-
PDF
- Split View
-
Views
-
Cite
Cite
Norbert Galldiks, Philipp Lohmann, Michel Friedrich, Jan-Michael Werner, Isabelle Stetter, Michael M Wollring, Garry Ceccon, Carina Stegmayr, Sandra Krause, Gereon R Fink, Ian Law, Karl-Josef Langen, Joerg-Christian Tonn, PET imaging of gliomas: Status quo and quo vadis?, Neuro-Oncology, Volume 26, Issue Supplement_9, December 2024, Pages S185–S198, https://doi.org/10.1093/neuonc/noae078
- Share Icon Share
Abstract
PET imaging, particularly using amino acid tracers, has become a valuable adjunct to anatomical MRI in the clinical management of patients with glioma. Collaborative international efforts have led to the development of clinical and technical guidelines for PET imaging in gliomas. The increasing readiness of statutory health insurance agencies, especially in European countries, to reimburse amino acid PET underscores its growing importance in clinical practice. Integrating artificial intelligence and radiomics in PET imaging of patients with glioma may significantly improve tumor detection, segmentation, and response assessment. Efforts are ongoing to facilitate the clinical translation of these techniques. Considerable progress in computer technology developments (eg quantum computers) may be helpful to accelerate these efforts. Next-generation PET scanners, such as long-axial field-of-view PET/CT scanners, have improved image quality and body coverage and therefore expanded the spectrum of indications for PET imaging in Neuro-Oncology (eg PET imaging of the whole spine). Encouraging results of clinical trials in patients with glioma have prompted the development of PET tracers directing therapeutically relevant targets (eg the mutant isocitrate dehydrogenase) for novel anticancer agents in gliomas to improve response assessment. In addition, the success of theranostics for the treatment of extracranial neoplasms such as neuroendocrine tumors and prostate cancer has currently prompted efforts to translate this approach to patients with glioma. These advancements highlight the evolving role of PET imaging in Neuro-Oncology, offering insights into tumor biology and treatment response, thereby informing personalized patient care. Nevertheless, these innovations warrant further validation in the near future.
In recent years, the role of PET imaging in the field of Neuro-Oncology has evolved considerably. For various indications in patients with glioma, particularly amino acid PET has gained broader international acceptance as an adjunct to anatomical MRI. This success is related to intensive research work, resulting in numerous publications, and prompted subsequently the preparation of clinical and technical guidelines for PET imaging in patients with glioma.1,2 Notably, these guidelines are joint efforts of experts from various major societies and organizations in the field of Neuro-Oncology (ie EANO, RANO, and SNO) and Nuclear Medicine (ie EANM and SNMMI). Moreover, most probably due to the visibility of these highly cited guidelines and the convincing results of the published research, a reimbursement of PET imaging using radiolabeled amino acids by statutory health agencies to differentiate treatment-related changes from tumor relapse in patients with glioma has now been granted, especially in various countries in Europe (eg Germany).
Beyond the status quo of PET imaging in patients with glioma, there are currently several emerging topics both in the field of Nuclear Medicine and Neuro-Oncology, which may be of considerable interest to this group of patients in the near future. First of all, integrating artificial intelligence (AI) together with radiomics opened new perspectives for managing patients with brain tumors including gliomas. The advent of quantum computers allows us to process large amounts of data in parallel, which is far superior to the sequential processing speed of conventional computers. Furthermore, quantum computers may considerably improve the ability of AI technologies and machine learning to solve complex problems, which could also become relevant in Neuro-Oncology.
Second, from a technical point of view, the above advances are paralleled by considerable progress in PET scanner development. Recently introduced long-axial field-of-view PET/CT scanners represent one of the most significant advancements in Nuclear Medicine since the advent of PET/CT and hybrid PET/MR imaging.3,4 The main advantages of these systems are improved image quality, including noise reduction, resulting in significantly improved lesion detection, a tremendously shorter acquisition time, and increased body coverage due to the extended field-of-view. This progress allowed extending the spectrum of indications for PET imaging in Neuro-Oncology to spinal cord tumors and CNS pathologies with systemic involvement (eg primary CNS lymphoma with suspected systemic spread). In pediatric Neuro-Oncology, a high-quality whole-body PET scan using long-axial field-of-view PET/CT can be obtained within only a few minutes, making only low-dose or even no anesthesia necessary.
Furthermore, PET imaging of therapeutically relevant targets for novel anticancer agents in gliomas has gained considerable interest, especially for response assessment. The mutant isocitrate dehydrogenase (IDH)-1 and -2 enzymes are critical treatment targets. In particular, the phase-3 INDIGO trial tested vorasidenib, a dual inhibitor of mutant IDH-1 and IDH-2 enzymes, in patients with residual or recurrent CNS WHO grade 2 gliomas against placebo. The main encouraging finding was that the progression-free survival and the time to the next intervention were significantly prolonged.5 Notwithstanding, a limited specificity for neoplastic tissue and unchanged MR imaging findings following IDH inhibition may impede response assessment based on anatomical MRI alone. Consequently, efforts were initiated to develop selective PET tracers with high specificity for IDH mutations to improve response assessment concerning these agents.
Finally, the success of theranostics in treating extracranial neoplasms such as neuroendocrine tumors and prostate cancer has recently prompted efforts to translate this approach to patients with brain tumors.6 By exchanging the radionuclide, the same tracer can be used either for diagnostic purposes or therapy, which reflects the concept of theranostics. An exchange of the positron emitter Ga-68 or F-18 used for diagnostic PET with a β−-emitter such as Lu-177 or Y-90 allows radionuclide therapy. A few clinical applications of theranostics in glioma patients have surpassed the preclinical level and are currently evaluated in clinical trials.
This review aims to briefly summarize the status quo of PET imaging in patients with glioma and to discuss several more recent developments both in the field of Nuclear Medicine and Neuro-Oncology with potential implications for the future of this group of patients.
Search Strategy
A PubMed search of the published literature with the combination of the search terms “glioma,” “glioblastoma,” “astrocytoma,” “scanner,” “IDH,” “PET,” “positron,” “theranostics,” “RANO,” “amino acid,” “MET,” “FET,” “FDOPA,” “fluciclovine,” “artificial intelligence,” “AI,” “radiomics”, and combinations thereof before and inclusive of February 2024 was performed. Additionally, articles identified through searches of the authors’ own files were included in the search.
