-
PDF
- Split View
-
Views
-
Cite
Cite
Arjun Sahgal, Mark Ruschin, Lijun Ma, Wilko Verbakel, David Larson, Paul D. Brown, Stereotactic radiosurgery alone for multiple brain metastases? A review of clinical and technical issues, Neuro-Oncology, Volume 19, Issue suppl_2, April 2017, Pages ii2–ii15, https://doi.org/10.1093/neuonc/nox001
- Share Icon Share
Abstract
Over the past three decades several randomized trials have enabled evidence-based practice for patients presenting with limited brain metastases. These trials have focused on the role of surgery or stereotactic radiosurgery (SRS) with or without whole brain radiation therapy (WBRT). As a result, it is clear that local control should be optimized with surgery or SRS in patients with optimal prognostic factors presenting with up to 4 brain metastases. The routine use of adjuvant WBRT remains debatable, as although greater distant brain control rates are observed, there is no impact on survival, and modern outcomes suggest adverse effects from WBRT on patient cognition and quality of life. With dramatic technologic advances in radiation oncology facilitating the adoption of SRS into mainstream practice, the optimal management of patients with multiple brain metastases is now being put forward. Practice is evolving to SRS alone in these patients despite a lack of level 1 evidence to support a clinical departure from WBRT. The purpose of this review is to summarize the current state of the evidence for patients presenting with limited and multiple metastases, and to present an in-depth analysis of the technology and dosimetric issues specific to the treatment of multiple metastases.
Over the past several decades, randomized trials have been conducted to enable evidence-based practice for patients presenting with limited brain metastases (1–4 tumors). These trials have focused on the role of surgery or stereotactic radiosurgery (SRS) with or without whole brain radiotherapy (WBRT). As a result, it is now clear that local control should be optimized with surgery or SRS in patients with optimal prognostic factors presenting with up to 4 brain metastases. However, the routine use of adjuvant WBRT remains a source of debate,1,2 as although distant brain control rates have been shown to be greater with WBRT, there is no impact on survival, and modern outcomes testing the effects of WBRT on cognition and quality of life (QoL) suggest a negative patient impact. This review will discuss the high-level evidence that has led to these conclusions.
With dramatic technologic advances in radiation oncology facilitating the adoption of SRS into mainstream practice, the optimal management of patients with multiple brain metastases is now being put forward. Practice is evolving to SRS alone in these patients despite a lack of level 1 evidence to support a departure from WBRT. The purpose of this review is to also summarize the current state of the evidence for patients presenting with multiple metastases, and provide an in-depth analysis of the technology and dosimetric issues specific to the treatment of multiple metastases with SRS.
The Evolution of SRS as a Sole Treatment for Patients Presenting With Limited (<5) Metastases
There have been several randomized trials intended to answer the question whether or not WBRT in addition to local aggressive therapy is needed for patients presenting with limited brain metastases.3–6 The definition of “limited” is most applicable in surgical trials to a patient presenting with a single brain metastasis5,6 but has evolved to include those with up to 4 metastases upon the advent of SRS.3,7 The primary endpoint of these trials has also evolved over time from the intent to determine the impact of WBRT on survival, to brain tumor recurrence, and most recently to a focus on neurocognition and QoL as patient-specific toxicities of WBRT.7
We have learned from the initial trials comparing WBRT alone with WBRT in combination with either SRS or surgery, that intensification of treatment to the gross tumor improves local control which may also improve overall survival.6,8,9 Although surgical trials were restricted to patients with a single metastasis, most recently Sperduto et al showed that when SRS is combined with WBRT, a survival advantage in those prognostically favorable patients (based on the graded prognostic assessment [GPA]) is observed irrespective of the number of metastases compared with WBRT alone (up to 3 metastases were included in the trial).9 Although there has been no direct comparative trial of WBRT alone to SRS alone, a recent analysis based on the Surveillance, Epidemiology, End Results program reported survival outcomes favoring SRS in patients with breast and lung cancer brain metastases.10 Therefore, the overall trend in the data supports suboptimal local tumor control and a potential negative survival impact associated with WBRT, when used as a sole modality, in patients presenting with limited brain metastases.
This assertion is further strengthened by those randomized trials comparing surgery alone with surgery plus WBRT5 and those comparing SRS alone with WBRT plus SRS.11–14 These trials report essentially equivalent survival rates, which may be a direct result of local control being optimized in both treatment arms. Therefore, at present, it is reasonable to conclude that in patients with limited brain metastases with optimal prognostic factors (ie, younger age, recursive partitioning analysis [RPA] class 1, GPA of 3.5–4.0, controlled extracranial disease), maximizing local control with SRS or surgery may improve the patients’ survival, and that WBRT as a sole treatment in these patients represents suboptimal therapy.
The impact of distant brain control, which is an arguable rationale for WBRT in the setting of SRS in patients with limited brain metastases, could only be determined in those trials comparing SRS alone with WBRT plus SRS.11,12,14,15 There are now 4 such randomized trials comprising relatively consistent populations with favorable baseline prognostic factors: most commonly a primary histology of lung cancer, up to 3 or 4 metastases at presentation, and a KPS of at least 70. However, what was inconsistent within these trials was the primary endpoint as summarized in Table 1. Importantly, none of the trials were powered for survival comparisons. The challenge with powering these trials for survival is highlighted by the Aoyama et al study, which was designed to have an 80% power to detect an absolute difference of 30% in the median survival time, with a 2-sided α level of 0.05.13 However, at the interim analysis, it was determined that a total of 805 patients would be needed to achieve this level of significance. Given that the number of patients appeared sufficient to detect a significant difference in brain tumor recurrence rates, the primary endpoint was revised and the trial reported with 132 patients randomized.
Summary of the randomized trials evaluating SRS to WBRT plus SRS for patients presenting with up to 4 metastases
RCT . | Patient Inclusion Criteria . | % Single Brain Metastases . | Primary Endpoint . | Local Control . | Distant Control . | Overall Survival . |
---|---|---|---|---|---|---|
Aoyama et al13 SRS (N = 67) vs WBRT+SRS (N = 65) | 1 to 4 metastases, KPS≥70, maximum diameter ≤3 cm | 49% vs 48% | Brain tumor recurrence | 72.5% vs 88.7% @ 1 y (P = .002) | 36.3% vs 58.5% @ 1 y (P = .003) | 28.4% vs 38.5% @ 1 y (P = .42) |
Chang et al12 SRS (N = 30) vs WBRT +SRS (N = 28) | 1 to 3 metastases, RPA 1 or 2, KPS≥70, maximum diameter ≤4 cm | 60% vs 54% | Neurocognition: HVLT-R total recall @ 4 mo | 67% vs 100% @ 1 y (P = .012) | 45% vs 73% @ 1 y (P = .02) | 63% vs 21% @ 1 y (P = .003) |
Kocher et al14 SRS (N = 100) vs WBRT+SRS (N = 99) | 1 to 3 metastases WHO ≤2, stable disease or symptomatic synchronous primary tumor | 68% vs 66% | Duration of functional independence based on a WHO ≥2 | 69% vs 81% @ 2 y (P = .04) | 52% vs 67% @ 2 y (P = .023) | Median OS (including surgical patients): 10.9 mo vs 10.7 mo (P = .89) |
Brown et al11 SRS (N = 102) vs WBRT + SRS (N = 111) | 1 to 3 metastases, diameter ≤3 cm | 55% vs 56% | Decline >1 SD from baseline on at least 1 of the 7 cognitive tests @ 3 mo | 72.8% vs 90.1% @ 1 y (P = .003) | 69.9% vs 92.3% @ 1 y (P < 0.001) | Median OS: 10.7 mo vs 7.5 mo (P = .92) |
RCT . | Patient Inclusion Criteria . | % Single Brain Metastases . | Primary Endpoint . | Local Control . | Distant Control . | Overall Survival . |
---|---|---|---|---|---|---|
Aoyama et al13 SRS (N = 67) vs WBRT+SRS (N = 65) | 1 to 4 metastases, KPS≥70, maximum diameter ≤3 cm | 49% vs 48% | Brain tumor recurrence | 72.5% vs 88.7% @ 1 y (P = .002) | 36.3% vs 58.5% @ 1 y (P = .003) | 28.4% vs 38.5% @ 1 y (P = .42) |
Chang et al12 SRS (N = 30) vs WBRT +SRS (N = 28) | 1 to 3 metastases, RPA 1 or 2, KPS≥70, maximum diameter ≤4 cm | 60% vs 54% | Neurocognition: HVLT-R total recall @ 4 mo | 67% vs 100% @ 1 y (P = .012) | 45% vs 73% @ 1 y (P = .02) | 63% vs 21% @ 1 y (P = .003) |
Kocher et al14 SRS (N = 100) vs WBRT+SRS (N = 99) | 1 to 3 metastases WHO ≤2, stable disease or symptomatic synchronous primary tumor | 68% vs 66% | Duration of functional independence based on a WHO ≥2 | 69% vs 81% @ 2 y (P = .04) | 52% vs 67% @ 2 y (P = .023) | Median OS (including surgical patients): 10.9 mo vs 10.7 mo (P = .89) |
Brown et al11 SRS (N = 102) vs WBRT + SRS (N = 111) | 1 to 3 metastases, diameter ≤3 cm | 55% vs 56% | Decline >1 SD from baseline on at least 1 of the 7 cognitive tests @ 3 mo | 72.8% vs 90.1% @ 1 y (P = .003) | 69.9% vs 92.3% @ 1 y (P < 0.001) | Median OS: 10.7 mo vs 7.5 mo (P = .92) |
Abbreviations: RCT, randomized controlled trial; SD, standard deviation; OS, overall survival; WHO, World Health Organization; HVLT, Hopkins Verbal Learning Test–Revised.
