-
PDF
- Split View
-
Views
-
Cite
Cite
Fatih Kircelli, Mirjam E. Peter, Ebru Sevinc Ok, Fatma Gul Celenk, Mumtaz Yilmaz, Sonja Steppan, Gulay Asci, Ercan Ok, Jutta Passlick-Deetjen, Magnesium reduces calcification in bovine vascular smooth muscle cells in a dose-dependent manner, Nephrology Dialysis Transplantation, Volume 27, Issue 2, February 2012, Pages 514–521, https://doi.org/10.1093/ndt/gfr321
- Share Icon Share
Abstract
Vascular calcification (VC), mainly due to elevated phosphate levels, is one major problem in patients suffering from chronic kidney disease. In clinical studies, an inverse relationship between serum magnesium and VC has been reported. However, there is only few information about the influence of magnesium on calcification on a cellular level available. Therefore, we investigated the effect of magnesium on calcification induced by β-glycerophosphate (BGP) in bovine vascular smooth muscle cells (BVSMCs).
BVSMCs were incubated with calcification media for 14 days while simultaneously increasing the magnesium concentration. Calcium deposition, transdifferentiation of cells and apoptosis were measured applying quantification of calcium, von Kossa and Alizarin red staining, real-time reverse transcription–polymerase chain reaction and annexin V staining, respectively.
Calcium deposition in the cells dramatically increased with addition of BGP and could be mostly prevented by co-incubation with magnesium. Higher magnesium levels led to inhibition of BGP-induced alkaline phosphatase activity as well as to a decreased expression of genes associated with the process of transdifferentiation of BVSMCs into osteoblast-like cells. Furthermore, estimated calcium entry into the cells decreased with increasing magnesium concentrations in the media. In addition, higher magnesium concentrations prevented cell damage (apoptosis) induced by BGP as well as progression of already established calcification.
Higher magnesium levels prevented BVSMC calcification, inhibited expression of osteogenic proteins, apoptosis and further progression of already established calcification. Thus, magnesium is influencing molecular processes associated with VC and may have the potential to play a role for VC also in clinical situations.
Introduction
Vascular calcification (VC) is common in patients suffering from chronic kidney disease (CKD) and has a progressive nature which eventually leads to increased mortality rates compared to the general population [ 1 , 2 ]. It has been shown that VC is not only due to passive precipitation of calcium phosphate but rather resembles an active process involving transdifferentiation of vascular smooth muscle cells (VSMCs) into osteoblast-like cells, including changes in the expression of bone-associated and mineralization-regulating proteins as well as apoptosis [ 3 , 4 ]. However, the underlying pathophysiologic mechanisms are poorly understood. It is proposed that along with traditional cardiovascular risk factors, non-traditional risk factors, such as those related to uraemic status and a disturbed bone and mineral metabolism, may play a role in the increased prevalence of VC and cardiovascular disease in CKD patients.
Magnesium, in addition to its involvement in the functions of many enzymes, plays a role in skeletal and mineral metabolism and vascular tone. Recently, data from observational clinical studies suggested that serum magnesium concentrations are inversely related with the presence of VC or atherosclerosis [ 5 , 6 ] and also with cardiovascular and all-cause mortality in uraemic as well as non-uraemic populations [ 7–9 ]. However, so far, only a limited number of experimental studies, mostly performed in animals have confirmed these findings, whereas the majority investigated the role of lowering dietary magnesium on VC [ 10–12 ]. To date, only one group has investigated the role of magnesium in an in vitro model in a prechondrogenic cell line and reported a favourable effect of magnesium on bone matrix mineralization [ 13 ]. However, the effect of magnesium on calcification has not yet been studied in in vitro models.
Therefore, the aim of the present study was to investigate whether increasing magnesium concentrations have effects on calcification of bovine vascular smooth muscle cells (BVSMCs).
Materials and methods
Cell culture
BVSMCs were purchased from Corriell Cell Repositories and were used between Passages 2 and 8. Cells were cultured in glucose Dulbecco's modified Eagle's medium (DMEM) (0.9 mM P, 1.8 mM Ca 2+ , 0.8 mM MgSO 4 ) supplemented with 10% fetal bovine serum (FBS). After addition of FBS, Mg concentration was measured to be 1.0 mM.
Calcification assay
BVSMC calcification was induced as previously described [ 14 ]. Briefly, 0.6 × 10 4 cells per well (6-well format) were incubated for 7–14 days with high-glucose DMEM, supplemented with 15% FBS, 10 mM β-glycerophosphate (BGP), 50 mg/mL ascorbic acid, 10 −7 mol/L insulin, 10 mmol/L sodium pyruvate and 10 000 units/mL penicillin G, 10 mg/mL streptomycin sulphate and 25 μg/mL amphotericin B (v/v 1:100) (calcification media). MgCl 2 was added to reach final Mg 2+ concentrations of 2 and 3 mM. Control medium was prepared equally but without BGP.