Status Quo of Amino Acid PET for Gliomas
Tracers
For clinical management of patients with gliomas, PET-based imaging using amino acid tracers complementing anatomical MRI has become a valuable diagnostic tool. Compared to contrast enhancement on MRI, the uptake of radiolabeled amino acids is not limited to blood–brain barrier disruption, allowing the identification of nonenhancing glioma subregions. The amino acid tracer O-(2-[18F]-fluoroethyl)-l-tyrosine (18F-FET) is most commonly used for patients with gliomas in Europe, along with 11C-methyl-l-methionine (11C-MET) and 3,4-dihydroxy-6-[18F]-fluoro-l-phenylalanine (18F-DOPA). In contrast to 2-[18F]fluoro-2-deoxy-d-glucose (18F-FDG) PET, radiolabeled amino acids show a relatively low uptake in healthy brain tissue, improving tumor-to-brain contrast. The uptake of amino acids is mainly facilitated by the overexpression of amino acid transporters of the L-type (ie subtypes LAT1 and LAT2). The radioisotope F-18 has a longer half-life than C-11 (110 vs. 20 min), allowing amino acids labeled with F-18 to be transported to other facilities and eliminating the need for on-site cyclotrons.
Based on the abovementioned advantages, radiolabeled amino acids are increasingly used in the field of Neuro-Oncology.7 Nevertheless, the availability and broad clinical application of amino acid PET vary considerably, even in specialized brain tumor centers. More recent joint efforts by various major Nuclear Medicine and Neuro-Oncology societies have already resulted in several published expert consensus recommendations8 and guidelines,1,2 with further ongoing activities to support the use of radiolabeled amino acids in patient management. The overarching goal is to achieve broad access to amino acid PET through approval by regulatory authorities and reimbursement by statutory health insurance agencies on an international level. Currently, the reimbursement of amino acid PET in glial tumors by statutory health insurance agencies is not yet uniformly accomplished in Europe, varying from country to country.
Clinical Applications of Amino Acid PET
In patients with glioma, amino acid PET is most frequently used for differentiating glioma relapse from treatment-related changes in almost 50% of this patient group.9 Several studies in patients with predominantly IDH-wildtype glioblastoma reported a high accuracy of 80–90% in differentiating between tumor relapse and treatment-related changes with early (ie pseudoprogression) or late onset (eg radiation necrosis) for the tracers 18F-FET and 18F-DOPA.10–14 Slightly lower accuracies were demonstrated for the tracer 11C-MET.15
Due to the ability of amino acid PET to delineate nonenhancing glioma subregions, this method is also increasingly used to guide invasive diagnostic procedures and treatment planning. Critical applications are biopsy guidance in patients with nonenhancing gliomas, target volume definition for radiotherapy, and the identification of metabolically active glioma regions without contrast enhancement for resection planning. These clinically relevant applications are related to the fact that parameters obtained from both static and dynamic amino acid PET acquisition were histomolecularly and spatially correlated with the most malignant tumor parts.16–21
Following glioma therapy, both an increase of the contrast-enhancing volume and the size of signal hyperintensity on T2 or fluid-attenuated inversion recovery MRI sequences may be unspecific and related to perifocal edema, radiation injury, demyelination, inflammation, or ischemia,22–24 which hampers a reliable response assessment. In recent years, amino acid PET has been used to evaluate the response to various glioma treatment options. In particular, it has been demonstrated that metabolic responders on amino acid PET to local (eg radiotherapy with concomitant temozolomide chemotherapy) and systemic treatment options (e.g. alkylating and antiangiogenic agents, targeted therapies) predicted a significantly longer survival than metabolic nonresponders and MRI responders.25–29 For use in prospective clinical trials, the PET RANO 1.0 criteria for response assessment based on amino acid PET have recently been introduced.1 Efforts to validate these newly defined criteria are currently ongoing.
Another important indication for amino acid PET in clinical routine is the differential diagnosis of newly diagnosed brain lesions suspected of glial neoplasms. In contrast to non-neoplastic etiologies, gliomas generally exhibit a considerably higher uptake of radiolabeled amino acids, thereby informing differential diagnosis.30,31
Amino Acid PET Tracer Developments
A wide range of natural and non-natural amino acids have been labeled with positron emitters and investigated in the last 2 decades, which stimulated further research in this field.32 Of considerable interest not only for PET imaging of gliomas but also brain metastases, is the synthetic amino acid analog anti-1-amino-3-[18F]fluorocyclobutane-1-carboxylic acid (18F-FACBC or 18F-Fluciclovine). 18F-Fluciclovine transport is mediated to a lesser extent by LAT1 but predominantly by the neutral alanine serine cysteine transporter, especially the subtype 2 (ASCT2).33 For glioma imaging in the United States, 18F-Fluciclovine received orphan drug status. A recent prospective study validated neuropathologically the value of 18F-Fluciclovine PET for differentiating pseudoprogression from actual tumor progression in patients with glioblastoma after temozolomide chemoradiation with 90% sensitivity and 83% specificity.34 The development of radiolabeled glutamine derivates for PET imaging, e.g. 4-(2S,4R)-[18F]fluoroglutamine (18F-FGln), capitalizes on the fact that glutamine is one of the major nutrients for tumor cells. Like 18F-Fluciclovine, experimental studies have shown that 18F-FGln is also mainly transported through the ASC transporter system, particularly subtype ASCT2.35 First studies demonstrated a high tumor-to-brain contrast with 18F-FGln similar to that observed with 18F-Fluciclovine but it remains unclear whether this tracer delineates nonenhancing glioma subregions.36,37
Tryptophan derivates are another group of amino acid PET tracers of considerable interest for glioma imaging due to the expression of indoleamine 2,3-dioxygenase, the initial and rate-limiting enzyme of the kynurenine pathway, which is upregulated in various cancers including gliomas.38 At present, the clinical utility of radiolabeled tryptophan derivates such as the l-tryptophan analogue [11C]-methyl-l-tryptophan is hampered by its C-11 labeling with a short half-life. Several F-18-labeled tryptophan derivates are currently under investigation and may facilitate the clinical use of this group of tracers.39
Artificial Intelligence and Radiomics for PET Applications
Current Status
In recent years, integrating AI and radiomics has opened new perspectives for managing patients with brain tumors. The benefits of AI in medicine require exceptional levels of accuracy and reliability, which depend to a great extent on the availability of large, multicenter data sets for the development and evaluation of AI models. This need poses an intrinsic challenge for AI in neuro-oncology, where the number of patients and available datasets is usually low. In contrast, the growing amount and complexity of the available data derived from brain tumor diagnostics and follow-up examinations such as multimodal neuroimaging, genomics, methylomics, or transcriptomics cause computational tasks to be increasingly challenging, requiring powerful computing resources.
