-
PDF
- Split View
-
Views
-
Cite
Cite
Shannon Coy, Jong Suk Lee, Sabrina J Chan, Terri Woo, Jacquelyn Jones, Sanda Alexandrescu, Patrick Y Wen, Peter K Sorger, Keith L Ligon, Sandro Santagata, Systematic characterization of antibody–drug conjugate targets in central nervous system tumors, Neuro-Oncology, Volume 26, Issue 3, March 2024, Pages 458–472, https://doi.org/10.1093/neuonc/noad205
- Share Icon Share
Abstract
Antibody–drug conjugates (ADCs) enhance the specificity of cytotoxic drugs by directing them to cells expressing target antigens. Multiple ADCs are FDA-approved for solid and hematologic malignancies, including those expressing HER2, TROP2, and NECTIN4. Recently, an ADC targeting HER2 (Trastuzumab-Deruxtecan) increased survival and reduced growth of brain metastases in treatment-refractory metastatic breast cancer, even in tumors with low HER2 expression. Thus, low-level expression of ADC targets may be sufficient for treatment responsiveness. However, ADC target expression is poorly characterized in many central nervous system (CNS) tumors.
We analyzed publicly available RNA-sequencing and proteomic data from the children’s brain tumor network (N = 188 tumors) and gene-expression-omnibus RNA-expression datasets (N = 356) to evaluate expression of 14 potential ADC targets that are FDA-approved or under investigation in solid cancers. We also used immunohistochemistry to measure the levels of HER2, HER3, NECTIN4, TROP2, CLDN6, CLDN18.2, and CD276/B7-H3 protein in glioblastoma, oligodendroglioma, meningioma, ependymoma, pilocytic astrocytoma, medulloblastoma, atypical teratoid/rhabdoid tumor (AT/RT), adamantinomatous craniopharyngioma (ACP), papillary craniopharyngioma (PCP), and primary CNS lymphoma (N = 575).
Pan-CNS analysis showed subtype-specific expression of ADC target proteins. Most tumors expressed HER3, B7-H3, and NECTIN4. Ependymomas strongly expressed HER2, while meningiomas showed weak-moderate HER2 expression. ACP and PCP strongly expressed B7-H3, with TROP2 expression in whorled ACP epithelium. AT/RT strongly expressed CLDN6. Glioblastoma showed little subtype-specific marker expression, suggesting a need for further target development.
CNS tumors exhibit subtype-specific expression of ADC targets including several FDA-approved for other indications. Clinical trials of ADCs in CNS tumors may therefore be warranted.
CNS tumors express multiple ADC targets including B7-H3, HER3, and NECTIN4.
Ependymoma and meningioma express HER2, while AT/RT express CLDN6.
Craniopharyngiomas strongly express B7-H3, with TROP2 and HER2 in whorled stem-like tumor cells in ACP and TROP2 uniformly expressed in PCP.
Although some CNS tumors have actionable drug targets, most are driven by molecular mechanisms that are difficult to target such as aneuploidy, tumor suppressor gene deletion, and epigenetic changes. The blood-brain-barrier and immunosuppressive tumor microenvironment further reduce drug efficacy in the CNS. ADCs may potentially overcome these challenges by combining the selectivity of monoclonal antibodies with the anti-tumor effects of chemotherapeutic payloads. Although ADCs are approved for many cancers, they have seen little investigation in CNS tumors due to concern for limited brain penetration. However, the recent discovery that Trastuzumab-Deruxtecan reduces growth of brain metastases in treatment-refractory breast cancer suggests that ADCs may be more effective against CNS tumors than previously believed, particularly in those that express target antigens. The systematic characterization of FDA-approved and investigational ADC targets presented in this paper highlights multiple opportunities for the use of ADCs in CNS tumors and could ultimately guide future clinical trials.
Numerous tumors arise in the central nervous system (CNS), developing from the diverse cell lineages of the brain parenchyma, ventricles, meninges, and neuroendocrine organs.1 A few CNS tumors have therapeutically actionable molecular alterations such as BRAF V600E2,3 and respond well to targeted therapies4,5; however, most are driven by alterations that are not readily targetable such as aneuploidy, tumor suppressor deletions, chromosomal rearrangements, or epigenetic alterations. Accordingly, while next-generation sequencing, chromosomal copy-number analysis, and individualized targeted therapy trials have been underway for years, they infrequently reveal actionable alterations and have yielded only limited improvements in the standard-of-care of CNS tumors. Immunotherapeutic approaches may potentially leverage the immune system’s ability to adaptively respond to heterogeneous tumor cell states and populations, however, these approaches face additional challenges in the CNS, including the “privileged” CNS immune system,6 tumors with low tumor mutational burden,7 and local and systemic immunosuppression.8–10 The repertoire of effective agents is further limited by the blood-brain barrier (BBB) or blood-tumor barrier (BTB), which limit penetration of chemotherapeutic agents.11–13 Novel treatment strategies that overcome these challenges and target tumors refractory to the current standard-of-care are critically needed.
Antibody–drug conjugates (ADCs) are monoclonal antibodies attached to chemotherapeutic payloads via linker molecules.14 ADCs improve the specificity and therapeutic window of cytotoxic agents by directing them to cells expressing tumor-specific antigens. Upon antigen binding, ADCs are internalized, and the linker may be cleaved, thereby releasing the cytotoxic payload. In early-generation ADCs, cytotoxic payloads typically included a drug-antibody ratio (DAR) of 3–4 and effects were limited to cells that internalized the drug; however, next-generation ADCs incorporate linkers allowing for a higher DAR (~8–10) and increased payload membrane permeability. These ADCs can have substantial bystander effects on surrounding cells that do not express the ADC target.15,16 Additionally, ADCs have pleiotropic effects on the tumor microenvironment, including inhibition of oncogenic antigen targets and immune cell recruitment.17 A major limitation of drugs that target cell-intrinsic mechanisms in signaling pathways (e.g., BRAF inhibitors) is that the oncogene targeted must be a driver in that specific cancer subtype. In contrast, ADCs may act on cells in which the target is not an oncogene per se. These targets may include a broader array of tumor-specific cell surface antigens including lineage-associated proteins like CD19 in B-cell neoplasms.18
While originally developed for hematologic malignancies,19 ADCs are increasingly used in solid cancers. An early example was trastuzumab emtansine (T-DM1) a conjugate between an anti-HER2 antibody and the microtubule inhibitor mertansine; T-DM1 has proven highly efficacious in HER2-amplified breast carcinoma.20 Currently, fourteen ADCs are FDA-approved for the treatment of human cancer targeting 11 distinct antigens. Of these, 5 have targets predominantly expressed by solid cancers, including HER2, TROP2, folate receptor 1 (FOLR1), NECTIN4, and tissue factor (TF). ADCs have seen only limited investigation in CNS tumors, due to exclusion of patients with brain metastases from solid cancer clinical trials, concerns for limited BBB/BTB penetration, and observations that early-generation ADC were generally ineffective in the CNS.21 For example, the first phase 3 ADC clinical trial in primary CNS cancer, the EGFR-targeted ADC Depatuxizumab Mafodotin, recently failed to improve overall survival (OS) in GBM,22 and trials targeting EGFRVIII have also shown limited efficacy.23 Like other early-generation ADC, Depatuxizumab Mafodotin has a low DAR (~3), and minimal bystander effect.24
However, a phase 3 trial of the next-generation HER2 ADC Trastuzumab-Deruxtecan (T-DXd) recently demonstrated dramatically improved event-free survival in treatment-refractory metastatic breast carcinoma.25 This ADC is a HER2-targeting monoclonal antibody linked to a topoisomerase-I inhibitor payload, which benefits from linker chemistry allowing greater bystander effects and a higher DAR (~8).16 While HER2 ADCs have traditionally been used to treat HER2-amplified breast carcinoma with high HER2 expression, scored as “3+” using companion IHC assays,26 T-DXd showed remarkable efficacy even in tumors with low-or-absent (undetectable) HER2 expression. Moreover, sub-group analysis showed a significant reduction in brain metastasis growth,27–31 suggesting that T-DXd has substantial efficacy in the CNS. Other next-generation ADCs including the anti-TROP2 Sacituzumab Govitecan-hziy have shown similar effects, indicating that next-generation ADC have improved efficacy in the CNS.32
These early data on next-generation ADCs suggest that the use of ADCs to treat CNS tumors warrants further study and thoughtful implementation. While prior CNS ADC trials have been targeted to oncogenic antigens, such as EGFR in GBM, it is likely that a broad range of target antigens, particularly those validated in other cancers, might indicate susceptibility to next-generation ADC. Targeting such antigens may circumvent current limitations of genomically guided personalized medicine in the CNS; namely, the absence of actionable driver targets and the difficulty of maintaining therapeutic drug concentrations over time. While the expression patterns of some ADC targets have been described in human CNS tumors, most have not been profiled in pathologically well-annotated pan-CNS tumor cohorts. In this study, we systematically characterize the expression of ADC targets in a wide range of adult and pediatric CNS tumors.
