-
PDF
- Split View
-
Views
-
Cite
Cite
Sandro Mazzaferro, Marzia Pasquali, Vitamin D: a dynamic molecule. How relevant might the dynamism for a vitamin be?, Nephrology Dialysis Transplantation, Volume 31, Issue 1, January 2016, Pages 23–30, https://doi.org/10.1093/ndt/gfv340
- Share Icon Share
Abstract
Cholecalciferol, the precursor of Vitamin D3, is a very old, highly conserved, molecule. Its presence is evident in non-mineralized 750 million-year-old living species, such as plankton. The more active metabolites, a receptor and a D binding protein, appear later, along with the increasing complexity of animal species living in the sea. In the sea, however, the biological function of vitamin D is unlikely to be linked with mineral metabolism, and we can hypothesize a relationship with an immune response. It is in terrestrial animals exhibiting cellular bone that the complexity of vitamin D increases. At this stage of evolution, we see the appearance of bone cells that are capable of producing hormones that regulate and are regulated by vitamin D. This interaction starts a sophisticated metabolic system that modulates both mineral and energy metabolism for the requirements of the musculoskeletal system. Among the so-called pleiotropic effects of vitamin D, those resulting from the inhibitory effect on the renin-angiotensin system are of particular interest for nephrologists. Intriguingly, however, more than for anti-hypertensive effects, this interaction could be relevant for anti-inflammatory actions, possibly representative of a residual ancestral role of vitamin D. In addition, this evolutionary dynamism of the vitamin D system should not be separated from the chemical dynamism that characterizes the ligand molecule and its specific receptor. Both are capable of significant tridimensional modifications that contribute to an increase in the variability and the partial predictability of their final biological effect. A dynamic overview of this system that takes into account its evolutionary and adaptive aspects may be helpful to understand its biological complexity and to envisage why using vitamin D metabolites for therapeutic purposes is still a matter of debate.
INTRODUCTION
The number of publications on vitamin D is constantly increasing and numbered over 4000 in 2014. The explanation for this increasing interest stems from the accumulative knowledge of this vitamin's mechanisms of action and from the parallel increase of potential clinical applications. Discovered as an anti-rachitic compound, vitamin D still represents the emblematic vitamin that fixes calcium to bone. However, epidemiologic and experimental data allow us to hypothesize numerous extra-skeletal effects [1]. Moreover, in recent years, we have begun to regard vitamin D as not just a simple vitamin but as a hormone and component of a sophisticated ‘vitamin D endocrine system’ that includes, besides the active hormone (calcitriol), specific enzymes, a number of metabolites, a definite receptor, a dedicated plasma transport protein and hormones regulating vitamin D synthesis and catabolism [2].
The aim of this review is to provide an evolutionary perspective of this hormone to help visualize how its biological and biochemical dynamisms could have been relevant for its transformation. It is necessary to note that the term vitamin D refers to any compound with the biological activity of 1,25-dihydroxyvitamin D and encompasses both vitamin D3 (cholecalciferol, typical of animal life) and ergocalciferol (vitamin D2, typical of vegetal life). In this paper we will refer, unless specified, to the compound of animal origin and its natural metabolites.
THE EVOLUTION OF VITAMIN D
We can find the precursor of vitamin D3, 7-deydrocholesterol or pro-vitamin D3, in very old living organisms, such as plankton. This allows dating its origin back to some 750 million years ago (MYA). The synthetic pathway of this precursor is much conserved, being shared with cholesterol. We can in fact assimilate pro-vitamin D3 to a cholesterol molecule without two hydrogens (Figure 1A). This resemblance allows us to hypothesize that in unicellular organisms, similar to cholesterol, vitamin D3 was a component of the plasma membrane. However, a specific feature characterizes this pro-vitamin D3: the capacity of absorbing UV-B rays in the same wavelength of DNA. For this reason, the biological role of pro-vitamin D3 in ancient organisms could have been that of protecting DNA from the toxic effects of UV-B rays, rather than regulating Ca metabolism [3]. After UV-B ray absorption, pro-vitamin D3 is transformed into the thermodynamically unstable pre-vitamin D3, which requires a final transformation into the more stable cholecalciferol or vitamin D3 (Figure 1B). As such, this new molecule could be regarded as an inactivated end-product; however, in very old organisms like algae, it is capable of promoting growth activity, which points to the persistence of some biological effect [4].