Given that contrast-enhanced anatomical MRI is the method of choice for brain tumor diagnostics, the number of studies investigating the benefits of AI technologies based on PET is relatively small. Nevertheless, due to the undisputed clinical importance and the growing availability of amino acid PET for patients with gliomas or secondary brain tumors in the past years,8,40 encouraging results have been obtained using PET-based radiomics or AI methods. For example, Lohmann et al. developed a deep learning-based segmentation algorithm for the automated detection and segmentation of gliomas and brain metastases based on 18F-FET PET.41 The automated detection of these tumors offered a quality level comparable to an experienced physician but in a fraction of the time. The tool can potentially yield more reproducible and standardized results for a PET-based response assessment, which is particularly useful for less experienced clinicians.
Furthermore, several studies investigated the use of PET-based radiomics for the noninvasive prediction of clinically relevant genetic alterations (radiogenomics) such as the O6-methylguanine DNA methyltransferase promoter methylation,42 an IDH gene mutation,43,44 and the telomerase reverse transcriptase promoter mutational status.45 Overall, the prediction of these markers could be obtained with a high diagnostic accuracy.
PET-based AI methods have also been developed for early and reliable differentiation of treatment-related changes such as pseudoprogression or radiation necrosis from tumor relapse. Besides promising results reporting diagnostic accuracies ranging from an area under the receiver operating characteristic curve from 0.85 to 0.98 in validation data sets of glioma patients,46–49 a few studies additionally demonstrated the model’s generalizability in independent test datasets.46,48,49
To reduce the variability and improve the reproducibility of AI-based studies50 to facilitate clinical translation, researchers should adhere more closely to existing guidelines.51–53 In addition, promising AI methods need to be integrated into future clinical trials to fully assess and demonstrate their clinical value.54 Despite the challenges and dynamic developments in this field, AI and radiomics have the potential to improve diagnostics in the field of Neuro-Oncology substantially.
A Glimpse into the Future: Quantum Computing
The AI technologies and machine learning models described above benefit from high computing power, enabling some of the developments of recent years. The advent of quantum computers may open completely new possibilities. The fundamental difference between conventional and quantum computers lies in their respective computational principles and functions.
Conventional computers use binary units, ie bits, the most basic data unit that exists in only one of 2 values: 0 or 1. These bits are analogous to simple on or off switches. The operations of conventional computers—whether running complex medical tasks, managing patient databases, or performing statistical analyses—are based on sequences of these binary bits that can be manipulated in a controlled and systematic way, thereby encoding and decoding instructions. The information is processed sequentially, 1 bit at a time, which is efficient for a range of tasks but limits processing speed.
On the other hand, quantum computers work with qubits and use principles of quantum mechanics, which is often deemed counter-intuitive as systems with quantum behavior do not follow classical physical rules, e.g. they can exist in different states at the same time and even change depending upon whether they are observed or not. Unlike bits, a qubit can represent 0, 1, or any quantum superposition (“mixture”) of these states until it is measured. This probabilistic nature of qubits allows quantum computers to perform complex calculations by handling many possibilities at once.
In addition to the quantum superposition, quantum computers exploit another quantum phenomenon called entanglement, where pairs or groups of qubits become interconnected, and the state of 1 qubit instantaneously influences the state of the other. This connection allows quantum computers to process large amounts of data in parallel, which is far superior to the sequential processing speed of conventional computers.
The enhanced quantum computer capabilities compared to conventional computers are related to these quantum properties, which does not mean that quantum computers are universally superior. Although still in their infancy, quantum computers are expected to be especially advantageous for certain types of tasks, such as solving complex algorithms or optimization problems. Furthermore, it is expected that quantum computers will considerably improve the AI technologies and machine learning’s ability to solve complex problems, which could also become relevant in Neuro-Oncology.
Hybrid algorithms, which combine quantum and conventional computing, are a promising approach to leveraging quantum computing in medical applications.55 In this case, the quantum computer interacts with a conventional computer so that the quantum computer calculates only those parts of the algorithm that benefit from the efficiency of quantum computers. A promising example of such a hybrid algorithm is the quantum convolution neural network (QCNN). Conventional convolutional neural networks (CNN) are often used in Neuro-Oncology for tumor detection and segmentation. CNN usually consider only 1 or several subsections of the original images in the so-called convolution layer. Replacing the conventional convolution layer with a quantum convolution layer requires just a few qubits and is somewhat independent of errors made by the quantum computer hardware. In any case, the quantum convolution layer is likely to perform much more efficiently than the conventional convolution layer. QCNN could require less training data and fewer iterations to achieve the same quality as traditional CNN. Some initial studies show promising results for the successful use of QCNN for differentiating brain tumor subtypes,56,57 suggesting a future for quantum-enhanced diagnostics in patients with brain tumors.
In summary, quantum computers offer unprecedented possibilities in terms of computing speed and problem-solving capabilities for medical applications. On the other hand, it is essential to recognize this emerging field’s technological maturity and practical limitations. The future of quantum computing in medicine appears to be promising, but further research, development, and practical applications are needed to realize its full potential.
Technical Progress in the Field of PET Scanner Development
Recently, one of the most significant advancements in Nuclear Medicine has been introduced in the commercially available and FDA-approved long-axial field-of-view (LAFOV) PET/CT systems.3,4 The most widely implemented system is the Siemens Biograph Vision Quadra with a 106 cm LAFOV, enabling both simultaneous whole-body imaging from top of head to thigh combined with an exceptional 10-fold increase in effective sensitivity. Although a clinical PET/CT or PET/MRI system will be reasonably sufficient to meet the majority of routine clinical needs in Neuro-Oncology, LAFOV PET/CT offers immediate solutions for known limitations and a number of long-term clinical research applications of potential patient benefits.