Materials and Methods
Ethics
Our research was reviewed and approved by the Institutional Review Boards (IRB) at Brigham and Women’s Hospital (BWH) and Dana-Farber Cancer Institute. The study is compliant with all relevant ethical regulations regarding research involving human tissue specimens.
Discarded human formalin-fixed paraffin-embedded (FFPE) tissue samples were used after diagnosis under excess discarded tissue protocol 2018P001627 (reviewed and managed by the Mass General Brigham Institutional Review Board), which waives the requirement for patient consent. All cases were reviewed and classified according to the revised W.H.O. 2021 Classification of Tumours of the Central Nervous System (S.C., S.S.). Samples subjected to genomic analysis were used after written informed patient consent had been obtained under Dana-Farber Cancer Institute IRB protocol #10–417. For pediatric patients, written informed consent for genomic analysis was obtained from the legally authorized representatives/guardians.
Expression Profiling
Publicly available processed pediatric brain tumor data were obtained from CPTAC (https://www.cbioportal.org). Data include bulk RNA-sequencing, whole-genome sequencing (WGS), and proteomic data (mass spectrometry).33 Oncoprints were generated using the cBioPortal Oncoprinter tool (https://www.cbioportal.org/oncoprinter). Statistics were calculated and plotted in R (v.4.2.2) using the ggplot2 (v.3.4.1) and ggpubr (v.0.6.0) packages. All statistical comparisons used a 2-sided,-unpaired, t-test with α = 0.05.
Expression profiling data from publicly available brain tumor data sets were obtained from Gene Expression Omnibus (GEO). Data sets include: GSE16581 (meningioma), GSE34824 (pediatric), GSE19404 (PNET), GSE35493 (ATRT, medulloblastoma, normal brain, PNET), GSE34771 (PCNSL), GSE36245 (adult glioblastoma), GSE33331 (adult astrocytoma), GSE16155 (ependymoma), and GSE5675 (pilocytic astrocytoma). Expression data was normalized and analyzed as previously described.34
Immunohistochemistry and Scoring
FFPE sections were de-paraffinized, rehydrated, and endogenous peroxidase activity was blocked. Antigen retrieval was performed in Dako citrate buffer 122 °C+/−2 in a pressure cooker for 45 s at 15 ± 2 PSI. Primary antibodies were incubated for 45 min at room temperature or overnight at 4 °C (see Supplementary Table S1 for details). Following wash steps, secondary antibody was added for 30 min at room temperature (labeled polymer-HRP anti-rabbit: DakoCytomation K4011 or labeled polymer-HRP anti-mouse: K4001). Slides were then incubated with DAKO DAB + solution for 3–5 min and counterstained with hematoxylin. Images were acquired using an Olympus BX41 microscope and an Olympus DP26 digital camera.
For each marker, relative IHC expression was assessed in each CNS tumor sample using an index scoring system similar to clinically validated HER2 scoring.26 Each marker was scored on a scale from 0 to 3+, with 0 = no detectable expression, 1+ = low expression in tumor cells (>10% of cells), 2+ = strong expression in scattered tumor cells (<10%) and/or moderate expression in >10% of tumor cells, and 3+ = strong expression in >10% of tumor cells (see Figure 3A for examples).
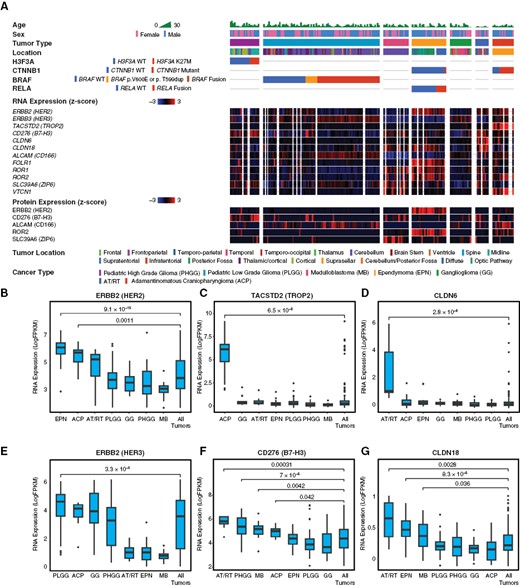
Molecular profiling of ADC targets in pediatric CNS tumors. To characterize expression of potential ADC targets in pediatric CNS tumors, we analyzed publicly available bulk-RNA sequencing, selected whole-genome sequencing (WGS), and proteomic (mass spectrometry) data from the CPTAC database. This dataset included 188 tumors from seven major subtypes, including pediatric high-grade glioma (PHGG), pediatric low-grade glioma (PLGG), medulloblastoma (MB), ependymoma (EPN), ganglioglioma (GG), atypical teratoid/rhabdoid tumor (AT/RT), and adamantinomatous craniopharyngioma (ACP). Relevant oncogenic drivers were analyzed for each histologic subtype, including H3F3A in PHGG, BRAF in PLGG, CTNNB1 in CP, and RELA in ependymoma (A). ERBB2/HER2 RNA expression was enriched relative to other tumors in EPN (P = 9.1 × 10−10) and ACP (P = .001) (B), though only EPN showed enrichment in proteomic data (A). TACSTD2/TROP2 RNA was strongly enriched in ACP (P = 6.5 × 10−8) and very low in other subtypes (C). CLDN6 RNA was strongly enriched in AT/RT (P = 2.8 × 10−6), with low expression in other subtypes (D). ERBB3/HER3 RNA showed moderate expression in multiple subtypes, with slight enrichment in PLGG (P = 3.3 × 10−6) (E). CD276/B7-H3 RNA was moderately expressed by all tumors, with relative enrichment in ACP (P = .042), AT/RT (P = .0003), MB (P = .004), and PHGG (P = 7 × 10−5) (F). CLDN18 RNA showed very low expression in all subtypes, with slight relative enrichment in AT/RT (P = .0028), EPN (P = 8.3 × 10−5), and MB (P = .036) (G). All statistical comparisons performed using a Student’s t-test, unpaired, 2-sided with α = .05.