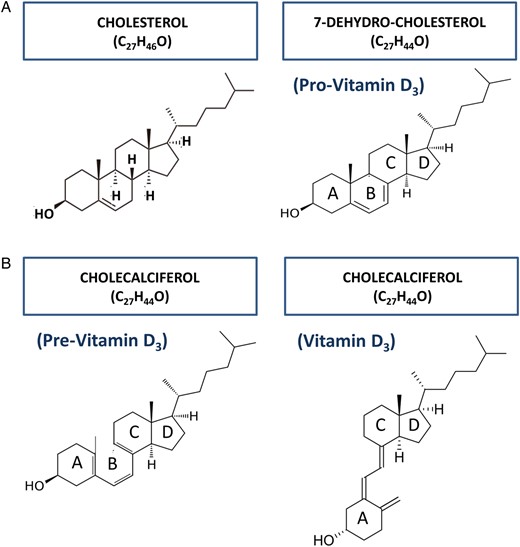
(A) 7-dehydrocholesterol, or pro-vitamin D3, shares the synthetic pathway with cholesterol, from which it differs by the absence of two hydrogen atoms. (B). Following UV-B ray absorption, the B ring of pro-vitamin D3 opens, thus becoming pre-vitamin D3. The new molecule is unstable and prone to transform into vitamin D3, by simply rotating around the C6–C7 single bond.
Similar to cholecalciferol, the specific enzymes capable of producing hydroxylated metabolites appear early in evolution, in yeasts and old plants that have no calcified structure. A role for vitamin D in the process of mineralization can be seen some 550 MYA in snails and crustaceans, in which 25-hydroxyvitamin D (25OHD3) [5] and 1,25-dihydroxyvitamin D [1,25(OH)2D3] [6] could have some implication in the mineralization processes (Figure 2).
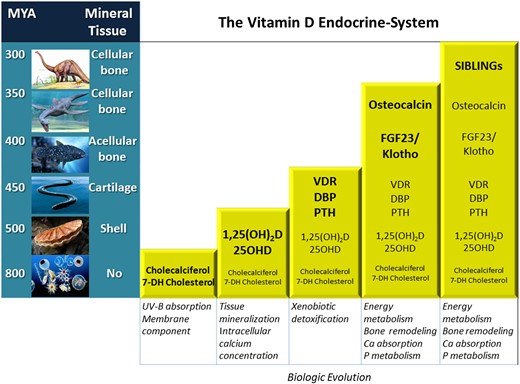
Accrual of the vitamin D system components along with the evolution of species. Notably, the appearance of secreting bone cells renders the system sophisticated and involved with mineral and energy metabolism. In addition, Klotho appearance antedates that of FGF23 by roughly 100 MY.
Later in evolution, in jawless fishes that possess a type of vitamin D receptor (VDR), the complexity of the vitamin D system increases slightly. In these cartilaginous (non-calcified) fishes, however, this ancestral receptor is involved in xenobiotic detoxification—a type of immune response—and not with calcification [7] (Figure 2). It is in teleosts with cellular and remodelling bone that VDR activation, for the first time, is involved with calcium and phosphate absorption and bone mineralization [8]. It is subsequently in terrestrial animals that the VDR becomes functionally divergent from that of aquatic animals by acquiring more definite ‘calcium-phosphate-oriented’ effects [9].
Along with the VDR, in vertebrates a specific vitamin D binding protein (DBP) also appears seemingly functional to the need of systemic transportation of the hydrophobic vitamin D metabolites [10] (Figure 2). This protein has a specific ligand binding domain (LBD) for vitamin D metabolites and circulates in excess amounts compared with ligands, thus representing a storage compartment. Further, it has different affinity for each of the vitamin D metabolites [e.g. 600 times higher for 25OHD3 than for 1,25(OH)2D3] and in this way regulates circulating levels of the free fractions of each metabolite [10].
In parallel with DBP appearance, a feedback system suppressing 1,25(OH)2D3 synthesis when VDR is activated becomes manifest [11]. The complexity of this system illustrates how tightly regulated are calcitriol levels, whose free circulating fraction is the one that is biologically active but, at the same time, also more susceptible to catabolism.