Quantification of calcium deposition
BVSMCs were decalcified with 0.6N HCl for 24 h at the time point indicated and calcium content of the supernatants was determined by o -cresolphthalein complex method (Calcium kit; Pointe Scientific, Canton, MI). Calcium content was normalized to the protein content using BCA protein assay kit (Pierce, Rockford, IL).
Alkaline phosphatase (ALP) activity
Alkaline phosphatase (ALP) activity was quantified using a commercially available kit (Pointe Scientific) according to the manufacturer’s protocol. Protein concentrations were quantified using BCA protein assay kit and ALP activity was normalized for cellular protein content.
Von Kossa and Alizarin red staining
Nodules were fixed in 10% buffered neutral formalin phosphate for 24 h at 4°C, washed, incubated with 5% silver nitrate solution for 30–90 min and exposed to 5% sodium thiosulphate for 2 min. Counterstaining was performed in 2 mL nuclear fast red. For Alizarin red staining, cells were incubated with 10% neutral buffered formalin for 15 min, rinsed and incubated with a 2% aqueous Alizarin red solution (pH 4.0–4.2) for 10–15 min.
Real-time reverse transcription–polymerase chain reaction
The quantification of selected transcripts was performed using real-time TaqMan® PCR technology. Complementary DNA (cDNA) was synthesized from 2 μg of RNA using a commercially available kit (High Capacity cDNA Archive Kit; Applied Biosystems Inc., Foster City, CA) according to the manufacturer’s instructions. Quadruplicate real-time TaqMan® polymerase chain reactions (PCR) was carried out with the ABI Prism 7900HT Sequence Detector System (Applied Biosystems) using cDNA equivalent to 5 ng of RNA with specific real-time PCR assays (Assays-on-Demand Gene Expression System; Applied Biosystems). Expression levels were determined applying the comparative threshold cycle (ΔCt) method using glyceraldehyde-3-phosphate dehydrogenase as endogenous control.
Enzyme-linked immunosorbent assay measurements
After 14 days of incubation with the indicated media, supernatants of the cells were stored at −80°C. ELISA kit for Matrix-Gla protein (MGP) was purchased from Biomedica (Vienna, Austria), for bone morphogenetic protein 2 (BMP2) from R&D Systems (Minneapolis, MN) and measurements were performed according to the manufacturer’s protocol. Media calcium, phosphorus and magnesium were determined by standard biochemical methods using an autoanalyzer (Architect c16000; Abbott Diagnostics, Abbott Park, IL).
Western blot analysis
BVSMCs were incubated with calcification media in a 6-well format for 14 days. Cells were lysed in a buffer containing 20 mM Tris–HCl, 2 mM ethyleneglycoltetraacetic acid, 5 mM ethylenediaminetetraacetic acid and 80 μg of total protein was fractioned by 12% sodium dodecyl sulphate–polyacrylamide gel electrophoresis and electro-transferred to nitrocellulose membranes. The membranes were blocked with Tris-buffered saline (pH 7.4)/5% skimmed milk powder/0.1% Tween and incubated with the indicated antibodies according to the manufacturer’s protocol. Horseradish peroxidase (HRP)-labelled antibodies were visualized with enhanced chemiluminescence detection system (Amersham Biosciences, Piscataway, NJ) on standard X-ray film. After scanning of the films, software-aided densitometric analysis was performed (ImageQuant TL v2005; Amersham Biosciences). Runx2 (M-70) antibody was from Santa Cruz (Santa Cruz Biotechnology Inc., CA) and β-actin antibody and HRP-labelled secondary antibody from Sigma–Aldrich (St Louis, MO).
Apoptosis and cell viability
The percentage of apoptotic cells was determined by incubating BVSMCs under the indicated conditions and performing annexin V-FITC staining according to the manufacturer’s protocol (Becton Dickinson, Franklin Lakes, NJ). Cell viability was assessed by Celltiter 96 Aqueous One Cell Proliferation Assay (Promega, Madison, WI).
Statistical analysis
Means of more than two variables were compared by performing an analysis of variance. In the case of significant difference in means of more than two variables, means of all groups were compared pairwise adjusting the alpha according to the method of Bonferroni to correct for multiple testing.