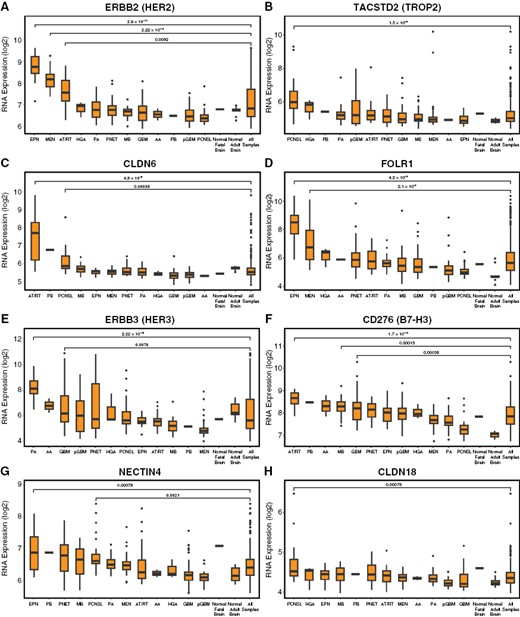
Molecular profiling of ADC targets in adult and pediatric CNS tumors. To broaden and validate our analysis of ADC targets in CNS tumors, we analyzed RNA microarray expression data from publicly available CNS tumor GEO datasets (n = 356 total tumors). We again found that ERBB/HER2 was selectively enriched in EPN (P = 2.6 × 10−10), and also found significant enrichment in meningioma (MEN, P = 2.22 × 10−16) and AT/RT (P = .009) (A) TACSTD2/TROP2 was modest in most tumors with relative enrichment in primary central nervous system lymphoma (PCNSL) (P = 1.5 × 10−6); craniopharyngiomas were not present for comparison (B). CLDN6 was strongly enriched in AT/RT (P = 4.9 × 10−6), with slight enrichment in PCNSL (P = .0003) and a single PB (C). FOLR1 was enriched in EPN (P = 4.2 × 10−9) and MEN (2.1 × 10−8) (D). HER3/ERBB3 was broadly expressed with enrichment in pilocytic astrocytoma (PA, P = 2.22 × 10−16) and to a lesser extent adult GBM (P = .0076) (E). CD276/B7-H3 showed broad expression, with relative enrichment in AT/RT (P = 1.7 × 10−10), GBM (P = .0004), and MB (.0002) (F). NECTIN4 showed broad expression with relative enrichment in EPN (P = .008) and PCNSL (P = .002) (G). CLDN18 showed low expression in all subtypes, with slight relative enrichment in PCNSL (P = .0008) (H). All statistical comparisons performed using a Student’s t-test, unpaired, 2-sided with α = .05.
Images and slides were reviewed and scored by a neuropathologist using an Olympus CX41 light microscope. Primary antibody information is available in Supplementary Table S1 and scoring data in Supplementary Table S2. For each tumor, the reviewing neuropathologist was initially blind to patient demographics and clinical information (e.g., age, sex, location), tumor subtype (e.g., IDH-mutant vs. IDH-wildtype glioblastoma), grade (e.g., W.H.O. grade 1 vs. W.H.O. grade 2 meningioma), genotype, and recurrence status. It was not possible to fully blind the reviewer to tumor diagnosis, as tumors were organized into tissue microarrays by broad tumor types (e.g., glioblastoma, meningioma, ependymoma), and tumor types are readily discernable via morphologic features in most cases.
Results
Pan-CNS Molecular Profiling Demonstrates Expression of Antibody–Drug-Conjugate Targets in CNS Tumors
To characterize the expression of ADC targets across adult and pediatric CNS tumor types, we first analyzed publicly available data from the Clinical Proteomic Tumor Analysis Consortium (CPTAC). This cohort includes most major subgroups of CNS tumors as pathologically defined by the W.H.O.,1 including gliomas, glioneuronal tumors, ependymal tumors, craniopharyngiomas, and embryonal tumors. We sought to include many lethal and difficult-to-treat tumors such as pediatric high-grade gliomas, medulloblastoma, and AT/RT, as well as lower-grade neoplasms. We accessed bulk RNA-sequencing (RNA-seq), whole-genome sequencing, quantitative proteomic (mass spectrometry), and deidentified clinical data from 188 pediatric brain tumors, including 83 pediatric low-grade glioma (PLGG), 22 pediatric high-grade glioma (PHGG), 16 medulloblastoma (MB), 28 ependymoma (EPN), 18 ganglioglioma (GG), 12 adamantinomatous craniopharyngioma (ACP), and 9 atypical teratoid/rhabdoid tumors (ATRT). We examined RNA and protein expression of ADC targets previously FDA-approved for use in solid tumors (ERBB2/HER2, TACSTD2/TROP2, FOLR1, TF/CD142) and investigational next-generation ADC targets that have shown promise in solid tumor trials (ERBB3/HER3, CD276/B7-H3, CLDN6, CLDN18, ALCAM/CD166, ROR1, ROR2, SLC39A6/ZIP6, VTCN1). NECTIN4 expression data were not available in this dataset. Our analysis showed that CNS tumors express multiple ADC targets, with distinctive expression patterns in each subtype (Figure 1A). In general, we found that ADC targets exhibited several categories of expression, including (1) highly selective enrichment in specific subtype(s); (2) broad expression with relative enrichment in some tumors; (3) ubiquitous low expression in all tumors.
In the first category, ERBB2/HER2 mRNA was highly expressed in EPN (P = 9.1 × 10−10) and ACP (P = .0011) compared to the mean expression level in all tumors (Figure 1B). While some AT/RT cases showed higher expression (LogFPKM > 5) comparable to EPN or ACP (56% of cases), mean ERBB2/HER2 expression was not significantly elevated in AT/RT (P = .28). While ERBB2/HER2 mRNA expression was elevated in both EPN and ACP, protein expression was significantly enriched only in EPN (P = 9.9 × 10−13; Supplementary Figure S1). Though modest in most cases, ERBB2/HER2 mRNA expression was higher in WT PLGG (P = .006) or those with p.V600E or p.T599dup mutations (P = .02) compared to tumors with BRAF fusions (pilocytic astrocytomas), but there was no significant difference in protein expression (Supplementary Figure S1). TACSTD2/TROP2 was selectively enriched in ACP (P = 6.5 × 10−8; Supplementary Figure S1C), while claudin 6 (CLDN6) expression was selectively elevated in AT/RT (P = 2.8 × 10−6; Figure 1D). Folate receptor 1 (FOLR1) was significantly enriched in EPN (P = 1 × 10−11; Supplementary Figure S2). The tyrosine-protein-kinase ROR1 was enriched in EPN (P = 2.2 × 10−7) and MB (P = .00068), while ROR2 was significantly enriched in EPN (P = 3.8 × 10−7) and ACP (P = .00046; Supplementary Figure S2).