Summary of the randomized trials evaluating SRS to WBRT plus SRS for patients presenting with up to 4 metastases
RCT . | Patient Inclusion Criteria . | % Single Brain Metastases . | Primary Endpoint . | Local Control . | Distant Control . | Overall Survival . |
---|---|---|---|---|---|---|
Aoyama et al13 SRS (N = 67) vs WBRT+SRS (N = 65) | 1 to 4 metastases, KPS≥70, maximum diameter ≤3 cm | 49% vs 48% | Brain tumor recurrence | 72.5% vs 88.7% @ 1 y (P = .002) | 36.3% vs 58.5% @ 1 y (P = .003) | 28.4% vs 38.5% @ 1 y (P = .42) |
Chang et al12 SRS (N = 30) vs WBRT +SRS (N = 28) | 1 to 3 metastases, RPA 1 or 2, KPS≥70, maximum diameter ≤4 cm | 60% vs 54% | Neurocognition: HVLT-R total recall @ 4 mo | 67% vs 100% @ 1 y (P = .012) | 45% vs 73% @ 1 y (P = .02) | 63% vs 21% @ 1 y (P = .003) |
Kocher et al14 SRS (N = 100) vs WBRT+SRS (N = 99) | 1 to 3 metastases WHO ≤2, stable disease or symptomatic synchronous primary tumor | 68% vs 66% | Duration of functional independence based on a WHO ≥2 | 69% vs 81% @ 2 y (P = .04) | 52% vs 67% @ 2 y (P = .023) | Median OS (including surgical patients): 10.9 mo vs 10.7 mo (P = .89) |
Brown et al11 SRS (N = 102) vs WBRT + SRS (N = 111) | 1 to 3 metastases, diameter ≤3 cm | 55% vs 56% | Decline >1 SD from baseline on at least 1 of the 7 cognitive tests @ 3 mo | 72.8% vs 90.1% @ 1 y (P = .003) | 69.9% vs 92.3% @ 1 y (P < 0.001) | Median OS: 10.7 mo vs 7.5 mo (P = .92) |
RCT . | Patient Inclusion Criteria . | % Single Brain Metastases . | Primary Endpoint . | Local Control . | Distant Control . | Overall Survival . |
---|---|---|---|---|---|---|
Aoyama et al13 SRS (N = 67) vs WBRT+SRS (N = 65) | 1 to 4 metastases, KPS≥70, maximum diameter ≤3 cm | 49% vs 48% | Brain tumor recurrence | 72.5% vs 88.7% @ 1 y (P = .002) | 36.3% vs 58.5% @ 1 y (P = .003) | 28.4% vs 38.5% @ 1 y (P = .42) |
Chang et al12 SRS (N = 30) vs WBRT +SRS (N = 28) | 1 to 3 metastases, RPA 1 or 2, KPS≥70, maximum diameter ≤4 cm | 60% vs 54% | Neurocognition: HVLT-R total recall @ 4 mo | 67% vs 100% @ 1 y (P = .012) | 45% vs 73% @ 1 y (P = .02) | 63% vs 21% @ 1 y (P = .003) |
Kocher et al14 SRS (N = 100) vs WBRT+SRS (N = 99) | 1 to 3 metastases WHO ≤2, stable disease or symptomatic synchronous primary tumor | 68% vs 66% | Duration of functional independence based on a WHO ≥2 | 69% vs 81% @ 2 y (P = .04) | 52% vs 67% @ 2 y (P = .023) | Median OS (including surgical patients): 10.9 mo vs 10.7 mo (P = .89) |
Brown et al11 SRS (N = 102) vs WBRT + SRS (N = 111) | 1 to 3 metastases, diameter ≤3 cm | 55% vs 56% | Decline >1 SD from baseline on at least 1 of the 7 cognitive tests @ 3 mo | 72.8% vs 90.1% @ 1 y (P = .003) | 69.9% vs 92.3% @ 1 y (P < 0.001) | Median OS: 10.7 mo vs 7.5 mo (P = .92) |
Abbreviations: RCT, randomized controlled trial; SD, standard deviation; OS, overall survival; WHO, World Health Organization; HVLT, Hopkins Verbal Learning Test–Revised.
Nevertheless, we observed from these randomized trials a consistent benefit for adjuvant WBRT with respect to distant brain control, and rates ranging from 37%–70% if WBRT was omitted to 59%–92% if treated with adjuvant WBRT (Table 1). Local control was also consistently improved with adjuvant WBRT; however, local control rates were still high with SRS alone and the issues surrounding determining local control in the context of clinical trials with inconsistent response criteria, measurements still based on linear dimensions as opposed to volume, and lack of methodology to account for necrosis or pseudoprogression16 make this outcome measure not as reliable compared with distant brain control, where new metastases emerge or not. Ultimately, from the individual trials we can conclude that the overall increased intracranial control rates associated with adjuvant WBRT has not translated to a survival benefit. Moreover, a recent individual patient data meta-analysis of 3 of the 4 randomized trials (reported prior to the Brown et al trial11) observed a survival advantage in a subgroup of patients 50 years and younger treated with SRS alone.17 The hypothesis supporting this result centered on the distant brain failure rate subgroup analysis, which was observed not to be influenced in this cohort of younger patients (50 years and younger) despite treatment with adjuvant WBRT, as opposed to the older patient (>50 y) cohort where adjuvant WBRT was efficacious in reducing the risk of new brain metastases. Sahgal et al17 concluded that when a therapeutic gain in distant brain control is not observed by the adjuvant WBRT, the adverse effects of WBRT which have been shown to impact both neurocognition and QoL11,12,18 could explain the negative impact on survival. That hypothesis requires validation.