Results
The aim of this study was to determine the effect of magnesium on calcification in a cell culture model in order to reveal mechanisms underlying the above described positive effects of magnesium on cardiovascular parameters. Therefore, the influence of different medium magnesium levels on BGP-induced calcification was assessed in BVSMCs. Read outs were tissue content of calcium as well as other molecular markers, known to be involved in the progression of VC.
BGP-induced deposition of calcium in BVSMCs
The addition of BGP to the culture media induces high phosphate levels and the deposition of calcium in VSMCs [ 15 ]. Concordantly, we could observe a strong accumulation of calcium after incubation of BVSMCs for 7 days with 10 mM BGP ( Figure 1A ). The medium concentration of 1.1 mM magnesium matches the normal serum magnesium concentrations in cattle as well as in humans. To evaluate the effect of magnesium on BVSMC calcification, the amount of calcium per mg protein was quantified in the presence of BGP as well as with increasing magnesium concentrations. Higher magnesium concentrations led to a strong reduction of calcium content in the cells compared to BGP alone. This inhibitory effect of magnesium was even more pronounced after a cultivation period of 21 days, showing a reduction of calcium content in the presence of 2 mM magnesium by 76% ( Figure 1B ). To test whether the progression of already established calcification could be prevented, magnesium was added to the cells 7 and 14 days after initiation of calcification with BGP ( Figure 1C ). Addition of magnesium at day 7 strongly inhibited further accumulation of intracellular calcium. At day 14, cells incubated with BGP and magnesium showed a significant inhibition compared to incubation with BGP alone. Further incubation with magnesium (until day 14, measured 21 days after initiation of calcification) even led to a slight but significant reduction compared to measurement on day 14. Addition of magnesium at day 14 of calcification still inhibited progression and measurement on day 21 showed that the established calcification of day 14 was significantly reduced.
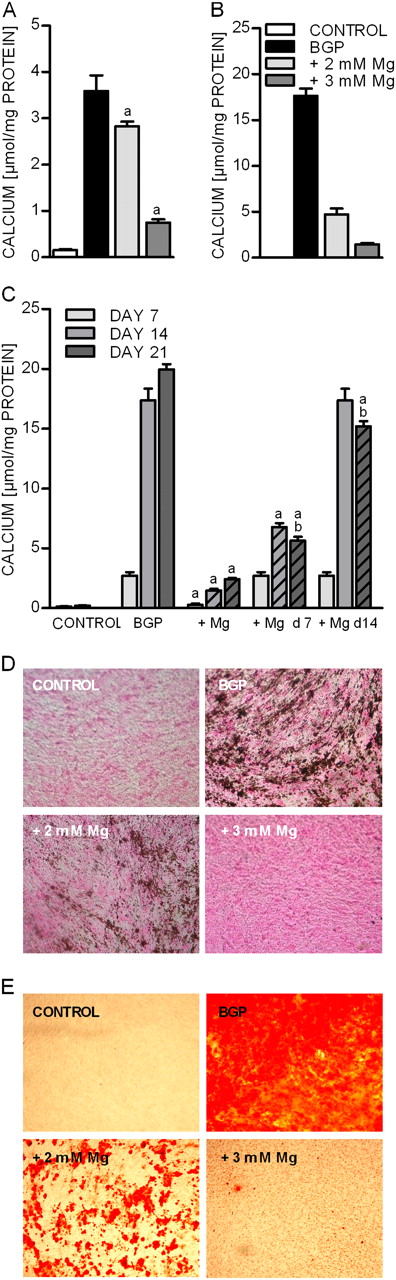
Effect of magnesium on BGP-induced deposition of calcium in BVSMCs. BVSMCs were incubated for 7 days ( A ) and 21 days ( B ) under calcifying conditions (+10 mM BGP) either in the presence of physiological (1.1 mM = control) or elevated extracellular magnesium (2 and 3 mM). Magnesium was added from start (A and B) or at the time points indicated ( C ). Dashed lines in graph C indicate the presence of magnesium. Data are given as means and SD of at least six replicates (a = difference versus BGP: P < 0.05, b = difference versus same condition Day 14: P < 0.05). In parallel, von Kossa ( D ) Alizarin red ( E ) staining was performed with cells treated for 14 days under the same conditions as mentioned above.
The inhibition of calcium deposition by elevated extracellular magnesium concentrations could be confirmed in von Kossa and Alizarin red stainings ( Figure 1D and E ).
