-
PDF
- Split View
-
Views
-
Cite
Cite
Joanna Luczkowiak, Nuria Labiod, Gonzalo Rivas, Marta Rolo, Fátima Lasala, Jaime Lora-Tamayo, Mikel Mancheno-Losa, David Rial, Alfredo Pérez-Rivilla, María D Folgueira, Rafael Delgado, Prime-Boost Vaccination With BNT162b2 Induces High Neutralizing Activity Against SARS-CoV-2 Variants in Naïve and COVID-19-Convalescent Individuals, Open Forum Infectious Diseases, Volume 8, Issue 10, October 2021, ofab468, https://doi.org/10.1093/ofid/ofab468
- Share Icon Share
Abstract
The objective of this study was to investigate the neutralizing response against severe acute respiratory syndrome coronavirus 2 (SARS-CoV-2) variants of concern (VoC) during coronavirus disease 2019 (COVID-19) convalescence and after vaccination.
COVID-19-convalescent and -naïve individuals were tested for neutralizing activity against SARS-CoV-2 VoC Alpha, Beta, Gamma, and Delta at 1 and 7 months postinfection and 4–6 weeks after BNT162b2 vaccination.
Vaccination induced a high neutralizing response in naïve individuals. Interestingly, vaccination of convalescent patients induced a boosted response that was able to neutralize all VoC at high titers.
Vaccination with BNT162b2 induced high levels of neutralization against SARS-CoV-2 VoC in most patients; this is especially beneficial in COVID-19-convalescent individuals.
Circulation of severe acute respiratory syndrome coronavirus 2 (SARS-CoV-2) variants has raised concern as changes in the Spike protein and especially in the RBD sequence are associated with an increased transmissibility and can also determine immune escape to neutralizing antibodies induced by natural infection or vaccination, thus jeopardizing pandemic control. Although reinfection with SARS-CoV-2 is relatively uncommon [1] and protection from vaccines based on original sequences has been demonstrated to be high for most candidates [2], the breadth against infection with variants of concern (VoC) in convalescent individuals and vaccinees is largely unknown and requires continuous investigation. We have aimed to investigate the magnitude and breadth of the neutralizing response against the main circulating SARS-CoV-2 VoC during coronavirus disease 2019 (COVID-19) convalescence and after vaccination.
Patient Consent
The design of the work has been approved by the local ethical committee: Comité Investigación Clínica Hospital Universitario 12 de Octubre (Reference CEIm 20/157). Written consent was obtained from all participants.
METHODS
Within the World Health Organization (WHO) Solidarity II serology network, we have studied the neutralizing response against SARS-CoV-2 in 19 COVID-19 convalescent health care workers (HCWs) from the Hospital Universitario 12 de Octubre in Madrid, Spain. Seventeen had a reverse transcription polymerase chain reaction–documented SARS-CoV-2 infection, 2 had a positive detection of anti-S immunoglobulin G upon entry into the study, and an additional group of 17 HCWs were negative for SARS-CoV-2 detection during follow-up. All cases of COVID-19 were mild to moderate not requiring oxygen therapy or hospitalization. Two HCW were asymptomatic and detected only by serology. All infection events took place during the epidemic wave of COVID-19 affecting Madrid, Spain, during March–April 2020; thus infections were presumably produced with SARS-CoV-2 with the ancestral sequence or the Spike D614G change [3] before the circulation of VoC. Blood samples from convalescent individuals were obtained at 1 and 7 months postinfection (mpi) and from all participants at 4–6 weeks after administration of the second dose of BNT162b2 vaccine [2]. All participants were immunized with BNT162b2 during January–February 2021. Neutralization activity was tested using a SARS-CoV-2-pseudotyped rVSV-luc (PSV) system. PSV were produced following a previously published protocol by co-transfection of BHK-21 with expression vector coding for VSV-G and rVSVΔG-luciferase vector (G*ΔG-luciferase; Kerafast) that contains firefly luciferase instead of the VSV-G open reading frame [4]. The expression vector encoding SARS-CoV-2 Spike_Wuhan-Hu-1 protein was kindly provided by J. Garcia-Arriaza (CNB-CSIC, Madrid, Spain). The SARS-CoV-2 Spike mutant D614G was generated by site-directed