-
PDF
- Split View
-
Views
-
Cite
Cite
Helio S Sader, Rodrigo E Mendes, John H Kimbrough, Valerie Kantro, Mariana Castanheira, Impact of the Recent Clinical and Laboratory Standards Institute Breakpoint Changes on the Antimicrobial Spectrum of Aminoglycosides and the Activity of Plazomicin Against Multidrug-Resistant and Carbapenem-Resistant Enterobacterales From United States Medical Centers, Open Forum Infectious Diseases, Volume 10, Issue 2, February 2023, ofad058, https://doi.org/10.1093/ofid/ofad058
- Share Icon Share
Abstract
The Clinical and Laboratory Standards Institute (CLSI) lowered the Enterobacterales-susceptible/-resistant breakpoints for amikacin in 2023 from ≤16/≥64 mg/L to ≤4/≥16 mg/L and the breakpoints for gentamicin and tobramycin from ≤4/≥16 mg/L to ≤2/≥8 mg/L. Because aminoglycosides are frequently used to treat infections caused by multidrug-resistant (MDR) and carbapenem-resistant Enterobacterales (CRE), we evaluated the impact of these changes on the susceptibility rates (%S) of Enterobacterales collected from US medical centers.
A total of 9809 Enterobacterales isolates were consecutively collected (1/patient) from 37 US medical centers in 2017–2021 and susceptibility was tested by broth microdilution. Susceptibility rates were calculated using CLSI 2022, CLSI 2023, and US Food and Drug Administration 2022 criteria. Aminoglycoside-nonsusceptible isolates were screened for genes encoding aminoglycoside-modifying enzymes (AMEs) and 16S rRNA methyltransferases (16RMT).
The CLSI breakpoint changes mostly affected amikacin, especially against MDR (94.0%S to 71.0%S), extended-spectrum β-lactamase (ESBL)-producing (96.9%S to 79.7%S), and CRE (75.2%S to 59.0%S) isolates. Plazomicin was active against 96.4% of isolates and retained potent activity against CRE (94.0%S), ESBL-producing (98.9%S), and MDR (94.8%S) isolates. Gentamicin and tobramycin showed limited activity against resistant subsets of Enterobacterales. The AME-encoding genes and 16RMT were observed in 801 (8.2%) and 11 (0.1%) isolates, respectively. Plazomicin was active against 97.3% of the AME producers.
The spectrum of activity of amikacin against resistant subsets of Enterobacterales was drastically reduced when interpretative criteria based on pharmacokinetic/pharmacodynamic parameters that are currently used to establish breakpoints for other antimicrobials were applied. Plazomicin was markedly more active than amikacin, gentamicin, or tobramycin against antimicrobial-resistant Enterobacterales.
Aminoglycosides are a valuable class of antimicrobial agents for the treatment of serious infections caused by Gram-negative bacteria due to their rapid, concentration-dependent, bactericidal action and ability to act synergistically with other antibiotics. Other favorable characteristics of the aminoglycosides include lack of drug-related allergy, low protein bind, and minimal drug metabolism, plus a negligible interaction with other drugs and the intestinal microbiome of the patient [1]. However, given the potential toxicity of aminoglycosides and the advent of less toxic compounds with potent activity against Gram-negative bacteria such as the third- and fourth-generation cephalosporins and the carbapenems, the clinical use of these compounds declined markedly in the 1980s [2].
Due to improved dosing regimens to minimize toxicities and the emergence and global dissemination of multidrug-resistant (MDR) Gram-negative bacteria, especially carbapenem-resistant Enterobacterales (CRE), the clinical usefulness of aminoglycosides [3] was revisited in the late 1990s. This occurred despite the elevated resistance rates to amikacin, gentamicin, and tobramycin seen in many geographic regions [4]. Moreover, the breakpoints for aminoglycosides, which were published by Clinical and Laboratory Standards Institute (CLSI) until 2022 [5] and are currently published by the US Food and Drug Administration (FDA) [6], were shown to be too high based on recent pharmacokinetic/pharmacodynamic (PK/PD) data; thus, resistance rates to the older generation of aminoglycosides are certainly higher than those being previously reported, especially against CRE and MDR isolates [7, 8].
The main mechanism of aminoglycoside resistance in Enterobacterales is alteration of the drug through the activity of aminoglycoside-modifying enzymes (AMEs). These enzymes have limited the utility of aminoglycosides in the management of infections due to MDR Enterobacterales, including extended-spectrum β-lactamase (ESBL)-producing strains and CRE [9]. The AMEs catalyze the covalent modification of amino or hydroxyl groups on aminoglycoside molecules, resulting in poor binding to the ribosome target and elevating minimum inhibitory concentration (MIC) results to different aminoglycosides depending on the residue and type of modification [10]. The AME-encoding genes are often carried by mobile genetic structures harboring β-lactamases and/or other resistance determinants that can be selected and disseminated concomitantly [10, 11]. In the United States, approximately 90% of aminoglycoside-nonsusceptible Enterobacterales (isolates that are nonsusceptible to amikacin, gentamicin, and/or tobramycin) and >50% of CRE carry at least 1 AME gene [9].
Plazomicin is a new semisynthetic aminoglycoside approved by the US FDA in June 2018 to treat complicated urinary tract infections when limited treatment options are available [12]. The plazomicin molecule contains structural modifications that makes it stable to hydrolysis by the vast majority of AMEs [13, 14]. Plazomicin exhibits potent in vitro activity against Enterobacterales, including both ESBL-producing and CRE isolates. Plazomicin's enhanced Enterobacterales activity is due to its stability to commonly encountered AMEs that compromise the activity of traditional aminoglycosides. However, as with most other aminoglycosides, isolates carrying 16S rRNA methyltransferases exhibit high MIC values for plazomicin [12].
Based on new PK/PD and microbiology data as well as an extensive review of clinical data, CLSI lowered the Enterobacterales-susceptible/-resistant breakpoints for amikacin from ≤16/≥64 mg/L to ≤4/≥16 mg/L and the breakpoints for gentamicin and tobramycin from ≤4/≥16 mg/L to ≤2/≥8 mg/L (Table 1) [15]. Because aminoglycosides are frequently used to treat infections caused by MDR Enterobacterales and CRE, we evaluated the activity of plazomicin and the impact of these breakpoint changes on the susceptibility rates of Enterobacterales collected from US medical centers to the old aminoglycosides.
Aminoglycoside Breakpoints Published by CLSI 2023, CLSI 2022, the US FDA, EUCAST, and USCAST
Antimicrobial . | Breakpoint (Susceptible/Resistant) in mg/L . | ||||
---|---|---|---|---|---|
. | CLSI (2023) . | CLSI (2022) . | US FDAa . | EUCAST . | USCAST . |
Plazomicin | ≤2/≥8 | NA | ≤2/≥8 | NA | ≤4/≥8 |
Amikacin | ≤4/≥16 | ≤16/≥ 64a | ≤16/≥64a | ≤8/≥ 16 | ≤4/≥8 |
Gentamicin | ≤2/≥8 | ≤4/≥ 16a | ≤4/≥16a | ≤2/≥ 4 | ≤2/≥4 |
Tobramycin | ≤2/≥8 | ≤4/≥16a | ≤4/≥16a | ≤2/≥ 4 | ≤2/≥4 |
Antimicrobial . | Breakpoint (Susceptible/Resistant) in mg/L . | ||||
---|---|---|---|---|---|
. | CLSI (2023) . | CLSI (2022) . | US FDAa . | EUCAST . | USCAST . |
Plazomicin | ≤2/≥8 | NA | ≤2/≥8 | NA | ≤4/≥8 |
Amikacin | ≤4/≥16 | ≤16/≥ 64a | ≤16/≥64a | ≤8/≥ 16 | ≤4/≥8 |
Gentamicin | ≤2/≥8 | ≤4/≥ 16a | ≤4/≥16a | ≤2/≥ 4 | ≤2/≥4 |
Tobramycin | ≤2/≥8 | ≤4/≥16a | ≤4/≥16a | ≤2/≥ 4 | ≤2/≥4 |
Abbreviations: CLSI, Clinical and Laboratory Standards Institute; EUCAST, European Committee on Antimicrobial Susceptibility Testing; NA, not applicable; US FDA, United States Food and Drug Administration; USCAST, United States Committee on Antimicrobial Susceptibility Testing.
US FDA recognized the CLSI M100 (2022) standard for amikacin, gentamicin, and tobramycin [6].
