-
PDF
- Split View
-
Views
-
Cite
Cite
Stephen A Rawlings, Brianna Scott, Laura Layman, Pramod Naranatt, Roy Heltsley, Caroline Ignacio, Magali Porrachia, Sara Gianella, Davey Smith, Antoine Chaillon, Can’t Work From Home: Pooled Nucleic Acid Testing of Laboratory Workers During the COVID-19 Pandemic, Open Forum Infectious Diseases, Volume 8, Issue 7, July 2021, ofab129, https://doi.org/10.1093/ofid/ofab129
- Share Icon Share
Abstract
Together with protective measures, routine screening for severe acute respiratory syndrome coronavirus 2 infection helps provide a safe working environment. We evaluated a pooled nucleic acid testing strategy in a research laboratory. It allowed lab activity to be maintained and would save 25 920 person-hours and $1 684 800/year by increasing the margin of safety for returning to work.
Many businesses across the United States have temporarily closed down to help reduce surges in hospitalizations [1]. As the US economy restarts, methods must be developed to protect employees in the workplace. This includes biomedical laboratories working on severe acute respiratory syndrome coronavirus 2 (SARS-CoV-2), as 1 infected employee could cause the entire lab to be shut down for weeks. This is because all employees in contact with the index case may need to be quarantined for at least 2 weeks. The process of quarantining after potential exposure can also reduce workforce capacity in essential service settings, such as hospitals, clinics, police stations, and fire departments. Regular screening could theoretically decrease the risk of workplace infections, but individual tests are in short supply, costly, and not always performed in real time to ascertain asymptomatic infections and thus impact viral spread.
A pooling strategy could represent a solution to the testing supply issue because biological specimens from multiple persons are combined into a testing pool and tested via a single test. This is also a cost-effective approach for routine screening of a population where suspicion of a positive is very low. A similar approach has been used to the monitoring of persons with HIV [2]. Pooling approaches for SARS-CoV-2 screening began to be granted Emergency Use Authorization from the US Food and Drug Administration (FDA) in July of 2020 [3]. Pooling approaches generally have used pools of 4 samples based on initial guidance by the FDA [4, 5], but pools as large as 10–15 have also been assessed [6, 7].
This study evaluated pooled nucleic acid testing (NAT) strategies to screen for SARS-CoV-2 infection in laboratory employees. Such pooled NAT strategies have been used for screening for acute HIV across communities [8] and in blood donors for HIV and HCV [9]. This pooled NAT strategy was implemented in a new point-of-care, low-complexity NAT platform (Fluxergy, Inc., Irvine, CA, USA).
METHODS
Patient Consent Statement
The study was conducted under a protocol for collecting samples from persons with known or possible SARS-CoV-2 infection approved by the Institutional Review Board of the University of California San Diego (UCSD). Written informed consent was obtained from all participants involved in the study. Laboratory employees had the option to enroll in the study but were not required to participate.
Sample Collection, Pooling, and Testing
The study took place in an academic virology laboratory at UCSD. Participants in the study were offered the choice to perform their own anterior nasal swab (self-swabbing) or have an anterior nasal swab collected by an on-site physician.
The Fluxergy platform was evaluated in the lab by comparing results with the RT-PCR Diagnostic Panel authorized for Emergency Use Authorization [10]. The limit of detection was also evaluated before the study and was estimated to be 2.4 copies/μL (Supplementary Data). Swabbing was offered to all laboratory research personnel between April 9 and December 22, 2020. The sampling and testing protocol was designed as follows (Figure 1).
