-
PDF
- Split View
-
Views
-
Cite
Cite
David Schmit, Danilo Fliser, Thimoteus Speer, Proprotein convertase subtilisin/kexin type 9 in kidney disease, Nephrology Dialysis Transplantation, Volume 34, Issue 8, August 2019, Pages 1266–1271, https://doi.org/10.1093/ndt/gfz122
- Share Icon Share
Abstract
Chronic kidney disease (CKD) is associated with a substantially increased risk for the development of atherosclerotic cardiovascular (CV) disease. Accordingly, CV mortality is increased even in the earliest stages of CKD. In the general population and in CKD patients, high plasma levels of low-density lipoprotein cholesterol (LDL-C) are crucially involved in the initiation and progression of atherosclerotic vascular lesions. Lowering LDL-C by use of statins and/or ezetimibe represents the gold standard of lipid-lowering therapy, with a great body of evidence from several large clinical trials. Statin therapy reduces CV events in patients with normal and impaired kidney function alike, while the evidence for patients on maintenance haemodialysis is weaker. The inhibition of proprotein convertase subtilisin/kexin type 9 (PCSK9) serine protease represents a novel lipid-lowering tool. Currently the monoclonal antibodies evolocumab and alirocumab are the approved PCSK9 inhibitors. Despite maximum-tolerated statin therapy, they efficiently further reduce LDL-C plasma levels without any major adverse effects. Moreover, in large clinical outcome trials, both antibodies have been proven to lower CV events. Notably, the LDL-lowering capacity was independent of baseline kidney function and also efficient in patients with moderate CKD. However, patients with severely impaired kidney function, that is, the population at the highest CV risk, have been excluded from those trials. The relevance of the LDL-independent effects of PCSK9 inhibitors, such as lowering lipoprotein(a) or ameliorating dyslipidaemia in patients with nephrotic syndrome, has to be determined. Therefore further specific studies assessing the effects and outcomes of PCSK9-inhibiting treatment in CKD patients are warranted.
INTRODUCTION
Chronic kidney disease (CKD) is associated with a substantially increased risk for atherosclerotic cardiovascular diseases (CVDs) [1, 2]. Beside the presence of CKD-related cardiovascular (CV) risk factors (e.g. oxidative stress, inflammation, anaemia), the prevalence of traditional CV risk factors such as arterial hypertension, diabetes mellitus and dyslipidaemia in CKD patients is high [2, 3]. For several years the pathophysiological role of low-density lipoprotein (LDL) in the development of atherosclerotic CVD has been extensively studied in the general population as well as in CKD patients [4–7]. In 1971, it was documented in the Framingham study that higher serum cholesterol levels are associated with an increased risk for CV events [8]. In a plethora of subsequent experimental studies, a detailed role for LDL in the initiation and progression of atherosclerotic vascular lesions was established [5]. LDL activates endothelial cells in the inner layer of the vascular wall, inducing endothelial dysfunction (Figure 1). Such activated endothelial cells express cell adhesion molecules, such as vascular cell adhesion molecule 1, on their surface, which mediate adhesion of circulating leucocytes (e.g. monocytes) and their transmigration into the subendothelial layer. There these cells are again stimulated by LDL, which induces their differentiation into tissue macrophages. These macrophages acquire LDL and finally become foam cells. This represents a crucial initial step in the pathogenesis of atherosclerosis. Notably, it has been documented that LDL accumulating in the vascular wall is prone to be post-translationally modified, e.g. by oxidation or carbamylation, which is particularly relevant to patients with CKD [9–13]. Such modified LDL represents a strong promoter of atherosclerotic CVD.
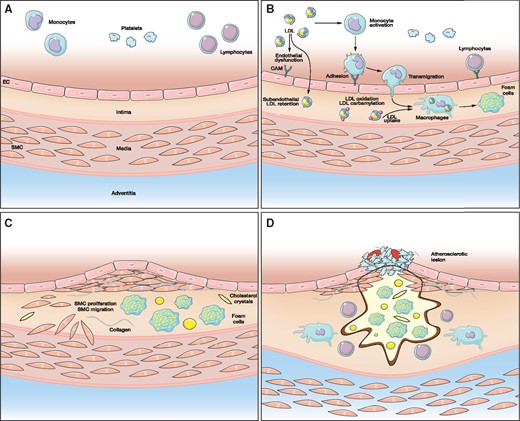
Role of LDL in the development of atherosclerotic lesions. (A) normal vascular wall. (B) endothelial dysfunction. (C) initiation of atherosclerotic lesion formation. (D) atherosclerotic plaque formation. EC, endothelial cells; SMC, smooth muscle cells; CAM, cell adhesion molecules.
