-
PDF
- Split View
-
Views
-
Annotate
-
Cite
Cite
Yang Shen, Xin Zhang, Ming Li, Yuanhua Lin, Ce-Wen Nan, Polymer nanocomposite dielectrics for electrical energy storage, National Science Review, Volume 4, Issue 1, January 2017, Pages 23–25, https://doi.org/10.1093/nsr/nww066
- Share Icon Share
Dielectric materials store and release electrical energy electrostatically through dielectric polarization and depolarization by the application and removal of an electric field (as shown in Fig. 1). As the only energy-storage device that is capable of delivering power density on the order of mega or even giga watts, electrostatic capacitors based on dielectric materials are recently considered as the major enabler for a number of modern electrical applications, such as high-frequency inverters, IGBT snubbers, power factor correction and pulsed power generation [1]. The application of electrostatic capacitors as viable energy-storage devices has currently been limited by their low energy-storage density. The energy-storage density (Ue) of dielectric materials is determined by electrical displacement (D) and electric field (E) as Ue = ∫EdD and is usually obtained from the D–E loop (as shown by the area shaded in green). For linear dielectrics, Ue scales linearly with dielectric permittivity (εr) and quadrically with E as Ue = 1/2ε0εrE2, where ε0 is the vacuum dielectric permittivity. The breakdown strength (Eb) that signifies the highest electric field applicable to the dielectrics is thus the most critical parameter that defines the maximal energy density achievable. Polymers has been the dielectrics of current use for power capacitors due to their high Eb and graceful failure with an open circuit during breakdown. However, due to their low intrinsic εr, and hence small electrical polarization, polymer dielectrics only bear rather lower energy density.
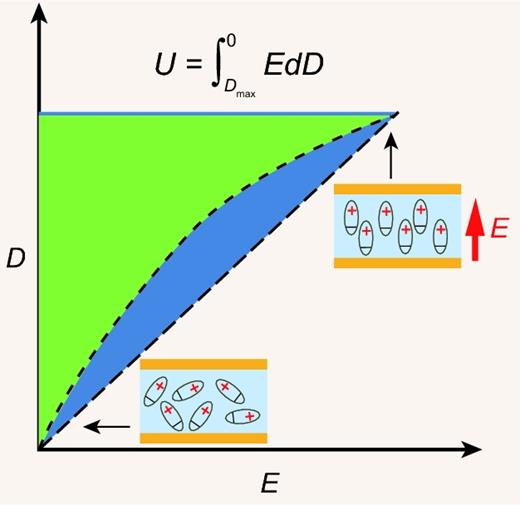
Electrical displacement (D)–electric field (E) loop of dielectrics. Upon the application of an external electric field, the electric dipoles inside the dielectric are oriented along the direction of the external electric field. The electric energy stored during the poling process is determined by the formula superimposed and could be calculated from the shaded area in green.
To raise Ue, the nanocomposites approach has been intensively explored, where polymeric and inorganic phases are integrated facially [2]. The rationale behind the nanocomposite approach lies in their potential of combining the high breakdown strength and low dielectric loss of the polymer with ceramic fillers of high dielectric constant, leading to high energy density. Straightforward at first sight, this approach can be complicated by a number of factors, such as the filler/matrix interfaces and the anisotropy of fillers, which may to a large extent determine the dielectric behavior of these nanocomposites. Moreover, incorporation of dielectric ‘soft’ nanofillers of high εr in dielectric ‘hard’ polymer matrix of low εr induces inhomogeneous distribution of local electric field and gives rise to a local hot spot of concentrated local electric field in the polymer matrix, which results in early breakdown of polymer nanocomposites at an electric field well below the intrinsic Eb of the polymer matrix [3]. Innovative strategies have to be developed in order to balance the seemingly contradictory demands of enhancing dielectric constants while maintaining high dielectric strength and to fully explore the promises and potentials of nanocomposites.
The most straightforward approach to modulating the filler/matrix interfaces has been the employment of nanofillers with a ‘core-shell’ structure [4]. By coating the surface of nanofiller ‘cores’ with organic or inorganic ‘shells’ of moderate dielectric permittivity, an interface layer is formed between the nanofiller and the polymer matrix, which mitigates the dielectric mismatch between the nanofiller and the polymer matrix, improves the compatibility between filler and matrix, and gives rise to improved Eb of the polymer nanocomposites [5]. To reduce the number of nanofillers needed for enhanced εr, nanofillers with large aspect ratios, such as 1D [6] nanofibers or nanowires and 2D [7] nanosheets, have been intensively explored. The orientation of these large-aspect-ratio nanofillers along the directions perpendicular to the external electric field provides ordered scattering centers as well as tortuous paths for free electrons during breakdown, giving rise to enhanced Eb in these polymer nanocomposites.