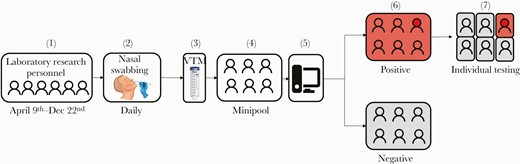
Study schematic. 1. Nasal swabbing was offered to all laboratory research personnel between April 9 and 24, 2020. 2. Nasal swabs (NS) were collected on a daily basis for all eligible participants (ideally at the beginning of their shift). 3. After collection, the NS was placed in viral transport media (VTM). 4. VTM of all participants from the same shift were combined into 1 pool (“minipool”). 5. Each minipool underwent testing in the Fluxergy 1-hour platform. 6. If a minipool test was positive, all samples from individuals who provided NS samples for that pool were tested individually (7).
Nasal swabs were collected on a daily basis for all eligible participants at the beginning of their respective shift (2 daily shifts). Polyester flocked swabs were used in all nasal swabs (COPAN, Murrieta, CA, USA).
After collection, the nasal swabs were placed in 3 mL of viral transport media (VTM).
Equal volumes (14 µL) of VTM from each participant in the same shift were combined into 1 pool (“minipool”). The remaining VTM was stored individually for subsequent testing should the pool require deconvoluting.
Each minipool underwent testing in the Fluxergy platform (Irvine, CA, USA), which is currently available as a Research Use Only (RUO) or Investigational Use Only (IUO) device for the development of new diagnostic products. This platform was chosen because of its quick turnaround time and simplicity of use.
If a minipool test was positive, all samples from that pool were tested individually.
Experimental pools were also evaluated to determine how many samples could be pooled together to identify a positive nasal swab in the pool.
RESULTS
On day 1 (April 9), a laboratory technician reported that a member of their household tested positive for SARS-CoV-2 infection. This index case was asymptomatic at the time. All 7 members of the laboratory at work that day, including the index case, volunteered for screening for SARS-CoV-2 infection by individual and pooled NAT. The minipool of 7 nasal swab samples was found to be positive within an hour of swabbing, and individual testing of each specimen confirmed positivity for only 1 sample, while a minipool of 6 without the index case was negative. The index case was sent home to follow up with their primary care provider and for self-isolation.
Lab workers reported overall satisfaction with routine self-swabbing because it increased the margin of safety for returning to work, and thus the program of swabbing and pooling continued. From April 9 to December 22, the program was expanded to 18 essential staff in the lab who self-swabbed a median of 3 (95% CI, 1–10) times per week. Pools were regularly scheduled throughout the week (usually Monday, Wednesday, and Friday) and offered in the morning and afternoon to capture 2 shifts throughout the day. Results were routinely available 1.5–2 hours after the start of a shift. Pools were limited to 10 swabs maximum in order to maintain sensitivity, but the average pool size (range) was 6 (2–10). A median of 40 tests per week (95% CI, 13–105) were performed (Figure 2). The essential staff members were tested a median of 90 times (95% CI, 34–122) in the 37-week period. Of the 237 pools, 15 (6%, n = 96 samples) were considered positive, but upon deconvolution all but the very first pool (on April 9) were determined to be false positives. This information was used to optimize the testing platform, which was still investigational at the time of use. False positives were defined as a pool that was positive on initial testing but where no positive was identified after deconvolution and testing of samples individually. As deconvolution could be done in 1–2 hours, no initial steps were taken to send employees home during this testing, as routine measures such as masking and physical distancing were also in place.
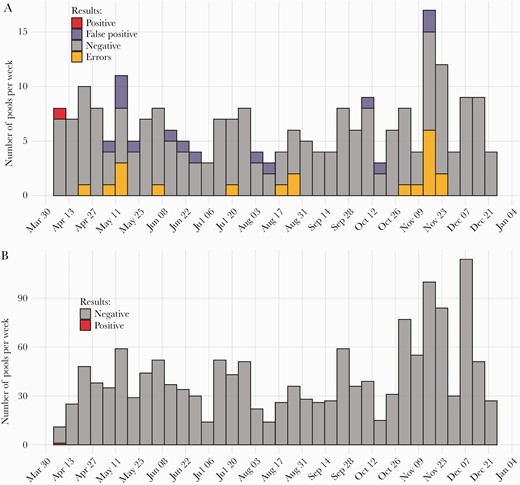
The number of pools (A) and the number of samples processed (B) per week. All false-positive pools were deconvoluted for individual testing. Pools resulting in error messages were repeated for validation.