Markers of transdifferentiation into osteoblast-like cells
It has been shown that VC is not only a passive process due to an elevated calcium phosphate product. The pathophysiological mechanisms also include active processes like the transdifferentiation of BVSMCs into osteoblast-like cells, which are then capable of producing extracellular matrix components normally involved in bone formation [ 3 , 4 ]. To assess a possible influence of magnesium on the formation of osteoblast-like cells, we investigated the expression of two transcription factors for osteoblastic differentiation: CBFA1 and MSX2 as well as a chondrocyte transcription factor SOX9 in the presence of normal and elevated magnesium concentrations, respectively. As expected, CBFA1, MSX2 as well as SOX9 were induced in the presence of BGP ( Figure 2A and B ). CBFA1 showed 2.13-fold expression in the presence of 10 mM BGP which was strongly inhibited in the presence of 2 and 3 mM magnesium. The concentration-dependent inhibition of BGP-induced CBFA1 by magnesium was confirmed on protein level by western blot analysis ( Figure 2D ). BGP-induced MSX2 up-regulation was restored to control levels by incubation with 2 or 3 mM magnesium ( Figure 2A ). The prominent induction of SOX9 by BGP was reduced in the presence of 2 mM magnesium and nearly completely inhibited in the case of 3 mM magnesium ( Figure 2B ).
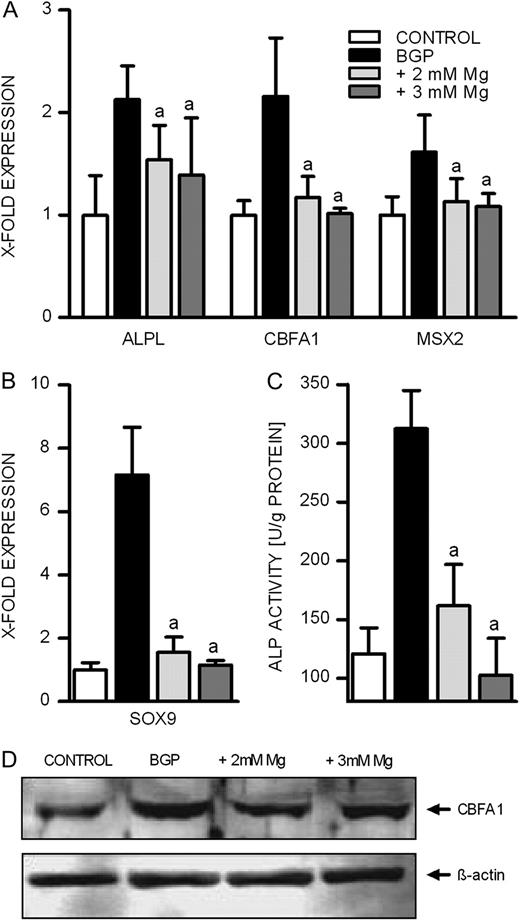
Effect of magnesium on BGP-induced expression of proteins involved in calcification in BVSMCs. BVSMCs were incubated for 14 days under calcifying conditions either in the presence of physiological or elevated extracellular magnesium. ALP activity was quantified in cell lysates ( C ) and normalized to the amount of protein. For western blot analysis equal amounts of proteins were stained for CBFA1> and ß-actin ( D ). ( A , B and C) data shown are means and SD of eight replicates (a = difference versus BGP: P < 0.05).
ALP expression and activity
ALP up-regulation has been shown to be involved in the process of calcification of BVSMCs. In our experiments, BGP led to an ∼2-fold up-regulation of ALP expression relative to control conditions ( Figure 2A ). This increase was reduced with 2 and 3 mM magnesium by 52 and 66%, respectively. On a functional level, measured in terms of enzyme activity, we observed an even stronger inhibitory effect of magnesium ( Figure 2C ): elevating the magnesium concentration to 2 mM led to a 79% reduction of the BGP-induced increase in ALP activity and 3 mM extracellular magnesium even reduced ALP activity to levels below control conditions.
MGP secretion under calcifying conditions
Under physiological conditions, there are various mechanisms in BVSMCs to counteract the transdifferentiation into osteoblast-like cells and the deposition of calcium in form of hydroxyapatite crystals. MGP is one known inhibitor of calcification and was shown to be down-regulated in calcified vessels [ 16 ]. Incubating BVSMCs with BGP resulted in an 86% decrease of MGP secretion, while simultaneously increasing the extracellular magnesium concentration normalized the MGP secretion to control levels ( Figure 3A ). Surprisingly, further addition of magnesium (3 mM) even increased MGP concentrations by 68% above normal values.
