_pcaa107f8.jpeg?Expires=1748239879&Signature=hfWE2C2TSeA8oTYMjElpaGUWXxHooXB0pxwKNOpfZDNS0lPTFLfmODckbh7v7smCoyr7Q4TgqE5eGivBeh5xy4GJO7bZlo8Dvhm3IqbwhWOjOItXPWO5PfZ6QFIsFLSnnb15jAMNxXIrrYsUVNMcHvkO-XKySd9akRVp7xE-8SPgc7MxEcLkIV8G2UBi~fm-dcxsFUGCy8QDDDAlEFoB1R8-RfQmJz2qUInVVAlzv-2zGIuPMQz1QZtZMsiXuasmouymWniA4Do-BdfH5lh5VZJ~Tz3NL9X6VyFnr1jZy4PuAZuLKoWwe32zQizBsgVwK12yTxuL6piiSf2oLX1lXQ__&Key-Pair-Id=APKAIE5G5CRDK6RD3PGA)
Effects of RCS scavengers on MeJA-induced [Ca2+]cyt elevations in Arabidopsis guard cells. Representative yellow cameleon 3.6 fluorescence emission ratios (535 nm/480 nm) showing [Ca2+]cyt elevations in Arabidopsis guard cells in response to the indicated treatments: (A) 0.1% ethanol (n = 22), (B) 10 µM MeJA (n = 22), (C) 10 µM MeJA + 1 mM carnosine (n = 22), (D) 10 µM MeJA + 0.5 mM pyridoxamine (n = 20), (E) 1 mM carnosine (n = 20) and (F) 0.5 mM pyridoxamine (n = 22). (G) Percentage bar chart showing the number of control (0.1% Ethanol)-, MeJA-, MeJA + carnosine-, MeJA + pyridoxamine-, carnosine- and pyridoxamine-induced [Ca2+]cyt elevations in the Arabidopsis guard cells. The [Ca2+]cyt transient elevations were counted when changes in [Ca2+]cyt ratios were ≥0.1 units from the baseline.
Discussion
MeJA signaling cascades share several signaling components with ABA signaling cascades in guard cells. Like ABA-induced stomatal closure, MeJA-induced stomatal closure is accompanied by the production of ROS, which is mediated by NAD(P)H oxidases in A. thaliana guard cells (Pei et al. 2000, Kwak et al. 2003, Suhita et al. 2004, Munemasa et al. 2007, Islam et al. 2010). Both MeJA-induced stomatal closure and ABA-induced stomatal closure require cytosolic calcium elevation and S-type anion channel activation. Our previous studies using AER-OE tobacco plants (Mano et al. 2005) have demonstrated that RCS mediates the ABA-induced stomatal closure and functions downstream of the H2O2 production in the ABA signaling pathway in the guard cells (Islam et al. 2016, Islam et al. 2019), and this study reveals that RCS is involved in the MeJA signaling pathway in guard cells.
Stomatal closure and RCS accumulation in response to MeJA and H2O2 were disrupted in AER-OE plants but not in WT tobacco (Figs. 2, 4, 5; Islam et al. 2016). Moreover, the MeJA-induced stomatal closure was also inhibited by RCS scavengers in WT tobacco and Arabidopsis (Fig. 3). By contrast, the MeJA-induced H2O2 production was not inhibited either by AER-OE or by the application of RCS scavengers (Figs. 6, 7). Our previous study has demonstrated that RCS induced stomatal closure in the atrbohD atrbohF double mutant plants and that suppression of ROS production by an NAD(P)H oxidase inhibitor diphenylene iodonium chloride impaired RCS production in ABA-treated WT tobacco guard cells (Islam et al. 2016, Islam et al. 2019). These results suggest that MeJA induces RCS production in guard cells via ROS production and that RCS is likely involved in MeJA-induced stomatal closure.