The most accurate and recommended PET tracers for glioma are the radiolabeled amino acids, e.g. 18F-FET, with a superior lesion-to-background ratio. However, the tracer extraction across the blood–brain barrier is relatively poor, necessitating a comparatively long PET acquisition of over 20 min. The extraordinary sensitivity of LAFOV PET/CT enables either very short acquisition times, ie 0.5–5 min at the recommended injected activity for static PET acquisition, or conversely, regular acquisition times at only 10% of activity, which additionally reduces radiopharmaceutical cost (Figure 1). Apart from increasing patient throughput and diminishing movement artifacts, short scans are particularly useful in pediatric Neuro-Oncology by reducing the length or changing the type of anesthesia used, thereby diminishing the patient trauma and expense (Figure 2).
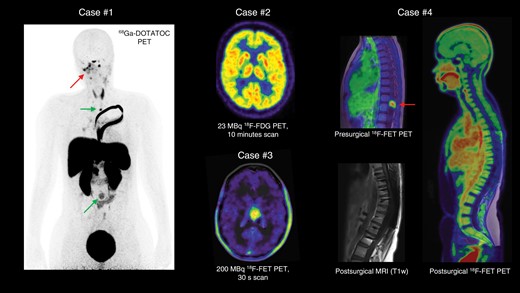
Illustrative imaging examples obtained by a long-axial field-of-view (LAFOV) PET/CT scanner displayed in maximum intensity projection.
Case #1: PET using the somatostatin receptor subtype 2 ligand 68Ga-DOTA-Tyr3-octreotide (68Ga-DOTATOC) was performed to evaluate treatment response in a 15-year-old female patient with a residual CNS WHO grade 3 meningioma at the skull base and on the right side of the neck (arrow). The scan additionally identified previously unknown metastases to the spine at 2 locations (arrows).
Case #2: With the high scanner sensitivity, a clinical quality brain 18F-FDG PET scan can be obtained at only 10–15% (23 MBq) of the recommended radioactive dose of 200 MBq (performed in a healthy volunteer; acquisition time, 10 min).
Case #3: Example of considerably short 18F-FET PET scan duration of 30 s after injection of the recommended radioactive dose (200 MBq; delay from the injection, 30 min) in a patient with an anaplastic pilocytic glioma of CNS WHO grade 3 in the left thalamus.
Case #4: A 12-year-old female patient was resected for a CNS WHO grade 1 ganglioglioma with increased 18F-FET uptake located in the medullary conus (arrow). Postoperative evaluation using anatomical MRI was obscured due to signal loss and distortions caused by metal implants in the spine from the surgical stabilization procedure (bottom). In spatial correlation, postoperative high-resolution LAFOV PET/CT using the tracer 18F-FET showed no metabolically active tumor remnants, referencing tumor activity to healthy brain tissue. [T1w = T1-weighted MRI sequence]
![High-resolution whole-body LAFOV 18F-FET PET/CT performed in general anesthesia in only 5 min in an 11-month-old male patient with a medulloblastoma. Early postoperative anatomical MRI (48 h) superimposed on the brain part (top row) revealed nonenhancing, but metabolically active remnants (arrows) lateral right to the pons (shown with physiological uptake, arrow), in the right cerebellar peduncle, and in the right cerebellar hemisphere. In maximum intensity projection, the image shows the total body activity distribution (bottom row) with the tumor in situ (arrow) and allows for screening for active drop metastases of the spine (e). [FLAIR = T2-weighted fluid-attenuation inversion recovery MRI sequence; CE-T1w = contrast-enhanced T1-weighted MRI sequence]](https://oup.silverchair-cdn.com/oup/backfile/Content_public/Journal/neuro-oncology/26/Supplement_9/10.1093_neuonc_noae078/1/m_noae078_fig2.jpeg?Expires=1750205472&Signature=blP85S0sYJne-uwNj3FOb8l7ZnKdCrICfnnLrROduLE8oQkJrM~Rfz5zJS5dOP5-D11o~vALBmhzwN-pQlOBGtkxXiQvdO4VC0cWQHZ7v3KtOLo8nlYtgNCL28gtS5UEAUTrySD1tIGzOCZ8qW-0GBD1~~d1yAdXORmM04~9VTdF4k4FoeFqdon51RyY8n26lB1eKvxVx62OVIOQ72mTO6dG2NqLIUT0BmNKq6uCHveaDpzYw8pIgEVzaymIS6S3uAru9IfWELEw2IHYyHffhSa-c0CxP0SyQlGL0ubZjMpC2ygbmM3PZqyynZY-5a5prLPsF87ZbrpPbtwsq7O0Ag__&Key-Pair-Id=APKAIE5G5CRDK6RD3PGA)
High-resolution whole-body LAFOV 18F-FET PET/CT performed in general anesthesia in only 5 min in an 11-month-old male patient with a medulloblastoma. Early postoperative anatomical MRI (48 h) superimposed on the brain part (top row) revealed nonenhancing, but metabolically active remnants (arrows) lateral right to the pons (shown with physiological uptake, arrow), in the right cerebellar peduncle, and in the right cerebellar hemisphere. In maximum intensity projection, the image shows the total body activity distribution (bottom row) with the tumor in situ (arrow) and allows for screening for active drop metastases of the spine (e). [FLAIR = T2-weighted fluid-attenuation inversion recovery MRI sequence; CE-T1w = contrast-enhanced T1-weighted MRI sequence]
Another limitation of radiolabeled amino acids is their dynamic uptake, reflecting transport. Thus, they are not metabolically trapped as 18F-FDG, nor receptor bound as DOTA-conjugated peptides for meningioma imaging (Figure 1), and the tumor uptake will be time-dependent necessitating single bed imaging making whole-body coverage of CNS tumors with possible systemic spread or seeding to the spinal cord unattainable (Figure 2). With the larger body coverage of LAFOV PET/CT, this is no longer a limitation.