mutagenesis using as an input DNA the expression vector encoding SARS-CoV-2 Spike_614D by Q5 Site Directed Mutagenesis Kit (New England Biolabs, Werfen, Barcelona, Spain) following the manufacturer’s instructions. SARS-CoV-2 variants Alpha (B0.1.1.7, GISAID: EPI_ISL_608430) [5], Beta (B0.1.351, GISAID: EPI_ISL_712096) [6], Gamma (P1, GISAID: EPI_ISL_833140) [7], and Delta (B0.1.617.2, GISAID: EPI_ISL_1970335) [8] were synthesized and cloned into pcDNA3.1 by GeneArt technology (Thermo Fisher Scientific GENEART GmbH, Regensburg, Germany). BHK-21 were transfected using the Lipofectamine 3000 protocol (Thermo Fisher Scientific, Madrid, Spain) to express the SARS-CoV-2 Spike protein, and after 24 hours the transfected cells were inoculated with a replication-deficient VSV-G-rVSV-luc pseudotype (MOI: 3). Following 1 hour of incubation at 37°C, the inoculum was removed, cells were washed intensively with PBS, and then the medium added. SARS-CoV-2-pseudotyped rVSV-luc particles were harvested 20 hours postinoculation, clarified from cellular debris by centrifugation, and stored at –80ºC. Infectious titers were estimated as tissue culture infectious dose per mL by limiting dilution of the SARS-CoV-2 rVSV-luc-containing supernatants on Vero E6 cells. Luciferase activity was determined by luciferase assay (Steady-Glo Luciferase Assay System, Promega, Madrid, Spain) in a GloMax Navigator Microplate Luminometer (Promega, Madrid, Spain). Plasma samples from the patients were tested at dilutions of 1: 80, 160, 320, 640, 1280, 2560, 5120, 10240. All samples were heat-inactivated at 56°C for 30 minutes to reduce the risk from any potential residual virus in the plasma. For neutralization experiments, viruses containing transfection supernatants were normalized for infectivity to an MOI of 0.5–1 and incubated with the dilutions of plasma samples at 37°C for 1 hour in 96-well plates. After the incubation time, 2 × 104 Vero E6 cells were seeded onto the virus–plasma mixture and incubated at 37°C for 24 hours. Cells were then lysed and assayed for luciferase expression. Neutralizing titer 50% (NT50) was calculated using a nonlinear regression model fit with settings for log inhibitor vs normalized response curves in GraphPad Prism, version 8. The PSV-based neutralizing methodology was validated trough participation in the SARS-CoV-2 Neutralization Assay Concordance Survey (SNACS) issued by D. Montefiori, Duke Unversity [9]. Statistical analysis was performed by Wilcoxon matched-pair signed-rank test in GraphPad Prism, version 8.
RESULTS
Results are summarized in Figure 1. Levels of neutralizing activity against the reference SARS-CoV-2 S sequence (D614G) in the early convalescent period (1 mpi) were heterogeneous (NT50 geometric mean [GM] = 1/562), as previously described [10]. As compared with the reference sequence, sera from convalescent individuals showed a partial decrease of neutralizing activity against variants Alpha, Gamma, and Delta at 1 mpi (1.5-, 1.9-, and 1.6-fold, respectively) and 7 mpi (1.0, 1.0 and 1.6-fold) that was more prominent against Beta (6.5- and 2.5-fold reduction at 1 and 7 mpi, respectively; both reductions were statistically significant; P < .01) (Figure 1). At these time points, 1 and 7 mpi during convalescence, 7 (36.8%) and 13 (68.4%) out of 19 convalescent individuals had an NT50 below the cutoff of our neutralizing assay (1/66) for the Beta variant. In naïve individuals, vaccination induced a high neutralizing response (GM NT50 1/799 for the reference sequence), and although there was a higher decrease in neutralizing titer against Beta (4.7-fold reduction; GM NT50 = 1/168; P < .01), only 1/17 individuals had neutralization activity below the cutoff. Neutralizing activity was only partially reduced against Alpha, Gamma, or Delta (1.6-, 1.5-, and 2.2-fold reduction, respectively). Interestingly, vaccination of convalescent individuals induced an important boosting response able to neutralize all variants at high titers (GM NT50 = 1/4894; 8.7-fold of the levels at the acute convalescent phase against the reference sequence), and again, although significantly reduced (2.8-fold; P < .01) as compared with control, neutralizing titer for Beta VoC was considerable (GM NT50 1/1740).