Most recently, the adverse impact on neurocognition associated with adjuvant WBRT has been confirmed by the Brown et al randomized trial.11 Similar to the initial Chang et al trial,12 they also observed greater rates of cognitive deterioration with adjuvant WBRT despite the lower risk of intracranial relapse rates based on the Hopkins Verbal Learning Test. The Brown et al trial also reported QoL outcomes, and confirmed worse QoL with adjuvant WBRT.11 This result also confirms the QoL results from the Kocher et al trial that randomized patients following either surgery or SRS to observation versus adjuvant WBRT.18 These trials have clarified that relapse is not critical to the patient-related outcomes of cognition and QoL as long as patients are monitored with serial MRI and salvaged. It is the upfront use of WBRT that is detrimental.1
At present, it would be reasonable to conclude that adjuvant WBRT results in harm to both neurocognition and QoL and does not improve survival. These results have resulted in statements supporting SRS alone by international organizations such as the American Society for Therapeutic Radiation Oncology (ASTRO)19 and the National Comprehensive Cancer Network,20 in addition to experts in the field,1,21 specific to patients presenting with limited metastases. What remains to be answered is the role of SRS in patients presenting with more than 4 metastases, as current clinical practice is to deliver WBRT alone. However, early adopters of SRS for multiple metastases have reported data using SRS alone in these patients.22 The purpose of this review is to summarize the current clinical experience with SRS alone for patients with 5 or more metastases, the current state of the technology to deliver such treatment, and clinical trials in progress specific to this population.
Clinical Data Specific to SRS for Patients With More Than 4 Metastases
A literature search in accordance with PRISMA was performed on Embase (1947–June 2015), Medline (1946–June 2015), and Cochrane Central databases (May 2015). The search was limited to the English language. Search words included brain metastases/brain neoplasms, brain cancer/metastases, radiosurgery, stereotactic radiosurgery, and Gamma Knife (GK) radiosurgery. In total, 3677 articles were identified (Medline = 1390, Embase = 2245, Cochrane = 42). After eliminating for duplicates (593), 3084 articles were reviewed with a visual check of the title and abstract. The visual check and removal of abstracts led to 111 articles remaining for in-depth review. From the 15 articles that met the exclusion criteria of outcomes reported specific to patients with ≥5 metastases, only 10 remained when we specified the additional requirement of reporting outcomes specific to distant brain relapse rates. These 10 articles are summarized in Table 2.22–31
Summary of those studies reporting on SRS for patients with ≥5 brain metastases that met prespecified inclusion criteria
Author (year) . | Range of Metastases/ Total Volume/ Number of Patients . | Key Patient Inclusion Criteria . | Median Follow-up . | Local Recurrence . | Distant Brain Failure (DBF)/ Time to DBF . | Overall Survival . |
---|---|---|---|---|---|---|
Yamamoto et al22 (2014) | 5–10 mets (median 6)/ mean = 3.54 cc/208 | No single met >3 cm or 10 cc Cumulative tumor volume ≤15 cc KPS ≥70 (100% no prior WBRT) | 12 mo | 1 y = 6.5% 2y = 9.8% | 1 y = 63.8% 2 y = 72%/ median = 8.04 mo | Median = 10.8 mo |
Salvetti et al23 (2013) | 5–15 (median 7)/ median per patient = 6.12 cc/ 96 | Histology other than small cell or unknown primary KPS≥70 (53% no prior WBRT) | 4.1 mo | 1 y = 15.2% 2 y = 25.1% | Crude: 41.0% /NR | 5–9 mets = median 4.8 mo, 10–15 mets = median 3.4 mo |
Rava et al24 (2013) | 10–34 (mean 11)/NR/53 | KPS≥70 (36% no prior WBRT) | NR | Crude: 13.2% | 1 y = 90%/ median = 3 mo | median = 6.5 mo |
Mohammadi et al25 (2012) | 5–20 (median 6)/ median = 3.2 cc/178 | KPS≥70 (46% no prior WBRT) | 6.2 mo | Crude: 3% | Crude: 40%/ median = 2.1 mo | median = 6.7 mo |
Grandhi et al26 (2012) | 10–28 (mean 13.2)/ median = 4.86 cc/61 | 77% KPS 90–100 (37.7% no prior WBRT) | 4 mo | 1 y = 58.3% | 1 y = 77.6%/ median = 3 mo | Median = 4 mo |
Lee et al27 (2011) | 4–14/NR /36 | Median KPS 90 (range, 60–80) (80.6% no prior WBRT) | 4.5 mo | 9 mo = 15.8% | Crude = 22.2%/ median = 4 mo | Median: 4.5 mo |
Chang et al28 (2010) | Group 2: 6–10/NR /58 Group 3: 11–15 /NR/17 Group 4: >15/NR/33 | KPS≥70 RPA 1 or 2 (Group 4, 42.4% had prior WBRT) | *Group 2: 10.7 moGroup 3: 12.3 moGroup 4: 8 mo | *Group 2: 1 y = 83% Group 3: 1 y = 92%Group 4: 1 y = 89%(Group 4, 36.8% of the 57.6% developing distantmets were within 3 mo) | *Group 2: 1 y = 47.2%/median = 8.8 moGroup 3: 1 y = 53.1%/median = 5.3 mo Group 4: 1 y = 80.3%/ median = 5.0 mo | *Group 2: 1 y = 83% Group 3: 1 y = 92%Group 4: 1 y = 88% |
Kim et al29 (2008) | 10–37 (mean 16.6)/mean 10.9 cc/26 | KPS≥70 (69% prior/ adjuvant WBRT) | NR | 6 mo = 20.6% | 6 mo = 26.9% /NR | Median = 7.8 mo |
Bhatnagar et al30 (2006) | 4–18 (median 5)/NR/205 | >3 brain mets (83% prior or adjuvant WBRT) | Mean = 8 mo | 1 y = 29% | 1 y = 43%/ median = 9 mo | Median = 8 mo |
Nam et al31 ( 2005) | 4–10/NR/46 | Not specified for this cohort | Mean = 13.3 mo | 1 y = 30.5% | 1 y = 78.1%/NR | Median = 5.4 mo |
Author (year) . | Range of Metastases/ Total Volume/ Number of Patients . | Key Patient Inclusion Criteria . | Median Follow-up . | Local Recurrence . | Distant Brain Failure (DBF)/ Time to DBF . | Overall Survival . |
---|---|---|---|---|---|---|
Yamamoto et al22 (2014) | 5–10 mets (median 6)/ mean = 3.54 cc/208 | No single met >3 cm or 10 cc Cumulative tumor volume ≤15 cc KPS ≥70 (100% no prior WBRT) | 12 mo | 1 y = 6.5% 2y = 9.8% | 1 y = 63.8% 2 y = 72%/ median = 8.04 mo | Median = 10.8 mo |
Salvetti et al23 (2013) | 5–15 (median 7)/ median per patient = 6.12 cc/ 96 | Histology other than small cell or unknown primary KPS≥70 (53% no prior WBRT) | 4.1 mo | 1 y = 15.2% 2 y = 25.1% | Crude: 41.0% /NR | 5–9 mets = median 4.8 mo, 10–15 mets = median 3.4 mo |
Rava et al24 (2013) | 10–34 (mean 11)/NR/53 | KPS≥70 (36% no prior WBRT) | NR | Crude: 13.2% | 1 y = 90%/ median = 3 mo | median = 6.5 mo |
Mohammadi et al25 (2012) | 5–20 (median 6)/ median = 3.2 cc/178 | KPS≥70 (46% no prior WBRT) | 6.2 mo | Crude: 3% | Crude: 40%/ median = 2.1 mo | median = 6.7 mo |
Grandhi et al26 (2012) | 10–28 (mean 13.2)/ median = 4.86 cc/61 | 77% KPS 90–100 (37.7% no prior WBRT) | 4 mo | 1 y = 58.3% | 1 y = 77.6%/ median = 3 mo | Median = 4 mo |
Lee et al27 (2011) | 4–14/NR /36 | Median KPS 90 (range, 60–80) (80.6% no prior WBRT) | 4.5 mo | 9 mo = 15.8% | Crude = 22.2%/ median = 4 mo | Median: 4.5 mo |