In addition to purely improving Eb, novel approaches have also been proposed for the concomitant enhancement of εr, hence electrical polarization, and Eb of polymer nanocomposites. The most straightforward approach has been to employ dual nanofillers to improve both electrical polarization and breakdown strength. BaTiO3 spherical nanoparticles have been the most commonly used nanofillers to induce enhanced electrical polarization while montmorillonite (MMT) nanoplatelets [8] or hexagonal boron nitride nanosheets (BNNSs) [9] were used to improve dielectric breakdown strength. Recently, we proposed and demonstrated that very high energy density could be achieved by simultaneous modulation of interfacial polarization and dielectric anisotropy in the polymer nanocomposites. In the first demo system [10], we prepared a class of topological-structure modulated nanocomposite, in which BaTiO3 nanoparticles were introduced in the outer composite layers to induce high electrical polarization, while BaTiO3 nanofibers were used in the central composite layer for improving the breakdown strength. The outer layers filled with BaTiO3 nanoparticles can be polarized up to a higher electric field, hence enhancing the electric displacement. Enhanced electric displacement and breakdown strength were achieved concomitantly. By carefully tuning the relative thickness of the constituent layers, a maximum discharged energy density of 9.7 J/cm3 was achieved. In the second demo system [11], we directed our attention to the inside of the ceramic nanofibers with the objective of introducing hierarchical interfaces into the corresponding polymer nanocomposites. TiO2 nanofibers embedded with BaTiO3 nanoparticles (TiO2@BaTiO3 nanofibers) were prepared via electrospinning and then fused with poly (vinylidene fluoride-hexafluoropropylene) (P(VDF-HFP)) into polymer nanocomposite films. Inside the TiO2@BaTiO3 nanofibers, atomic-scale engineering of the hierarchical interfaces between TiO2@BaTiO3 generates a much-increased dielectric permittivity while the large aspect ratio and partial orientation of TiO2@BaTiO3 nanofibers render the nanocomposites with improved breakdown strength, giving rise to an ultrahigh energy density of ∼31 J/cm3 with a dielectric breakdown strength at ∼800 kV/mm.
The ever-increasing demands for miniaturization and functionality of electrical and electronic devices also impose higher challenges for the thermal stability of dielectric materials, especially in the scenarios where capacitors are subjected to both high temperature and high voltage. When subjected to high voltage, even the most robust engineering polymers, such as polyether ether ketone (PEEK) or polycarbonate (PC) etc. which exhibit high glass-transition temperatures (Tg) and are theoretically capable of withstanding a high temperature up to 300°C, fail at rather low temperatures (<150°C), exhibiting significantly increased dielectric loss and dramatically decreased discharge efficiency. With low intrinsic thermal conductivity (usually < 5 Wm/K), pure polymers are susceptible to thermal runaway. When the joule heat generated by the much-increased high field electrical conduction is accumulated from inside the polymer, the local temperature is substantially raised well above Tg, resulting in drastically decreased Young's modulus and Eb. Wang et al. [12] recently demonstrated that incorporation of hexagonal boron nitride (h-BN) nanosheets in a polymer matrix dramatically improves the thermal stability of nanocomposites at high electric field. The BN-filled nanocomposites exhibit high Eb of 403 kV/mm at a record high temperature of 250°C. When composed with the polymer matrix, h-BN nanosheets of high intrinsic thermal conductivity (∼300 Wm/K) form networks throughout the polymer matrix, which accelerate heat conduction from inside and prevent the adverse temperature rise. Moreover, the h-BN networks also render nanocomposites with robust mechanical properties, making them less susceptible to electromechanical failure at high electric field.
Finally, we note the technical issues associated with the massive fabrication of polymer nanocomposite films. Polymer nanocomposites filled with inorganic nanoparticles are not compatible with the extrusion-stretching techniques currently used for the roll-to-roll fabrication of pure polymer thin-films, such as BOPP or PET. The nanoparticles could act as a center for stress concentration and lead to cracks during the stretching process. Post hot-pressing or thermal treatments are thus required after the film-casting (either from solution or from melt) process to reduce the thickness of the as-cast thin-films and more importantly to eliminate any pores or other structural imperfections induced. Novel apparatuses have thus to be designed and developed for this more complicated fabrication protocol.
FUNDING
This work was supported by the National Basic Research Program of China (2015CB654603), the National Natural Science Foundation of China (51572141 and 51532003) and the Research Fund of Science and Technology in Shenzhen (JSGG20150331155519130).
REFERENCES