Furthermore, the high sensitivity and image quality can be converted to a tremendous increase in temporal resolution (e.g. using 1-s frames) that when combined with simultaneous coverage of the brain and large central vascular structures, such as the aorta, which allows noninvasive pharmacokinetic modeling without the need for arterial cannulation. This can be achieved using traditional compartment modelling or novel techniques adapted from MRI, e.g. the Tikhonov model-free deconvolution.58 When applied to a dynamic 18F-FET PET acquisition over 40 min, conventional clinical metrics of tumor-to-background ratio and volume can be obtained, combined with detailed knowledge of quantitative in vivo tumor physiology. These insights include the tracer volume of distribution, the mean tracer transit time in tissue, tissue perfusion, and tracer extraction across the blood–brain barrier.58 These measurements may be physiologically more informative and robust than visual classifications of time-activity curves,59 and provide new insight into tumor heterogeneity. Furthermore, this method may be critical for PET tracer and drug development, as it allows for quantitative estimations of essential characteristics of drug behavior in the brain and brain tumors.
The simultaneous whole-body acquisition with high measurement accuracy provides a remarkable opportunity for studying physiological interactions between normal brain and tumor tissue, as well as internal organs during treatment interventions and disease stages. The improved scanner sensitivity may allow multitracer acquisitions on the same day by maintaining the residual tissue activity after an initial scan sufficiently low enough to cause minimal interference with a subsequently administered tracer injected at ten times the activity level. Furthermore, the sensitivity gain is particularly advantageous in immuno-PET studies using Zr-89 labeled antibodies,60 where the long physical half-life (78.4 h) combines well with the long circulation and penetration times of the biomolecule and the need for delayed postinjection imaging after 6–7 days, but at the expense of poor tissue signal and image quality, that LAFOV PET/CT can now be profoundly improved.
The main limitation of LAFOV PET/CT is the high cost corresponding tof 3 conventional clinical PET/CT systems. On the other hand, cheaper and modularly designed PET/CT systems based on other detector materials are in the pipeline and will appear commercially within the next 2 years. Furthermore, handling large data quantities is challenging yielding image reconstruction times of 4 h for a 60-frame high-resolution whole-body dynamic PET series. The time needed for handling such large data sets is likely to decrease significantly through AI-based direct reconstruction CNN approaches.61
PET Imaging of Therapeutically Relevant Targets for Novel Anticancer Agents in Gliomas
IDH PET for the Evaluation of Response to IDH Inhibitors
Clinical relevance.—
Mutations in the genes encoding for the enzymes IDH-1 and -2 are important molecular markers for characterizing gliomas according to the latest classification for CNS tumors of the World Health Organization (WHO).62 These mutations are present in many CNS WHO grade 2 and 3 diffuse gliomas. The mutant enzyme catalyzes the conversion of alpha-ketoglutarate to 2-hydroxyglutarate (2-HG), which accumulates in glioma tissue and competitively inhibits various metabolic pathways.63 Gliomas with an additional unbalanced translocation between chromosomes 1 and 19 (1p/19q codeletion) are defined as oligodendrogliomas, whereas IDH-mutant gliomas without 1p/19q codeletion are defined as astrocytomas. Currently, the standard care for the postoperative treatment of patients with IDH-mutant grades 2 and 3 gliomas with a risk constellation (ie age > 40 years, neurological deficits, incomplete resection) is a combination of radiotherapy and alkylating chemotherapy.64 Of note, this regimen may be associated with relevant neurocognitive dysfunction and other potentially severe adverse effects.
In the last decade, a novel class of therapeutics has been developed aiming at the disruption of the activity of epigenetic regulators in IDH-mutant gliomas.65 These therapeutics include direct mutant IDH inhibitors, histone deacetylase inhibitors, bromodomain inhibitors, poly-ADP ribose polymerase inhibitors, and nicotinamide phosphoribosyl transferase inhibitors.66
Recently, vorasidenib, a dual inhibitor of the mutant IDH-1 and IDH-2 enzymes, has shown encouraging results. The phase 3 INDIGO trial tested vorasidenib in patients with residual or recurrent CNS WHO grade 2 gliomas against a placebo. The main finding was that the progression-free survival and the time to the next intervention (e.g. radiotherapy) was significantly prolonged.5 In a perioperative phase 1 trial, patients with recurrent CNS WHO grade 2 or 3 gliomas were randomized to treatment with vorasidenib, the IDH-1 inhibitor ivosidenib (AG-120), or no treatment before surgery. Both agents were well tolerated and reduced 2-HG concentrations by more than 90%.67 A retrospective study investigated ivosidenib in patients with progressive CNS WHO grade 2 or 3 gliomas.68 Responders showed a significant reduction in tumor growth rates and volume reductions after 5 months of treatment. The authors concluded that ivosidenib is useful in controlling tumor growth, potentially delaying more toxic therapies in this group of patients.
Noninvasive imaging of IDH mutations.—
The IDH mutation and its oncometabolites such as 2-HG are of considerable interest for noninvasive diagnostic imaging. One approach is proton MR spectroscopy, which enables the detection of the oncometabolite 2-HG.69 Unfortunately, the 2-HG signal is relatively small, this technique is prone to susceptibility artifacts, and may even be false-positive in 20% of patients with newly diagnosed IDH-wildtype glioblastoma.70
Radiolabeled amino acids are currently the preferred tracers for glioma imaging using PET.24 Uptake parameters derived from static and dynamic amino acid PET acquisition, and combinations thereof,71 showed some correlation with the IDH-mutational status, but the diagnostic accuracy remains limited.72 For example, in 153 patients with newly diagnosed CNS WHO grade 2 or 3 IDH-mutant gliomas molecularly characterized according to the most recent WHO classification, increased 11C-MET uptake could be observed in 73% of gliomas, whereas 21% were iso- or even hypometabolic (6%).73 Despite the undisputed additional clinical value of radiolabeled amino acids for evaluating response compared to anatomical MRI as proposed by our group8,26,41,74 and others,75 the lack of uptake in a considerable fraction of these tumors may limit the usefulness of amino acid PET for assessing response to IDH inhibitors. This should be considered if this technique is considered for this indication in upcoming clinical trials.
A diagnostic accuracy of 93% for predicting the IDH genotype could be achieved in untreated tumors using textural features radiomics derived from static and dynamic 18F-FET uptake parameters.44 Similar results could be obtained in a subsequent radiomics study.76 The role of this approach in pretreated gliomas and for response assessment warrants further investigation.