We can thus consider that many components of the vitamin D system (metabolites, enzymes, receptors and binding proteins) are present in early fishes but with an unlikely role in calcium homeostasis. In fact, plenty of calcium has been available in seawater (some 40 mg/dL) since at least the Cambrian explosion (the biological era characterized by an exponential increase in the number of life species in the sea). According to an evolutionary hypothesis, an increase in calcium concentration in seawater (secondary to rock dissolution) preceded the Cambrian era and was determinant for the explosion in the number of life species [12]. Accordingly, defensive or detoxifying roles are the most probable functions of vitamin D in more simple life species in the sea. Involvement with bone and mineral homeostasis seems to start in more evolved aquatic bony fishes and becomes progressively more evident later in terrestrial animals with cellular and remodelling bone. In these animals, the interaction with bone cells is a determinant for vitamin D to acquire a systemic role (Figure 2). A fine-tuned calcium regulation mechanism becomes evident through integrated activation of VDRs not only in bone but also in intestinal and renal cells, also thanks to cooperation with parathyroid hormone (PTH), PTH-related peptide and calcitonin, whose origin and evolution is still not settled. We know however, that PTH-like peptides are first present in cartilaginous fishes, suggesting roles unrelated to bone formation and an origin successive to vitamin D [13]. In any case, a considerable increase in the complexity of the vitamin D system became evident with the discovery that by interacting with bone cells, vitamin D regulates not only the process of mineralization but also the synthesis of two hormones, fibroblast growth factor 23 (FGF23) and osteocalcin (OC), which both have relevant metabolic actions [14].
FGF23 originates in early vertebrates from the duplication of the ancestral canonical FGF gene [15] and is mainly produced by osteocytes. It is an essential phosphate and vitamin D-regulating hormone, which lowers both phosphate and 1,25(OH)2D3 serum concentrations. Since 1,25(OH)2D3 can stimulate osteocyte synthesis of FGF23, a feedback system is clear. FGF23 null animals develop hyperphosphataemia, increased 1,25(OH)2D3 levels, hypercalcaemia, diffuse ectopic calcifications and premature aging [16]. This phenotype overlaps with that of Klotho null mice. In fact, Klotho is a co-receptor of FGF23, responsible for imparting tissue-specific affinity and sensitivity to FGF receptors. The complete biological relevance of the FGF23/Klotho system is still not settled and could possibly go beyond mineral metabolism, since direct cardiac effects have been demonstrated for both FGF23 [17] and Klotho [18] in experimental conditions and in renal patients [17]. Further, by regulating renal tubular Na-Cl co-transporters, FGF23 may influence blood volume and pressure [19]. In any case, it is deeply linked with vitamin D regulation and can be considered part of the vitamin D system.
OC, which first appeared in bony vertebrates [20], definitely represents another important endocrine function of the bone. Since its discovery, and due to the presence in its molecule of three γ-carboxylated glutamic acid residues that avidly bind calcium, OC has been considered a bone protein involved with mineralization processes. However, OC null mice show increased bone mass without mineralization defects in front of a clear phenotype of diabetes-obesity [21]. In fact, we now know that a metabolite of OC, undercarboxylated OC, which is mainly produced during bone resorption, stimulates insulin secretion by the pancreatic β-cells and promotes insulin sensitivity in peripheral organs while also modulating adipocyte activity [22]. Importantly, by stimulating its own receptor on osteoblasts, insulin inhibits the synthesis of osteoprotegerin in these cells, thus increasing osteoclast recruitment, bone resorption and further OC production (feed-forward feedback). Vitamin D, which regulates bone cells in general and can either stimulate transcription [23] or reduce circulating levels of OC [24], is thus involved with energy metabolism [25].
Further to FGF23 and OC, vitamin D has inhibitory effects on the bone synthesis of a protease-resistant circulating factor, the acidic serine aspartate rich MEPE-associated motif (ASARM). This peptide derives from the shedding of a motif from any of the small integrin-binding ligand N-linked glycoproteins (SIBLINGs), which is a special family of bone proteins. While the SIBLINGs are essential for regular mineralization of the extracellular matrix of bone and teeth [26], ASARM circulating peptide is a powerful inhibitor of mineralization. In addition, ASARM peptide can reduce the pH of the local matrix, thus favouring the production of undercarboxylated OC in the lacunae of bone resorption. We can regard the SIBLINGs and ASARM peptide as the most recently discovered components of the vitamin D endocrine system contributing to strengthen the link between bone and energy metabolism [27].