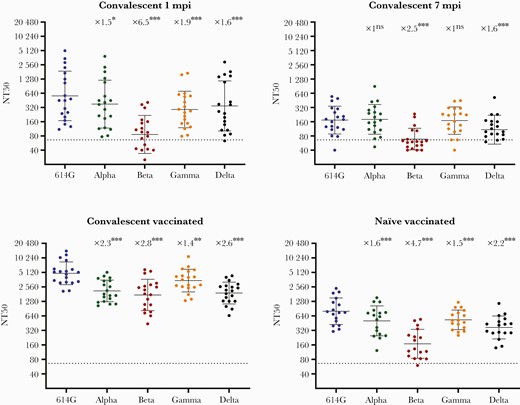
Plasma neutralizing levels (NT50) against SARS-CoV-2: Reference S D614G and VoC Alpha, Beta, Gamma, and Delta during COVID-19 convalescence and after BNT161b2 vaccination. Convalescent individuals (n = 19) were tested at 1 mpi, 7 mpi, and after BNT162b2 vaccination, and a naïve group (n = 17) was tested after BNT162b2 vaccination. Individual NT50 dilution values are presented as reciprocals in a scatter dot plot. Solid lines correspond to the geometric mean. Error bars indicate the geometric SD. Dashed lines mark the cutoff titer (NT50 = 1/66). NT50 was calculated from individual results obtained in triplicate using a nonlinear regression model fit with settings for log inhibitor vs normalized response curve by GraphPad Prism, version 8. Fold decreases in NT50 as compared with SARS-CoV-2 S D614G, together with statistical significance, are indicated above scatter dot results for each variant. Statistical analysis was performed by Wilcoxon matched-pair signed-rank test in GraphPad Prism, version 8. *P < .05; **P < .01; ***P ≤ .001. Abbreviations: COVID-19, coronavirus disease 2019; mpi, months postinfection; ns, not significant; SARS-CoV-2, severe acute respiratory syndrome coronavirus 2.
DISCUSSION
The level of neutralizing antibodies is the main surrogate marker for vaccine efficacy [11]; however, in COVID-19 the correlates for protection for the different vaccines are currently unknown; it is also unknown whether a certain reduction in neutralizing levels can determine a lack of protection against VoC [12]. Also, memory B- and T-cell responses are thought to play an important role as severe COVID-19 develops within a time frame that allows their activation and effector functions [13]. Nevertheless, the effective production of neutralizing antibodies requires specific CD4+ T-cell cooperation, and the levels of neutralizing response could recapitulate significant functions of both humoral and cellular immunity; furthermore, it has been proposed that neutralizing activity could be a reliable marker for vaccine protection [14]. Circulating SARS-CoV-2 VoC present changes in the spike RBD region that allow a higher transmissibility and immune-scape through mutations, such as S E484K, in epitopes frequently targeted by neutralizing antibodies [15]. This is clearly evident for SARS-CoV-2 variant Beta as sera from convalescent individuals frequently lack a measurable neutralizing activity against this VoC as it has been described [16] and as our own data verify (Figure 1). Immunization with the mRNA vaccine BNT162b2 induced a more homogenous response in naïve individuals than in natural infection and relatively high levels of neutralization against VOC Alpha and Gamma and also against the recently characterized Delta variant. This is particularly important as Delta is rapidly spreading globally, and a reduction of neutralizing response by convalescent or vaccinee sera has also been described [17]. We found a modest reduction of NT50 (2.2-fold; P < .01) in naïve vaccinees against the Delta VoC, and all vaccinees had a significant neutralizing level 4–6 weeks after vaccination (GM NT50 1/369). The Beta VoC exhibited the highest reduction in neutralization levels (4.7-fold) after vaccination in naïve individuals, although the majority (16/17) had a detectable neutralizing response (GM NT50 1/168). As has been described, in COVID-19-convalescent individuals vaccination elicited production of high titers of wide-breadth cross-neutralizing antibodies showing high neutralizing levels against VoC [18]. This high neutralizing response includes also the Delta variant (GM NT 1/1907). Whether this is related to the occurrence of affinity maturation of antibodies long after acute infection, as has been recently demonstrated [19, 20], and whether vaccination is stimulating the production of a repertoire of antibodies with higher affinity and breadth remain to be investigated. Nevertheless, our results support the beneficial effect of vaccination for convalescent patients due to this boosting neutralizing response against VoC. We also found a high neutralizing response after prime-boosting vaccination with BNT162b2 in naïve individuals with a reasonable coverage of SARS-CoV-2 VoC Alpha, Beta, Gamma, and Delta at 4–6 weeks after immunization. This neutralizing response against the present or future diversity of SARS-CoV-2 VoC should be monitored over time. Its evolution could have important implications in our understanding of immune protection and in the development of future strategies for immunization.
Acknowledgments
We are greatly thankful to the group of health worker participants for their generous contribution to the study, along with the WHO Solidarity 2 project for supporting and maintaining a great collaborative network.
Financial support. Research in the RD lab is supported by grants from the Instituto de Investigación Carlos III, ISCIII (FIS PI 1801007), by the European Commission Horizon 2020 Framework Programme: Project VIRUSCAN FETPROACT-2016: 731868, and by Fundación Caixa-Health Research HR18-00469 (Project StopEbola).
Potential conflicts of interest. All the authors declare not having any conflict of interest related to this publication. All authors have submitted the ICMJE Form for Disclosure of Potential Conflicts of Interest. Conflicts that the editors consider relevant to the content of the manuscript have been disclosed.
Comments