Aminoglycoside Breakpoints Published by CLSI 2023, CLSI 2022, the US FDA, EUCAST, and USCAST
Antimicrobial . | Breakpoint (Susceptible/Resistant) in mg/L . | ||||
---|---|---|---|---|---|
. | CLSI (2023) . | CLSI (2022) . | US FDAa . | EUCAST . | USCAST . |
Plazomicin | ≤2/≥8 | NA | ≤2/≥8 | NA | ≤4/≥8 |
Amikacin | ≤4/≥16 | ≤16/≥ 64a | ≤16/≥64a | ≤8/≥ 16 | ≤4/≥8 |
Gentamicin | ≤2/≥8 | ≤4/≥ 16a | ≤4/≥16a | ≤2/≥ 4 | ≤2/≥4 |
Tobramycin | ≤2/≥8 | ≤4/≥16a | ≤4/≥16a | ≤2/≥ 4 | ≤2/≥4 |
Antimicrobial . | Breakpoint (Susceptible/Resistant) in mg/L . | ||||
---|---|---|---|---|---|
. | CLSI (2023) . | CLSI (2022) . | US FDAa . | EUCAST . | USCAST . |
Plazomicin | ≤2/≥8 | NA | ≤2/≥8 | NA | ≤4/≥8 |
Amikacin | ≤4/≥16 | ≤16/≥ 64a | ≤16/≥64a | ≤8/≥ 16 | ≤4/≥8 |
Gentamicin | ≤2/≥8 | ≤4/≥ 16a | ≤4/≥16a | ≤2/≥ 4 | ≤2/≥4 |
Tobramycin | ≤2/≥8 | ≤4/≥16a | ≤4/≥16a | ≤2/≥ 4 | ≤2/≥4 |
Abbreviations: CLSI, Clinical and Laboratory Standards Institute; EUCAST, European Committee on Antimicrobial Susceptibility Testing; NA, not applicable; US FDA, United States Food and Drug Administration; USCAST, United States Committee on Antimicrobial Susceptibility Testing.
US FDA recognized the CLSI M100 (2022) standard for amikacin, gentamicin, and tobramycin [6].
METHODS
Organism Collection
Bacterial isolates were collected via the SENTRY Antimicrobial Surveillance Program in 2017–2021 and sent to JMI Laboratories (North Liberty, IA) for susceptibility testing [16]. Only isolates determined to be the probable cause of infection by local criteria were included in the investigation. A total of 9809 isolates were obtained from patients hospitalized with urinary tract infection (n = 3879; 39.5%), bloodstream infection (n = 2570; 26.2%), pneumonia (n = 1927; 19.6%), intra-abdominal infection (n = 814; 8.3%), skin and skin structure infection (n = 494; 5.0%), and other infections (n = 125; 1.3%). Species identification was confirmed by using standard biochemical tests and/or a MALDI Biotyper (Bruker Daltonics, Billerica, MA), when necessary.
Antimicrobial Susceptibility Testing
Antimicrobial susceptibility testing was performed by the reference broth microdilution method as described in CLSI document M07-A11 [17]. The following antimicrobial classes were tested: aminoglycoside, cephalosporin, carbapenem, β-lactamase inhibitor combination, fluoroquinolone, glycylcycline, and polymyxin. Susceptibility rates were calculated using both the 2022 CLSI [5] and 2022 US FDA [6] breakpoints as well as the recently revised 2023 CLSI breakpoints for aminoglycosides [15].
Resistant Subsets
The CRE isolates were defined as displaying imipenem or meropenem MIC values of ≥4 mg/L. Imipenem was not applied to Proteus mirabilis or indole-positive Proteeae due to their intrinsically elevated MIC values. Multidrug-resistant Enterobacterales was defined as nonsusceptible to at least 1 drug from ≥3 classes and extensively drug resistant (XDR) as susceptible to ≤2 classes per 2022 CLSI criteria (Table 1) [18].
Molecular Characterization of Resistance Mechanisms
Escherichia coli, Klebsiella spp, Proteus spp, and Enterobacter spp isolates resistant to amikacin, gentamicin, and/or tobramycin per 2022 CLSI criteria were screened for the presence of genes encoding AMEs and 16S rRNA methyltransferases by whole genome sequencing, as previously described [9]. In addition, E coli and Klebsiella spp isolates displaying MIC values of ≥2 mg/L for at least 2 of the β-lactams (aztreonam, cefepime, ceftazidime, or ceftriaxone) as well as all Enterobacterales isolates displaying carbapenem MIC values ≥2 mg/L for imipenem and/or meropenem were screened for β-lactamase-encoding genes, including narrow spectrum, ESBL, transferrable (plasmid-mediated) AmpC, and carbapenemases [19]. Moreover, all Enterobacterales isolates with plazomicin MIC results ≥128 mg/L were screened for the presence of 16S rRNA methyltransferase-encoding genes.
RESULTS
Overall susceptibility rates were 96.4% for plazomicin (MIC50/90, 0.5/1 mg/L), 94.6% for amikacin (MIC50/90, 2/4 mg/L), 90.6% for gentamicin (MIC50/90, 0.5/2 mg/L), and 88.0% for tobramycin (MIC50/90, 0.5/4 mg/L) based on 2023 CLSI breakpoint criteria (Table 2). The MIC distributions for amikacin and plazomicin against the Enterobacterales collection and key resistant subsets are shown in Supplementary Table 1. The most active compounds from the other antimicrobial classes were meropenem (MIC50/90, 0.03/0.06 mg/L; 98.7% susceptible) and tigecycline (MIC50/90, 0.25/1 mg/L; 96.6% susceptible per FDA) (Table 2).
Activity of Plazomicin and Comparator Antimicrobial Agents Tested Against Enterobacterales Isolates From US Medical Centers in 2017–2021
Antimicrobial Agent . | mg/L . | Clsi (2023)a . | Us fdab . | |||
---|---|---|---|---|---|---|
. | Mic50 . | Mic90 . | %s . | %r . | %s . | %r . |
Enterobacterales (9809)c | ||||||
Plazomicin | 0.5 | 1 | 96.4 | 0.8 | 96.4 | 0.8 |
Amikacin | 2 | 4 | 94.6 | 1.4 | 99.4d | 0.2 |
Gentamicin | 0.5 | 2 | 90.6 | 8.5 | 91.5d | 7.7 |
Tobramycin | 0.5 | 4 | 88.0 | 9.4 | 90.6d | 6.1 |
Cefepime | ≤0.12 | 8 | 88.4 | 9.4 | 88.4 | 9.4 |
Ceftazidime | 0.25 | 16 | 86.0 | 12.3 | 86.0d | 12.3 |
Ceftriaxone | ≤0.06 | >8 | 82.3 | 16.9 | 82.3d | 16.9 |
Imipenem | ≤0.12 | 1 | 92.4 | 2.1 | 92.4d | 2.1 |