Chang et al28 (2010) | Group 2: 6–10/NR /58 Group 3: 11–15 /NR/17 Group 4: >15/NR/33 | KPS≥70 RPA 1 or 2 (Group 4, 42.4% had prior WBRT) | *Group 2: 10.7 moGroup 3: 12.3 moGroup 4: 8 mo | *Group 2: 1 y = 83% Group 3: 1 y = 92%Group 4: 1 y = 89%(Group 4, 36.8% of the 57.6% developing distantmets were within 3 mo) | *Group 2: 1 y = 47.2%/median = 8.8 moGroup 3: 1 y = 53.1%/median = 5.3 mo Group 4: 1 y = 80.3%/ median = 5.0 mo | *Group 2: 1 y = 83% Group 3: 1 y = 92%Group 4: 1 y = 88% |
Kim et al29 (2008) | 10–37 (mean 16.6)/mean 10.9 cc/26 | KPS≥70 (69% prior/ adjuvant WBRT) | NR | 6 mo = 20.6% | 6 mo = 26.9% /NR | Median = 7.8 mo |
Bhatnagar et al30 (2006) | 4–18 (median 5)/NR/205 | >3 brain mets (83% prior or adjuvant WBRT) | Mean = 8 mo | 1 y = 29% | 1 y = 43%/ median = 9 mo | Median = 8 mo |
Nam et al31 ( 2005) | 4–10/NR/46 | Not specified for this cohort | Mean = 13.3 mo | 1 y = 30.5% | 1 y = 78.1%/NR | Median = 5.4 mo |
Abbreviations: mets, metastases; NR, not reported; * data provided by corresponding author
Summary of those studies reporting on SRS for patients with ≥5 brain metastases that met prespecified inclusion criteria
Author (year) . | Range of Metastases/ Total Volume/ Number of Patients . | Key Patient Inclusion Criteria . | Median Follow-up . | Local Recurrence . | Distant Brain Failure (DBF)/ Time to DBF . | Overall Survival . |
---|---|---|---|---|---|---|
Yamamoto et al22 (2014) | 5–10 mets (median 6)/ mean = 3.54 cc/208 | No single met >3 cm or 10 cc Cumulative tumor volume ≤15 cc KPS ≥70 (100% no prior WBRT) | 12 mo | 1 y = 6.5% 2y = 9.8% | 1 y = 63.8% 2 y = 72%/ median = 8.04 mo | Median = 10.8 mo |
Salvetti et al23 (2013) | 5–15 (median 7)/ median per patient = 6.12 cc/ 96 | Histology other than small cell or unknown primary KPS≥70 (53% no prior WBRT) | 4.1 mo | 1 y = 15.2% 2 y = 25.1% | Crude: 41.0% /NR | 5–9 mets = median 4.8 mo, 10–15 mets = median 3.4 mo |
Rava et al24 (2013) | 10–34 (mean 11)/NR/53 | KPS≥70 (36% no prior WBRT) | NR | Crude: 13.2% | 1 y = 90%/ median = 3 mo | median = 6.5 mo |
Mohammadi et al25 (2012) | 5–20 (median 6)/ median = 3.2 cc/178 | KPS≥70 (46% no prior WBRT) | 6.2 mo | Crude: 3% | Crude: 40%/ median = 2.1 mo | median = 6.7 mo |
Grandhi et al26 (2012) | 10–28 (mean 13.2)/ median = 4.86 cc/61 | 77% KPS 90–100 (37.7% no prior WBRT) | 4 mo | 1 y = 58.3% | 1 y = 77.6%/ median = 3 mo | Median = 4 mo |
Lee et al27 (2011) | 4–14/NR /36 | Median KPS 90 (range, 60–80) (80.6% no prior WBRT) | 4.5 mo | 9 mo = 15.8% | Crude = 22.2%/ median = 4 mo | Median: 4.5 mo |
Chang et al28 (2010) | Group 2: 6–10/NR /58 Group 3: 11–15 /NR/17 Group 4: >15/NR/33 | KPS≥70 RPA 1 or 2 (Group 4, 42.4% had prior WBRT) | *Group 2: 10.7 moGroup 3: 12.3 moGroup 4: 8 mo | *Group 2: 1 y = 83% Group 3: 1 y = 92%Group 4: 1 y = 89%(Group 4, 36.8% of the 57.6% developing distantmets were within 3 mo) | *Group 2: 1 y = 47.2%/median = 8.8 moGroup 3: 1 y = 53.1%/median = 5.3 mo Group 4: 1 y = 80.3%/ median = 5.0 mo | *Group 2: 1 y = 83% Group 3: 1 y = 92%Group 4: 1 y = 88% |
Kim et al29 (2008) | 10–37 (mean 16.6)/mean 10.9 cc/26 | KPS≥70 (69% prior/ adjuvant WBRT) | NR | 6 mo = 20.6% | 6 mo = 26.9% /NR | Median = 7.8 mo |
Bhatnagar et al30 (2006) | 4–18 (median 5)/NR/205 | >3 brain mets (83% prior or adjuvant WBRT) | Mean = 8 mo | 1 y = 29% | 1 y = 43%/ median = 9 mo | Median = 8 mo |
Nam et al31 ( 2005) | 4–10/NR/46 | Not specified for this cohort | Mean = 13.3 mo | 1 y = 30.5% | 1 y = 78.1%/NR | Median = 5.4 mo |
Author (year) . | Range of Metastases/ Total Volume/ Number of Patients . | Key Patient Inclusion Criteria . | Median Follow-up . | Local Recurrence . | Distant Brain Failure (DBF)/ Time to DBF . | Overall Survival . |
---|---|---|---|---|---|---|
Yamamoto et al22 (2014) | 5–10 mets (median 6)/ mean = 3.54 cc/208 | No single met >3 cm or 10 cc Cumulative tumor volume ≤15 cc KPS ≥70 (100% no prior WBRT) | 12 mo | 1 y = 6.5% 2y = 9.8% | 1 y = 63.8% 2 y = 72%/ median = 8.04 mo | Median = 10.8 mo |
Salvetti et al23 (2013) | 5–15 (median 7)/ median per patient = 6.12 cc/ 96 | Histology other than small cell or unknown primary KPS≥70 (53% no prior WBRT) | 4.1 mo | 1 y = 15.2% 2 y = 25.1% | Crude: 41.0% /NR | 5–9 mets = median 4.8 mo, 10–15 mets = median 3.4 mo |
Rava et al24 (2013) | 10–34 (mean 11)/NR/53 | KPS≥70 (36% no prior WBRT) | NR | Crude: 13.2% | 1 y = 90%/ median = 3 mo | median = 6.5 mo |
Mohammadi et al25 (2012) | 5–20 (median 6)/ median = 3.2 cc/178 | KPS≥70 (46% no prior WBRT) | 6.2 mo | Crude: 3% | Crude: 40%/ median = 2.1 mo | median = 6.7 mo |
Grandhi et al26 (2012) | 10–28 (mean 13.2)/ median = 4.86 cc/61 | 77% KPS 90–100 (37.7% no prior WBRT) | 4 mo | 1 y = 58.3% | 1 y = 77.6%/ median = 3 mo | Median = 4 mo |
Lee et al27 (2011) | 4–14/NR /36 | Median KPS 90 (range, 60–80) (80.6% no prior WBRT) | 4.5 mo | 9 mo = 15.8% | Crude = 22.2%/ median = 4 mo | Median: 4.5 mo |
Chang et al28 (2010) | Group 2: 6–10/NR /58 Group 3: 11–15 /NR/17 Group 4: >15/NR/33 | KPS≥70 RPA 1 or 2 (Group 4, 42.4% had prior WBRT) | *Group 2: 10.7 moGroup 3: 12.3 moGroup 4: 8 mo | *Group 2: 1 y = 83% Group 3: 1 y = 92%Group 4: 1 y = 89%(Group 4, 36.8% of the 57.6% developing distantmets were within 3 mo) | *Group 2: 1 y = 47.2%/median = 8.8 moGroup 3: 1 y = 53.1%/median = 5.3 mo Group 4: 1 y = 80.3%/ median = 5.0 mo | *Group 2: 1 y = 83% Group 3: 1 y = 92%Group 4: 1 y = 88% |
Kim et al29 (2008) | 10–37 (mean 16.6)/mean 10.9 cc/26 | KPS≥70 (69% prior/ adjuvant WBRT) | NR | 6 mo = 20.6% | 6 mo = 26.9% /NR | Median = 7.8 mo |
Bhatnagar et al30 (2006) | 4–18 (median 5)/NR/205 | >3 brain mets (83% prior or adjuvant WBRT) | Mean = 8 mo | 1 y = 29% | 1 y = 43%/ median = 9 mo | Median = 8 mo |
Nam et al31 ( 2005) | 4–10/NR/46 | Not specified for this cohort | Mean = 13.3 mo | 1 y = 30.5% | 1 y = 78.1%/NR | Median = 5.4 mo |
Abbreviations: mets, metastases; NR, not reported; * data provided by corresponding author
From the 10 articles summarized in Table 2, consistencies are observed. First, the minimum number of metastases treated was 5 with a range of 5 to 37. In those series that provided more specific data, the median and/or mean number of metastases treated ranged from 6 to 17, and the mean intracranial total target volume ranged from 3.2 cc to 10.9 cc. The most consistent patient inclusion criterion was that of a KPS of ≥70. Only the data from Yamamoto et al22 were specific to patients with no prior WBRT, otherwise there was some proportion of patients with prior WBRT and salvaged with SRS for their ≥5 metastases. The comparability of SRS in a patient treated for salvage as opposed to de novo is unknown with respect to distant brain control rates, but may influence observed survival rates and local tumor control.32 Similarly, with the exception of Yamamoto et al,22 all the data are retrospective single institution series.