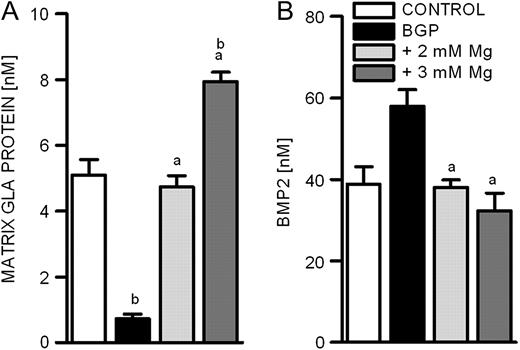
Influence of magnesium on the secretion of proteins involved in calcification. Protein concentrations of MGP and BMP2 were quantified in the supernatants of BVSMCs by ELISA ( A and B ). Cells were incubated for 14 days under calcifying conditions either in the presence of physiological or elevated extracellular magnesium concentrations. Data shown are means and SD of eight replicates (a = difference versus BGP: P < 0.05; b = difference versus control: P < 0.05).
MGP is known to mediate its action in part by binding BMP2, thereby inhibiting its pro-calcifying actions [ 17 ]. BMP2 was increased in BVMCs after 7 days of incubation with calcification media ( Figure 3B ). When co-incubating the cells with 2 mM magnesium, we observed a restoration to control levels and a further elevation to 3 mM magnesium led to strong reduction of BMP2 secretion to levels below control.
Apoptosis and elevated phosphate concentrations
Apoptosis has been identified as an important contributor to VC [ 18 ]. We therefore evaluated the effects of magnesium on BGP-induced apoptosis and on cell viability. After 14 days of incubation, the addition of BGP doubled the percentage of apoptotic BVSMCs compared to physiological conditions ( Figure 4A ). Higher magnesium levels (2mM) during the calcification period reduced this effect and in the case of 3 mM extracellular magnesium led to levels of apoptosis similar to the control group. The observed effects were reflected in cell viability, which was reduced after incubation with the phosphate source BGP and could be restored by the addition of further magnesium to the cell culture media ( Figure 4B ).
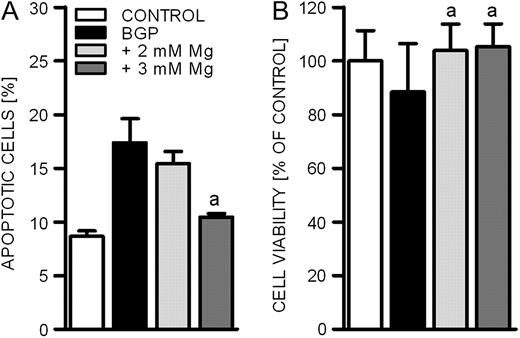
Effect of calcification on the induction of apoptosis and on cell viability. To induce calcification, BVSMCs were incubated in the presence of 10 mM BGP for 14 days. The inhibitory effect of magnesium was assessed by raising the physiological magnesium concentration in the media from 1.1 mM (control) to 2 and 3 mM. The percentage of apoptotic cells ( A ) and cell viability ( B ) was quantified. Data shown are mean and SD of 4 (A) and 3 (B) experiments (a = difference versus BGP: P < 0.05).
Extracellular magnesium concentrations and calcium entry in BVSMCs
Most of the effects shown to be involved in the process of calcification in BVSMCs were influenced by magnesium in our experiments. Therefore, we evaluated the influence of magnesium on calcium entry into the cells, a prerequisite for calcification. To estimate the amounts of magnesium and calcium taken up by BVSMCs, we measured the respective concentration in the media after an incubation period of 48 h in the presence of increasing magnesium concentrations ( Tables 1 and 2 ). Calculating the difference to concentrations present in the media before incubation with BVSMCs, we estimated the total amount of magnesium and calcium taken up by the cells ( Table 1 ). Increasing extracellular magnesium led to a dose-dependent increase in estimated uptake of magnesium itself, while simultaneously decreasing calcium entry into the cells ( Table 2 ). This was true, albeit the fact that calcium concentration at the beginning of the experiment was kept constant under all conditions investigated.