Meropenem | 0.03 | 0.06 | 98.7 | 1.0 | 98.7d | 1.0 |
Piperacillin-tazobactam | 2 | 16 | 88.0 | 7.9 | 88.0d | 7.9 |
Levofloxacin | 0.06 | 8 | 80.3 | 16.4 | 80.3d | 16.4 |
Tigecycline | 0.25 | 1 | … | … | 96.6 | 0.4 |
Colistin | 0.25 | >8 | [84.7]e | 15.3 | … | … |
CRE (117)f | ||||||
Plazomicin | 0.25 | 1 | 94.0 | 5.1 | 94.0 | 5.1 |
Amikacin | 4 | 32 | 59.0 | 31.6 | 75.2d | 8.5 |
Gentamicin | 4 | >16 | 47.0 | 43.6 | 56.4d | 35.9 |
Tobramycin | 16 | >16 | 27.4 | 70.1 | 29.9d | 61.5 |
Levofloxacin | 16 | >16 | 14.5 | 78.6 | 14.5d | 78.6 |
Tigecycline | 0.5 | 2 | … | … | 94.9 | 0.9 |
Colistin | 0.25 | 0.5 | [91.4]e | 8.6 | … | … |
ESBL Producers (1011)g | ||||||
Plazomicin | 0.5 | 1 | 98.9 | 0.9 | 98.9 | 0.9 |
Amikacin | 4 | 8 | 79.7 | 8.4 | 96.9d | 1.3 |
Gentamicin | 1 | >16 | 54.5 | 44.3 | 55.7d | 42.2 |
Tobramycin | 8 | >16 | 40.4 | 56.1 | 43.9d | 41.7 |
Cefepime | >16 | >16 | 6.4 | 82.9 | 6.4 | 82.9 |
Ceftazidime | 32 | >32 | 15.1 | 73.9 | 15.1d | 73.9 |
Ceftriaxone | >8 | >8 | 0.4 | 98.9 | 0.4d | 98.9 |
Imipenem | ≤0.12 | 0.5 | 94.7 | 4.9 | 94.7d | 4.9 |
Meropenem | 0.03 | 0.12 | 93.5 | 5.5 | 93.5d | 5.5 |
Piperacillin-tazobactam | 8 | 128 | 63.1 | 23.2 | 63.1d | 23.2 |
Levofloxacin | 8 | >16 | 23.2 | 67.2 | 23.2d | 67.2 |
Tigecycline | 0.25 | 1 | … | … | 97.2 | 0.7 |
Colistin | 0.25 | 0.25 | 98.2 | 1.8 | … | … |
AME Producers (801)h | ||||||
Plazomicin | 0.5 | 1 | 97.3 | 1.4 | 97.3 | 1.4 |
Amikacin | 4 | 16 | 68.4 | 13.1 | 94.3d | 2.0 |
Gentamicin | >16 | >16 | 14.0 | 84.8 | 15.2d | 83.3 |
Tobramycin | 16 | >16 | 2.0 | 88.0 | 12.0d | 68.0 |
Cefepime | >16 | >16 | 32.5 | 63.0 | 32.5 | 63.0 |
Ceftazidime | 16 | >32 | 34.0 | 59.8 | 34.0d | 59.8 |
Ceftriaxone | >8 | >8 | 28.0 | 71.5 | 28.0d | 71.5 |
Imipenem | ≤0.12 | 1 | 90.9 | 7.7 | 90.9d | 7.7 |
Meropenem | 0.03 | 0.25 | 91.4 | 7.7 | 91.4d | 7.7 |
Piperacillin-tazobactam | 8 | >128 | 58.9 | 27.4 | 58.9d | 27.4 |
Levofloxacin | 8 | >16 | 29.0 | 63.4 | 29.0d | 63.4 |
Tigecycline | 0.25 | 2 | … | … | 95.5 | 0.7 |
Colistin | 0.25 | 0.25 | [95.7]e | 4.3 | … | … |
Amikacin-Nonsusceptible (528)i | ||||||
Plazomicin | 1 | 4 | 86.4 | 6.4 | 86.4 | 6.4 |
Amikacin | 8 | 32 | 0.0 | 26.5 | 89.4d | 3.6 |
Gentamicin | 2 | >16 | 62.9 | 33.1 | 66.9d | 31.8 |
Tobramycin | 8 | >16 | 42.2 | 54.0 | 46.0d | 49.4 |
Cefepime | 0.5 | >16 | 56.8 | 38.6 | 56.8 | 38.6 |
Ceftazidime | 1 | >32 | 56.1 | 39.6 | 56.1d | 39.6 |
Ceftriaxone | 1 | >8 | 50.8 | 47.3 | 50.8d | 47.3 |
Imipenem | ≤0.12 | 4 | 80.5 | 11.2 | 80.5d | 11.2 |
Meropenem | 0.03 | 0.5 | 90.7 | 8.7 | 90.7d | 8.7 |
Piperacillin-tazobactam | 4 | >128 | 67.6 | 24.4 | 67.6d | 24.4 |
Levofloxacin | 4 | >16 | 42.6 | 54.4 | 42.6d | 54.4 |
Tigecycline | 0.25 | 2 | … | … | 93.4 | 1.7 |
Colistin | 0.25 | >8 | [83.5]e | 16.5 | … | … |
MDR (844)j | ||||||
Plazomicin | 0.25 | 1 | 94.8 | 2.5 | 94.8 | 2.5 |
Amikacin | 4 | 16 | 71.0 | 13.3 | 94.0d | 2.0 |
Gentamicin | >16 | >16 | 33.8 | 63.6 | 36.4d | 59.0 |
Tobramycin | 16 | >16 | 15.2 | 79.9 | 20.1d | 59.1 |
Cefepime | >16 | >16 | 16.7 | 73.5 | 16.7 | 73.5 |
Ceftazidime | 32 | >32 | 11.4 | 79.4 | 11.4d | 79.4 |
Ceftriaxone | >8 | >8 | 4.3 | 93.0 | 4.3d | 93.0 |
Imipenem | ≤0.12 | 4 | 83.2 | 12.9 | 83.2d | 12.9 |
Meropenem | 0.03 | 4 | 85.5 | 12.0 | 85.5d | 12.0 |
Piperacillin-tazobactam | 16 | >128 | 35.8 | 44.0 | 35.8d | 44.0 |
Levofloxacin | 8 | >16 | 16.5 | 72.8 | 16.5d | 72.8 |
Tigecycline | 0.5 | 2 | … | … | 93.5 | 1.2 |
Colistin | 0.25 | 0.5 | [91.4]e | 8.6 | … | … |
XDR (84)k | ||||||
Plazomicin | 0.25 | 1 | 94.0 | 6.0 | 94.0 | 6.0 |
Amikacin | 8 | >32 | 41.7 | 44.0 | 65.5d | 11.9 |
Gentamicin | 8 | >16 | 29.8 | 59.5 | 40.5d | 48.8 |
Tobramycin | >16 | >16 | 0.0 | 100.0 | 0.0d | 86.9 |
Cefepime | >16 | >16 | 1.2 | 90.5 | 1.2 | 90.5 |
Ceftazidime | >32 | >32 | 0.0 | 98.8 | 0.0d | 98.8 |
Ceftriaxone | >8 | >8 | 0.0 | 100.0 | 0.0d | 100.0 |
Imipenem | 8 | >8 | 9.5 | 86.9 | 9.5d | 86.9 |
Meropenem | 16 | >32 | 2.4 | 88.1 | 2.4d | 88.1 |
Piperacillin-tazobactam | >128 | >128 | 0.0 | 100.0 | 0.0d | 100.0 |
Levofloxacin | >16 | >16 | 0.0 | 95.2 | 0.0d | 95.2 |
Tigecycline | 0.5 | 2 | … | … | 94.0 | 1.2 |
Colistin | 0.25 | 0.5 | [92.9]e | 7.1 | … | … |
Antimicrobial Agent . | mg/L . | Clsi (2023)a . | Us fdab . | |||
---|---|---|---|---|---|---|
. | Mic50 . | Mic90 . | %s . | %r . | %s . | %r . |
Enterobacterales (9809)c | ||||||
Plazomicin | 0.5 | 1 | 96.4 | 0.8 | 96.4 | 0.8 |
Amikacin | 2 | 4 | 94.6 | 1.4 | 99.4d | 0.2 |
Gentamicin | 0.5 | 2 | 90.6 | 8.5 | 91.5d | 7.7 |
Tobramycin | 0.5 | 4 | 88.0 | 9.4 | 90.6d | 6.1 |
Cefepime | ≤0.12 | 8 | 88.4 | 9.4 | 88.4 | 9.4 |
Ceftazidime | 0.25 | 16 | 86.0 | 12.3 | 86.0d | 12.3 |
Ceftriaxone | ≤0.06 | >8 | 82.3 | 16.9 | 82.3d | 16.9 |
Imipenem | ≤0.12 | 1 | 92.4 | 2.1 | 92.4d | 2.1 |
Meropenem | 0.03 | 0.06 | 98.7 | 1.0 | 98.7d | 1.0 |
Piperacillin-tazobactam | 2 | 16 | 88.0 | 7.9 | 88.0d | 7.9 |
Levofloxacin | 0.06 | 8 | 80.3 | 16.4 | 80.3d | 16.4 |
Tigecycline | 0.25 | 1 | … | … | 96.6 | 0.4 |
Colistin | 0.25 | >8 | [84.7]e | 15.3 | … | … |
CRE (117)f | ||||||
Plazomicin | 0.25 | 1 | 94.0 | 5.1 | 94.0 | 5.1 |
Amikacin | 4 | 32 | 59.0 | 31.6 | 75.2d | 8.5 |
Gentamicin | 4 | >16 | 47.0 | 43.6 | 56.4d | 35.9 |
Tobramycin | 16 | >16 | 27.4 | 70.1 | 29.9d | 61.5 |
Levofloxacin | 16 | >16 | 14.5 | 78.6 | 14.5d | 78.6 |