The Yamamoto et al prospective observation study22 deserves an in-depth analysis, as it is the highest quality of evidence to be reported to date, with all patients treated with SRS alone. The trial included 208 patients with 5 to 10 metastases, 531 with 2 to 4 metastases, and 455 with a single brain metastasis. The primary endpoint was overall survival with the intention to determine non-inferiority in the cohort with 5 to 10 metastases compared with the cohort with 2 to 4 metastases. Notably, the median cumulative total volume of metastases was similar between the 2 cohorts at 3.07 cc versus 3.54 cc, respectively, and a similar upper bound of the volume range at 14.96 cc versus 13.9 cc, respectively. The population was consistent with the maximum diameter of any individual tumor 3 cm or less, the majority RPA 2, KPS ≥80, and metastases secondary to a lung cancer primary.
From this trial we have learned many critical details. First and foremost is that survival is not compromised by the presence of multiple metastases given the caveat of a total intracranial volume of disease ranging from 0.02 to 13.9 cc, and the median survival in the cohort of patients with 5 to 10 metastases was 10.8 months (similar to the cohort with 2 to 4 metastases). Moreover, the rates of neurologic death did not differ between cohorts and did not exceed 10% in any of the subgroups. Therefore, one can conclude that systemic disease progression is still the predominant cause of death with the caveat of the brain metastases locally controlled with SRS. Second, local control is not influenced by the number of metastases, as local control rates were not significantly different in any of the 3 cohorts, including those with a single metastasis. Third, distant brain failure rates are lowest for patients with a single metastasis, and no significant difference was otherwise observed in patients with 2 to 10 metastases. However, an increasing tumor burden was associated with an increase in the risk of manifesting leptomeningeal disease, as the rates were highest in the 5 to 10 cohort with a 2-year rate of 21.9% versus 13.2% and 11% in the 2 to 4 cohort and single metastasis cohort, respectively. This result could also reflect the potential for imbalances within the cohorts associated with molecular subtypes within the patients with lung (ie, proportion of epidermal growth factor receptor/anaplastic lymphoma kinase–positive patients) and breast cancer (ie, proportion of human epidermal growth factor receptor 2 positive and triple negative patients) and the proportion of small cell lung cancer patients, who are known to influence the risk of developing leptomeningeal disease. Nevertheless, this finding is of significance, as whether or not adjuvant WBRT could reduce the risk of manifesting leptomeningeal disease is unknown. Lastly, the rates of grades 3 to 5 toxicity were similar and less than 5% within each cohort. It is noteworthy to mention that fewer than 10% of patients were salvaged with WBRT, and ~40% did receive additional SRS. This also highlights the importance of serial MRI follow-up when treating with SRS alone, as new metastases will arise in ~50% of the population and the rate of distant brain failure is known to increase with time. The intent of imaging surveillance following SRS, whether or not prior WBRT had been used, is to salvage patients with new metastases prior to symptoms or neurologic deterioration to maintain and optimize both function and survival.
The other articles determined from the search summarized in Table 2 compare reasonably well in general to the outcomes reported by Yamamoto et al,22 with certain exceptions. For example, within these studies the local tumor actuarial recurrence rates at 1 year range from 15.2% to 58.3%, which is higher than the 6.5% reported by Yamamoto et al22 but not so inconsistent from those rates reported in the randomized trials for up to 4 metastases (Table 1). This wide range in local tumor control rates among the data are again likely a function of the heterogeneity in the cohorts with respect to histology and definition of local tumor control; and although typically tumors greater than 4 cm are excluded from single fraction SRS, we know that local control is impacted significantly by diameter, volume, and dose prescribed.33,34 Therefore, although conclusions are difficult to draw specific to local control, it is reasonable to conclude that the data are consistent with the literature overall. Moreover, among those few studies that compared within their single institution experience local control outcomes for those with multiple metastases with those with limited metastases, no significant differences were observed.28,31 Essentially each metastasis can be considered an independent variable, and not impacted by the intent to treat multiple lesions versus even a single lesion.
With respect to distant brain failure, we observed new metastases rates ranging from 22% to 90%. This wide range is also a reflection of several factors that include heterogeneity in primary tumor type and definition of extracranial disease control status; furthermore, many of the patients had been previously treated with WBRT. In those series reporting the median time to progression, we observed a range of 2.1 to 9 months. Notably, the Chang et al study28 subdivided cohorts into patients with 6–10 metastases, 11–15 metastases, and >15 metastases. They observed a significantly greater probability of developing new brain metastases in those with >15 metastases. However, in the 58% of patients who did fail distantly, only 37% did so within 3 months. Nam et al31 also reported on a cohort with 1 to 3 metastases and found no significant difference in distant relapse rates compared with those with 4–10 metastases (similar to Yamamoto et al22). Therefore, it is reasonable that in patients with up to 10 metastases, the expected distant brain failure rate is no greater than would be expected for patients with limited brain metastases. However, in those with >10 metastases, we cannot speculate on expected outcomes, as limited data exist.
Survival outcomes are also comparable to medians ranging from 3.4 to 13 months. Even in extreme cohorts, as reported by Chang et al28 treating patients with >15 metastases and Kim et al29 treating patients with 10 to 37 metastases, a prolonged median overall survival was observed at ~8 months. With respect to prognostic factors, a better RPA classification, higher KPS, favorable histology such as breast cancer, controlled extracranial tumor, and age have been reported within these series as favorable, and these are consistent overall with the limited brain metastases literature. Only 1 of the 10 series selected observed a total intracranial target volume as a prognostic factor. Bhatnagar et al30 reported on patients with 4 to 18 metastases treated with SRS with only 17% having a history of prior WBRT. They observed a relationship with increasing total volume of metastases and worse survival, but no details as to a specific volume threshold as a prognostic factor. Among those 5 excluded studies for not reporting distant brain control, Amendola et al35 reported survival results among those patients with >10 metastases treated with SRS and observed a total volume less than 30 cc as prognostic. A prior series by Yamamoto et al36 reported in 2013 compared survival outcomes for (i) 1553 patients with 1 to 4 metastases (median N = 2 and a mean cumulative total target volume of 8.71 cc [range, 0.01–126.2 cc]) with (ii) 560 patients with 5 to 51 metastases (median N = 8, mean cumulative total target volume of 11.80 cc [range, 0.10–115.3 cc]). The median survival times were 7.9 months and 7.0 months for the 1 to 4 and 5 to 51 metastases cohorts, respectively, and comparisons did not achieve statistical significance. However, within both groups, a cumulative volume of >10 cc was prognostic for survival. This result has not been reproduced in other retrospective series, including those summarized in Table 2. Notably, this association was not observed in the prospective study by Yamamoto et al,22 which may reflect selection bias, as the median total target volumes were much smaller than in their prior retrospective series. Therefore, at this time we cannot conclude a total volume or even number of metastases to guide case selection, and it is our position that it is the suitability of SRS as determined by patient (eg, patient performance status) and brain metastases (eg, lesion size) factors that should dictate treatment decisions until we have more data. Lastly, none of the series summarized in Table 2 reported increased adverse events profiles when treating multiple metastases, with the caveat that these series are all based on GK technology (Elekta AB, Stockholm, Sweden). The potential significance of the technology platform specific to multiple metastases will be discussed in the following sections.