Pooled NAT Validation
To evaluate the sensitivity of the pooling strategy, we additionally performed an experimental pooling of 30 stored samples collected during the study period, including 1 sample from the index case. Evaluation of pool sizes found that this positive sample from the index case could be detected up to a pool size of 30 samples. As mentioned above, though, pools were limited in size to 10 for both practical (not usually more than 10 employees per shift) and test sensitivity purposes.
Cost Estimates and Budget Impact
When testing is not widely available, the Centers for Disease Control and Prevention currently recommends that all potentially exposed persons self-quarantine for 14 days [11, 12]; thus 1360 hours of laboratory work would have been lost after the positive result on April 9, that is, 17 employees over 170 shifts and 8 hours of work per shift. With an average technician wage of $65/hour, the estimated salary costs would have been $88 400 for this quarantine period. With a current cost per assay, including technician time of $75/assay, the cost of screening the 17 other employees during the same period (2 weeks, 5 days of work per week, 2 shifts per day) following the confirmed positivity of one sample of the first pool after deconvolution, was $2250. Hence, the estimated costs saved by the pooled screening would have been $86 150 for the quarantine period (ie, $88 400 in salary minus $2250 in testing costs). This is assuming the wages would be paid from current funding sources but the work would be lost. The “cost” of quarantine could vary if there were other sources for paying a worker’s salary when quarantined (eg, disability insurance, government stimulus, university general funds, etc.).
Current UCSD guidelines recommend decreasing staffing levels to 25% of pre-COVID occupancy. To allow the safe continuation of all (100%) lab activities, daily screening of the same 18 lab employees over 1 year would cost $42 750 (assuming 1% deconvolution) and would permit 25 920 person-hours of work (34 560 vs 8640 if at 25% capacity), saving $1 684 800 in laboratory wages that might otherwise be lost. A similar approach with individual NAT would cost $648 000 (thus saving $605 250 by pooling). The “lost wages” here assume either that the individuals furloughed by 75% would not be paid for work they did not do or that the employer would continue to pay that 75% but not otherwise have that productivity due to furlough.
DISCUSSION
As the world’s economies seek to re-open and reduce shelter-in-place measures, testing for asymptomatic and presymptomatic carriers will be a critical step for employees in the workplace [13]. Testing, however, can be cost-prohibitive, especially when used for frequent screening of a population with a low incidence of infection. For this reason, pooling of samples from all persons who will be working together can provide a sensitive and cost-efficient method of detecting virus shedding within a work environment. This study found that, in an academic virology laboratory, pooled NAT was acceptable to laboratory employees and that it could save ~$86 000 in laboratory wages in the situation of a single asymptomatic case that would otherwise require 17 individuals to quarantine for 14 days. Importantly, this calculation is based on the guidelines that were in place at the time (April 2020), but quarantine guidelines are updated regularly and savings could depend on the worker type (eg, health care worker vs teacher) as well as clinical situation (eg, where individuals have been vaccinated and are no longer required to quarantine). Additionally, some workplaces are reducing worker density as a precaution, but where working in-person is essential (such as in a research laboratory), using daily, pooled testing to increase the safety margin for working may permit 100% capacity. While there is a cost to this testing, the use of pooling dramatically reduces that cost and is minimal compared with lost wages should work not be done at 100% density.