Tigecycline | 0.5 | 2 | … | … | 94.9 | 0.9 |
Colistin | 0.25 | 0.5 | [91.4]e | 8.6 | … | … |
ESBL Producers (1011)g | ||||||
Plazomicin | 0.5 | 1 | 98.9 | 0.9 | 98.9 | 0.9 |
Amikacin | 4 | 8 | 79.7 | 8.4 | 96.9d | 1.3 |
Gentamicin | 1 | >16 | 54.5 | 44.3 | 55.7d | 42.2 |
Tobramycin | 8 | >16 | 40.4 | 56.1 | 43.9d | 41.7 |
Cefepime | >16 | >16 | 6.4 | 82.9 | 6.4 | 82.9 |
Ceftazidime | 32 | >32 | 15.1 | 73.9 | 15.1d | 73.9 |
Ceftriaxone | >8 | >8 | 0.4 | 98.9 | 0.4d | 98.9 |
Imipenem | ≤0.12 | 0.5 | 94.7 | 4.9 | 94.7d | 4.9 |
Meropenem | 0.03 | 0.12 | 93.5 | 5.5 | 93.5d | 5.5 |
Piperacillin-tazobactam | 8 | 128 | 63.1 | 23.2 | 63.1d | 23.2 |
Levofloxacin | 8 | >16 | 23.2 | 67.2 | 23.2d | 67.2 |
Tigecycline | 0.25 | 1 | … | … | 97.2 | 0.7 |
Colistin | 0.25 | 0.25 | 98.2 | 1.8 | … | … |
AME Producers (801)h | ||||||
Plazomicin | 0.5 | 1 | 97.3 | 1.4 | 97.3 | 1.4 |
Amikacin | 4 | 16 | 68.4 | 13.1 | 94.3d | 2.0 |
Gentamicin | >16 | >16 | 14.0 | 84.8 | 15.2d | 83.3 |
Tobramycin | 16 | >16 | 2.0 | 88.0 | 12.0d | 68.0 |
Cefepime | >16 | >16 | 32.5 | 63.0 | 32.5 | 63.0 |
Ceftazidime | 16 | >32 | 34.0 | 59.8 | 34.0d | 59.8 |
Ceftriaxone | >8 | >8 | 28.0 | 71.5 | 28.0d | 71.5 |
Imipenem | ≤0.12 | 1 | 90.9 | 7.7 | 90.9d | 7.7 |
Meropenem | 0.03 | 0.25 | 91.4 | 7.7 | 91.4d | 7.7 |
Piperacillin-tazobactam | 8 | >128 | 58.9 | 27.4 | 58.9d | 27.4 |
Levofloxacin | 8 | >16 | 29.0 | 63.4 | 29.0d | 63.4 |
Tigecycline | 0.25 | 2 | … | … | 95.5 | 0.7 |
Colistin | 0.25 | 0.25 | [95.7]e | 4.3 | … | … |
Amikacin-Nonsusceptible (528)i | ||||||
Plazomicin | 1 | 4 | 86.4 | 6.4 | 86.4 | 6.4 |
Amikacin | 8 | 32 | 0.0 | 26.5 | 89.4d | 3.6 |
Gentamicin | 2 | >16 | 62.9 | 33.1 | 66.9d | 31.8 |
Tobramycin | 8 | >16 | 42.2 | 54.0 | 46.0d | 49.4 |
Cefepime | 0.5 | >16 | 56.8 | 38.6 | 56.8 | 38.6 |
Ceftazidime | 1 | >32 | 56.1 | 39.6 | 56.1d | 39.6 |
Ceftriaxone | 1 | >8 | 50.8 | 47.3 | 50.8d | 47.3 |
Imipenem | ≤0.12 | 4 | 80.5 | 11.2 | 80.5d | 11.2 |
Meropenem | 0.03 | 0.5 | 90.7 | 8.7 | 90.7d | 8.7 |
Piperacillin-tazobactam | 4 | >128 | 67.6 | 24.4 | 67.6d | 24.4 |
Levofloxacin | 4 | >16 | 42.6 | 54.4 | 42.6d | 54.4 |
Tigecycline | 0.25 | 2 | … | … | 93.4 | 1.7 |
Colistin | 0.25 | >8 | [83.5]e | 16.5 | … | … |
MDR (844)j | ||||||
Plazomicin | 0.25 | 1 | 94.8 | 2.5 | 94.8 | 2.5 |
Amikacin | 4 | 16 | 71.0 | 13.3 | 94.0d | 2.0 |
Gentamicin | >16 | >16 | 33.8 | 63.6 | 36.4d | 59.0 |
Tobramycin | 16 | >16 | 15.2 | 79.9 | 20.1d | 59.1 |
Cefepime | >16 | >16 | 16.7 | 73.5 | 16.7 | 73.5 |
Ceftazidime | 32 | >32 | 11.4 | 79.4 | 11.4d | 79.4 |
Ceftriaxone | >8 | >8 | 4.3 | 93.0 | 4.3d | 93.0 |
Imipenem | ≤0.12 | 4 | 83.2 | 12.9 | 83.2d | 12.9 |
Meropenem | 0.03 | 4 | 85.5 | 12.0 | 85.5d | 12.0 |
Piperacillin-tazobactam | 16 | >128 | 35.8 | 44.0 | 35.8d | 44.0 |
Levofloxacin | 8 | >16 | 16.5 | 72.8 | 16.5d | 72.8 |
Tigecycline | 0.5 | 2 | … | … | 93.5 | 1.2 |
Colistin | 0.25 | 0.5 | [91.4]e | 8.6 | … | … |
XDR (84)k | ||||||
Plazomicin | 0.25 | 1 | 94.0 | 6.0 | 94.0 | 6.0 |
Amikacin | 8 | >32 | 41.7 | 44.0 | 65.5d | 11.9 |
Gentamicin | 8 | >16 | 29.8 | 59.5 | 40.5d | 48.8 |
Tobramycin | >16 | >16 | 0.0 | 100.0 | 0.0d | 86.9 |
Cefepime | >16 | >16 | 1.2 | 90.5 | 1.2 | 90.5 |
Ceftazidime | >32 | >32 | 0.0 | 98.8 | 0.0d | 98.8 |
Ceftriaxone | >8 | >8 | 0.0 | 100.0 | 0.0d | 100.0 |
Imipenem | 8 | >8 | 9.5 | 86.9 | 9.5d | 86.9 |
Meropenem | 16 | >32 | 2.4 | 88.1 | 2.4d | 88.1 |
Piperacillin-tazobactam | >128 | >128 | 0.0 | 100.0 | 0.0d | 100.0 |
Levofloxacin | >16 | >16 | 0.0 | 95.2 | 0.0d | 95.2 |
Tigecycline | 0.5 | 2 | … | … | 94.0 | 1.2 |
Colistin | 0.25 | 0.5 | [92.9]e | 7.1 | … | … |
Abbreviations: AME, aminoglycoside-modifying enzyme; CLSI, Clinical and Laboratory Standards Institute; CRE, carbapenem-resistant Enterobacteriaceae; ESBL, extended-spectrum β-lactamase; MIC, minimum inhibitory concentration; MDR, multidrug-resistant; %R, percentage resistant; %S, percentage susceptible; US FDA, United States Food and Drug Administration; XDR, extensively drug resistant.
Criteria as published by CLSI [15].
Criteria as published by US FDA [5].
Organisms include Citrobacter freundii (4), C freundii species complex (367), Citrobacter koseri (283), Enterobacter cloacae (89), E cloacae species complex (208), Escherichia coli (3271), Klebsiella aerogenes (305), Klebsiella oxytoca (715), Klebsiella pneumoniae (3185), Klebsiella variicola (1), Morganella morganii (289), Proteus mirabilis (334), Proteus penneri (2), Proteus vulgaris (76), P vulgaris group (99), Providencia alcalifaciens (1), Providencia rettgeri (134), Providencia stuartii (139), Serratia marcescens (302), unspeciated Klebsiella (1), and unspeciated Providencia (4).
The CLSI M100 (2022) standard is recognized by the US FDA [6].
The value in brackets indicates the percentage intermediate; CLSI does not publish a susceptible breakpoint for colistin [15].
Organisms include C freundii species complex (7), C koseri (1), E cloacae species complex (3), E coli (9), K aerogenes (4), K oxytoca (4), K pneumoniae (82), P rettgeri (2), and S marcescens (5).
Organisms include C freundii species complex (3), E cloacae species complex (1), E coli (509), K oxytoca (24), K pneumoniae (471), P vulgaris (1), S marcescens (1), and unspeciated Klebsiella (1).