A growing body of evidence suggests that lowering LDL cholesterol (LDL-C) reduces CV events in patients with prevalent CVD (i.e. secondary prevention) and patients at high risk for CVD (i.e. primary prevention). Until now, 3-hydroxy-3-methylglutaryl coenzyme A reductase inhibitors (statins) have been extensively studied and are currently the most widely used LDL-lowering agents. It has been shown that lowering LDL-C by 1 mmol/L (i.e. 38.7 mg/dL) reduces the relative risk of coronary events by 22% in an almost linear manner [14, 15]. This has been further supported by genetic association studies in which, genetically, lifelong lowered LDL caused by single nucleotide polymorphisms (SNPs) in LDL-regulating genes is associated with reduced CV risk [16, 17]. Since statins have been used in clinical practice for many years, analyses on their long-term use are now available. In the West of Scotland Coronary Prevention Study, 20 years after randomization to either pravastatin or placebo, the CV event rate in participants of the pravastatin treatment arm was still significantly reduced as compared with the placebo group, without any serious safety concerns [18]. Patients with CKD are considered at high or very high CV risk and therefore recent guidelines recommend lipid-lowering therapy in all CKD patients not on dialysis with an estimated glomerular filtration rate (eGFR) <60 mL/min/1.73 m2 [19]. In dialysis patients, the evidence for lipid-lowering therapy is weaker [20, 21]. Therefore the Kidney Disease: Improving global Outcomes (KDIGO) guidelines suggest continuing statin treatment initiated before renal replacement therapy and in dialysis patients with manifest CVD, but not newly initiating lipid-lowering therapy as a primary prevention approach [22]. While the KDIGO guidelines do not recommend a specific LDL-C target to be achieved [22], the guidelines of the European Society of Cardiology recommend an LDL-C target of <70 mg/dL (<1.82 mmol/L) for CKD patients with an eGFR <30 mL/min/1.73 m2 and <100 mg/dL (<2.6 mmol/L) for those with an eGFR of 30–59 mL/min/1.73 m2.
Reduced kidney function may represent a risk factor for statin-related adverse outcomes such as myopathy. It has been shown that the relative risk of myopathy in patients treated with high-dose simvastatin (i.e. 80 mg daily) was 2.5 [95% confidence interval (CI) 1.6–3.9] times higher in subjects with an eGFR <60 mL/min/1.73m2 as compared with those with an eGFR ≥60 mL/min/1.73 m2 [23]. Moreover, treatment with higher doses of statins such as atorvastatin has not yet been evaluated to be safe in patients with advanced CKD. In 28 randomized intervention trials with statin therapy comprising 183 419 subjects, <3% of the participants had an eGFR <30 mL/min/1.73 m2 [20]. In patients with lower eGFR, the reduction of major CV events was smaller under statin treatment and, in addition, in patients on dialysis, lower reductions of LDL-C have been achieved [20]. Therefore a combination of statins with other lipid-lowering agents might represent a reasonable approach in CKD patients to adequately lower LDL-C. For this purpose, novel LDL-lowering agents such as inhibitors of the proprotein convertase subtilisin/kexin type 9 (PCSK9) serine protease could be of particular interest for the treatment of hypercholesterolaemia in CKD patients.
DEVELOPMENT OF PCSK9 INHIBITORS
In 2003 it was shown that mutations in the PCSK9 locus may cause familial hypercholesterolaemia [24]. Subsequently it was documented that LDL-C plasma levels in black subjects carrying a PCSK9 loss-of-function variant were significantly lower as compared with subjects without this mutation, resulting in a lower prevalence of coronary artery disease (CAD) in this population [25, 26]. In parallel, experimental evidence suggests that PCKS9 plays an important role in LDL metabolism [27, 28]. PCSK9 protein reduces the expression of LDL receptor on the surface of liver cells and thereby decreases cellular uptake of LDL and thus its clearance from the circulation (Figure 2). Based on these findings, inhibition of PCSK9 was suggested as a promising treatment approach to lower LDL-C. This led to the development of humanized PCSK9-inhibiting monoclonal antibodies by several pharmaceutical companies, which have now been evaluated in large study programmes [29, 30]. Currently evolocumab and alirocumab are available for therapeutic use. Another PCSK9 inhibitor, bococizumab, did not reach the market. Treatment with bococizumab was associated with a substantial risk for the development of neutralizing antibodies, resulting in a lower LDL-C-reducing efficacy and a higher rate of adverse reactions at the injection site [31].