In the second category of targets with broad expression, ERBB3/HER3 was moderately expressed by PLGG, PHGG, GG, and ACP, with relative enrichment in PLGG (P = 3.3 × 10−6; Figure 1E). CD276/B7-H3 was expressed by all tumors, with relative enrichment in ACP (P = .042), AT/RT (P = .0003), MB (P = .004), and PHGG (P = 7 × 10−5), and lower expression in PLGG (Figure 1F). However, PLGG with BRAF p.V600E or p.T599dup showed significantly higher CD276/B7-H3 mRNA and protein expression than wild-type or BRAF-fused tumors, suggesting they represent a distinct subset of nonpilocytic LGG (Supplementary Figure S1). Among PHGG, H3F3A K27M-mutant diffuse midline gliomas showed significant enrichment of CD276/B7-H3 relative to H3F3A-wildtype tumors (P = 0.005; Supplementary Figure S1). Tissue factor (TF/CD142) showed broad expression across tumors except AT/RT, with relative enrichment in PLGG (P = 2.5 × 10−8) and GG (P = .043; Supplementary Figure S2). The zinc transporter ZIP6/SLC39A6 was moderately expressed in all tumors with relative enrichment in AT/RT (P = .03), MB (p = .0004), and PHGG (P = .014; Supplementary Figure S2). The transmembrane glycoprotein ALCAM/CD166 was moderately expressed by most tumors, with enrichment in ACP (P = 7.2 × 10−5) and PLGG (P = 3.3 × 10−8; Supplementary Figure S2).
In the third category of targets with low expression, CLDN18 expression was low in all tumors (mean LogFPKM < 1), with slight relative enrichment in AT/RT (P = .0028), EPN (P = 8.3 × 10−5), and MB (P = .036; Figure 1G). VTCN1/B7-H4, an immune-checkpoint protein, also showed low expression in all tumors, with relative enrichment in ACP (P = .0008) and MB (P = .03; Figure S2).
We next validated these findings using an independent set of adult and pediatric CNS tumors with publicly available RNA expression data in GEO, including 356 tumors from 12 subtypes. In addition to previously analyzed subtypes, this dataset included pilocytic astrocytoma (PA) (a subgroup of PLGG), anaplastic astrocytoma, primitive neuroectodermal tumor (PNET), adult-type glioblastoma (GBM), meningioma (MEN), pineoblastoma (PB), high-grade astrocytoma, NOS (HGA), primary central nervous system lymphoma (PCNSL), and normal fetal and adult brain samples. Thus, this dataset extended our analysis to common tumors that cause substantial morbidity and mortality in adults which are only rarely seen in pediatric populations, such as meningioma and adult-type glioblastoma. Confirming our observations from the CPTAC dataset, we found that tumors exhibited similar categories of ADC target expression.
ERBB2/HER2 was again strongly enriched in EPN (P = 2.6 × 10−10) and was also elevated in MEN (P = 2.22 × 10−16) and AT/RT (P = .0092) relative to normal brain and the mean of all tumors (Figure 2A). TACSTD2/TROP2 expression was again modest in most tumors, with slight enrichment in PCNSL (P = 1.5 × 10−6); however, craniopharyngiomas were not present in this dataset for comparison (Figure 2B). CLDN6 was again strongly expressed in AT/RT (P = 4.9 × 10−6), with minimal enrichment in other tumors except for PCNSL (P = .00034), and a single PB (Figure 2C). FOLR1 was again strongly enriched in EPN (P = 4.2 × 10−9), with additional enrichment in MEN (2 × 10−8; Figure 2D). ROR1 was again enriched in EPN (P = 1.4 × 10−5) and MB (P = 2.7 × 10−5), with additional enrichment in MEN (P = 2.2 × 10−8), while ROR2 was selectively enriched in EPN (P = 4 × 10−7; Supplementary Figure S3).
Among targets with broad expression, ERBB3/HER3 was expressed by many tumors, at levels similar to normal brains, again with relative enrichment in PA (P < 2.22 × 10−16) and GBM (P = .008) (Figure 2E). CD276/B7-H3 was strongly expressed by all tumors and normal fetal brain, with relative enrichment in AT/RT (P = 1.7 × 10−10), GBM (P = .0004), and MB (P = .00015) (Figure 2F). NECTIN4 was moderately expressed by most tumors and normal brain, with relative enrichment in EPN (P = .0079) and PCNSL (P = .002; Figure 2G). TF/CD142 again showed broad expression with enrichment in PA (P < 2.22 × 10−16) and PCNSL (P = 3 × 10−5; Supplementary Figure S3). SLC39A6/ZIP6 was broadly expressed with relative enrichment in AT/RT (P = .007), MEN (P = .005), and PA (P = 4.8 × 10−7), while ALCAM/CD166 was broadly expressed by most subtypes and normal brain with enrichment in MEN (P < 1 × 10−16) and PA (P = 9.7 × 10−9; Supplementary Figure S3).
In the third category, CLDN18 was again minimally expressed by all tumors and normal brains, with slight relative enrichment in PCNSL (P = .00079; Figure 2H). VTCN1/B7-H4 showed low expression comparable to normal brain, with relative enrichment in AT/RT (P = .004), EPN (P = .0005), and MB (P = 9.7 × 10−5; Supplementary Figure S3). Thus, multiple independent datasets confirm that a wide variety of adult and pediatric CNS tumors express ADC target antigens, with distinctive expression patterns for each target.
Pan-CNS Human Tissue Immunohistochemistry Demonstrates Expression of Multiple Antibody–Drug Targets in CNS Tumors
ADC are often indicated for clinical use based on the results of IHC assays, used either as a laboratory developed test or an FDA-approved companion diagnostic, such as for HER2 protein expression.26 To confirm the expression patterns observed in profiling data and explore the possibility of using IHC assays as a biomarker for ADC target expression in CNS tumors, we performed tissue IHC assays for 6 ADC targets that have promising clinical development to date and from each different expression group (1–3), including ERBB/HER2, ERBB3/HER3, CD276/B7-H3, NECTIN4, CLDN6, and CLDN18.2, first validating with control tissues with known marker expression patterns (e.g., ovarian tumors for CLDN6, gastric tumors for CLDN18.2). For each marker, a neuropathologist scored relative IHC expression in each CNS tumor sample on a scale of 0–3+, using a system similar to clinically qualified HER2 assays, with 0 = no detectable expression, 1+ = low expression in tumor cells (>10% of tumor cells), 2+ = strong expression in scattered tumor cells (<10%) and/or moderate expression in >10% of tumor cells, and 3+ = strong expression in >10% of tumor cells (Figure 3A). For most markers, expression was present in CNS tumors at levels similar to those in solid tumors for which ADCs are under clinical investigation.35–39 HER2 companion IHC diagnostics are titrated to detect cases with HER2 gene amplification and extremely high protein levels. However, recent data show that low-level HER2 expression can result in a significant therapeutic response to T-DXd. We therefore titrated HER2 antibody to detect a broad range of HER2 levels, including those below the normal threshold for positivity in the Ventana Pathway 4B5 or Herceptest assays, as CNS tumors are not typically HER2 amplified.