Organisms include E cloacae species complex (13), E coli (409), K aerogenes (1), K oxytoca (31), K pneumoniae (328), P mirabilis (16), P vulgaris (2), and unspeciated Klebsiella (1).
Based on 2023 CLSI criteria.
Organisms include C freundii species complex (29), C koseri (5), E cloacae species complex (21), E coli (267), K aerogenes (15), K oxytoca (35), K pneumoniae (413), M morganii (19), P mirabilis (6), P vulgaris (1), P rettgeri (4), P stuartii (13), S marcescens (15), and unspeciated Klebsiella (1).
Organisms include C freundii species complex (4), E cloacae species complex (1), E coli (5), K aerogenes (1), K oxytoca (2), K pneumoniae (68), and S marcescens (3).
Activity of Plazomicin and Comparator Antimicrobial Agents Tested Against Enterobacterales Isolates From US Medical Centers in 2017–2021
Antimicrobial Agent . | mg/L . | Clsi (2023)a . | Us fdab . | |||
---|---|---|---|---|---|---|
. | Mic50 . | Mic90 . | %s . | %r . | %s . | %r . |
Enterobacterales (9809)c | ||||||
Plazomicin | 0.5 | 1 | 96.4 | 0.8 | 96.4 | 0.8 |
Amikacin | 2 | 4 | 94.6 | 1.4 | 99.4d | 0.2 |
Gentamicin | 0.5 | 2 | 90.6 | 8.5 | 91.5d | 7.7 |
Tobramycin | 0.5 | 4 | 88.0 | 9.4 | 90.6d | 6.1 |
Cefepime | ≤0.12 | 8 | 88.4 | 9.4 | 88.4 | 9.4 |
Ceftazidime | 0.25 | 16 | 86.0 | 12.3 | 86.0d | 12.3 |
Ceftriaxone | ≤0.06 | >8 | 82.3 | 16.9 | 82.3d | 16.9 |
Imipenem | ≤0.12 | 1 | 92.4 | 2.1 | 92.4d | 2.1 |
Meropenem | 0.03 | 0.06 | 98.7 | 1.0 | 98.7d | 1.0 |
Piperacillin-tazobactam | 2 | 16 | 88.0 | 7.9 | 88.0d | 7.9 |
Levofloxacin | 0.06 | 8 | 80.3 | 16.4 | 80.3d | 16.4 |
Tigecycline | 0.25 | 1 | … | … | 96.6 | 0.4 |
Colistin | 0.25 | >8 | [84.7]e | 15.3 | … | … |
CRE (117)f | ||||||
Plazomicin | 0.25 | 1 | 94.0 | 5.1 | 94.0 | 5.1 |
Amikacin | 4 | 32 | 59.0 | 31.6 | 75.2d | 8.5 |
Gentamicin | 4 | >16 | 47.0 | 43.6 | 56.4d | 35.9 |
Tobramycin | 16 | >16 | 27.4 | 70.1 | 29.9d | 61.5 |
Levofloxacin | 16 | >16 | 14.5 | 78.6 | 14.5d | 78.6 |
Tigecycline | 0.5 | 2 | … | … | 94.9 | 0.9 |
Colistin | 0.25 | 0.5 | [91.4]e | 8.6 | … | … |
ESBL Producers (1011)g | ||||||
Plazomicin | 0.5 | 1 | 98.9 | 0.9 | 98.9 | 0.9 |
Amikacin | 4 | 8 | 79.7 | 8.4 | 96.9d | 1.3 |
Gentamicin | 1 | >16 | 54.5 | 44.3 | 55.7d | 42.2 |
Tobramycin | 8 | >16 | 40.4 | 56.1 | 43.9d | 41.7 |
Cefepime | >16 | >16 | 6.4 | 82.9 | 6.4 | 82.9 |
Ceftazidime | 32 | >32 | 15.1 | 73.9 | 15.1d | 73.9 |
Ceftriaxone | >8 | >8 | 0.4 | 98.9 | 0.4d | 98.9 |
Imipenem | ≤0.12 | 0.5 | 94.7 | 4.9 | 94.7d | 4.9 |
Meropenem | 0.03 | 0.12 | 93.5 | 5.5 | 93.5d | 5.5 |
Piperacillin-tazobactam | 8 | 128 | 63.1 | 23.2 | 63.1d | 23.2 |
Levofloxacin | 8 | >16 | 23.2 | 67.2 | 23.2d | 67.2 |
Tigecycline | 0.25 | 1 | … | … | 97.2 | 0.7 |
Colistin | 0.25 | 0.25 | 98.2 | 1.8 | … | … |
AME Producers (801)h | ||||||
Plazomicin | 0.5 | 1 | 97.3 | 1.4 | 97.3 | 1.4 |
Amikacin | 4 | 16 | 68.4 | 13.1 | 94.3d | 2.0 |
Gentamicin | >16 | >16 | 14.0 | 84.8 | 15.2d | 83.3 |
Tobramycin | 16 | >16 | 2.0 | 88.0 | 12.0d | 68.0 |
Cefepime | >16 | >16 | 32.5 | 63.0 | 32.5 | 63.0 |
Ceftazidime | 16 | >32 | 34.0 | 59.8 | 34.0d | 59.8 |
Ceftriaxone | >8 | >8 | 28.0 | 71.5 | 28.0d | 71.5 |
Imipenem | ≤0.12 | 1 | 90.9 | 7.7 | 90.9d | 7.7 |
Meropenem | 0.03 | 0.25 | 91.4 | 7.7 | 91.4d | 7.7 |
Piperacillin-tazobactam | 8 | >128 | 58.9 | 27.4 | 58.9d | 27.4 |
Levofloxacin | 8 | >16 | 29.0 | 63.4 | 29.0d | 63.4 |
Tigecycline | 0.25 | 2 | … | … | 95.5 | 0.7 |
Colistin | 0.25 | 0.25 | [95.7]e | 4.3 | … | … |
Amikacin-Nonsusceptible (528)i | ||||||
Plazomicin | 1 | 4 | 86.4 | 6.4 | 86.4 | 6.4 |
Amikacin | 8 | 32 | 0.0 | 26.5 | 89.4d | 3.6 |
Gentamicin | 2 | >16 | 62.9 | 33.1 | 66.9d | 31.8 |
Tobramycin | 8 | >16 | 42.2 | 54.0 | 46.0d | 49.4 |
Cefepime | 0.5 | >16 | 56.8 | 38.6 | 56.8 | 38.6 |
Ceftazidime | 1 | >32 | 56.1 | 39.6 | 56.1d | 39.6 |
Ceftriaxone | 1 | >8 | 50.8 | 47.3 | 50.8d | 47.3 |
Imipenem | ≤0.12 | 4 | 80.5 | 11.2 | 80.5d | 11.2 |
Meropenem | 0.03 | 0.5 | 90.7 | 8.7 | 90.7d | 8.7 |
Piperacillin-tazobactam | 4 | >128 | 67.6 | 24.4 | 67.6d | 24.4 |
Levofloxacin | 4 | >16 | 42.6 | 54.4 | 42.6d | 54.4 |
Tigecycline | 0.25 | 2 | … | … | 93.4 | 1.7 |
Colistin | 0.25 | >8 | [83.5]e | 16.5 | … | … |
MDR (844)j | ||||||
Plazomicin | 0.25 | 1 | 94.8 | 2.5 | 94.8 | 2.5 |
Amikacin | 4 | 16 | 71.0 | 13.3 | 94.0d | 2.0 |
Gentamicin | >16 | >16 | 33.8 | 63.6 | 36.4d | 59.0 |
Tobramycin | 16 | >16 | 15.2 | 79.9 | 20.1d | 59.1 |
Cefepime | >16 | >16 | 16.7 | 73.5 | 16.7 | 73.5 |
Ceftazidime | 32 | >32 | 11.4 | 79.4 | 11.4d | 79.4 |
Ceftriaxone | >8 | >8 | 4.3 | 93.0 | 4.3d | 93.0 |
Imipenem | ≤0.12 | 4 | 83.2 | 12.9 | 83.2d | 12.9 |
Meropenem | 0.03 | 4 | 85.5 | 12.0 | 85.5d | 12.0 |
Piperacillin-tazobactam | 16 | >128 | 35.8 | 44.0 | 35.8d | 44.0 |
Levofloxacin | 8 | >16 | 16.5 | 72.8 | 16.5d | 72.8 |
Tigecycline | 0.5 | 2 | … | … | 93.5 | 1.2 |
Colistin | 0.25 | 0.5 | [91.4]e | 8.6 | … | … |
XDR (84)k | ||||||
Plazomicin | 0.25 | 1 | 94.0 | 6.0 | 94.0 | 6.0 |
Amikacin | 8 | >32 | 41.7 | 44.0 | 65.5d | 11.9 |
Gentamicin | 8 | >16 | 29.8 | 59.5 | 40.5d | 48.8 |
Tobramycin | >16 | >16 | 0.0 | 100.0 | 0.0d | 86.9 |
Cefepime | >16 | >16 | 1.2 | 90.5 | 1.2 | 90.5 |
Ceftazidime | >32 | >32 | 0.0 | 98.8 | 0.0d | 98.8 |
Ceftriaxone | >8 | >8 | 0.0 | 100.0 | 0.0d | 100.0 |
Imipenem | 8 | >8 | 9.5 | 86.9 | 9.5d | 86.9 |
Meropenem | 16 | >32 | 2.4 | 88.1 | 2.4d | 88.1 |