IDH PET.—
While radiolabeled amino acids provide only indirect evidence for the presence of an IDH mutation, PET imaging using selective tracers for IDH mutations would offer definite advantages for clinical applications. Uptake changes of these tracers could be used as surrogate parameters for response to specific agents targeting IDH mutations. Furthermore, the noninvasive detection of mutated IDH proteins may be helpful in the differential diagnosis of newly diagnosed brain lesions and delineating glioma extent. Meanwhile, many potential compounds targeting IDH mutations are available for labeling with positron emitters, and it is beyond the scope of this review to summarize the physiological prerequisites and chemical properties of these molecules. For a more detailed overview, we refer to a recent review article.77
Recently, successful labeling of the IDH-1 inhibitor ivosidenib (AG-120) with the positron emitter F-18 has been reported.78 Besides, 18F-AG120 showed excellent metabolic stability in vivo. However, there was no significant difference in tracer uptake between IDH-mutant and IDH-wildtype tumors.78 Similar findings were reported for 18F-AGI-5198, a potent and selective inhibitor of the mutant IDH-1 enzyme.79 These findings are in line with our group’s preclinical experiments. A significantly different uptake of the IDH PET tracer 18F-FE-mIDH80 in human U-87 wildtype and IDH-mutant gliomas implanted into the chicken embryo brain and onto the chorio-allantoic-membrane could also not be observed (Figure 3).
![Uptake of the IDH PET tracer 18F-FE-mIDH (4-(((6-[18F]fluoro-2-oxo-1,2-dihydrochinolin-3-yl)methyl)amino)-2-(2-fluorethoxy)benzonitrile)80 in chicken embryos bearing human U-87 tumors in the brains and on the chorio-allantoic-membrane (CAM), respectively. (A and B) Nuclear staining and autoradiography of a U-87 IDH-1 R132H mutant tumor-bearing brain; (C and D) nuclear staining and autoradiography of U-87 IDH-1 wildtype tumor-bearing brain. Tumoral tracer uptake does not exceed the uptake in normal brain tissue. Sixty minutes after tracer injection, no difference in uptake between wildtype and mutant IDH-1 tumor is visible. (E and F) Time-activity curves of 1 chicken embryo’s various organs and CAM tumors. The uptake kinetics in the wild type and IDH-1 mutant tumors are slightly different.](https://oup.silverchair-cdn.com/oup/backfile/Content_public/Journal/neuro-oncology/26/Supplement_9/10.1093_neuonc_noae078/1/m_noae078_fig3.jpeg?Expires=1750205472&Signature=xJgo0UctX7V3cIpwjmzhasDUoyZU-E40Qc2qCNoVvfqEuQhG4~zTXONIxp9lXXok-lcg31k2ZMwBsoQKxz47~9JtPROhQOnM98YNJ2eFaYTzXiwG8-N08XNk1uzLY7KuW756Tr2JVg4Ay-i08lyATKG2u64y8MBvbLXUj6JsF4vMPbde3Fb1tAeksrVKUz6I365hrmzvRoOhtEJQSyQN72a7D4R6M0UHrjwTlay1D-3y~UaMVAMcb494pHlKE4HvnqZiR4oHaXwqHbbTmDzEWJVe4kgeVh6CeTQ3ZZiz5F0RFvfXpL~-lj2bASjCZakNA7-NBYpigvGnCFQfK8jZpQ__&Key-Pair-Id=APKAIE5G5CRDK6RD3PGA)
Uptake of the IDH PET tracer 18F-FE-mIDH (4-(((6-[18F]fluoro-2-oxo-1,2-dihydrochinolin-3-yl)methyl)amino)-2-(2-fluorethoxy)benzonitrile)80 in chicken embryos bearing human U-87 tumors in the brains and on the chorio-allantoic-membrane (CAM), respectively. (A and B) Nuclear staining and autoradiography of a U-87 IDH-1 R132H mutant tumor-bearing brain; (C and D) nuclear staining and autoradiography of U-87 IDH-1 wildtype tumor-bearing brain. Tumoral tracer uptake does not exceed the uptake in normal brain tissue. Sixty minutes after tracer injection, no difference in uptake between wildtype and mutant IDH-1 tumor is visible. (E and F) Time-activity curves of 1 chicken embryo’s various organs and CAM tumors. The uptake kinetics in the wild type and IDH-1 mutant tumors are slightly different.
An explanation for this discrepancy was recently provided by a biochemical and biophysical analysis investigating the binding affinity and inhibition selectivity of IDH inhibitors.81 In brief, it could be observed that a high number of IDH inhibitors bind to the IDH-1 wildtype enzyme despite not, or only weakly, inhibiting it. Thus, selective inhibition of the IDH-1 R132H mutant enzyme compared to the IDH-1 wildtype enzyme does not principally relate to the binding affinities of these agents for the resting forms of the enzymes. Instead, the independent binding of the Mg2+ and α-ketoglutarate complex to the IDH-1 R132H mutant enzyme makes this variant more susceptible to allosteric binding of IDH inhibitors, compared to tighter binding of the isocitrate-Mg2+ complex substrate to the IDH-1 wildtype enzyme.82 This indicates that the inhibition selectivity is mainly due to the higher susceptibility of the reduction of α-ketoglutarate to 2-HG catalyzed by the mutant IDH-1 enzyme to allosteric inhibition compared to the IDH-1 wildtype-catalyzed isocitrate oxidation to α-ketoglutarate (Figure 4).
