-
PDF
- Split View
-
Views
-
Cite
Cite
Emilie Bres, Laetitia Koppe, Is there still a place for prebiotics in chronic kidney disease?, Nephrology Dialysis Transplantation, Volume 34, Issue 11, November 2019, Pages 1812–1816, https://doi.org/10.1093/ndt/gfz124
- Share Icon Share
As glomerular filtration rate declines, the capacity of the kidneys to eliminate metabolic waste is gradually lost, resulting in the retention of various compounds. Unfortunately, conventional dialysis provides limited clearance of organic solutes. Although recent progress in dialysis treatments like haemodiafiltration and adsorptive strategies has increased the removal of uraemic toxins, it cannot be applied to a large population of pre-dialysis patients. Almost 50 years ago, Mitch et al. [1] observed that intestinal bacteria adversely affect uraemic patients by promoting catabolism and producing toxins that accumulate in the body fluids. But evidence about colonic microbial metabolism as a key contributor to uraemic toxin production has only been gathered over the past decade, with the help of new techniques in metabolomics. This has allowed the comparison of plasma metabolite profiles of haemodialysis patients, with and without colons, and in germ-free mice [2, 3]. These studies were able to identify >150 compounds, including p-cresyl sulphate (PCS), indoxyl sulphate (IS) and indole 3-acetic acid (IAA), that could only be observed in the presence of an intact colon and its microbiota. PCS is a putrefaction metabolite of tyrosine, while IAA and IS are generated by the fermentation of tryptophan. The toxicity of these solutes has been demonstrated in numerous experimental studies. In particular, it has been found that IS and/or PCS induce insulin resistance [4], activate the renin–angiotensin–aldosterone system and renal fibrosis and cause endothelial dysfunction and atherogenesis [5]. Several clinical studies have significantly demonstrated independent associations between these metabolites and negative outcomes [6, 7], although not all confirmed this correlation [8]. For protein-bound toxins, only the free fraction is biologically active. Free PCS and IS levels may be a better indicator for potential toxicity of uraemic solutes and can explain contradictory results.
The interactions between gut microbiota and renal dysfunction may be bidirectional, as shown in Figure 1. Besides passive accumulation of uraemic toxins, there is now accruing evidence that chronic kidney disease (CKD) causes dysbiosis of the intestinal microbiome by increasing the presence of pathogenic flora over symbiotic bacteria, potentially contributing to the overproduction of uraemic toxins. Recently, microbial deoxyribonucleic acid has been isolated from the stools of haemodialysis patients and analysed by phylogenetic microarray [9]. The α-diversity indices are commonly used to describe the ecological diversity. The simplest measure is richness, the number of species [or operational taxonomic units (OTUs)] observed in the sample. Other metrics consider the abundances (frequencies) of the OTUs. These studies showed that patients with CKD were associated with a decrease in microbial α-diversity and with marked differences in the abundance of bacterial OTU. In particular, it has been highlighted that the CKD group had a significantly higher abundance of Proteobacteria and a lower abundance of Lactobacillaceae. To isolate the effect of uraemia from inter-individual variability, comorbid conditions and dietary and antibiotic interventions, experimental studies in uraemic rodent models were carried out and found a similar modification of the composition of the gut microbiome [9, 10]. Additional studies also demonstrated that CKD is associated with an increased number of bacteria possessing urease, uricase, p-cresol and indole-forming enzymes [11]. Consequently, targeted interventions have been developed to restore symbiosis and reduce the production of uraemic toxins. In particular, as nutrient intake is one of the most important factors driving colonic microbial behaviour, dietary interventions are a logical co-strategy to re-establish microbial balance and reduce circulating uraemic toxin levels. Among them, prebiotics appear to be an attractive strategy. Prebiotics are a non-digestible food ingredient that stimulate the growth and/or activity of one or a limited number of bacteria, such as Bifidobacteria and Lactobacilli, and increase the resistance towards pathogens.
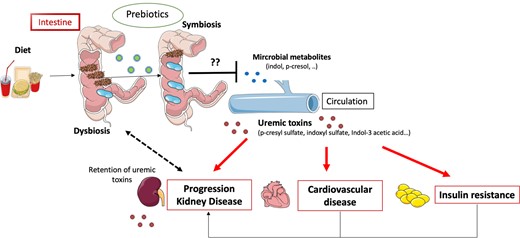
Hypothesis of the vicious diet–gut–kidney axis. Dysbiosis induced by an imbalanced diet and CKD leads to overproduction and accumulation of precursor of uraemic toxins in the intestine. Consequently, influx of uraemic toxins into the blood circulation contributes to progression of renal failure, insulin resistance and cardiovascular disease. Prebiotics could stimulate the growth of different indigenous gut bacteria and help restore a healthy gut flora and prevent deleterious uraemic toxin production.