Technical Fundamentals of the SRS Apparatus in Common Clinical Use
The 3 major categories of state-of-the-art SRS treatment apparatus consist of the (i) GK, (ii) robotic multi-leaf collimator (MLC)–based X-band CyberKnife (CK) system (Accuray), and (iii) high-definition (HD) MLC-based S-band linear accelerator (linac) systems.
The Gamma Knife SRS System
In 2006, GK underwent a major redesign resulting in the GK Perfexion (PFX).37–39 The latest development in GK technology occurred in 2015 with the integration of an on-board stereotactic cone-beam CT (CBCT) image-guidance system, resulting in a new system named the GK Icon (GKI),40 as shown in Fig. 1. This development has been realized primarily for delivering image-guided frameless SRS and hypofractionated (2 to 5 treatments with a dose per fraction of ≥5 Gy) GK treatments.
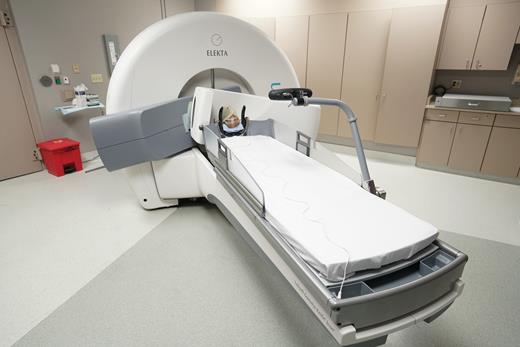
Gamma Knife Icon system with a retractable 90 kV CBCT unit mapped in submillimeter stereotactic coordinates for treatment setups. Shown is the starting position of the CBCT arm for online imaging acquisitions. This picture is provided by Dr Dheerendra Prasad, Rosswell Park, Department of Radiation Oncology.
One key physical dosimetry update in PFX and GKI systems compared with prior models is the change in the largest reference field collimator size from an 18-mm to a 16-mm field, while maintaining the smallest collimator size to 4 mm in nominal full-width at half-maximum. Because Co-60 beamlets possess well-known energy spectra with 2 distinctive gamma rays (energies 1.17 MeV and 1.33 MeV), the output factors and beamlet profiles of GK SRS have been carefully determined with Monte Carlo calculation and validated via different measurements.41–44 By simply adjusting the prescription isodose level, small lesions of 1 mm in size have been routinely and precisely targeted via frame-based GK SRS including focal areas within the brain tissue for functional disorders such as trigeminal neuralgia45 and refractory tremor.46
One of the workflow changes in the current system is the ability to preplan based on the diagnostic MRI. Once the patient is simulated in the frame, then the preplanning image is co-registered to the stereotactic planning CT or MRI and the treatment plan superimposed based on the stereotactic reference coordinates. The shots can then be adjusted to account for the actual patient position within the frame system, which may be rotated with respect to the preplanning image set. The key to the success of such an approach, given that the couch cannot rotate in 6 degrees of freedom (6-DOF) to compensate for rotations, is that the dose distribution of an individual shot is invariant to small translations due to the simultaneous exposure of 192 beams around the head focused at the isocenter. Thus, translational or rotational errors can accurately be corrected for via a simple mathematical transformation of shot locations. For the GKI, online adaptive patient positioning detection and immediate 3D dose review are based on the GKI CBCT imaging studies with again real-time interactive replanning before a treatment delivery. GKI clinical data are forthcoming to define the accuracy of the stereotactic CBCT functionality in targeting small lesions and hypofractionation for larger lesions.
The Robotic MLC-Based CyberKnife System
Robotic CK SRS has traditionally relied on tertiary collimated cone-shaped beams (ranging from 5 mm to 60 mm in diameter) to target intracranial lesions.47–49 Recently, the system has been upgraded to the M6 platform (Fig. 2), which is equipped with a HD (3.85 mm leaf width) MLC system (InCise2) that extends the treatment field to 11.5 cm × 10 cm.50 The tungsten leaves are tilted 0.5 degrees to minimize interleaf transmission. The system facilitated 100% overtravel capability, which has extended the CK system to deliver traditional MLC-based intensity modulated radiotherapy treatments of regular fractionation of 1.8 Gy/day as well as radiosurgical treatments in single or hypofractionated sessions. One of the key advantages of the M6 platform is the interchangeability of each of the tertiary collimation systems, which include the cones, variable aperture Iris collimator, and the InCise2 MLC system. The robotic arm of the system can select and attach a collimation device in the treatment room as required prior to treatment setup.
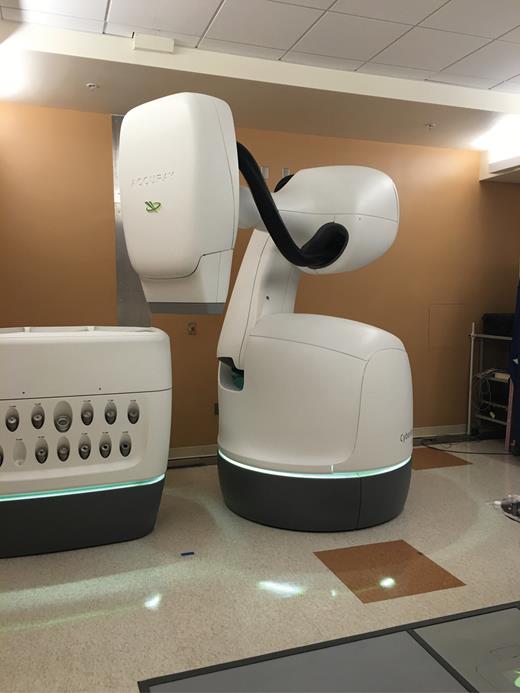
The Cyberknife M6 which is equipped with the InCise2 high definition MLC (leaf width = 3.85 mm at 80 cm SAD) allowing for a maximum clinical field size of 11.5 x 10 cm2. Picture provided by Dr Lei Wang of Stanford University, Department of Radiation Oncology.
High-Definition MLC-Based Linac SRS Systems
A new generation of digitally controlled linacs coupled with on-board image guidance, 6-DOF robotic couches, beam modulation with fast leaf-motion, flattening filter-free (FFF) X-ray beams (Fig. 3), and advanced software for treatment planning allowing for volumetric modulated arc therapy (VMAT) have enabled rapid treatment of single or multiple intracranial lesions.51,52 In VMAT, the planner specifies the arcs (either coplanar or non-coplanar) and the collimator angle and size. Objectives for dose to the tumor/planning target volume (PTV), organs at risk, and often ring structures around the tumor are set and a computer-driven optimization determines the MLC apertures that produce the dose distribution best fitting the objectives. This often involves complex segment shapes and even shielding of the target for part of the arc. The total beam-on time has further been substantially shortened due to high beam outputs on the order of 10–24 Gy/min.