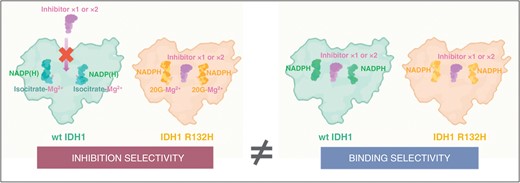
IDH inhibitors bind wildtype IDH-1 (left image in the right panel) despite not, or only weakly, inhibiting it (left image in the left panel). It has been described by Liu et al. that selective inhibition of the IDH-1 R132H mutant enzyme compared to the IDH-1 wildtype enzyme does not principally relate to the binding affinities of these agents for the resting forms of the enzymes (images in the right panel).81 Instead, the independent binding of the α-ketoglutarate (2-OG) and Mg2+ complex to the IDH-1 R132H mutant enzyme (right image in the left panel) makes this variant more susceptible to allosteric binding of IDH inhibitors, compared to tighter binding of the isocitrate-Mg2+ complex substrate to the IDH-1 wildtype enzyme (left image in the left panel). This observation indicates that the inhibition selectivity is mainly due to the higher susceptibility of the reduction of α-ketoglutarate to 2-HG catalyzed by the mutant IDH-1 enzyme to allosteric inhibition compared to the IDH-1 wildtype-catalyzed isocitrate oxidation to α-ketoglutarate. Modified, with permission, from Liu and colleagues.81
Therefore, using IDH inhibitors to develop PET ligands for IDH mutations in gliomas appears to be more difficult than originally assumed. Further research for molecules with high binding selectivity for IDH-mutant gliomas is required to develop PET ligands that are able to differentiate IDH-mutant and IDH-wildtype gliomas.
Potential Targets for Glioma Theranostics
The success of theranostics in general oncology (e.g. the use of 177Lu-DOTATATE for treating neuroendocrine tumors and 177Lu-PSMA for prostate cancer), resulting in approval of these agents by the Food and Drug Administration (FDA) and European Medicines Agency, has currently prompted efforts to translate this approach to patients with brain tumors.6 A considerable limitation in this group of patients is the limited penetration of theranostic compounds through the intact blood–brain barrier. Strategies to overcome this obstacle have been developed (e.g. focused ultrasound, convection-enhanced delivery, and local application including intraventricular injection) and, due to their complexity, are mainly performed at centers with sufficient experience.
Several first-in-human and proof-of-concept studies in gliomas have shown encouraging results, prompting further evaluation in clinical trials. To this end, a few clinical applications of theranostics in glioma patients have surpassed the preclinical level and were evaluated in clinical trials. More recent efforts currently evaluated in ongoing clinical trials are summarized in the following paragraphs.
Carbonic anhydrase XII.—
One possible target for theranostics in glioma patients is the carbonic anhydrase XII (CA XII), a transmembrane enzyme expressed in glioma cells regardless of the CNS WHO grade and not expressed in the healthy brain parenchyma. It has been shown that a high CA XII expression is associated with poorer prognosis in patients with diffuse astrocytomas.83 Accordingly, specific CA XII inhibitors may help to overcome temozolomide resistance in glioblastomas by suppressing an important resistance mechanism.84 Furthermore, in vivo experiments have shown that the monoclonal antibody 6A10 binds to CA XII with high specificity.85 When radiolabeled with Lu-177, the resulting molecule 177Lu-CHX-A’’-DTPA-6A10 Fab (177Lu-6A10) remains stable under physiological conditions.86
Based on this knowledge, an investigator-initiated phase-1 trial (ClinicalTrials.gov Identifier NCT05533242) is currently investigating the feasibility of the intracavitary treatment with 177Lu-6A10 in patients with glioblastoma and a histologically confirmed expression of CA XII to prevent or postpone local tumor relapse following standard therapy. After surgery and radiotherapy with concomitant and adjuvant temozolomide chemotherapy, patients receive the calculated 177Lu-6A10 dose in 3 fractions via intracavitary injection using an implanted reservoir. The intervention is scheduled at the earliest 6 months after tumor resection, and resection with no or minimal tumor remnant (residual contrast enhancement not more than 5 mL) is mandatory. Possible advantages of this local treatment include the application of high doses while minimizing systemic side effects such as damage to other sensitive organs (e.g. bone marrow and kidneys).
L-type amino acid transporters.—
The uptake of several radiolabeled amino acids in glioma cells is based on specific transporters of the L-type (LAT), particularly the subtypes LAT1 and LAT2.87 It has been demonstrated that the overexpression of LAT1 enables the influx of amino acid–based tracers in glioma cells,88 while at the same time, these tracers seem to be a poor efflux substrate of LAT1 resulting in retention and accumulation in glioma cells.88 Translating this approach to the concept of theranostics, the amino acid phenylalanine, which represents the amino acid portion of the diagnostic tracer 18F-FDOPA, can also be labeled with the β−-emitter I-131, thereby creating, for example, 4-l-[131I]-iodo-phenylalanine (131I-IPA) for radionuclide therapy targeting LAT transporters in glioma cells.
The initial case series indicated a considerable reduction of antitumoral activity following well-tolerated 131I-IPA therapy in patients with progressive gliomas.89,90 Furthermore, an in vitro study suggested that radionuclide therapy using 131I-IPA may have an additive cytotoxic effect on glioma cells when combined with external radiotherapy.91 More recently, the phase-1/-2 IPAX-1 multicenter trial (ClinicalTrials.gov Identifier: NCT03849105) evaluated the safety, tolerability, dosing schedule, and efficacy of 131I-IPA in combination with concurrent and second-line radiotherapy in patients with glioblastoma at first recurrence.92 Preliminary results suggested a promising median overall survival of 16.0 months.93
Another phase-1 trial initiated in 2022 (IPAX-2; ClinicalTrials.gov Identifier: NCT05450744) aims to investigate the safety profile, possible drug interactions, and general feasibility of 131I-IPA (131I-TLX101) plus the standard of care consisting of postoperative radiotherapy with concomitant and adjuvant temozolomide chemotherapy in patients with newly diagnosed glioblastoma.
Overexpressed gastrin-releasing peptide receptor.—
Another target for radionuclide therapy is the gastrin-releasing peptide receptor (GRPR), which is consistently overexpressed in glioblastoma cells.94 Blockade of GRPR leads to inhibition of tumor cell growth, most probably related to downregulation of the c-fos oncogene.95,96 Preclinical experiments demonstrated that the inhibition of GRPR combined with temozolomide results in growth inhibition and cell cycle arrest in tumor cells, suggesting that a combinational approach may be potentially helpful in treating patients with glioblastoma.97
Based on these findings, an ongoing phase-1/-2a multicenter trial (ClinicalTrials.gov Identifier: NCT03872778) aimed to evaluate the safety, tolerability, whole-body distribution, dosimetry, and antineoplastic activity of 177Lu-NeoB, a radionuclide therapy agent targeting GRPR, in glioblastomas and other solid tumors overexpressing GRPR. In addition, a phase-1b dose finding study (ClinicalTrials.gov Identifier: NCT05739942) intends to assess the safety and activity of 177Lu-NeoB in combination with radiotherapy and temozolomide in patients with newly diagnosed glioblastoma, and as monotherapy in patients with recurrent glioblastoma.