Using IHC, we systemically analyzed ADC target expression in a diverse cohort of adult and pediatric CNS tumor specimens. As with RNA profiling, we sought to include representatives of each major histologic subgroup and those that have limited treatment options. Formalin-fixed-paraffin-embedded (FFPE; 5 μm) specimens were available as tissue microarrays or whole-slide-sections (n = 575 total tumors). Tumors included IDH-wildtype (IDH-WT) and IDH-mutant GBM, oligodendroglioma (OLIG), anaplastic oligodendroglioma (AOLIG), WHO grade 1 meningioma (MEN1), atypical meningioma (MEN2), anaplastic meningioma (MEN3), chordoid meningioma (cMEN), rhabdoid meningioma (rMEN), medulloblastoma (MB), ependymoma (EPN), pilocytic astrocytoma (PA), AT/RT, adamantinomatous craniopharyngioma (ACP), papillary craniopharyngioma (PCP), solitary fibrous tumor, and primary central nervous system lymphoma (PCNSL; Figure 3B).
We first analyzed pediatric brain tumors for which few treatments are available and data regarding ADC target protein expression is limited (Figure 4). As with molecular profiling data, ependymoma (n = 44) often exhibited strong HER2 (2+/3+ = 56% of cases) and HER3 expression (2+/3+ = 66%), with additional expression of B7-H3 (0/1+ = 86%, 2+/3+ = 14%), and NECTIN4 (0/1+ = 84%, 2+ = 16%). PA (n = 23) demonstrated moderate HER3 (2+ = 65%) and weak NECTIN4 (1+ = 65%). AT/RT (n = 9) showed strong CLDN6 expression (2+/3+ =55%), HER3 (3+ = 89%), and B7-H3 (3+ =78%), and weak-moderate NECTIN4 (1+ = 56%, 2+ = 44%). ACP (n = 15) is spatially organized into basaloid epithelium, cyst-lining keratinized epithelium, and whorled regions thought to represent stem-like signaling compartments.40 We observed exceptionally strong TROP2 expression in whorled epithelium (3+ = 100%), and weak HER2 (1+ = 100%) and moderate-strong HER3 (2+/3+ = 100%) in these regions. Conversely, B7-H3 and NECTIN4 were expressed by basaloid and cyst-lining epithelium (2+/3+ = 100%). PCP (n = 3) showed strong expression of B7-H3, TROP2, HER3, and NECTIN4 (2+/3+ = 100%), with weak HER2 (1+ = 100%). PCNSL showed strong HER3 expression (2+/3+ = 100%), and weak-moderate NECTIN4 (1+ = 58%, 2+ = 42% Supplementary Figure S4). These findings, along with those in meningioma and medulloblastoma, suggest that tumors may exhibit region, state, and lineage-specific ADC target expression, perhaps serving as a rationale for the use of combination therapy.
We then turned to common adult and pediatric brain tumors including GBM, MEN, and MB, which are typically stratified into molecular and histologic subgroups. GBM (n = 144) exhibited strong ERBB3/HER3 (2+/3+ = 70%) and low-moderate CD276/B7-H3 (1+ = 58%, 2+ = 16%) and NECTIN4 (1+ = 27%, 2+ = 27%; Figure 5A). Sub-group analysis of 58 clinically and molecular-profiled primary IDH-WT GBM showed no obvious correlation between ADC target expression and clinicopathologic variables such as genotype, outcome, or MGMT status (Figure 5B, Supplementary Figure S4). As with molecular profiling, meningiomas (n = 234) frequently demonstrated weak-moderate HER2 expression (1+ = 67%, 2+ = 19%) and strong HER3 (2+/3+ = 85%), moderate B7-H3 (1+ = 41%, 2+ = 40%, 3+ = 15%), and NECTIN4 (1+ = 60%, 2+ = 21%; Figure 5A). While most meningiomas were TROP2 negative, stratification by histologic subtype showed that secretory meningiomas (n = 6, ~2% of cases) have moderate-strong TROP2 expression (Figure 5C). Medulloblastoma (n = 49) were notable for moderate B7-H3 (1+ = 31%, 2+ = 35%), HER3 (1+ = 65%, 2+ = 27%) and NECTIN4 (1+ = 33%) (Figure 5A). Stratification of medulloblastomas into histologic and molecular subtypes showed that SHH-group tumors typically exhibited lower HER3 and B7-H3 expression (Figure 5D). A comparison of primary and recurrent glioblastoma specimens showed no significant difference in expression for any of the markers assessed, suggesting that ADC antigenicity remains intact following standard-of-care TMZ/radiation treatment (Supplementary Figure S4).
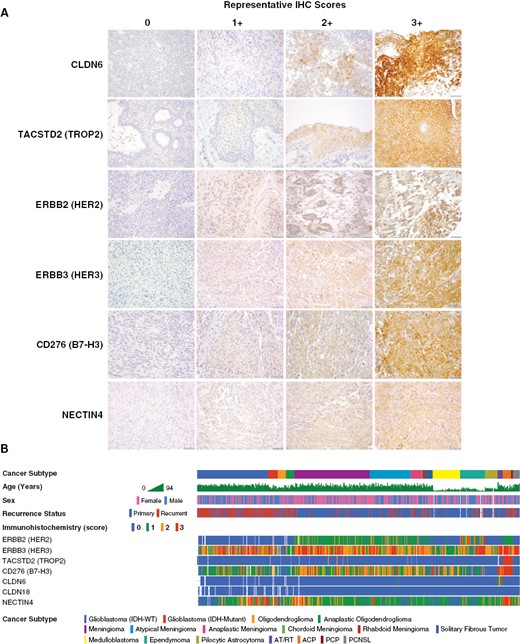
Immunohistochemical analysis of ADC target protein expression in human tumor tissue. To directly analyze ADC target antigen expression in human tumor tissues and explore the feasibility of antibody markers for clinical screening, we developed immunohistochemistry (IHC) assays for a selected group of ADC targets, including HER2, HER3, TROP2, B7-H3, NECTIN4, CLDN6, and CLDN18.2. Target marker expression was semi-quantitatively (0–3+) scored by a pathologist, with the same scoring system and relative intensity for any tumor type. Representative staining for each marker is shown in samples from AT/RT (CLDN6), craniopharyngioma (TROP2), ependymoma (HER2), and meningioma (HER3, NECTIN4, B7-H3) specimens (A). A cohort of 575 human CNS tumors from diverse histologic subtypes were stained by IHC using tissue microarrays (TMA) and whole-slide sections (WSS). These data were correlated with clinicopathologic data for each case, showing distinctive patterns of ADC target marker expression in each subtype (B). Scale bars 50 μm.