Piperacillin-tazobactam | >128 | >128 | 0.0 | 100.0 | 0.0d | 100.0 |
Levofloxacin | >16 | >16 | 0.0 | 95.2 | 0.0d | 95.2 |
Tigecycline | 0.5 | 2 | … | … | 94.0 | 1.2 |
Colistin | 0.25 | 0.5 | [92.9]e | 7.1 | … | … |
Antimicrobial Agent . | mg/L . | Clsi (2023)a . | Us fdab . | |||
---|---|---|---|---|---|---|
. | Mic50 . | Mic90 . | %s . | %r . | %s . | %r . |
Enterobacterales (9809)c | ||||||
Plazomicin | 0.5 | 1 | 96.4 | 0.8 | 96.4 | 0.8 |
Amikacin | 2 | 4 | 94.6 | 1.4 | 99.4d | 0.2 |
Gentamicin | 0.5 | 2 | 90.6 | 8.5 | 91.5d | 7.7 |
Tobramycin | 0.5 | 4 | 88.0 | 9.4 | 90.6d | 6.1 |
Cefepime | ≤0.12 | 8 | 88.4 | 9.4 | 88.4 | 9.4 |
Ceftazidime | 0.25 | 16 | 86.0 | 12.3 | 86.0d | 12.3 |
Ceftriaxone | ≤0.06 | >8 | 82.3 | 16.9 | 82.3d | 16.9 |
Imipenem | ≤0.12 | 1 | 92.4 | 2.1 | 92.4d | 2.1 |
Meropenem | 0.03 | 0.06 | 98.7 | 1.0 | 98.7d | 1.0 |
Piperacillin-tazobactam | 2 | 16 | 88.0 | 7.9 | 88.0d | 7.9 |
Levofloxacin | 0.06 | 8 | 80.3 | 16.4 | 80.3d | 16.4 |
Tigecycline | 0.25 | 1 | … | … | 96.6 | 0.4 |
Colistin | 0.25 | >8 | [84.7]e | 15.3 | … | … |
CRE (117)f | ||||||
Plazomicin | 0.25 | 1 | 94.0 | 5.1 | 94.0 | 5.1 |
Amikacin | 4 | 32 | 59.0 | 31.6 | 75.2d | 8.5 |
Gentamicin | 4 | >16 | 47.0 | 43.6 | 56.4d | 35.9 |
Tobramycin | 16 | >16 | 27.4 | 70.1 | 29.9d | 61.5 |
Levofloxacin | 16 | >16 | 14.5 | 78.6 | 14.5d | 78.6 |
Tigecycline | 0.5 | 2 | … | … | 94.9 | 0.9 |
Colistin | 0.25 | 0.5 | [91.4]e | 8.6 | … | … |
ESBL Producers (1011)g | ||||||
Plazomicin | 0.5 | 1 | 98.9 | 0.9 | 98.9 | 0.9 |
Amikacin | 4 | 8 | 79.7 | 8.4 | 96.9d | 1.3 |
Gentamicin | 1 | >16 | 54.5 | 44.3 | 55.7d | 42.2 |
Tobramycin | 8 | >16 | 40.4 | 56.1 | 43.9d | 41.7 |
Cefepime | >16 | >16 | 6.4 | 82.9 | 6.4 | 82.9 |
Ceftazidime | 32 | >32 | 15.1 | 73.9 | 15.1d | 73.9 |
Ceftriaxone | >8 | >8 | 0.4 | 98.9 | 0.4d | 98.9 |
Imipenem | ≤0.12 | 0.5 | 94.7 | 4.9 | 94.7d | 4.9 |
Meropenem | 0.03 | 0.12 | 93.5 | 5.5 | 93.5d | 5.5 |
Piperacillin-tazobactam | 8 | 128 | 63.1 | 23.2 | 63.1d | 23.2 |
Levofloxacin | 8 | >16 | 23.2 | 67.2 | 23.2d | 67.2 |
Tigecycline | 0.25 | 1 | … | … | 97.2 | 0.7 |
Colistin | 0.25 | 0.25 | 98.2 | 1.8 | … | … |
AME Producers (801)h | ||||||
Plazomicin | 0.5 | 1 | 97.3 | 1.4 | 97.3 | 1.4 |
Amikacin | 4 | 16 | 68.4 | 13.1 | 94.3d | 2.0 |
Gentamicin | >16 | >16 | 14.0 | 84.8 | 15.2d | 83.3 |
Tobramycin | 16 | >16 | 2.0 | 88.0 | 12.0d | 68.0 |
Cefepime | >16 | >16 | 32.5 | 63.0 | 32.5 | 63.0 |
Ceftazidime | 16 | >32 | 34.0 | 59.8 | 34.0d | 59.8 |
Ceftriaxone | >8 | >8 | 28.0 | 71.5 | 28.0d | 71.5 |
Imipenem | ≤0.12 | 1 | 90.9 | 7.7 | 90.9d | 7.7 |
Meropenem | 0.03 | 0.25 | 91.4 | 7.7 | 91.4d | 7.7 |
Piperacillin-tazobactam | 8 | >128 | 58.9 | 27.4 | 58.9d | 27.4 |
Levofloxacin | 8 | >16 | 29.0 | 63.4 | 29.0d | 63.4 |
Tigecycline | 0.25 | 2 | … | … | 95.5 | 0.7 |
Colistin | 0.25 | 0.25 | [95.7]e | 4.3 | … | … |
Amikacin-Nonsusceptible (528)i | ||||||
Plazomicin | 1 | 4 | 86.4 | 6.4 | 86.4 | 6.4 |
Amikacin | 8 | 32 | 0.0 | 26.5 | 89.4d | 3.6 |
Gentamicin | 2 | >16 | 62.9 | 33.1 | 66.9d | 31.8 |
Tobramycin | 8 | >16 | 42.2 | 54.0 | 46.0d | 49.4 |
Cefepime | 0.5 | >16 | 56.8 | 38.6 | 56.8 | 38.6 |
Ceftazidime | 1 | >32 | 56.1 | 39.6 | 56.1d | 39.6 |
Ceftriaxone | 1 | >8 | 50.8 | 47.3 | 50.8d | 47.3 |
Imipenem | ≤0.12 | 4 | 80.5 | 11.2 | 80.5d | 11.2 |
Meropenem | 0.03 | 0.5 | 90.7 | 8.7 | 90.7d | 8.7 |
Piperacillin-tazobactam | 4 | >128 | 67.6 | 24.4 | 67.6d | 24.4 |
Levofloxacin | 4 | >16 | 42.6 | 54.4 | 42.6d | 54.4 |
Tigecycline | 0.25 | 2 | … | … | 93.4 | 1.7 |
Colistin | 0.25 | >8 | [83.5]e | 16.5 | … | … |
MDR (844)j | ||||||
Plazomicin | 0.25 | 1 | 94.8 | 2.5 | 94.8 | 2.5 |
Amikacin | 4 | 16 | 71.0 | 13.3 | 94.0d | 2.0 |
Gentamicin | >16 | >16 | 33.8 | 63.6 | 36.4d | 59.0 |
Tobramycin | 16 | >16 | 15.2 | 79.9 | 20.1d | 59.1 |
Cefepime | >16 | >16 | 16.7 | 73.5 | 16.7 | 73.5 |
Ceftazidime | 32 | >32 | 11.4 | 79.4 | 11.4d | 79.4 |
Ceftriaxone | >8 | >8 | 4.3 | 93.0 | 4.3d | 93.0 |
Imipenem | ≤0.12 | 4 | 83.2 | 12.9 | 83.2d | 12.9 |
Meropenem | 0.03 | 4 | 85.5 | 12.0 | 85.5d | 12.0 |
Piperacillin-tazobactam | 16 | >128 | 35.8 | 44.0 | 35.8d | 44.0 |
Levofloxacin | 8 | >16 | 16.5 | 72.8 | 16.5d | 72.8 |
Tigecycline | 0.5 | 2 | … | … | 93.5 | 1.2 |
Colistin | 0.25 | 0.5 | [91.4]e | 8.6 | … | … |
XDR (84)k | ||||||
Plazomicin | 0.25 | 1 | 94.0 | 6.0 | 94.0 | 6.0 |
Amikacin | 8 | >32 | 41.7 | 44.0 | 65.5d | 11.9 |
Gentamicin | 8 | >16 | 29.8 | 59.5 | 40.5d | 48.8 |
Tobramycin | >16 | >16 | 0.0 | 100.0 | 0.0d | 86.9 |
Cefepime | >16 | >16 | 1.2 | 90.5 | 1.2 | 90.5 |
Ceftazidime | >32 | >32 | 0.0 | 98.8 | 0.0d | 98.8 |
Ceftriaxone | >8 | >8 | 0.0 | 100.0 | 0.0d | 100.0 |
Imipenem | 8 | >8 | 9.5 | 86.9 | 9.5d | 86.9 |
Meropenem | 16 | >32 | 2.4 | 88.1 | 2.4d | 88.1 |
Piperacillin-tazobactam | >128 | >128 | 0.0 | 100.0 | 0.0d | 100.0 |
Levofloxacin | >16 | >16 | 0.0 | 95.2 | 0.0d | 95.2 |
Tigecycline | 0.5 | 2 | … | … | 94.0 | 1.2 |
Colistin | 0.25 | 0.5 | [92.9]e | 7.1 | … | … |
Abbreviations: AME, aminoglycoside-modifying enzyme; CLSI, Clinical and Laboratory Standards Institute; CRE, carbapenem-resistant Enterobacteriaceae; ESBL, extended-spectrum β-lactamase; MIC, minimum inhibitory concentration; MDR, multidrug-resistant; %R, percentage resistant; %S, percentage susceptible; US FDA, United States Food and Drug Administration; XDR, extensively drug resistant.