In this issue of Nephrology Dialysis Transplantation, Ramos et al. [12] report findings on a prebiotic [fructooligosaccharide (FOS)] to reduce microbial-derived uraemic toxins in Stages 3 and 4 CKD patients. In this double-blind, placebo-controlled, randomized trial, they had not observed any difference in IS and IAA concentrations between the group treated with oral powder containing FOS (12 g/day) or the placebo (maltodextrin, 12 g/day) after 3 months. This leads us to wonder about the future role of the prebiotic strategy in improving the life quality and sustainability of CKD patients.
Preliminary experimental strategies using prebiotics were very encouraging. All of them demonstrated a decrease of uraemic toxin production [4, 13, 14] after a short administration of prebiotics, such as amylose maize–resistant, galacto‐oligosaccharides (GOS) or arabinoxylan oligosaccharides (AXOS). But human trials have not been equally convincing. Table 1 summarizes clinical studies examining the impact of prebiotics on gut-derived uraemic toxins. The protocols were all relatively short in duration (<3 months) and the results were divergent. However, the therapeutic interventions were all different, using diverse compounds and doses of prebiotic, designs and patient populations (CKD or dialysis patients). In haemodialysis patients, the baseline toxin levels were higher and a difference after prebiotic treatment should be easier to find. Therefore, administration of high-amylose corn starch or oligofructose-enriched inulin has decreased serum PCS (−20–28%) and IS (−29%) concentrations in this population [19, 20].
Human studies reporting the use of prebiotics alone in CKD on gut-derived uraemic toxins
References . | Prebiotics . | Study . | Diet . | Results . |
---|---|---|---|---|
Esgalhado et al. [15] |
|
| 24-h food recall conducted over 3 days, including 2 days of the week (1 day of dialysis and 1 day without dialysis) and 1 day over the weekend |
|
Ramos et al. [11] |
|
| A 3-day food record was used to assess protein and fibre intake at baseline and final visit |
|
Elamin et al. [16] |
|
| No diet management | No effect on IS |
Poesen et al. [17] |
|
| Food frequency questionnaires | No effect on PCS, IS |
Salmean et al. [18] | 10 g/day pea hull fibre and 15 g/day inulin |
| At baseline, 5-day stool frequency questionnaires and 3-day food records during each intervention period | ↓ p-cresol |
Sirich et al. [19] |
|
| No diet management | ↓ PCS and IS |
Meijers et al. [14] |
|
| No diet management |
|
References . | Prebiotics . | Study . | Diet . | Results . |
---|---|---|---|---|
Esgalhado et al. [15] |
|
| 24-h food recall conducted over 3 days, including 2 days of the week (1 day of dialysis and 1 day without dialysis) and 1 day over the weekend |
|
Ramos et al. [11] |
|
| A 3-day food record was used to assess protein and fibre intake at baseline and final visit |
|
Elamin et al. [16] |
|
| No diet management | No effect on IS |
Poesen et al. [17] |
|
| Food frequency questionnaires | No effect on PCS, IS |
Salmean et al. [18] | 10 g/day pea hull fibre and 15 g/day inulin |
| At baseline, 5-day stool frequency questionnaires and 3-day food records during each intervention period | ↓ p-cresol |
Sirich et al. [19] |
|
| No diet management | ↓ PCS and IS |
Meijers et al. [14] |
|
| No diet management |
|
HD, haemodialysis; eGFR, estimated glomerular filtration rate.