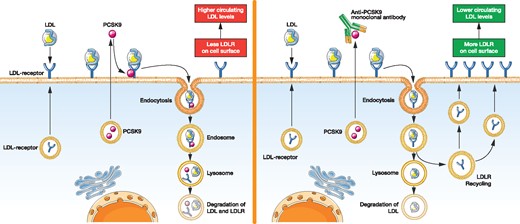
Hepatic LDL metabolism in the absence (left) and presence (right) of PCSK9-inhibiting antibodies.
Besides PCSK9-inhibiting antibodies, inclisiran, a hepa-tocyte-specific small interfering RNA directed against PCSK9, is currently under clinical investigation. Interestingly, in a Phase 2 study, inclisiran exerts a long-lasting LDL-C-lowering response persisting for at least 180 days [32]. Phase 3 studies are currently ongoing.
CLINICAL TRIALS ON THE EFFICACY OF PCSK9 INHIBITION
The outcome trials on evolocumab and alirocumab are summarized in Table 1.
Baseline characteristics and outcomes of the FOURIER and ODYSSEY Outcomes trials
Parameter . | FOURIER . | ODYSSEY Outcomes . | ||
---|---|---|---|---|
Antibody . | Evolocumab . | Alirocumab . | ||
Study group . | Placebo (n = 13 780) . | Evolocumab (n = 13 784) . | Placebo (n = 9462) . | Alirocumab (n = 9462) . |
eGFR exclusion criterion | <20 mL/min/1.73 m2 | <30 mL/min/1.73 m2 | ||
Median length of follow-up (years) | 2.2 | 2.8 | ||
MI (%) | 81.3 | 80.9 | 82.8 | 83.4 |
Median time from previous MI (months) | 39.6 | 40.8 | 2.8 | 2.8 |
High-intensity statin therapy (%) | 69.1 | 69.5 | 89.1 | 88.6 |
Moderate-intensity statin therapy (%) | 30.7 | 30.2 | 8.2 | 8.8 |
Ezetemibe therapy (%) | 5.2 | 5.3 | 3.0 | 2.8 |
Baseline LDL-C (mg/dL) | 92 | 92 | 92 | 92 |
Baseline LDL-C (mmol/L) | 2.39 | 2.39 | 2.39 | 2.39 |
Change of LDL-C (%) | −59 | −54.7 | ||
Primary endpoint (RRR, %) | 15 | 15 | ||
Key secondary endpoint (RRR, %) | 20 | 14 |
Parameter . | FOURIER . | ODYSSEY Outcomes . | ||
---|---|---|---|---|
Antibody . | Evolocumab . | Alirocumab . | ||
Study group . | Placebo (n = 13 780) . | Evolocumab (n = 13 784) . | Placebo (n = 9462) . | Alirocumab (n = 9462) . |
eGFR exclusion criterion | <20 mL/min/1.73 m2 | <30 mL/min/1.73 m2 | ||
Median length of follow-up (years) | 2.2 | 2.8 | ||
MI (%) | 81.3 | 80.9 | 82.8 | 83.4 |
Median time from previous MI (months) | 39.6 | 40.8 | 2.8 | 2.8 |
High-intensity statin therapy (%) | 69.1 | 69.5 | 89.1 | 88.6 |
Moderate-intensity statin therapy (%) | 30.7 | 30.2 | 8.2 | 8.8 |
Ezetemibe therapy (%) | 5.2 | 5.3 | 3.0 | 2.8 |
Baseline LDL-C (mg/dL) | 92 | 92 | 92 | 92 |
Baseline LDL-C (mmol/L) | 2.39 | 2.39 | 2.39 | 2.39 |
Change of LDL-C (%) | −59 | −54.7 | ||
Primary endpoint (RRR, %) | 15 | 15 | ||
Key secondary endpoint (RRR, %) | 20 | 14 |
RRR, relative risk reduction.