Because of the potent electrophilicity, RCS depletes GSH through conjugation (Davoine et al. 2006). Indeed, the addition of RCS to plant cells causes a rapid drop of GSH pool (Biswas and Mano 2016) and, consequently, it increases the cell’s sensitivity to ROS (Munemasa et al. 2013). MeJA-induced stomatal closure is accompanied by the depletion of intracellular GSH in Arabidopsis guard cells (Akter et al. 2012) and GSH does not affect MeJA-induced ROS production (Akter et al. 2013). These results suggest that RCS production triggered by MeJA induces GSH depletion in guard cells.
CDPKs function as Ca2+ signal transducers and the disruption of CPK6 impaired MeJA-induced stomatal closure (Munemasa et al. 2011). Although CPK6 is a positive regulator of the MeJA-induced stomatal closure, CPK6 is not involved in the MeJA-induced H2O2 accumulation in the guard cells (Munemasa et al. 2011). Our previous study has demonstrated that CPK6 also functions as a positive regulator in the acrolein- and HNE-induced stomatal closure (Islam et al. 2019). Taken together, these results suggest that RCS functions at a point between the ROS production and CPK6 activation in the MeJA signal pathways in guard cells.
Elevation of cytosolic free Ca2+ concentration [Ca2+]cyt is accompanied by MeJA-induced stomatal closure in guard cells (Islam et al. 2010) and Ca2+-permeable cation (ICa) channels are activated by H2O2, which can contribute to the elevation of [Ca2+]cyt in guard cells (Pei et al. 2000). Activation of the ICa channels by H2O2 was enhanced in a GSH-depletion mutant, cad2–1 (Munemasa et al. 2013), and exogenous RCS, such as acrolein, significantly activated ICa channels (Islam et al. 2019). Furthermore, exogenous RCS more effectively initiated [Ca2+]cyt elevation and induced stomatal closure than exogenous H2O2 did (Islam et al. 2019). Taken together, these findings suggest that RCS efficiently potentiates the downstream signal transduction events like the elevation of [Ca2+]cyt in the guard cell MeJA signal pathway, which is negatively regulated by GSH.
Conclusion
The presented results suggest that RCS is involved in MeJA-induced stomatal closure and functions as a signal mediator downstream of H2O2 production in guard cell MeJA signaling.
Materials and Methods
Plant materials and growth conditions
Three independent transgenic tobacco (N. tabacum) plants P1#11, P1#14 and P1#18 overexpressing A. thaliana 2-alkenal reductase (At5g16970) (AER-OE plants; Mano et al. 2005), WT tobacco and Arabidopsis (A. thaliana) WT (Columbia-0) were grown on soil containing 70% (v/v) vermiculite (Asahi-kogyo) and 30% (v/v) Kureha soil (Kureha Chemical) in a growth chamber at 21 ± 2°C and 60% relative humidity with a 16-h light period with 80 µmol m−2 s−1 photon flux density and 8 h of darkness. To provide the plants with necessary nutrients for their growth, water containing a commercial nutrient solution (0.1% Hyponex; Hyponex, Osaka, Japan) was applied to the plant growth tray 2–3 times a week.
RNA extraction and real-time PCR
Total RNA was isolated from isolated GCPs using TRIzol reagent (Invitrogen, Carlsbad, CA, USA). cDNA was prepared from 1 μg of RNA using the PrimeScript RT master mix (Perfect Real Time; Takara Bio) according to the manufacturer’s instructions. PCR was performed with 1 µl of reverse transcription reaction mixture using BIOTAQ DNA polymerase (Bioline). The primer sets used in PCR amplification are as follows: for AER (At5g16970), 5′-ACACTGGACTTTTAGGTATGCCTGG-3′ (F) and 5′-TCAATGCCATTAGGGAAACACC-3′ (R) and for Tobacco ACTIN, 5′-AAGGTTACGCCCTTCCTCAT-3′ (F) and 5′-CATCTGTTGGAAGGTGCTGA-3′ (R). Amplified DNA products were separated using an agarose gel followed by image analysis with LAS-3000 (GE Healthcare).