Criteria as published by CLSI [15].
Criteria as published by US FDA [5].
Organisms include Citrobacter freundii (4), C freundii species complex (367), Citrobacter koseri (283), Enterobacter cloacae (89), E cloacae species complex (208), Escherichia coli (3271), Klebsiella aerogenes (305), Klebsiella oxytoca (715), Klebsiella pneumoniae (3185), Klebsiella variicola (1), Morganella morganii (289), Proteus mirabilis (334), Proteus penneri (2), Proteus vulgaris (76), P vulgaris group (99), Providencia alcalifaciens (1), Providencia rettgeri (134), Providencia stuartii (139), Serratia marcescens (302), unspeciated Klebsiella (1), and unspeciated Providencia (4).
The CLSI M100 (2022) standard is recognized by the US FDA [6].
The value in brackets indicates the percentage intermediate; CLSI does not publish a susceptible breakpoint for colistin [15].
Organisms include C freundii species complex (7), C koseri (1), E cloacae species complex (3), E coli (9), K aerogenes (4), K oxytoca (4), K pneumoniae (82), P rettgeri (2), and S marcescens (5).
Organisms include C freundii species complex (3), E cloacae species complex (1), E coli (509), K oxytoca (24), K pneumoniae (471), P vulgaris (1), S marcescens (1), and unspeciated Klebsiella (1).
Organisms include E cloacae species complex (13), E coli (409), K aerogenes (1), K oxytoca (31), K pneumoniae (328), P mirabilis (16), P vulgaris (2), and unspeciated Klebsiella (1).
Based on 2023 CLSI criteria.
Organisms include C freundii species complex (29), C koseri (5), E cloacae species complex (21), E coli (267), K aerogenes (15), K oxytoca (35), K pneumoniae (413), M morganii (19), P mirabilis (6), P vulgaris (1), P rettgeri (4), P stuartii (13), S marcescens (15), and unspeciated Klebsiella (1).
Organisms include C freundii species complex (4), E cloacae species complex (1), E coli (5), K aerogenes (1), K oxytoca (2), K pneumoniae (68), and S marcescens (3).
When tested against CRE isolates, susceptibility rates based on the 2023 CLSI criteria were 94.0% for plazomicin (MIC50/90, 0.25/1 mg/L), 59.0% for amikacin (MIC50/90, 4/32 mg/L), 47.0% for gentamicin (MIC50/90, 4/>16 mg/L), and only 27.4% for tobramycin (MIC50/90, 16/>16 mg/L). It is notable that the susceptibility of the CREs to amikacin was 75.2% based on 2022 FDA and 2022 CLSI criteria and dropped to 59.0% when 2023 CLSI criteria was applied (Table 2 and Figure 1). The most active compounds against CRE were plazomicin (94.0% susceptible per CLSI and FDA) and tigecycline (94.9% susceptible per FDA) (Table 2).
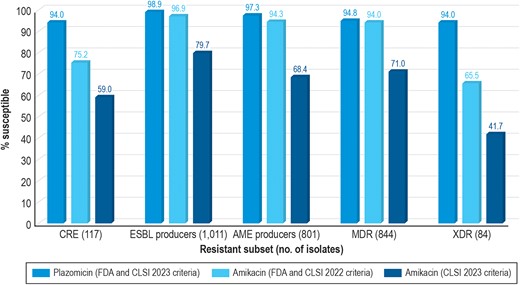
Spectrum of activity of plazomicin compared with amikacin using 2022 Clinical and Laboratory Standards Institute (CLSI)/US Food and Drug Administration (FDA) and 2023 CLSI breakpoint criteria. Provided separately as a TIFF. AME, aminoglycoside modifying enzyme; CRE, carbapenem-resistant Enterobacterales; ESBL, extended-spectrum β-lactamase; MDR, multidrug-resistant; XDR, extensively drug resistant.
An ESBL-encoding gene was identified in 1011 (10.3%) isolates; 98.9% were susceptible to plazomicin (MIC50/90, 0.5/1 mg/L) (Table 2 and Figure 1). The susceptibility of ESBL producers to amikacin were 96.9% based on 2022 FDA and 2022 CLSI criteria, but susceptibility dropped to 79.7% when 2023 CLSI criteria was applied (Table 2 and Figure 1). Gentamicin and tobramycin showed limited activity against ESBL producers, and meropenem was active against 93.5% of these isolates (Table 2).
Among 8286 isolates of genus Enterobacter, Escherichia, Klebsiella, or Proteus, 815 (9.8%) were resistant to at least 1 of the old aminoglycosides (amikacin, gentamicin, and tobramycin) based on 2022 CLSI criteria and were screened for AMEs by whole genome sequencing. Among those 815 aminoglycoside-resistant isolates, 801 (98.4%) had at least 1 AME-encoding gene, including genes encoding aminoglycoside acetyltransferases (AAC)-modifying enzymes (n = 777; 97.0% of AME producers), aminoglycoside phosphotransferase (APH)-modifying enzymes (n = 447; 55.9%), and aminoglycoside nucleotidyltransferase (ANT)-modifying enzymes (n = 442; 55.2%). aac(6′)-Ib-cr (n = 417) and aac(3)-IIa (n = 376) were the most prevalent AMEs alone or in combination. These 2 AMEs were found mostly among E coli and K pneumoniae. Among all isolates in this group, 300 harbored both aac(6′)-Ib-cr and aac(3)-IIa. A 16S RNA methyltransferase gene was identified in 11 isolates (0.1% of the collection).
Plazomicin was the most active agent tested against AME-producing strains (MIC50/90, 0.5/1 mg/L; 97.3% susceptible), followed by tigecycline (MIC50/90, 0.25/2 mg/L; 95.5% susceptible per FDA), meropenem (MIC50/90, 0.03/0.25 mg/L; 91.4% susceptible), and imipenem (MIC50/90, ≤0.12/1 mg/L; 90.9% susceptible). Only 68.4% of the AME producers were susceptible to amikacin according to 2023 CLSI criteria (Table 2 and Figure 1).