Baseline characteristics and outcomes of the FOURIER and ODYSSEY Outcomes trials
Parameter . | FOURIER . | ODYSSEY Outcomes . | ||
---|---|---|---|---|
Antibody . | Evolocumab . | Alirocumab . | ||
Study group . | Placebo (n = 13 780) . | Evolocumab (n = 13 784) . | Placebo (n = 9462) . | Alirocumab (n = 9462) . |
eGFR exclusion criterion | <20 mL/min/1.73 m2 | <30 mL/min/1.73 m2 | ||
Median length of follow-up (years) | 2.2 | 2.8 | ||
MI (%) | 81.3 | 80.9 | 82.8 | 83.4 |
Median time from previous MI (months) | 39.6 | 40.8 | 2.8 | 2.8 |
High-intensity statin therapy (%) | 69.1 | 69.5 | 89.1 | 88.6 |
Moderate-intensity statin therapy (%) | 30.7 | 30.2 | 8.2 | 8.8 |
Ezetemibe therapy (%) | 5.2 | 5.3 | 3.0 | 2.8 |
Baseline LDL-C (mg/dL) | 92 | 92 | 92 | 92 |
Baseline LDL-C (mmol/L) | 2.39 | 2.39 | 2.39 | 2.39 |
Change of LDL-C (%) | −59 | −54.7 | ||
Primary endpoint (RRR, %) | 15 | 15 | ||
Key secondary endpoint (RRR, %) | 20 | 14 |
Parameter . | FOURIER . | ODYSSEY Outcomes . | ||
---|---|---|---|---|
Antibody . | Evolocumab . | Alirocumab . | ||
Study group . | Placebo (n = 13 780) . | Evolocumab (n = 13 784) . | Placebo (n = 9462) . | Alirocumab (n = 9462) . |
eGFR exclusion criterion | <20 mL/min/1.73 m2 | <30 mL/min/1.73 m2 | ||
Median length of follow-up (years) | 2.2 | 2.8 | ||
MI (%) | 81.3 | 80.9 | 82.8 | 83.4 |
Median time from previous MI (months) | 39.6 | 40.8 | 2.8 | 2.8 |
High-intensity statin therapy (%) | 69.1 | 69.5 | 89.1 | 88.6 |
Moderate-intensity statin therapy (%) | 30.7 | 30.2 | 8.2 | 8.8 |
Ezetemibe therapy (%) | 5.2 | 5.3 | 3.0 | 2.8 |
Baseline LDL-C (mg/dL) | 92 | 92 | 92 | 92 |
Baseline LDL-C (mmol/L) | 2.39 | 2.39 | 2.39 | 2.39 |
Change of LDL-C (%) | −59 | −54.7 | ||
Primary endpoint (RRR, %) | 15 | 15 | ||
Key secondary endpoint (RRR, %) | 20 | 14 |
RRR, relative risk reduction.
Further Cardiovascular Outcome Research with PCSK9 Inhibition in Subjects with Elevated Risk (FOURIER) is a randomized, double-blind, placebo-controlled clinical trial including 27 564 patients between 40 and 85 years of age with high CV risk based on prior myocardial infarction (MI), stroke or symptomatic lower extremity peripheral arterial disease [30]. These patients failed to achieve the LDL-C treatment target of <70 mg/dL (1.82 mmol/L) despite maximal tolerated statin treatment with or without ezetimibe. After a lipid stabilization phase, patients were randomized to either evolocumab (140 mg subcutaneously every 2 weeks or 420 mg every 4 weeks) or placebo. After 48 weeks, LDL-C plasma decreased by 59%, that is, from 92 mg/dL (2.39 mmol/L) at baseline to 30 mg/dL (0.78 mmol/L) in the evolocumab treatment group. The primary endpoint, which was the composite of CV death, MI, stroke, hospitalization for unstable angina or coronary revascularization, was reduced by 15% in the treatment group [hazard ratio (HR) 0.85 (95% CI 0.79–0.92); P < 0.001]. Moreover, the key secondary endpoint, the composite of CV death, MI or stroke, was reduced by 20% [HR 0.80 (95% CI 0.73–0.88); P < 0.001]. Notably, the efficacy of evolocumab was independent of age, sex, race, primary affected vascular bed or baseline LDL-C. Even in participants with baseline LDL-C levels in the lowest quartile [i.e. <80 mg/dL (2.08 mmol/L)], a risk reduction of 20% was observed. Subgroup analyses revealed that treatment with evolocumab was particularly beneficial in patients with more recent MI, with at least one MI and in those with residual multivessel disease [33]. Finally, there were no serious safety concerns with evolocumab, such as diabetes mellitus, reactions at the injection site, rhabdomyolysis or cognitive dysfunction [34].