Human studies reporting the use of prebiotics alone in CKD on gut-derived uraemic toxins
References . | Prebiotics . | Study . | Diet . | Results . |
---|---|---|---|---|
Esgalhado et al. [15] |
|
| 24-h food recall conducted over 3 days, including 2 days of the week (1 day of dialysis and 1 day without dialysis) and 1 day over the weekend |
|
Ramos et al. [11] |
|
| A 3-day food record was used to assess protein and fibre intake at baseline and final visit |
|
Elamin et al. [16] |
|
| No diet management | No effect on IS |
Poesen et al. [17] |
|
| Food frequency questionnaires | No effect on PCS, IS |
Salmean et al. [18] | 10 g/day pea hull fibre and 15 g/day inulin |
| At baseline, 5-day stool frequency questionnaires and 3-day food records during each intervention period | ↓ p-cresol |
Sirich et al. [19] |
|
| No diet management | ↓ PCS and IS |
Meijers et al. [14] |
|
| No diet management |
|
References . | Prebiotics . | Study . | Diet . | Results . |
---|---|---|---|---|
Esgalhado et al. [15] |
|
| 24-h food recall conducted over 3 days, including 2 days of the week (1 day of dialysis and 1 day without dialysis) and 1 day over the weekend |
|
Ramos et al. [11] |
|
| A 3-day food record was used to assess protein and fibre intake at baseline and final visit |
|
Elamin et al. [16] |
|
| No diet management | No effect on IS |
Poesen et al. [17] |
|
| Food frequency questionnaires | No effect on PCS, IS |
Salmean et al. [18] | 10 g/day pea hull fibre and 15 g/day inulin |
| At baseline, 5-day stool frequency questionnaires and 3-day food records during each intervention period | ↓ p-cresol |
Sirich et al. [19] |
|
| No diet management | ↓ PCS and IS |
Meijers et al. [14] |
|
| No diet management |
|
HD, haemodialysis; eGFR, estimated glomerular filtration rate.
CKD is associated with metabolic complications like insulin resistance and dyslipidaemia [21]. In addition, the absence of a beneficial effect of FOS on insulin resistance, dyslipidaemia, serum markers of intestinal permeability and gut trophic factor is disappointing. Indeed, recent data reported that a daily supplementation of 10 g of inulin, resistant starches or FOS-enriched inulin could have beneficial effects on metabolic syndrome components in type 2 diabetes [22]. Experimental and clinical data have demonstrated that prebiotics may further improve the secretion of glucose-like peptide 1 and increase the production of short-chain fatty acids, which are both involved in glucose homoeostasis. Concordantly, in CKD mice models, a diet supplemented with AXOS was associated with improvement of insulin sensitivity and a reduction of PCS production [4]. However, as in this study [12], Poesen et al. [17] did not observe any difference on insulin resistance and on PCS concentration in non-dialysis patients after AXOS prebiotic treatment. In both these studies [12, 17], it is unclear whether the absence of effect after intake of a prebiotic is due to the lack of effect on serum PCS or whether other types of prebiotics may affect insulin resistance in CKD.
Diet composition, such as fibres or protein intake, was not monitored in most of the studies on prebiotic strategy, and this aspect may have influenced the results. In healthy rats, for example, the composition of microbiota has been significantly changed after a GOS-enriched inulin diet, including an increase of Bacteroides and a decrease of Alloprevotella, with a decrease in diversity. But such changes were different in the fibre-fed group (from bran), with a decrease in Bacteroides and an increase in Alloprevotella and Parabacteroides species. Again, there was a decrease in diversity. But in the group with ‘GOS plus fibre’ diet, it is intriguing that intestinal microbiota had the same structure as the control diet group without modification of its composition, and flora diversity was upregulated [23]. In humans, Wu et al. [24] observed that the pattern of diet modulates the composition of the gut microbiota, resulting in a predominance of Bacteroides when the diet is characterized by animal protein and fat intake and Prevotella when it is rich in carbohydrates. In CKD cohort studies, circulating levels of IS and PCS are negatively correlated with high dietary fibre and low protein intake [25, 26]. However, rigorous studies investigating the link between the modification of intestinal microbiota, uraemic toxin levels and diet have not yet been performed. Only recently, a small observational study suggested a modification of the intestinal microbiota composition in pre-dialysis patients adhering to a low-protein diet. However, analysis of gut microbiota was performed using the denaturing gradient gel electrophoresis technique, which is a global rather than a functional analysis [27]. The strength of the study by Ramos et al. [12] is the use of a 3-day food record to assess protein and fibre intake at baseline and at the final visit in order to limit the bias. The composition and quantity of dietary intake were comparable between groups at the beginning and end of the study, limiting diet-induced bias. In another study by Poesen et al. [17], testing the prebiotic AXOS in CKD, the diet was not different between groups and no difference in uraemic toxin concentration was found. The positive effects of the other studies [19, 20] could be due to a different diet between groups. In keeping with this theory, Salmean et al. [18] observed a lower p-cresol concentration after prebiotic treatment in the intervention group, but the fibre intake in this group was almost twice as much as in the control.