In summary, through a complex interaction with bone cells vitamin D becomes part of a broader metabolic regulatory system. In these cells vitamin D regulates several receptors and enzymes [like its own receptor, receptor activator of NF-κB ligand (RANKL), low-density lipoprotein receptor-related protein 5 (LRP5) and cystathionine B-synthase (CBS) among others] but also two bone hormones. From an evolutionary point of view, this hormonal interaction with bone marks a notable step in the evolution of vitamin D system as it moves progressively along with more complex animal species (Figure 2). This most probably reflects the necessary evolution and adaptation for life on Earth. Migration of living organisms from sea to land represented, in fact, a gigantic challenge. Prompt availability of calcium, which is abundant in the sea but scarce on land, was critical, and a storage organ, like bone, was necessary. On land, thanks to the cooperation with muscles, bone also allowed walking and running, which are essential to increase the probability of survival. Bones, which are packed with very active energy-requiring cells, and muscles—the musculoskeletal system—are an energy-expensive structure in need of a peculiar endocrine system capable of regulating extracellular mineral ion concentrations and energy metabolism at the same time. With its regulatory effects on bone cells, vitamin D represents a component of such a powerful and sophisticated system [28].
VITAMIN D PLEIOTROPISM
Vitamin D status is commonly assessed by serum levels of the metabolite 25OHD3, which reflects storage better than 1,25(OH)2D3. Optimal levels are not unanimously defined, but most experts agree that values <20 ng/mL indicate deficiency, values between 21 and 29 ng/mL indicate relative insufficiency and levels >30 ng/mL sufficiency [29]. With these limits, vitamin D insufficiency is increasingly appreciated in general and pathologic populations and the potential clinical consequences are feared.
In fact, besides the classical undisputed actions resulting from VDR activation in the intestine, bones and kidneys, vitamin D also has pleiotropic effects. In mammals, many tissues express VDR and, most importantly, numerous cell types in a variety of organs possess vitamin D hydroxylases allowing local, autocrine/paracrine production of active vitamin D metabolites. This gives a biological plausibility to vitamin D's claimed various non-skeletal health benefits. Indeed, observational studies link vitamin D deficiency with chronic illnesses like diabetes, cancer, infections, cardiovascular and autoimmune diseases. Experimental studies indicate, for example, that vitamin D may have favourable effects on prostate cancer cells through anti-proliferative effects (by modulation of Kreb's cycle, in an androgen-dependent way) [30] and pro-differentiating effects (by enhancement of gap junction formation) [31]. However, evidence for a protective role of adequate vitamin D intake is more convincingly established for rickets/osteomalacia [32] and osteoporotic fractures [33] than for falls [34] or premature mortality [35]. There is much less evidence for beneficial effects on cancer, infections, autoimmune or cardiovascular diseases, although this could be due to the existence of different therapeutic target levels for each disease [36].
Among the so-called pleiotropic effects of vitamin D, one possibly linked with evolution is the relationship with the renin-angiotensin system (RAS). Li et al. [37] unambiguously demonstrated this link in a model of VDR null mice characterized by hyperplasia of the macula densa and hyper-reninism. Since then the biological plausibility of observational studies reporting a negative association between vitamin D status and hypertension has increased. Actually, however, the clinical relevance of the association remains rather weak since a recent meta-analysis concluded that vitamin D supplementation is ineffective for lowering blood pressure [38]. Notwithstanding this argument, the link between vitamin D and RAS could be intriguing in a different way. The RAS is present in primitive animals that do not have a circulatory system, thus excluding a regulatory role on blood volume or pressure [39]. Alternatively, a pro-inflammatory effect of RAS is quite possible in these animals. Angiotensin I receptors, responsible for the biological effects of RAS, are expressed in several different tissues not involved with blood pressure regulation and can be found, in particular, in the mitochondria. Activation of these receptors increases oxidant production and reduces mitochondrial lifespan thus promoting inflammation and cellular aging [40]. On the other hand, we know that activation of VDRs, which are similarly detectable in mitochondria [41], result in anti-inflammatory effects through inhibition of reactive oxygen species and NF-κB synthesis [42]. Intriguingly, during evolution, VDR and RAS appear contemporarily in many animal species. Thus, according to Ferder et al. [43] it is possible to hypothesize that, in very old animals, vitamin D and RAS were antagonistic systems modulating the inflammatory response—i.e. an ancient type of immune response. Both systems evolved to acquire specialized functions essential for life on Earth. In fact, blood volume and pressure (necessary to circulate internal water) and bone (necessary to guarantee adequate ions and energy supply) are both essential for life on land. So it seems that when searching for pleiotropic effects of vitamin D or for non-blood pressure-related effects of RAS, we are in fact exploring ancient functions of these two systems. To what extent we will succeed in using these ancient properties for clinical purposes is still an open question warranting further research.