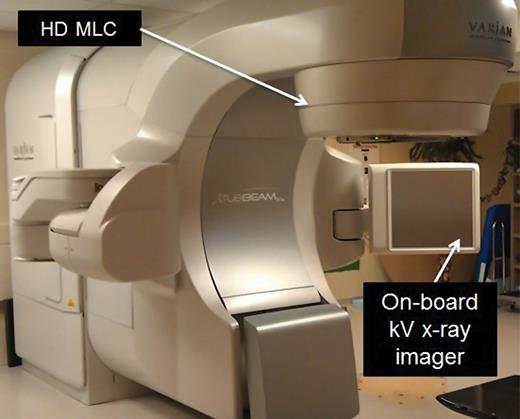
An example of a digitally controlled SRS/SRT linear accelerator system (Varian Truebeam Stx), which is equipped with a 120-leaf high-definition MLC and a kilo-voltage on-board imager. The minimum MLC leaf width is 2.5 mm for the central 32 leaf pairs (8 cm in field width) for the purpose of delivering SRS/SRT treatments.
Unlike GK and CK treatment planning systems, the majority of FFF linac photon beams are modeled and fit based on user-acquired beam data, which is a challenging process especially for narrow beams for which there is a large variation in measurement response between different instruments/detectors. Furthermore, treating at extended distances from the isocenter has raised the issue of rotational uncertainties on target coverage. It was noted that uncorrected rotational shifts may result in substantial underdosing, especially for small targets.53 As a result, adding a PTV margin is generally prudent practice for linac-based MLC SRS systems. As discussed more in depth in the next section, caution should be exhibited when using MLC to treat targets less than 10 mm in diameter, especially if treating off-axis.
Dosimetric Differences Between Major Platforms
As the number and complexity of brain metastases cases increase, so do the demands for high performance SRS systems. The previous section described the technical details of the major SRS modalities, and this section focuses on dosimetric aspects to SRS delivery, in particular for multiple metastases.
Limited (1–4) Brain Metastases
SRS treatment planning studies have shown that despite substantial differences in delivery methods and planning strategies, PFX, linac, and CK have similar dose fall-off characteristics in single-target SRS.54 An example of a relatively spherical single-target case is shown in Fig. 4. Conformality, coverage, the volume of tissue receiving 12 Gy (V12Gy), and the gradient index (GI) are near-equivalent for PFX and VMAT. The V5Gy is higher in the VMAT case (see pink isodose line), while the maximum dose in the target (Dmax) is greater for PFX. In terms of physical characteristics, PFX has a reported steep dose fall-off (penumbra) in the cranial-caudal direction: 1.6 mm (80%–20%) for a 4 mm “shot.”55 In other directions, modern modalities have reported similar penumbrae: 2.2 mm for CK for a 5-mm diameter field56; 2.8 mm for PFX (axially)55; and 2.5 mm–3 mm for narrow 6MV-linac MLC-defined fields.57,58 Translating these penumbrae into a composite plan is a complex issue involving overlapping shots (PFX) or beamlets (VMAT), in particular for multiple targets, as discussed below, but in general the literature supports all modalities as having somewhat equivalent dose fall-off for a single beam.
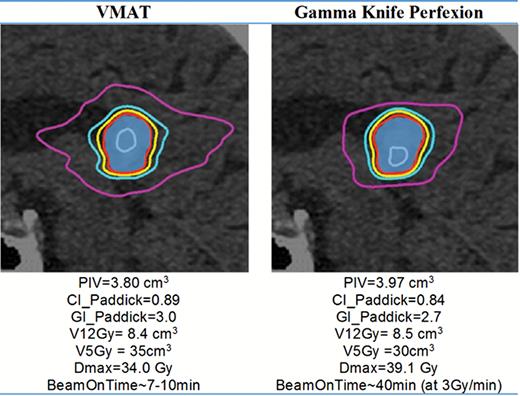
A typical dose distribution and resulting statistics for a single target shown in sagittal view. Blue colorwash = target volume; isodose lines: gray = 30 Gy, red = 20 Gy (prescribed dose); yellow = 16 Gy; light blue = 12 Gy; pink = 5 Gy. VMAT was planned using a 2-arc RapidArc Varian linac with HD 120 MLC. Plans were generated such that the target volume receiving 20 Gy was 98%.
Multiple (>4) Metastases
Multitarget (>4 targets) SRS is a complex scenario involving dose-interplay effects between targets,59 “island blocking” issues,60 multiple isocenter versus single isocenter approaches,61 etc. In the absence of evidence as to whether any modality confers a clinical benefit, SRS systems are being compared in the literature in terms of the basic planning metrics that have been shown to be predictive of toxicity, such as volume receiving 10 Gy (V10Gy), V12Gy (ie, dose fall-off), and conformality.62–64 Dose fall-off has implications for what maximum total dose can be safely prescribed for a given V12Gy (ie, “isotoxic”). In terms of dose fall-off, some studies favor PFX technology,61,65,66 while others indicate single-isocenter VMAT with the HD 120 MLC system able to yield equivalent dose fall-off.67,68 The comparisons are ultimately based on simple metric comparisons that render any conclusion specific to that comparison, as opposed to making conclusions on the ability of the apparatus in general to outperform another.
There is naturally an attraction for single isocenter VMAT treatment of multiple metastases, such as the single-isocenter dynamic conformal therapy employed by Brainlab,69 as beam-on time has been demonstrated to be substantially lower than PFX.67,70,71 Patient comfort and higher throughput are advantages of short beam-on time, whereas long treatment times may also require more imaging to ensure correct patient positioning, especially for frameless systems. The overall effort should also be considered: there is minimal delay between planning for PFX and actual beam-on time due to the rigid frame alignment and the known output rate from the56Co decay.66 In comparison, MLC calibration, isocenter fidelity checks, and patient-specific quality assurance (QA) are required for linac-based treatments, although the patient need not be present in the clinic during QA.72–75
In the absence of a definitive conclusion as to which system confers a dosimetric or clinical advantage, the focus should be on ensuring robust and accurate delivery, as treating multiple targets is a complex technique. There are 2 broad categories of QA for multitarget SRS. The first category involves small-field dosimetry. Targets <0.1 cm3 involve field sizes of <5 mm. Below a 1-cm field size, dosimetry is challenging: in 2 studies, air-filled ion chambers including pinpoint chambers exhibit deviations of >10%76 and >7%77 relative to Monte Carlo and scintillator detectors, respectively. The results of one study investigating RapidArc (arc modulated delivery, Varian Medical Systems) for SRS indicated that for a 0.4 cm3 target, their measured dose was 20% higher than the treatment planning system (TPS)–planned dose even when using a 1.25-mm dose calculation grid.78 For PFX,42,43,79–84 CK with cone inserts,56,85–94 or linac with cone inserts, small-field dosimetry is also challenging; however, the discrete nature of the fixed collimator sizes in such dedicated systems minimizes the risk of user-dependent beam modeling error and focuses more user attention to verifying a finite set of dosimetric properties rather than modeling them. The clinical relevance of over- or underdosing in the context of multiple metastases SRS is unknown, as there are limited outcome data available; however, a factor when considering systems may be how susceptible a given system is to dosimetric error.
The second area of QA is the mechanical and geometric complexity of treating multiple targets off-axis (ie, away from the linac isocenter). Recommended guidelines for isocenter variation and MLC position for SRS are ±1 mm (2-mm diameter) and 1 mm, respectively95; however, there is a lack of guidelines for off-axis delivery.96 Several studies have indicated that a substantial portion of a small (<1 cm) target can be missed for part of an MLC-defined delivery if treating >3 cm off-axis, where there are limited methods for QA of single-isocenter linac-based techniques.96,97 Furthermore, there are known concerns regarding the risk of geographical miss of small targets away from the isocenter due to uncorrected rotational errors.98 As previously mentioned, a common strategy to minimize risk of geographical miss (as well as improve dosimetric accuracy) is to use a 1- to 2-mm PTV margin, which will result in more healthy tissue irradiated and an increased risk of necrosis.99,100 Margins larger than 2 mm may ensure that the target receives the intended dose, and have been used in the setting of small targets to reach a minimal field size when using MLC-based single fraction SRS, but they are not recommended from the point of view of toxicity.100 The treatment of multiple metastases with a single isocenter is a relatively new technique for which clinical trial outcomes are yet unknown, emphasizing the importance of robust dosimetric and mechanical QA while standards and guidelines are being developed.