Another crucial point is the choice of prebiotic. Prebiotic fibres are a heterogeneous group with variable properties determined by their physical and chemical fibre composition. The actions of a particular prebiotic are determined by such properties, including susceptibility to bacterial fermentation, water-holding capacity, cation exchange and adsorptive functions. For example, chemically, inulin and FOS are both polydisperse fructans. Inulin ends with a terminal glucose monomer, whereas FOS is composed of partially hydrolysed inulin. Most FOS molecules end with a fructose rather than glucose monomer. FOS also differs from inulin in its degree of polymerization (degree of polymerization 2 (DP2)-8 for FOS and DP2-60 for inulin). FOS is fermented rapidly by microorganisms, whereas inulin is not digestible by digestive enzymes in the small intestine. As a result, it reaches the colon intact. The main site of production of PCS and IS is the colon; this suggests that positive results were mostly observed after inulin treatment [18, 19]. All of this may explain the different physiological responses obtained after prebiotic treatment. Until now there has been no study comparing the effect of different types of prebiotics on uraemic toxin levels in the context of CKD.
Another explanation of the failure of prebiotics studies is the lack of knowledge surrounding the precise composition of microbiota in the uraemic gut and its implication on uraemic toxin production and renal progression. All the studies with prebiotics in CKD are based on correlations and do not establish a causal link between modulation of the gut microbiota and the concentration of uraemic toxins. Furthermore, in this study [12], no analysis of microbiota has been performed and the negative results can be explained by the absence of modification of the intestinal microbiota. Until now, contrary to what has been achieved in other pathologies, such as obesity [28] and cirrhosis [29], no large studies using the metagenomic approach have been performed in CKD. This would not only allow a study of the taxonomic profile but also of the functionalities of gut bacteria in this population.
To potentiate the microbiologic effect of prebiotics, the symbiotic strategy (prebiotics plus probiotics, which are microorganisms that confer a health benefit) seems the most promising. Two randomized controlled trials with a total of 67 non-dialysis patients were successful in decreasing the PCS concentration [30, 31]. But this raises the same questions concerning the selection of probiotics: are these bacteria involved in the improvement of uraemic syndrome? Will they survive in the uraemic environment?
In particular, the potential adverse effects of ‘biotic’ strategy need to be underlined. This study [12], like all prebiotic studies, confirms that the overall adherence was excellent and gastrointestinal tolerance was good. This must be compared with the large number of non-adherences in the low-protein diet. For example, in a large meta-analysis, Pan et al. [32] found that the mean protein intake in the low-protein diet groups exceeded the prescribed protein intake by 20%. We also do not know if increasing the number of bacterial species that possess urease can have a negative impact. Urease activity may increase the generation of ammonium by the degradation of urea in the gut lumen; this may damage the epithelial tight junction, allowing endotoxins to enter the bloodstream [33].
In conclusion, this study does not contain the expected, convincing evidence to recommend prebiotics in routine clinical use nor to propose guidelines for dietary fibre intake in a CKD population. This is particularly the case in the absence of randomized clinical trials investigating the impact of prebiotics on hard clinical endpoints (e.g. cardiovascular events, mortality) in CKD. It is perhaps premature to eliminate prebiotics as a treatment to slow down CKD progression and complications. After all, we have few clinical studies available and more research is needed to examine the effectiveness of prebiotics. Accumulating evidence from both human and rodent studies highlights the central role of the gut microbiota in the gut–kidney axis and negative studies must not stop research on this topic. Research focused on mechanisms of action, characterizing responders or non-responders and understanding how structure relates to the function of prebiotics is particularly needed. The use of prebiotics to improve CKD patients cannot be viewed in isolation and will be part of a wider approach for healthy nutrition and lifestyle. In addition, a better characterization of intestinal microbiota in the future will help to select prebiotics of interest. Although the use of prebiotics and probiotics is one of the most widely used strategies to modulate the gut microbiota, other strategies, including other dietary components, such as a vegetarian diet, low-protein diet, ketoacid supplementation, modulation of the quality of protein and faecal microbial transplantation, should also be considered.
ACKNOWLEDGEMENTS
This study was supported by Hospices Civils de Lyon and University of Lyon. We thank Bryan Dunsmore for proofreading the manuscript.
CONFLICT OF INTEREST STATEMENT
None declared.
(See related article by Ramos et al. Effect of prebiotic (fructooligosaccharide) on uremic toxins of chronic kidney disease patients: a randomized controlled trial. Nephrol Dial Transplant 2019; 34: xxx--xxx)
Comments