CHEMICAL AND BIOCHEMICAL DYNAMISM OF VITAMIN D AND ITS RECEPTOR
The chemical properties of the vitamin D3 molecule are unique compared with parent sterol ligands (e.g. oestrogens), similarly capable of inducing genomic and non-genomic effects by acting on the same receptor [44]. In fact, while other sterols have rigid molecular structures, vitamin D3 should be seen as a dynamic molecule capable of quickly sampling numerous different conformations, resulting in different tridimensional shapes [45, 46]. This dynamism, which, as illustrated later, also involves the VDR, allows explanation of how vitamin D metabolites or synthetic analogues may elicit somewhat different responses by activating the same receptor.
The dynamism of the molecule starts with the UV-B-induced opening of the B ring that prompts the A ring to rotate around the C6–C7 single bond and give rise to vitamin D3 (Figure 1B). In the new molecule, the central C-D ring is regarded as rigid (the rigid backbone) but the A ring and the side chain are definitely flexible. The A ring, by freely rotating above and below the C-D ring plain, adapts to either cis- or trans-conformations [47]; it may also change into two further shapes, called α-chair or β-chair [48]. The side chain, which is the most dynamic region, can rotate 360 degrees around the single bond that links it to the C-D ring, and each carbon of the chain also has some flexibility (Figure 3). The number of tridimensional conformations that a metabolite can sample is thus very high and interconversion of shapes occur millions of times per second. For example, the two most powerful metabolites among the numerous natural vitamin D sterols, namely 25OHD3 and 1,25(OH)2D3, are also the most conformationally dynamic [49]. Importantly, modifications occurring in the carbon scaffold (e.g. hydroxylation, methylation, etc.) will decrease or increase the number of possible tridimensional conformations that the molecule can sample, thus affecting its biologic activity. In fact, as an example, both 25OHD3 and 1,25(OH)2D3 interact with the VDR, but due to a more precise stereospecific binding, the second is much more powerful. Nonetheless, 25OHD3 also has specific biologic effects, which have been claimed for many years and confirmed, even recently, to occur through VDR activation [50]. Further, since as illustrated in Figure 3, specific regions in the vitamin D molecule have specific functions [49, 51, 52], we can predict that changes in the shape of the molecule in one of these regions will modify a specific step in the vitamin D metabolism or receptor interaction. It is thus accepted that conformational flexibility is one of the components of the physicochemical and biologic properties of each vitamin D metabolite.
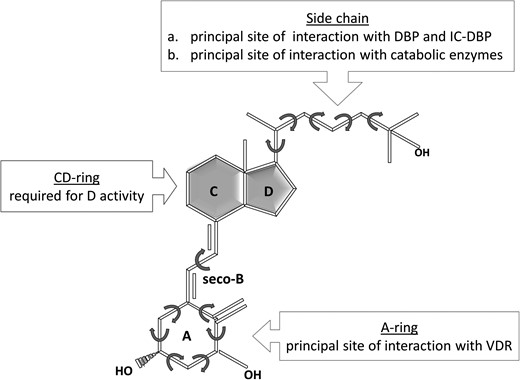
Vitamin D3 molecule is characterized by unique dynamic properties and can sample numerous tridimensional shapes. Also, different functional roles can be recognized in the three different parts of this ligand. DBP = vitamin D binding protein; IC-DBP = intracellular DBP.
To exert their genomic effect ligands need to interact not just with the VDR, but also with other proteins (RXR-co-receptor, activator and repressor complexes) that are determinants of the final effect. Notably, ligand interaction with the VDR produces critical conformational receptor changes that are relevant, as illustrated below, for the eventual activation process of this complex cascade that has been renamed ‘the VDR machine’.