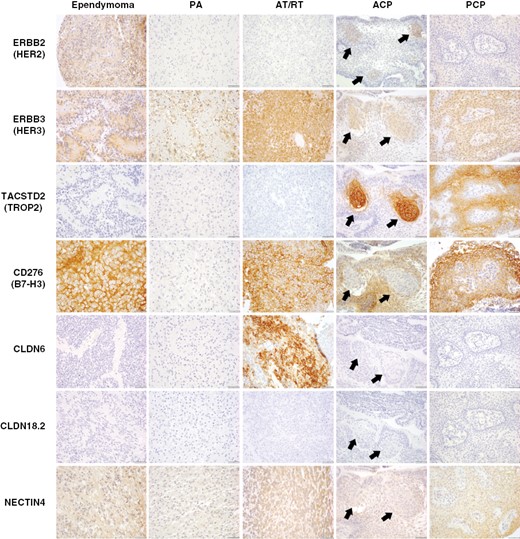
Characterization of ADC target proteins in pediatric and rare brain tumors. Immunohistochemistry for ADC targets was performed in a broad panel of pediatric CNS tumors, including pilocytic astrocytoma (PA), ependymoma (EPN), atypical teratoid/rhabdoid tumor (AT/RT), adamantinomatous craniopharyngioma (ACP), and papillary craniopharyngioma (PCP). HER2 showed moderate-strong expression in ependymoma, as well as region-specific expression in whorled epithelial cells in ACP (arrows). HER3 was expressed by all tumor subtypes, with frequent strong expression in ependymoma, AT/RT, and PCP, as well as expression in whorled cells in ACP. TROP2 showed strong/diffuse expression in all PCP examined, and exceptionally strong expression in whorled epithelial cells in ACP. B7-H3 was moderately to strongly expressed by all tumors except PA. In contrast to prior markers, B7-H3 was expressed by basaloid rather than whorled cells in ACP. CLDN6 was uniquely and strongly expressed by AT/RT. No expression of CLDN18.2 was identified in any tumor subtype. NECTIN4 typically showed weak-moderate expression in each tumor subtype.
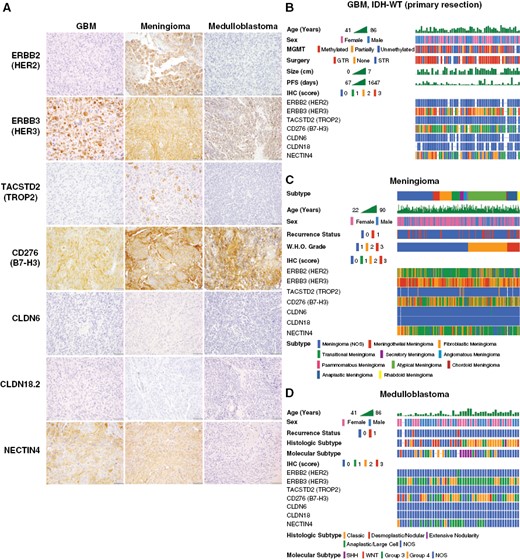
Characterization of ADC target protein expression in brain tumors by histologic and molecular subtype. Immunohistochemistry for ADC target antigens were analyzed in common adult and pediatric CNS tumors by histologic and molecular subtype, including GBM, meningioma, and medulloblastoma. GBM, meningioma, and medulloblastoma frequently show moderate-strong expression of HER3, B7-H3, and NECTIN4. There was no expression of CLDN6 or CLDN18.2 in GBM, meningioma, or medulloblastoma. Meningiomas frequently exhibited weak-moderate expression of HER2, while this antigen target was absent in GBM and medulloblastoma. While TROP2 was absent in GBM and medulloblastoma, meningiomas rarely showed expression of TROP2 in scattered tumor cells (A). Sub-group analysis of primary IDH-WT GBM cases (n = 58) showed a typical distribution of clinicopathologic characteristics but no definite correlations with ADC marker expression (B). Meningiomas (n = 234) were stratified according to WHO histologic group, revealing that rare cases with TROP2 expression are predominantly secretory meningiomas, while chordoid meningiomas show lower expression of HER2 and B7-H3 (C). Analysis of medulloblastoma cases (n = 49) by molecular and histologic subtype revealed lower expression of HER3 and B7-H3 in SHH-group tumors, with no definite correlation with histologic subtype (D).
As most tumors were analyzed via IHC staining of TMAs, to evaluate marker heterogeneity we next analyzed concordance of IHC scoring between replicate cores, which were obtained from multiple different regions of each tumor. We found high concordance in IHC scores (range, 83%–100%) for all markers between replicate cores (Supplementary Table S3), confirming that while individual tumor cells may exhibit variable expression of target antigens, tumors typically exhibit broadly consistent patterns of staining by our scoring criteria.
Further analysis of IHC scoring data confirmed that ADC target protein expression is also generally represented by three main categories: (1) selective expression, (2) broad expression, (3) ubiquitous low expression. In the first group, HER2 is strongly enriched in EPN (P = 3 × 10−15), with lower-level expression in meningiomas and craniopharyngiomas (Figure 6A). TROP2 was markedly enriched in ACP (P < 1 × 10−15) and PCP (P < 1 × 10−15), with rare expression in secretory-type meningiomas (a subgroup of MEN1/2) (Figure 6B). CLDN6 was strongly enriched in AT/RT (P < 1 × 10−15) (Figure 6C). In the second group, HER3 was broadly expressed, with relatively less expression in oligodendrogliomas and MB (Figure 6D). B7-H3 was expressed by most tumors, with relative enrichment in AT/RT (P = 5 × 10−6), ACP (P = 1 × 10−10), and PCP (P = .002) (Figure 6E). NECTIN4 showed weak-moderate expression across all tumors, with relative enrichment in ACP (P = 2 × 10−13) (Figure 6F). CLDN18.2 showed no detectable expression in any tumor (not shown).