Evaluation of Cardiovascular Outcomes After an ACS During Treatment with Alirocumab (ODYSSEY Outcomes) is a randomized, double-blind, placebo-controlled trial that enrolled 18 924 patients with MI during the previous 12 months who failed to achieve the LDL-C target of <70 mg/dL (1.82 mmol/L) despite high-intensity statin treatment [29]. Patients on high-intensity or maximum tolerated doses of atorvastatin or rosuvastatin were randomized to an additional treatment with alirocumab or placebo. The LDL-C-lowering efficacy of alirocumab was comparable to that of evolocumab. After 4 years, LDL-C was lowered by 54.7%, with an absolute difference of 48.1 mg/dL (1.25 mmol/L). The primary endpoint was time to CAD death or non-fatal MI, fatal or non-fatal ischaemic stroke or unstable angina requiring hospitalization. The relative risk reduction of the primary efficacy endpoint in ODYSSEY Outcomes was 15%, with an absolute risk reduction of 1.6. These results were virtually identical to those of the FOURIER trial. The decrease in the primary endpoint was mainly due to reductions of non-fatal MI, ischaemic stroke and unstable angina. Importantly, all-cause mortality was significantly lower (15% relative risk reduction) in alirocumab-treated patients as compared with the placebo group. Similar to evolocumab, there were no differences in the LDL-C-lowering efficacy by alirocumab across different subgroups (e.g. age, sex and geographic region). The greatest benefit was observed in patients with LDL-C >100 mg/dL (2.6 mmol/L). In addition, there were no serious safety concerns.
These findings indicate that treatment with PCSK9-inhibiting antibodies represents an additional treatment approach to lower LDL-C. Both evolocumab and alirocumab have been approved by the European Medicines Agency and the US Food and Drug Administration. PCSK9 inhibition should be considered as a treatment approach in patients at very high CV risk, in patients with familiar hypercholesterolaemia on maximally tolerated doses of statins and/or ezetemibe or lipid apheresis with persistently high LDL-C plasma levels [19]. While neither the FOURIER nor the ODYSSEY Outcomes trial reported serious adverse effects in patients treated with evolocumab and alirocumab, future studies are necessary to determine the long-term safety of these compounds. In the FOURIER trial, 0.3% of the participants developed new binding antibodies directed against evolocumab [30] and, in the ODYSSEY Outcomes trial, neutralizing antibodies against alirocumab could have been detected in 0.5% of patients treated with this PCSK9 inhibitor [29]. It remains unclear whether long-term or even lifelong treatment would further increase the development of anti-drug directed antibodies, potentially reducing treatment efficacy. Interestingly, in a recent study, potential long-term adverse effects of PCSK9 inhibition have been explored using a genetic approach. The authors found an LDL-C-lowering variant in the PCSK9 gene locus—as a model for lifelong PCSK9 inhibition—is associated with an increased risk for type 2 diabetes and higher body mass index, waist:hip ratio and diastolic blood pressure [35]. It has to be determined if this is also the case for long-term PCSK9-inhibiting therapies.