Initial Mg [mM] | Mg after 48 h [mM] | Δ Mg [mM] | Δ Mg [nmol] | |
Control | 1.1 | 0.77 ± 0.02 | 0.33 ± 0.02 | 0.66 ± 0.05 |
BGP | 1.1 | 0.75 ± 0.06 | 0.35 ± 0.06 | 0.69 ± 0.12 |
+2 mM Mg | 2 | 0.84 ± 0.12 | 1.16 ± 0.12 | 2.23 ± 0.24 |
+3 mM Mg | 3 | 1.62 ± 0.14 | 1.38 ± 0.14 | 2.76 ± 0.29 |
Initial Mg [mM] | Mg after 48 h [mM] | Δ Mg [mM] | Δ Mg [nmol] | |
Control | 1.1 | 0.77 ± 0.02 | 0.33 ± 0.02 | 0.66 ± 0.05 |
BGP | 1.1 | 0.75 ± 0.06 | 0.35 ± 0.06 | 0.69 ± 0.12 |
+2 mM Mg | 2 | 0.84 ± 0.12 | 1.16 ± 0.12 | 2.23 ± 0.24 |
+3 mM Mg | 3 | 1.62 ± 0.14 | 1.38 ± 0.14 | 2.76 ± 0.29 |
Calcification was induced in BVSMCs in the presence of physiological or increasing magnesium concentrations for 14 days. Magnesium uptake was estimated by calculating the differences of magnesium present in the media before and after incubation for 48 h with fresh media containing the same additives as before.
Initial Mg [mM] | Mg after 48 h [mM] | Δ Mg [mM] | Δ Mg [nmol] | |
Control | 1.1 | 0.77 ± 0.02 | 0.33 ± 0.02 | 0.66 ± 0.05 |
BGP | 1.1 | 0.75 ± 0.06 | 0.35 ± 0.06 | 0.69 ± 0.12 |
+2 mM Mg | 2 | 0.84 ± 0.12 | 1.16 ± 0.12 | 2.23 ± 0.24 |
+3 mM Mg | 3 | 1.62 ± 0.14 | 1.38 ± 0.14 | 2.76 ± 0.29 |
Initial Mg [mM] | Mg after 48 h [mM] | Δ Mg [mM] | Δ Mg [nmol] | |
Control | 1.1 | 0.77 ± 0.02 | 0.33 ± 0.02 | 0.66 ± 0.05 |
BGP | 1.1 | 0.75 ± 0.06 | 0.35 ± 0.06 | 0.69 ± 0.12 |
+2 mM Mg | 2 | 0.84 ± 0.12 | 1.16 ± 0.12 | 2.23 ± 0.24 |
+3 mM Mg | 3 | 1.62 ± 0.14 | 1.38 ± 0.14 | 2.76 ± 0.29 |
Calcification was induced in BVSMCs in the presence of physiological or increasing magnesium concentrations for 14 days. Magnesium uptake was estimated by calculating the differences of magnesium present in the media before and after incubation for 48 h with fresh media containing the same additives as before.
Initial Ca [mM] | Ca after 48 h [mM] | Δ Ca [mM] | Δ Ca [nmol] | |
Control | 1.8 | 1.78 ± 0.07 | 0.03 ± 0.06 | 0.06 ± 0.13 |
BGP | 1.8 | 0.53 ± 0.05 | 1.27 ± 0.05 | 2.55 ± 0.10 |
+2 mM Mg | 1.8 | 0.78 ± 0.01 | 1.02 ± 0.15 | 2.04 ± 0.03 |
+3 mM Mg | 1.8 | 1.58 ± 0.07 | 0.22 ± 0.07 | 0.44 ± 0.14 |
Initial Ca [mM] | Ca after 48 h [mM] | Δ Ca [mM] | Δ Ca [nmol] | |
Control | 1.8 | 1.78 ± 0.07 | 0.03 ± 0.06 | 0.06 ± 0.13 |
BGP | 1.8 | 0.53 ± 0.05 | 1.27 ± 0.05 | 2.55 ± 0.10 |
+2 mM Mg | 1.8 | 0.78 ± 0.01 | 1.02 ± 0.15 | 2.04 ± 0.03 |
+3 mM Mg | 1.8 | 1.58 ± 0.07 | 0.22 ± 0.07 | 0.44 ± 0.14 |
Calcification was induced in BVSMCs in the presence of physiological or increasing magnesium concentrations for 14 days. Ca uptake was estimated by calculating the differences of magnesium present in the media before and after incubation for 48 h.
Initial Ca [mM] | Ca after 48 h [mM] | Δ Ca [mM] | Δ Ca [nmol] | |
Control | 1.8 | 1.78 ± 0.07 | 0.03 ± 0.06 | 0.06 ± 0.13 |
BGP | 1.8 | 0.53 ± 0.05 | 1.27 ± 0.05 | 2.55 ± 0.10 |
+2 mM Mg | 1.8 | 0.78 ± 0.01 | 1.02 ± 0.15 | 2.04 ± 0.03 |
+3 mM Mg | 1.8 | 1.58 ± 0.07 | 0.22 ± 0.07 | 0.44 ± 0.14 |
Initial Ca [mM] | Ca after 48 h [mM] | Δ Ca [mM] | Δ Ca [nmol] | |
Control | 1.8 | 1.78 ± 0.07 | 0.03 ± 0.06 | 0.06 ± 0.13 |
BGP | 1.8 | 0.53 ± 0.05 | 1.27 ± 0.05 | 2.55 ± 0.10 |
+2 mM Mg | 1.8 | 0.78 ± 0.01 | 1.02 ± 0.15 | 2.04 ± 0.03 |
+3 mM Mg | 1.8 | 1.58 ± 0.07 | 0.22 ± 0.07 | 0.44 ± 0.14 |
Calcification was induced in BVSMCs in the presence of physiological or increasing magnesium concentrations for 14 days. Ca uptake was estimated by calculating the differences of magnesium present in the media before and after incubation for 48 h.