Somatostatin receptors.—
Theranostics have shown efficacy in a small series of patients with recurrent, heavily pretreated meningiomas98,99 using radionuclides with β−-radiation directed against somatostatin receptors (SSTR) and is currently also considered for gliomas expressing SSTR. Notably, more than 85% of gliomas overexpress more than 1 SSTR subtype.100
A phase-1b trial initiated in 2022 (ClinicalTrials.gov Identifier: NCT05109728) aims to investigate the safety profile and activity of 177Lu-DOTATATE in addition to radiotherapy with concomitant and maintenance temozolomide chemotherapy in patients with newly diagnosed glioblastoma and as monotherapy in patients with recurrent glioblastoma.
Outlook—Efforts of the RANO Group for PET
Clinical Guidelines and Expert Recommendations on the Use of PET in Patients With Gliomas
In recent years, numerous published studies and the increasing acceptance of PET imaging to complement anatomical MRI for various clinical applications (e.g. differential diagnosis, delineation of tumor extent, diagnosis of treatment-related changes) prompted the need for guidelines for PET imaging.
The first guideline for PET imaging in patients with glioma was published by the PET task force of the Response Assessment in Neuro-Oncology (PET/RANO) Working Group together with the European Association for Neuro-Oncology (EANO) in 2016.7 Since then, almost 500 further articles on PET imaging in glioma have been published. Hence, the overdue update of this RANO guideline paper is currently being assembled. Given an increasing number of studies reporting an added value of serial amino acid PET to assess response to various treatment options at an early timepoint,25–29,101 validated response criteria are mandatory for a structured implementation of PET imaging into clinical research and routine. Recently, a set of criteria for response assessment based on amino acid PET imaging was published the use in clinical trials enrolling participants with diffuse gliomas.1 These proposed PET RANO 1.0 criteria provide a framework that needs to be validated and, if necessary, adapted, at frequent intervals.
Furthermore, the contributions of amino acid PET to planning and monitoring radiotherapy in glioma patients have been summarized by the PET/RANO group in 2021.8 Target delineation and dose prescription are currently being evaluated in a randomized phase-2 trial comparing amino acid PET- versus MRI-guided re-irradiation in patients with recurrent glioblastoma (NOA-10/ARO 2013-1).102 In patients with glioblastoma, 18F-FET PET radiomics has recently been explored for survival prediction prior to chemoradiation103 and for differentiating pseudoprogression from actual tumor progression after chemoradiation.48 These and other new developments will be covered in the near future by the next update of the PET/RANO group radiotherapy expert consensus recommendation.
Technical Guideline for Imaging of Gliomas Using PET
In order to enhance comparability and reproducibility, procedure standards for obtaining, interpreting, and reporting the results of PET imaging in patients with glioma were developed collaboratively by the European Association of Nuclear Medicine (EANM), the Society of Nuclear Medicine and Molecular Imaging (SNMMI), the European Association of Neurooncology (EANO), and the PET/RANO group.2 This guideline aimed to achieve a high-quality imaging standard for PET in glioma using the radiolabeled amino acids 11C-MET, 18F-FET, and 18F-FDOPA. Furthermore, it might help not only to standardize imaging in neurooncological practice and clinical studies but could also contribute to reducing pitfalls in image acquisition and interpretation. Basic knowledge and exchange of experience are key to identifying, distinguishing, and avoiding these pitfalls. To this end, the PET/RANO group has just compiled a review of the challenges, limitations, and pitfalls of PET and advanced MRI in patients with brain tumors.104
Novel Tracers for Imaging of Gliomas Using PET
Novel tracers being explored for PET imaging of gliomas and to be designed to meet the needs of imaging targets for personalized therapies have been32 and will be further reviewed by the PET/RANO group. This will serve as a periodic inventory method to evaluate the growing armamentarium of PET imaging in gliomas and other brain tumors.
Conclusions
PET imaging, particularly amino acid PET, has become an essential tool in managing patients with glioma, offering valuable insights into tumor characteristics, treatment response, and patient outcomes. Furthermore, the integration of supercomputing to enhance the performance of AI and radiomics, developments in PET scanner technologies with an extended field-of-view, and innovative PET tracers and theranostics directing emerging druggable targets hold excellent promise for further enhancing the diagnostic and therapeutic capabilities in the field of Neuro-Oncology. Nevertheless, these innovations warrant further validation in the near future.
Funding
None.
Supplement sponsorship
This article appears as part of the supplement “Theranostics in CNS Tumors,” sponsored by Novartis/Advanced Accelerator Applications. The sponsor had no influence on the article content.
Conflict of interest statement
N.G.: Honoraria for lectures from Blue Earth Diagnostics and for advisory board participation from Telix Pharmaceuticals; P.L.: Honoraria for lectures from Blue Earth Diagnostics; G.R.F.: Serves as an editorial board member of Cortex, Neurological Research and Practice, NeuroImage: Clinical, Zeitschrift für Neuropsychologie, DGNeurologie, and Info Neurologie & Psychiatrie; receives royalties from the publication of the books Funktionelle MRT in Psychiatrie und Neurologie, Neurologische Differentialdiagnose, SOP Neurologie, and Therapiehandbuch Neurologie; receives royalties from the publication of the neuropsychological tests KAS and Köpps; received honoraria for speaking engagements from Deutsche Gesellschaft für Neurologie (DGN) and Forum für medizinische Fortbildung FomF GmbH; K.L.J.: Honoraria for consulting from Telix Pharmaceuticals; J.C.T.: Honoraria for lectures or consulting from Seagen and Novartis, and research grants from Novocure and Munich Surgical Imaging; All other authors reported no potential conflicts of interest.
Authorship statement
Conceptualization: N.G.; writing of manuscript drafts: N.G., P.L., M.F., J.-M.W., I.S., M.M.W., G.C., C.S., S.K., I.L., K.J.L., J.C.T.; revising manuscript: all; preparation of figures: C.S.; S.K., I.L.; approving final content of manuscript: all.
References
.