Remarkably, among isolates categorized as amikacin-nonsusceptible based on the 2023 CLSI breakpoints (n = 528; MIC, >4 mg/L), 86.4% remained susceptible to plazomicin, 62.9% remained susceptible to gentamicin (MIC, ≤2 mg/L), and 42.2% remained susceptible to tobramycin (MIC, ≤2 mg/L) (Tables 2 and Supplementary Table 1).
When tested against MDR isolates (n = 844; 8.6%), susceptibility rates based on the 2023 CLSI criteria were 94.8% for plazomicin (MIC50/90, 0.25/1 mg/L), 71.0% for amikacin (MIC50/90, 4/16 mg/L), 33.8% for gentamicin (MIC50/90, >16/>16 mg/L), and 15.2% for tobramycin (MIC50/90, 16/>16 mg/L). Susceptibility to amikacin was 94.0% based on 2022 CLSI and FDA criteria but declined to 71.0% when 2023 CLSI criteria was applied (Table 2 and Figure 1). The most active compounds against MDR isolates were plazomicin (94.8% susceptible per 2023 CLSI and FDA), tigecycline (93.5% susceptible per FDA), and meropenem (85.5% susceptible per 2022 CLSI and FDA) (Table 2). Moreover, plazomicin retained potent activity against XDR isolates (MIC50/90, 0.25/1 mg/L; 94.0% susceptible), whereas amikacin was active against only 41.7% of isolates based on the 2023 CLSI criteria (65.5% susceptible per 2022 CLSI and FDA criteria) (Table 2 and Figure 1).
DISCUSSION
The aminoglycosides breakpoints published by CLSI until 2022 (Table 1) were established in the 1970s when PK/PD data were not available [20]. Susceptible breakpoints were based mainly on blood levels related to toxicity, whereas resistant breakpoints were based on maximum blood levels achieved by these compounds [21]. In 2019, the US Committee on Antimicrobial Susceptibility Testing (USCAST) published a document [22] describing the rationale for lowering the breakpoints of older aminoglycosides based on contemporary PK/PD parameters that were not available when these compounds were first approved and introduced to market. More recently, CLSI reassessed the aminoglycoside breakpoints and lowered the Enterobacterales breakpoints for amikacin, gentamicin, and tobramycin based on currently available PK/PD and microbiology data and on an extensive review of published clinical data [15]. It is notable that the aminoglycosides breakpoints published by the European Committee on Antimicrobial Susceptibility Testing (EUCAST) for Enterobacterales since 2010 (≤8/>16 mg/L for amikacin and ≤2/>4 mg/L for gentamicin, netilmicin, and tobramycin for susceptible/resistant) [23] are 1 double dilution lower than those published by CLSI until 2022 [5] and still recognized by the US FDA [6].
The worldwide emergence of MDR Gram-negative bacteria led to a resurgence in the clinical use of aminoglycosides. However, these breakpoint changes will greatly impact the spectrum of activity of the old aminoglycosides against Enterobacterales. Our results indicate these changes mainly affect the spectrum of amikacin, especially against resistant subsets of organisms. The amikacin-susceptible breakpoint was lowered by 2 doubling dilutions, from ≤16 mg/L to ≤4 mg/L, and the overall coverage of amikacin against Enterobacterales decreased from 99.4% to 96.4%. Most importantly, the amikacin spectrum decreased from 75.2% to 59.0% against CRE, from 96.9% to 79.7% against ESBL producers, and from 94.3% to 68.4% against MDR isolates. Remarkably, 86.4% of isolates that were nonsusceptible to amikacin based on the revised breakpoint of ≤4 mg/L were susceptible to plazomicin. The antimicrobial spectrums of gentamicin and tobramycin were less affected by these changes compared with amikacin because their breakpoints decreased by only 1 doubling dilution and because these 2 aminoglycosides have more limited activity against resistant subsets of Enterobacterales compared with amikacin. It is also important to note that among isolates nonsusceptible to amikacin, 62.9% and 42.2% remained susceptible to gentamicin and tobramycin, respectively, when the 2023 CLSI breakpoints are applied.
The results of this investigation clearly showed the effect of the breakpoint changes on the spectrum of amikacin, gentamicin, and tobramycin against Enterobacterales causing infection in US hospitals. Due to the incorrect and overly high breakpoints, the activities of these compounds (their susceptibility rates) were believed to be much higher than they really were, leading to the inappropriate use of these compounds for decades. Our results also emphasize the greater activity of the newer aminoglycoside plazomicin compared with amikacin, gentamicin, and tobramycin against difficult-to-treat isolates when interpretative criteria established with similar PK/PD parameters are applied to all compounds. Based on 2023 CLSI and 2022 US FDA breakpoints, plazomicin was active against 94.0% of CREs, 98.9% of ESBL producers, 97.3% of AME producers, 94.8% of MDR isolates, and 94.0% of XDR isolates. Moreover, plazomicin retained activity against 86.4% of amikacin-nonsusceptible isolates.
Resistance to plazomicin is caused mainly by 16S rRNA ribosomal methyltransferases that protect the ribosome and prevent the binding of virtually all aminoglycosides producing high resistance levels. The results of this study and prior investigations demonstrate the rare occurrence of 16S rRNA ribosomal methyltransferases in the United States [9, 24]. Over this 5-year surveillance encompassing approximately 10 000 isolates, we identified only 11 (0.1%) isolates carrying these genes. In addition, AAC(2′)-Ia and APH(2′‘)-IVa have been shown to use plazomicin as a substrate, but these enzymes are uncommon and limited to Providencia stuartii and Enterococcus spp [25].
CONCLUSIONS
In summary, the differences between susceptibility rates for plazomicin and other aminoglycosides were greater when applying breakpoints generated using the same rigorous contemporary methods applied to determine plazomicin breakpoints. As a result, plazomicin is a more attractive alternative for the treatment of serious infections caused by Enterobacterales, especially CRE and MDR isolates.
Supplementary Data
Supplementary materials are available at Open Forum Infectious Diseases online. Consisting of data provided by the authors to benefit the reader, the posted materials are not copyedited and are the sole responsibility of the authors, so questions or comments should be addressed to the corresponding author.
Acknowledgments
We thank all participants of the SENTRY Antimicrobial Surveillance Program for their work in providing isolates. Editorial support was provided by Amy Chen at JMI Laboratories and was funded by AbbVie.
Financial support. This study was supported by Cipla Ltd . HSS, REM, JHK, VK, and MC are employees of JMI Laboratories , which was a paid consultant to Cipla in connection with the development of this manuscript.
References
Author notes
Potential conflicts of interest. None of the authors has a conflict of interest. JMI Laboratories was contracted to perform services in 2022 for AimMax Therapeutics, Amicrobe, Inc., Appili Therapeutics, Armata Pharmaceuticals, Astellas Pharma, Inc., Basilea Pharmaceutica AG, Becton, Dickinson and Company, bioMérieux, Biosergen AB, Bugworks, Cerba Research NV, Cidara Therapeutics, Cipla USA Inc., ContraFect Corporation, CorMedix Inc., Crestone, Inc., Curza Global, LLC, Diamond V, Discuva Ltd., Entasis Therapeutics, Enveda Biosciences, Evopoint Biosciences, Fedora Pharmaceuticals, Fox Chase Chemical Diversity Center, Genentech, Gilead Sciences, Inc., GSK plc, Institute for Clinical Pharmacodynamics, Iterum Therapeutics plc, Janssen Biopharma, Johnson & Johnson, Kaleido Biosciences, LifeMine Therapeutics, Medpace, Inc, Lysovant Sciences, Inc, Meiji Seika Pharma, Melinta Therapeutics, Menarini Group, Merck & Co., MicuRx Pharmaceutical Inc., Mundipharma International Ltd., Mutabilis, Nabriva Therapeutics, National Cancer Institute, National Institues of Health, Ohio State University, Omnix Medical Ltd., Paratek Pharmaceuticals, Pfizer, PolyPid Ltd., PPD, Prokaryotics, Inc., Pulmocide Ltd, Qpex Biopharma, Revagenix, Roche Holding AG, Roivant Sciences, Scynexis, Inc., SeLux Diagnostics, Shionogi & Co., Ltd., Sinovent Pharmaceuticals, Inc., Spero Therapeutics, Sumitovant Biopharma, Inc., TenNor Therapeutics, ThermoFisher Scientific, U.S. Food and Drug Administration, VenatoRx Pharmaceuticals, Washington University, Watershed Medical, LLC, Wockhardt, and Zoetis, Inc.
Comments