Discussion
Previous clinical and animal studies in both uraemic and non-uraemic conditions have shown an association of lower magnesium levels with increased VC as well as a decreased risk of peripheral VC with increased serum magnesium in non-diabetic haemodialysis patients [ 12 , 15 , 19–21 ]. Furthermore, magnesium supplementation reduced media intima thickness in these patients [ 6 ]. Recently, elevated dietary magnesium was shown to prevent tissue mineralization in an animal model and low magnesium diet in a mouse model for dystrophic cardiac calcification-induced marked cardiac calcifications [ 12 , 22 ]. However, only few in vitro studies providing mechanistic insight into the action of magnesium on VC were performed so far. Our study, for the first time, provides in vitro evidence for the preventive effect of magnesium on calcification in a cell culture model of BVSMCs.
In the present study, we found that increasing magnesium concentrations in the media of BVSMCs strongly inhibit BGP-induced calcification. This effect was dose- dependent and stable over a period of up to 21 days. It has been shown that magnesium is able to impair hydroxyapatite crystal growth in in vitro experiments and it has been suggested that the effect of magnesium on VC and atherosclerosis may be mediated via this mechanism [ 23–25 ], which is supported by the decrease in calcium deposition with increasing magnesium concentrations observed in our experiments. In the present study, magnesium was not only able to prevent BVSMC calcification but also to inhibit progression of already established calcification. With longer incubation time, magnesium even partly reverted calcification, which would be of great clinical importance. Although CKD patients are treated with phosphate binders in order to lower serum phosphorus concentrations, as one aspect of VC development, VC progresses overtime. Therefore, magnesium supplementation or treatment with magnesium-containing phosphate binders in CKD patients might be able to partially revert or at least inhibit the progression of VC.
In addition to the role of deposition of calcium–phosphate in terms of matrix mineralization, it is now well established that VC is an active, cell-mediated process including apoptosis as well as the osteochondrogenic differentiation of VSMCs showing similarities to bone formation [ 3 , 4 , 26 ]. The specific osteogenic transcription factor CBFA1 was shown to be up-regulated in VSMCs undergoing phosphate-induced transdifferentiation into osteoblast-like cells. Further genes like ALP, SOX9 and MSX2, normally linked to calcification in osteoblasts, are up-regulated by elevated phosphate concentrations in in vitro models [ 14 , 27 ]. This could be confirmed in our model. The enhanced expression of ALP, CBFA1, SOX9 and MSX2 induced by high phosphorus, decreased with higher magnesium levels, clearly showing that the inhibitory effect of magnesium on BVSMC calcification was not only true for the deposition of calcium within the cells but also affected the osteogenic transdifferentiation. Very recently, it has been shown that nanocrystals are actively involved in the process of calcification and rather than high phosphate concentrations alone are a prerequisite for the induction of genes like BMP2 and osteocalcin [ 28 , 29 ]. Therefore, also the mentioned inhibitory influences of magnesium on crystal formation, described in the literature, may be in part responsible for the inhibitory effect of magnesium on transdifferentiation into osteoblast-like cells, observed in our experiments [ 25 , 30 , 31 ]. It seems unlikely, that the inhibitory effect of magnesium on calcification will also account in the bone, as it has been shown that physiological magnesium concentrations present in bone are not able to inhibit crystal growth [ 24 ]. Our study suggests that magnesium inhibits the pathological transdifferentiation process from VSMCs into osteoblast-like cells, which does not take place in the physiological process of calcification in the bone. However, detailed clinical investigations about the effect of magnesium on bone metabolism, specifically in renal failure patients, are rare and contradictory [ 32 , 33 ]. Properly designed in vitro as well as clinical studies to evaluate further the influence of magnesium on bone are still needed.