In the VDR, similar to the ligand molecule, we recognize different structural and functional portions: LBD, connective hinge, DNA-binding domain (DBD) and A/B domains, each with specific roles in the steps leading to the genomic effects (Figure 4A). The LBD, which is critical for interaction with vitamin D, contains in its internal portion the so-called ligand binding pocket (LBP), into which the ligand will lodge. This pocket contains specific anchoring points and conformationally adapts to the ligand, the final shape being different and specific for each individual ligand (Figure 4B) [53]. The specific ligand-induced conformational modification of the LBP eventually affects the shape of the whole VDR, thus becoming a further variable in the activation of the VDR machine. In fact, the final shape of the activated receptor is responsible for the stability and selectivity of the interactions with the proteins (RXR-co-receptor, co-repressors and/or co-activators), which are an essential component of the machine. Since the VDR is expressed in cells of different tissues, the repressor or activator protein-complexes will be different and have a different affinity for the activated receptor, thus increasing the variability of the response (Figure 5A and B). The importance of this shape modification has been exploited by the industry to develop some 3000 synthetic analogues of vitamin D, with more selective biological properties than natural compounds.

Schematic of the VDR. (A). Different functional domains are present in the VDR. (B). Interaction with the ligand changes the shape of the LBD into a different one, specific of each potential ligand.
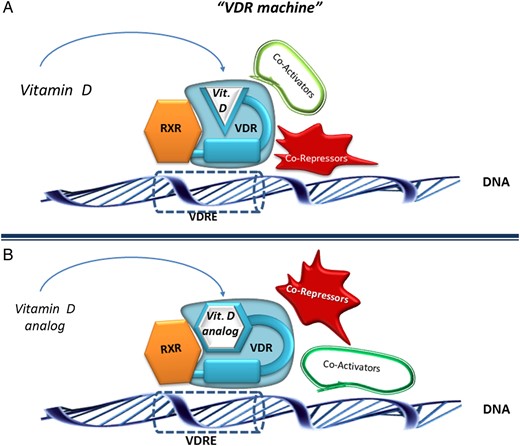
Schematic of the so-called ‘VDR machine’. The final shape of the VDR differs according to binding of a natural vitamin D ligand (A) or of a vitamin D analogue (B). This modifies the affinity of the VDR with co-activators or co-repressor protein-complexes, thus contributing the variability of the biological response. RXR: retinoid X receptor; VDR: vitamin D receptor; VDRE: vitamin D response element.
Vitamin D and VDR dynamism are also invoked by some authors to explain how either genomic or non-genomic effects can be elicited following the activation of the same receptor protein [54]. In fact, a synthetic analogue characterized by the A ring locked in cis-position has been shown to exert almost exclusively rapid (non-genomic) effects, suggesting that this naturally occurring conformation might be the one responsible for this type of effect [55].
There is one last consideration to make about the dynamism of vitamin D and its receptor: when we try to recreate any of the three-dimensional modelling of their interaction, we are actually taking a single picture of the whole film occurring in vivo.
CONCLUSIONS
Vitamin D should be regarded as a very conserved molecule that probably started as a component of the plasma membrane of simple organisms, with protective or defensive roles. Its function has changed to become a necessary regulatory element of the metabolic and energetic requirements of life on Earth. The dynamism of the molecule and its receptor certainly represents an element of the plasticity that allowed the acquisition of such highly specialized properties within the musculoskeletal system. It is impressive that during evolution the appearance of cellular bone increases the complexity of the system, with the production of bone proteins that counter-regulate the effects of vitamin D on bone and muscles. Significantly, the VDR, possibly because of its ancestral biological roles, is present in numerous tissues not necessarily involved with the musculoskeletal system. Among the claimed pleiotropic properties, the systemic but generic anti-inflammatory effects could be related to the RAS-inhibitory properties. Given this complex biology, it seems rather simplistic to try to deduce its function through the assay of one or two blood circulating metabolites. A dynamic view of the interaction with its receptor is certainly helpful to try to understand why we cannot exactly predict the biological effects of the natural compounds. In fact, the recommended doses of this ‘vitamin’ either for healthy people or for patients are still a matter of debate. A dynamic view also helps to understand why synthetic analogues can have very specific biologic effects and can be considered for very different clinical purposes (from cancer to infection) through activation of the same receptor. Vitamin D and its receptor should be regarded as a rather sophisticated molecular machine that we are still discovering. Much more remains to be discovered, but a general overview that takes into account the evolutionary aspects of this system seems useful to try to understand the multifaceted biology of this ‘vital amine’.
CONFLICT OF INTEREST STATEMENT
None declared.
Comments