Measurement of stomatal aperture
Stomatal apertures were measured as described previously (Islam et al. 2016). Excised leaves of 5- to 7-week-old tobacco and 4- to 6-week-old Arabidopsis were floated on a stomatal bioassay buffer solution containing 5 mM KCl, 50 µM CaCl2 and 10 mM 2-(N-morpholino)ethanesulfonic acid (MES) [pH 6.15 adjusted with tris(hydroxymethyl)aminomethane (Tris)]. The leaves were incubated in the light for 2 h to open the stomata. Then, MeJA (Sigma) was added and the leaves were kept in the light for 2 h before measurement. RCS scavengers, carnosine and pyridoxamine, were added 30 min prior to the MeJA application. For the measurement of stomatal apertures, the leaves were shredded in a commercial blender for 30 s, and epidermal tissues were collected using nylon mesh. The collected tissues were mounted on slides and observed under an inverted microscope (Olympus IX71; Tokyo, Japan). The widths of 20 stomatal apertures were measured for each experiment, and the experiment under the same condition was repeated at least three times.
RCS identification and quantification by HPLC
Excised leaves of 5- to 7-week-old tobacco were blended for 25 s, and epidermal tissues were collected and floated on a stomatal bioassay buffer solution containing 5 mM KCl, 50 µM CaCl2 and 10 mM MES, pH 6.15 (adjusted with Tris), for 2 h in the light followed by the addition of 50 µM MeJA for 30 min. Then, epidermal tissues were used for RCS analysis. RCS was extracted from the epidermal tissues and derivatized with DNPH and then identified and quantified by reverse-phase HPLC according to the method (Islam et al. 2016). We identified dinitrophenylhydrazone derivatives of RCS by their retention times and determined their contents by comparison with authentic compounds (Matsui et al. 2009).
Measurement of H2O2
Production of H2O2 in guard cells was analyzed using H2DCF-DA (Sigma, St. Louis, MO, USA) as described previously (Islam et al. 2016). The epidermal peels were incubated for 3 h in the stomatal bioassay buffer solution containing 5 mM KCl, 50 µM CaCl2 and 10 mM MES (pH 6.15 adjusted with Tris), and then, 50 µM H2DCF-DA was added to the sample. The epidermal tissues were incubated for 30 min at room temperature, and then, the excess dye was washed out using the stomatal bioassay buffer solution. Collected tissues were again incubated with the solution and 50 µM MeJA for 20 min in the dark condition. The image was captured under a fluorescence microscope (Bio Zero BZ-8000; KEYENCE), and the pixel intensity of the fluorescence in guard cells was measured using ImageJ 1.42q (National Institutes of Health, Bethesda, MD, USA).
Measurement of [Ca2+]cyt
Four- to six-week-old leaves of Arabidopsis YC3.6-expressing plants were employed to monitor cytosolic free Ca2+ concentration ([Ca2+]cyt) in Arabidopsis guard cells as described previously (Munemasa et al. 2011). The excised leaves were gently mounted on a glass slide using a medical adhesive. After the adaxial epidermis and the mesophyll tissue were removed, the remaining abaxial epidermal peels were incubated in a solution consisted of 5 mM KCl, 50 µM CaCl2 and 10 mM MES-Tris (pH 6.15) for 2 h under the light. The turgid guard cells were treated with 10 µM MeJA at 5 min after the start of measurement. Six to eight guard cells from one sample leaf for each experiment were selected. This experiment was repeated using different sample leaves on different days. The monitoring of YC3.6 was done by dual-emission ratio imaging. For the imaging of the dual-emission ratio, we used a 440AF21 excitation filter, a 445DRLP dichroic mirror and two emission filters, 480DF30 for cyan fluorescent protein (CFP) and 535DF25 for yellow fluorescent protein (YFP). The CFP and YFP fluorescent intensities of guard cells were measured and analyzed using AQUA COSMOS software (Hamamatsu Photonics).
Statistical analysis
The significance of differences between mean values was assessed by Tukey’s test in all parts of this article. We regarded differences at the level of P < 0.05 as significant.
Funding
Grants-in-Aid for Japan Society for the Promotion of Science Fellows and Grants-In-Aid for Scientific Research (C) No. 26440149 (to J.M.) from the Japan Society for the Promotion of Science.
Disclosures
The authors have no conflicts of interest to declare.