Whether one system confers a dosimetric advantage over another is a complex issue and the literature consists of varied metrics and nomenclature, making it difficult to draw definitive conclusions. For example, a user should be mindful of fundamental differences in applying peripheral isodose volumes such as V12Gy versus relative dose gradient metrics such as the GI in comparing and determining dose fall-off characteristics across different apparatuses, since V12Gy is also highly dependent on the prescribed dose. Additionally, most metrics, including GI, V12Gy, and conformity index (CI), are dependent on target volume. For CI, this is in part due to dose-grid resolution issues and partial volume effects.101,102 Thus any reported difference between modalities should be in the context of the existing variations and dependencies of metrics on target volume. Furthermore, dose fall-off depends on the study and on which isodose line is being interrogated. For example, V12Gy is lower for GK than VMAT in certain studies,61,66 while in other studies GK has lower V3Gy-V6Gy but higher V12Gy.67,68
To highlight these trends and present some challenges for intermodality comparisons, we present a complex and multitarget case in Fig. 5, involving 9 large brain metastases (range: 1.7 cm3 to 10.2 cm3, total target volume = 40 cm3) with a further challenge given that one of the targets was abutting the brainstem. In this example, both plans were considered clinically acceptable. The ranges of prescription/planned isodose levels were similar for GK and VMAT: 52%–54% and 54%–62%, respectively. The maximum dose of any target is indicated in Fig. 5 itself (33.4 Gy and 34.9 Gy for VMAT and GK, respectively). On average, VMAT yielded slightly higher conformality and a slightly lower V12Gy and prescription isodose volume (PIV) than GK. However, V10Gy down to V5Gy was all lower for GK relative to VMAT, as was the case in Fig. 4. In general, GK tends to yield tighter distributions at lower-intermediate isodose lines (V3Gy to V10Gy), despite having slightly elevated PIV compared with VMAT. The fractional normal brain volume receiving 3 Gy was 80% and 95% for GK and VMAT, respectively; and for 5 Gy, 38% and 61%, respectively. Thus even for this complex case of 9 large targets, an ablative dose is delivered to each tumor with a normal brain dose that is less than a single fraction of WBRT, with better low-dose sparing in the case of GK. Also note that both cases were planned to the same target volume, even though clinically a 1- to 2-mm margin may be added to targets for linac-based MLC treatments as discussed above, which would result in a substantially elevated V12Gy for the VMAT delivery. However, this was done intentionally in this exercise in order to highlight dosimetric differences intrinsic to the modalities. On the other hand, the treatment times for VMAT for multiple metastases are substantially shorter than for PFX, as shown in Fig. 5.
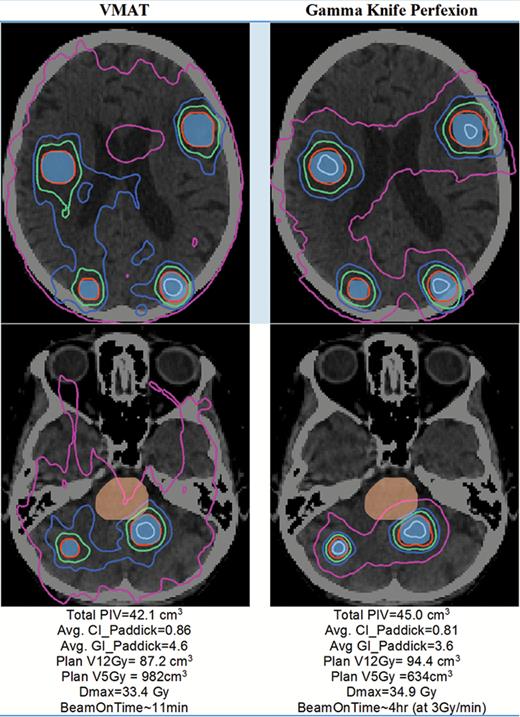
Sample dose distribution for a 9-target case shown in axial view (2 separate slices). Blue colorwash = target volume; orange colorwash = brainstem. Isodose lines: gray = 28 Gy, red = 18 Gy (prescribed dose); green = 12 Gy; blue = 9 Gy; pink = 5 Gy. VMAT was planned using 4 arcs RapidArc on a Varian linac with HD 120 MLC.
Some limitations in the literature, regarding multitarget planning, include small patient sample sizes and retrospective replanning of cases, which can both lead to biased results. Furthermore, differences in target coverage, conformality, and PIV between modalities can raise concerns about whether the dose fall-off is intrinsic to the technique, even for ideal plans, or a by-product of suboptimal plan quality in one technique or another. After accounting for target volume, further differences in dose fall-off can be realized through differences in clinical practice and technique.103–105 For example, allowing multiple non-coplanar arcs with heavy beam modulation and being willing to allow hotspots in the target in excess of 150% of the prescription may produce more rapid dose fall-off than a single arc with limited modulation and a target hotspot of <120%. Furthermore, GK is planned and prescribed fundamentally differently than VMAT, and it is not surprising that there is variability in the literature regarding dose fall-off due to planning practice differences.
In conclusion, the dose fall-off resulting from treating multiple targets is a complex matter involving dose-interplay effects, target heterogeneity, planning, and delivery methodology. Although dosimetric differences have been observed that may favor one technology over another, there are no clinical data to support superiority of one system over another.
Conclusion and Future Directions
At present there are no randomized trials evaluating the role of SRS in patients with multiple (>4) brain metastases. However, there are 3 registered phase III trials randomizing patients to WBRT alone or SRS alone specific to patients with more than 4 metastases. Although the North American Gamma Knife Consortium trial (NAGKC 12-01 [NCT01731704]) was intended to enroll patients with 5 or more brain metastases with a maximum total tumor volume ≤15 cc, this trial closed before completing accrual due to logistical issues, and it is uncertain whether the trial will reopen to accrual. The primary endpoint was cognitive function at 6 months, with cognitive function assessed with an online module. Another randomized phase III trial (NCT01592968) is currently accruing at the MD Anderson Cancer Center. The trial is randomizing patients with 4–15 brain metastases to SRS alone versus WBRT alone. The primary endpoints are local tumor control at 4 months and cognitive function at 4 months as measured by the Hopkins Verbal Learning Test–Revised. A third similar randomized trial (NCT02353000) is enrolling patients with 4–10 brain metastases in the Netherlands with a primary endpoint of QoL.
With the concern that WBRT alone does not yield sufficient local control, the Sunnybrook Odette Cancer Centre (University of Toronto) is in the process of finalizing its randomized phase III trial specific to patients with 5 to 20 brain metastases. The intent of this study is to treat all patients with SRS and randomize to no WBRT versus adjuvant WBRT. The primary outcome in this study is neurocognitive decline at 2 months as measured by the Hopkins Verbal Learning Test–Revised.
With the technical advances in radiation oncology advancing at a pace that surpasses the clinical evidence, the importance of high quality level 1 evidence to better define the role of SRS and WBRT in patients with multiple brain metastases cannot be underscored. If these trials are successful, our community will be able to educate the decision making for our patients, as patients with multiple brain metastases are increasing not only in number but also in complexity.
Disclosure. Dr Arjun Sahgal has received honoraria for past educational seminars from Medtronic, Elekta AB, Accuray Inc, and Varian Medical Systems and research grants from Elekta AB. Dr Sahgal also belongs to the Elekta MR Linac Research Consortium. Dr Wilko Verbakel has received an honorarium and travel expenses from Varian Medical Systems and institutional research grants from Varian Medical Systems. This work had no other funding sources.
References
Author notes
Corresponding Author: Arjun Sahgal, MD, Department of Radiation Oncology, Sunnybrook Health Sciences Centre, University of Toronto, 2075 Bayview Avenue, Toronto, Ontario, M4N 3M5, Canada ([email protected]).