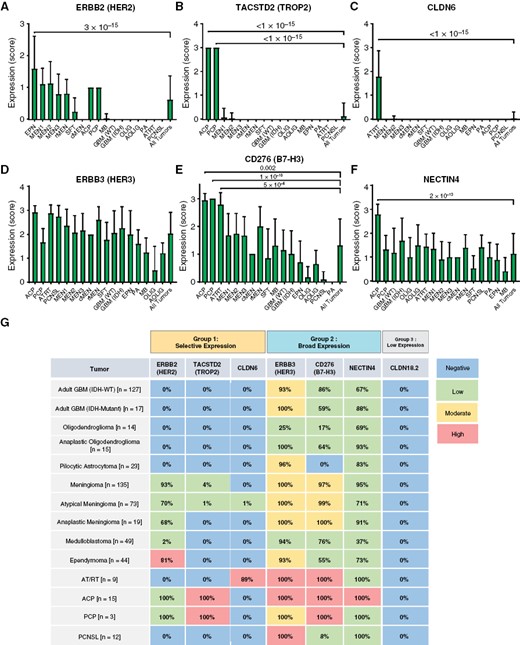
Semi-quantitative evaluation of ADC target expression in CNS tumors. ADC marker expression was compared in each subtype via semi-quantitative index scoring by a pathologist. HER2 expression was enriched in ependymoma (P = 3 × 10−15), with moderate expression in all meningioma subtypes and craniopharyngioma (A). TROP2 was strongly enriched in ACP and PCP (P < 1 × 10−15), with rare expression in meningioma (B). CLDN6 was strongly enriched in AT/RT (P < 1 × 10−15) with minimal expression in other tumors (C). HER3 was broadly expressed by all subtypes, with relatively lower expression levels in oligodendroglioma (D). B7-H3 was broadly expressed with enrichment in AT/RT (P = 5 × 10−6), ACP (P = 1 × 10−10), and PCP (P = .002) (E). NECTIN4 showed weak-moderate expression in all subtypes, with slight enrichment in AT/RT (P = 2 × 10−13) (F). Schematic representation of ADC target expression in CNS tumors. Colors indicate typical intensity of staining (IHC index score), while percentages indicate the overall number of cases with any detectable expression (IHC score = 1–3) (G). All statistical comparisons were performed using a Student’s t-test, unpaired, 2-sided with α = .05.
To further explore potential correlates of intratumoral heterogeneity for ADC target antigens, we analyzed publicly available single-cell RNA-sequencing data from 28 adult and pediatric GBM (N = 24,131 tumor cells).41 These data showed that ADC target antigens show lineage and cell-state-specific patterns of expression, with slight enrichment of HER2 in cells with astrocytic differentiation (AC-like), enrichment for ERBB3/HER3, ALCAM and TF in cells with oligodendrocyte progenitor cell-like (OPC-like), and NECTIN4 in cells with neural progenitor cell-like (NPC-like) differentiation (Supplementary Figure S5). No marker was specific for mesenchymal-like (MES-like) cells which are associated with tumor hypoxia and treatment resistance42; however, some showed strong expression across all cell states, including B7-H3/CD276 and LIV1/SLC39A6, suggesting that these may have broader effects in heterogeneous GBM tissues. These data suggest that in addition to broad profiling of ADC target marker expression in a variety of tumor subtypes, single-cell analyses of cell states may reveal underlying associations between differentiation and target marker expression that may inform treatment selection and development of resistance.
In general, our IHC results for each ADC target marker showed excellent correlation with the patterns observed in RNA sequencing data for both the CPTAC and GEO datasets for nearly all markers (Supplementary Figure S6). For tumor subtypes with high expression of target antigens by RNA profiling, we typically also observed high expression of protein by immunohistochemistry. One notable exception was HER3, for which mRNA and protein levels were not as well-matched in tumors such as AT/RT. This may indicate a biological lack of correlation between mRNA and protein levels for this antigen, a common phenomenon in a variety of settings given multiple levels of regulation between transcriptional and translational output,43 or incomplete optimization of this particular immunohistochemistry assay. While tumor samples with matched protein and RNA dataset were not available for analysis, these data nevertheless indicate that tumors exhibit recurrent patterns of targetable ADC marker expression in multiple independent cohorts by multiple analytic methodologies.
Collectively, tissue IHC data confirmed the findings from CPTAC and GEO mRNA and proteomic data, showing that a wide variety of human CNS tumors express ADC targets, with each histologic subtype moderately or highly expressing one or several ADC targets according to general expression categories (Figure 6G).
Discussion
This study represents the most comprehensive analysis conducted thus far examining the expression of ADC target antigens across a wide range of adult and pediatric CNS tumors. Using RNA profiling, proteomic data, and IHC analysis of marker expression in human tumor tissues, we have identified robust expression of ADC target antigens in CNS tumors. These include well-established targets such as HER2, TROP2, and NECTIN4, which have FDA-approved ADCs, and emerging investigational targets like CD276/B7-H3, CLDN6, and CLDN18. While some ADC targets are expressed by multiple tumor subtypes, including HER3, B7-H3, and NECTIN4, others exhibit more restricted expression in specific tumors (e.g., HER2, TROP2, CLDN6, FOLR1), while some show negligible expression across all subtypes (e.g., CLDN18, VTCN1). These findings suggest that FDA-approved drugs like Trastuzumab-Deruxtecan and Sacituzumab Govitecan-hziy, which have demonstrated clinical efficacy and safety in other contexts, may be useful in CNS tumors in the near future.
Recent trials have shown that next-generation ADCs exhibit substantial efficacy in brain metastases, suggesting that targeting these antigens in primary CNS tumors may be a promising strategy. The clearest opportunities revealed by our study are ADCs against HER2-expressing ependymoma (81% of tumors by IHC), meningioma (86%), and craniopharyngioma (100%); TROP2 in craniopharyngioma (100%) and secretory meningiomas (38%); and CLDN6 in AT/RT (89%). RNA and proteomic data also suggest additional immediate opportunities for trials, including ADC targeting FOLR1-expressing ependymoma and meningioma, ROR1-expressing ependymoma and medulloblastoma, ROR2-expressing ependymoma and ACP, and TF/CD142-expressing pediatric gliomas. CD276/B7-H3 is expressed by many solid tumors, and clinical trials are underway targeting this protein with CAR-T cells in pediatric glioma and treatment-refractory CNS tumors.44 Our study confirms that B7-H3 is expressed by many CNS tumors, most prominently meningioma, AT/RT, and craniopharyngioma.
Our data show that CNS tumors may express ADC targets as a result of lineage differentiation. For instance, ependymomas express HER2 and FOLR1, which may be selectively targetable despite having no known direct involvement in tumorigenesis. By this logic, ADCs could potentially be effective against tumor cell populations in ependymoma that: (i) lack clear oncogenic signaling targets, (ii) exhibit limited mutational burden, (iii) have minimal immune cell infiltration, (iv) are resistant to current targeted and immunotherapeutic approaches, and (v) frequently recur, leading to substantial morbidity and mortality.
By concentrating chemotherapeutic payloads at tumor sites, ADCs can potentially expand the repertoire of chemotherapeutic agents. This may enable the use of chemotherapeutic agents like topoisomerase inhibitors, which exhibit anti-tumor toxicity in vitro against high-grade tumors like GBM but have been limited by poor penetration into the CNS. Notably, clinical trials in breast carcinoma with T-DXd did not result in treatment-limiting neurotoxicity, despite efficacy against brain metastases. This suggests that next-generation ADCs containing topoisomerase payloads may have an acceptable CNS safety profile. ADCs may enable the use of single or combinations of chemotherapeutic drugs with broader mechanisms of action, raising the barrier for selective survival of resistant sub-clones within heterogeneous tumors like GBM.