Importantly, we did not rely on testing alone as the only means to reduce spread of SARS-CoV-2 in our workplace and would encourage others to use a multilayered approach as well. Our university still required—and our work environment complied with—a minimum 6-foot distancing, mask wearing whenever individuals were not eating or at their desk, mandatory symptom screening, and frequent disinfection of surfaces. We consider the testing protocol described here to be an additional layer of protection. We also took very seriously privacy in the workplace and to the best of our ability limited information about test results and who was or was not testing. All participants were assigned study IDs, and only the 2 study physicians (S.A.R. and D.S.) had access to the names of participants. While there is always room for employees to assume someone was positive based on their absence or determine which individuals are in a pool based on who is present for a particular shift, we did not publicly release any test results. When pools were negative, a general announcement in the work area that the morning or afternoon pool was negative would be made, but otherwise no effort was made to single out individuals in a pool to tell them they were negative. The lab did not require testing as a prerequisite to work, and we believe the evidence that the employees saw it as truly optional is in the wide range of tests per participant (95% CI, 34–122 times in the 37-week period of the study). Clearly, some participants opted to test roughly once per week while others tested nearly every time a pool was being constructed.
Based on this small study, true risk reduction in workplace infection could not be ascertained or generalized to other settings. Further, while the Fluxergy platform was used in this study, other NAT platforms could also be used, but their test characteristics would need to be evaluated. Limitations of the study include that there was—thankfully—only 1 true-positive case. As mentioned above, we used viral transport media from the positive case to internally validate that a positive could be detected among up to 29 negatives (ie, a total pool size of 30). The sensitivity of a pooling approach in a group setting such as a workplace is governed by 3 principles: (1) the viral load of any particular infected individual when sampling is done, (2) the consistency with which swabs are performed to collect nasal/nasopharyngeal secretions, and (3) the chance of diluting out (via pooling) any viral RNA collected by a swab below the limit of detection of the machine—which, at this limit, is governed by Poisson distribution dynamics. We felt that through frequent testing (eg, every 1–3 days) we were more likely to capture days when an asymptomatic infection is positive and that repetition of self-swabbing was likely to increase reliability of swabbing via familiarization. Even though swabbing by an on-site physician was offered, all participants opted to self-swab after the first 1–2 swabs. Finally, because we limited our pools to 10 swabs—but had data that a single positive in pools 3 times larger could still be detected—we felt that the loss of sensitivity due to pooling did not affect the results.
With current testing supplies being limited, the pooling of samples from persons who work together or are otherwise in close proximity offers a cost-efficient way to increase the surveillance of a population while enabling progressive deconfinement. In a real work, prospective setting, this study validated a fast, sensitive, and efficient platform for routine testing of SARS-CoV-2 infection. This approach could be applied in other settings to help ensure safe return-to-work procedures. It could be envisioned in high-risk settings such as screening of all health care workers in cancer or HIV clinics or nursing homes or screening essential personnel in police or fire departments.
Supplementary Data
Supplementary materials are available at Open Forum Infectious Diseases online. Consisting of data provided by the authors to benefit the reader, the posted materials are not copyedited and are the sole responsibility of the authors, so questions or comments should be addressed to the corresponding author.
Acknowledgments
The authors acknowledge Fluxergy, Inc.
Financial support. This work was supported by the Department of Veterans Affairs, the John and Mary Tu Foundation, the James B. Pendleton Charitable Trust and the Translational Virology Core of the San Diego Center for AIDS Research (CFAR, AI036214), a National Institutes of Health–funded program. S.A.R. was supported by the National Institutes of Health (5T32AI007384).
Potential conflicts of interest. S.A.R., B.S., L.L., C.I., M.P., S.G., A.C.: no conflicts. P.N. and R.H. are employed by Fluxergy, Inc., which provided the testing platform and supplies. D.S. is a member of the advisory board for Fluxergy, Inc., and Linear Therapies and a consultant for Kiadis, Arena, and Bayer Pharmaceuticals.
Author contributions. S.A.R. performed study procedures, analyzed the data, and reviewed the manuscript. B.S., L.L., M.P., and C.I. performed study procedures and reviewed the manuscript. P.N., R.H., and S.G. analyzed the data and reviewed the manuscript. A.C. and D.S. analyzed the data and wrote the manuscript.
References
Author notes
Equal contribution
Comments