PCSK9 INHIBITION IN PATIENTS WITH CKD
Importantly, the FOURIER and ODYSSEY Outcomes trials excluded patients with severely impaired kidney function (i.e. eGFR <20 mL/min/1.73 m2 and <30 mL/min/1.73 m2) [29, 30]. Therefore evidence for the use of PCSK9 inhibitors in patients with advanced CKD is limited. A recent post hoc analysis of eight Phase 3 ODYSSEY trials divided 4629 participants into those with or without impaired kidney function (i.e. eGFR <60 mL/min/1.73 m2), representing 10.1% of the entire study population [36]. Notably, the LDL-C-lowering efficacy of alirocumab was comparable in subjects with impaired kidney function and those without. In addition, alirocumab reduced lipoprotein(a) [Lp(a)], apolipoprotein B and triglycerides independently of the baseline kidney function. Importantly, the rate of adverse events did not differ between patients with eGFR <60 mL/min/1.73 m2 and those with eGFR ≥60 mL/min/1.73 m2 [36]. Similar results have been presented from the FOURIER trial [37]. The FOURIER trial included 4443 subjects with CKD Stage ≥3 (i.e. eGFR <60 mL/min/1.73 m2). The relative risk reduction with evolocumab was independent from the CKD stage. Moreover, there was a trend towards a larger reduction for the key secondary endpoint (i.e. CV death, MI or stroke) in patients with more advanced CKD, whereas the rate of adverse events was independent of kidney function. These findings suggest that treatment with PCSK9 inhibitors might be effective and safe in patients with mild to moderate CKD. Further subgroup analyses of the FOURIER and the ODYSSEY Outcomes trials will reveal whether PCSK9 inhibition is also associated with improved outcomes in patients with impaired kidney function. However, the effects of PCSK9 inhibition on LDL-C levels, safety and outcomes in patients with severely impaired kidney function remain enigmatic and should be the subject of future studies. Moreover, treatment with PCSK9 inhibitors is associated with considerable annual therapy costs. Several cost-effectiveness studies have reported that PCSK9 inhibition using the currently available antibodies could be cost effective in patients with familial hypercholesterolaemia, whereas cost-effectiveness was substantially lower in patients with prevalent atherosclerotic vascular diseases [38–40]. Both the FOURIER and ODYSSEY Outcomes trials reported similar numbers needed to treat (NNT) to prevent new CVD events (FOURIER, NNT 67; ODYSSEY Outcomes, NNT 63) [29, 30]. In particular, in patients with advanced CKD, the costs for the therapy have to be balanced against weak evidence for a benefit. A reduction of (annual) drug prices would be necessary to meet an acceptable threshold between incremental costs per quality-adjusted life year.
LDL-INDEPENDENT EFFECTS OF PCSK9 INHIBITION IN CKD PATIENTS
Besides lowering LDL-C, PCSK9 inhibitors have been shown to reduce Lp(a). In a recent meta-analysis, PCSK9 inhibition was associated with a reduction of Lp(a) plasma levels by 21.9% (95% CI 24.3–19.5) [41]. In the general population, Lp(a) represents a well-known risk factor for the development of CAD; however, in patients with prevalent CAD, actual Lp(a) levels and SNPs within the LPA gene locus associated with lifelong elevated Lp(a) were not associated with higher all-cause and CV mortality [42]. In CKD patients, Lp(a) plasma levels are increased and associated with CV events [43]. In a subgroup analysis of the ODYSSEY Outcomes trial, it has been shown that lowering of Lp(a) by alirocumab contributes to a reduction of adverse CV events independent of its LDL-C-lowering effects [44]. The relevance of Lp(a) lowering by PCSK9 inhibitors in patients with CKD has yet to be studied.
While the association between PCSK9 plasma levels and eGFR in general remains controversial [45], PCSK9 might be involved in the development of proteinuria-associated dyslipidaemia [46]. Plasma levels of PCSK9 were increased in experimental models of nephrotic syndrome by higher hepatic secretion and reduced clearance [47, 48]. In mice lacking PCSK9 treated with nephrotoxic serum, dyslipidaemia was ameliorated [48]. Therefore PCSK9 inhibitors could provide a novel treatment strategy for dyslipidaemia in patients with nephrotic syndrome.
CONCLUSION
High CV morbidity and mortality remain a persistent problem in patients with CKD. Many efforts have been made in subjects with normal kidney function to prevent and reduce the progression of CVD. However, in most of these studies, patients with advanced CKD have been excluded. Moreover, results from the general population can only be partially transferred to patients with CKD. PCSK9 inhibition represents a novel and successful treatment approach in patients with normal to moderately impaired kidney function to reduce LDL-C. Specific studies in CKD patients are needed to prove the efficacy and safety of PCSK9 inhibitors and to determine their ability to improve outcomes in these patients.
FUNDING
This work is funded by the Deutsche Forschungsgemeinschaft (DFG), SFB TRR 219 (DF and TS).
CONFLICT OF INTEREST STATEMENT
None declared. The results presented in this article have not been published previously in whole or part. T.S. has received speaker fees from Sanofi Aventis.
REFERENCES
Cholesterol Treatment Trialists Collaboration,
Cholesterol Treatment Trialists Collaboration,
Comments