In CNS tumors, drug delivery and therapeutic dose are often limited by the BBB/BTB. Accordingly, strategies or therapeutics that overcome this physiologic limitation are necessary for intra-axial tumors. Recent applications of methods such as focused ultrasound with microbubble-mediated delivery have shown promise to further enhance BBB/BTB penetration and may be applicable to ADC.45 To date, ADC trials in GBM have employed early-generation ADC with noncleavable linkers and minimal bystander effects. However, next-generation agents such as T-DXd show superior therapeutic profiles in CNS metastases, suggesting that next-generation ADC may have enhanced CNS penetration and efficacy. In principle, this may result from increased bystander effects and diffusion of payloads from regions in which the BBB/BTB is not intact, and the increased DAR of next-generation ADC. We also find that extra-axial tumors, including meningioma and craniopharyngioma, exhibit strong expression of ADC targets, and such tumors which are not behind the BBB/BTB represent attractive candidates for ADC trials.
Analysis of scRNA-seq data in glioblastoma shows that target antigen expression is correlated with underlying cell-state differentiation. Intratumoral heterogeneity in expression of ADC targets indicates that screening tumors for target expression will be essential to identify those broadly responsive to specific ADC agents. Such screening may be accomplished through transcriptional profiling, immunohistochemistry, or proteomic analyses. Emerging multiplexed tissue imaging assays additionally promise to provide quantitative measurement of multiple targets even in single tissue sections, which may capture tumors that express targets uncommon to that specific subtype.46 Immunohistochemistry and immunofluorescence approaches provide direct analysis of target antigen expression and heterogeneity and are accordingly more frequently used for clinical profiling. However, RNA sequencing panels may provide complementary high-throughput analysis and are under development in CNS tumors to predict treatment response and prognosis.47 We show strong concordance between overall protein and RNA expression across multiple independent cohorts, indicating that both methods may be feasible in CNS tumors. Furthermore, we find that target antigen expression is similar in multiple spatially distinct cores from the same tumor, suggesting that antigen expression and accordingly sensitivity to ADC treatment are relatively consistent across tumors.
While use of FDA-approved and investigational ADCs present immediate possibilities for clinical intervention, the cell surface proteins targeted by ADCs may also hold value as targets for other antibody-based therapies. For instance, bispecific antibodies, radioligand therapies, or cell-based therapies such as CAR-T and NK cells have been investigated as a means to target tumor cells expressing antigens such as CD276/B7-H3, HER2, EGFR, and others.44,48 Although most ADCs under development target antigens initially identified in non-CNS tumors, additional opportunities for ADC development will likely emerge through screening of additional antigens in CNS tumors.
Early-generation ADC were primarily designed to target tumors with extremely high levels of target antigens. However, data obtained from T-DXd trials in breast cancer suggest that cases with low or undetectable levels of antigen by conventional clinically qualified IHC assays may respond to next-generation ADC, likely due to their increased bystander effects. While previous ADC trials have relied on IHC to qualify cases with strong target expression for cohort selection, considering cases with very low expression by IHC could be beneficial in determining whether tumors with low expression of targets are still susceptible to ADC treatment. However, caution is warranted with such an approach, as applying ADCs to tumors with minimal target antigen expression may carry a higher risk of treatment failure and diminished interest in further clinical development. Accordingly, prioritizing ADCs that have demonstrated efficacy in other contexts that exhibit robust target expression, such as HER2 in ependymoma, CLDN6 in AT/RT, and TROP2 in craniopharyngioma, is likely to have a higher likelihood of success and represents a prudent path towards the early clinical development of these promising agents.
Supplementary Material
Supplementary material is available online at Neuro-Oncology (https://dbpia.nl.go.kr/neuro-oncology).
Acknowledgments
We thank Dana-Farber/Harvard Cancer Center in Boston, MA, for the use of the Specialized Histopathology Core, which provided tissue processing services.
Funding
This work was supported by National Institutes of Health grants [U2C-CA233262] (S.S., P.K.S.), [R01-CA194005] (S.S.), [R01-CA188288] (K.L.L.), [R01-CA219943] (K.L.L.), [P01-CA163205] (K.L.L.), [U54-CA210180], [P41-EB028741], [T32-CA009216] (S.C.), and by the Ludwig Center at Harvard (S.S., P.K.S.), by the Specialized Histopathology Core Facility of the Dana-Farber/Harvard Cancer Center [P30-CA06516]. K.L.L. received support from the National Brain Tumor Society (NBTS), 3000 Miles to the Cure, The Pediatric Brain Tumor Foundation (PBTF), and Accelerate Brain Cancer Cure (ABC2). P.Y.W. has received research support from Astra Zeneca/Medimmune, Beigene, Celgene, Chimerix, Eli Lily, Genentech/Roche, Kazia, MediciNova, Merck, Novartis, Nuvation Bio, Puma, Servier, Vascular Biogenics, and VBI Vaccines.
Conflict of interest statement
K.L.L. has received research support from Dana-Farber Cancer Institute from Rarecyte and Bristol Myers Squibb, consulting fees from BMS, Integragen, Blaze Biosciences, and Travera Inc., and is an equity holder and founder of Travera Inc. P.K.S. is a co-founder and member of the Board of Directors of Glencoe Software, a member of the Board of Directors for Applied Biomath, and a member of the Scientific Advisory Board for RareCyte, NanoString, and Montai Health; he holds equity in Glencoe, Applied Biomath, and RareCyte. P.K.S. is a consultant for Merck and the Sorger lab has received research funding from Novartis and Merck in the past five years. P.Y.W. has received honoraria for consulting from Astra Zeneca, Bayer, Black Diamond, Boehringer Ingelheim, Boston Pharmaceuticals, Celularity, Chimerix, Day One Bio, Genenta, Glaxo Smith Kline, Karyopharm, Merck, Mundipharma, Novartis, Novocure, Nuvation Bio, Prelude Therapeutics, Sapience, Servier, Sagimet, Vascular Biogenics, and VBI Vaccines.
Authorship statement
The study was conceived by S.C., S.S., and K.L.L. All authors contributed to writing and editing the manuscript. J.S.L. and S.C. analyzed mRNA and proteomic datasets. S.C., S.J.C., S.A., and J.J. obtained materials and annotated clinicopathologic characteristics of tumors. T.W. performed FFPE tissue sectioning, IHC assay development, and tumor staining. S.C. and S.S. reviewed and verified the histologic criteria of tumors per WHO 2021 criteria and scored IHC staining.
Data Availability
All data will be made available by the authors upon request. The publicly available processed CPTAC/CBTTC pediatric CNS tumor data in Figure 1 are available at: http://pbt.cptac-data-view.org/. The publicly available RNA microarray data in Figure 2 are available at the NCBI Gene Expression Omnibus (GEO) https://www.ncbi.nlm.nih.gov/geo/ (see Methods for datasets used). Immunohistochemistry scoring data are available in Supplementary Table S2.
References
- immunohistochemistry
- metastatic malignant neoplasm to brain
- cancer
- central nervous system neoplasms
- craniopharyngioma
- ependymoma
- glioblastoma
- antigens
- drug approval
- genes, erbb-2
- medulloblastoma
- meningioma
- receptor, erbb-2
- antibodies
- brain
- neoplasms
- primary central nervous system lymphoma
- pilocytic astrocytoma
- drug conjugates
- datasets