The remaining calcium deposition, observed in our experiments despite full inhibition of transdifferentiation in terms of marker genes, is in concordance with additional processes involved in calcification as apoptosis and matrix mineralization which were not fully inhibited in our settings [ 34 ]. MGP, a locally produced inhibitor of VC [ 35 ], which was significantly decreased during calcifying conditions in our experiments was not only restored to normal levels by magnesium but even strongly up-regulated when high magnesium concentrations were present. The inhibition is probably mediated by its capability to bind BMP2, which itself-via promotion of differentiation of VSMCs into osteochondrocyte-like cells-induces calcification [ 36 ]. Our finding that MGP levels significantly decreased when BVSMC calcification was induced by BGP, confirms the reported negative association of MGP with VC.
Recently, it has been reported that apoptosis is also involved in the process of VC and that elevated phosphate levels induce apoptosis in a type III inorganic phosphate transporter (Pit-1)-dependent manner [ 18 , 34 ]. In our model, magnesium was able to restore BGP-induced cell damage, which was reflected in reduced apoptosis and stimulation of cell proliferation. It has been shown that magnesium has in part calcimimetic actions in terms of stimulation of the calcium-sensing receptor (CaSR) [ 37 ]. Molostov et al. [ 38 ] could show that the stimulation of CaSR on VSMCs leads to enhanced cell proliferation and protects against apoptosis. This is consistent with earlier experiments with rat VSMCs, in which magnesium increased cell viability and proliferation, when added at concentrations of 2–4 mM [ 39 ]. Furthermore, it could be shown that the stimulation of CaSR with calcimimetics reduced mineral deposition in VSMCs [ 40 ]. This suggests that magnesium in our experimental model might inhibit apoptosis and enhance cell viability possibly at least in part via its calcimimetic action on the CaSR. As apoptosis was reported to initiate calcification [ 18 ] and inhibition of apoptosis reduced calcification and osteogenic transdifferentiation in experimental settings [ 18 , 26 , 34 ], this inhibitory effect of magnesium on apoptosis may be responsible for inhibiting the inappropriate osteogenic transdifferentiation of VSMCs induced by high phosphate levels and seem to be one part of the inhibitory mechanism of magnesium on BVSMC calcification observed in our studies.
It has been suggested that the effect of magnesium on VC and atherosclerosis may be mediated via competition with calcium for cell entry [ 41–43 ]. Calcium channels are the main entry mechanism for calcium into VSMCs as well as into matrix vesicles and play an important role in calcification of VSMCs [ 44 ]. Despite not being true for all blockers tested, inhibition of calcium channels have been shown to inhibit calcification [ 45 , 46 ]. Futhermore, there are several in vitro studies showing inhibitory action of elevated magnesium concentration on different types of calcium channels in VSMCs as well as other cell types of the cardiovascular system [ 47–49 ]. Our results confirm these findings in BVSMCs by showing that calcium entry seems to be inhibited at higher magnesium concentrations while simultaneously magnesium uptake is increased. Recently, it has been proposed that two members of the transient receptor potential (TRP) ion channel family, TRPM6 and TRPM7, play important roles in magnesium handling. In addition, these channels are permeable for calcium ions thereby regulating calcium homoeostasis and they are expressed on VSMCs [ 50–53 ]. Furthermore, crosstalk between members of the calcium-channel family of annexins, also involved in calcium entry in matrix vesicles, and Transient receptor potential cation channel subfamily M members (TRPMs) have been shown [ 54 ]. So magnesium may also via TRPM7 influence calcium uptake into the cells as well as into matrix vesicles and therefore seems to be involved in mediating the inhibitory effect of magnesium on calcification in our cell culture model. Very recently, indeed, a role of TRPM7 in mediating effect of magnesium on calcification could be confirmed [ 55 ]. Furthermore, it was shown that elevated calcium is able to up-regulate the phosphate transporter Pit-1, which in turn via an elevated phosphate uptake, leads by induction of CBFA1 to transdifferentiation of VSMCs [ 56 ]. Therefore, lowering the calcium load of the cells might, in addition to the mechanisms discussed above, may also be one aspect of inhibiting the transdifferentiation into osteoblast-like cells. Of course, these hypotheses are speculative and have to be confirmed in further studies to clarify the role of these channels in the inhibitory effect of magnesium on VC.
As a conclusion, higher magnesium levels prevented calcification and further progression of already established calcification in BVSMCs and further progression of already established calcification. In addition to the deposition of calcium, osteogenic transdifferentiation was inhibited by elevated magnesium concentrations. Increase in MGP levels, alterations in cell viability and apoptosis as well as inhibition of calcium entry into the cells may be partly involved in mediating this effect. However, the exact mechanism by which magnesium acts on this process needs to be further clarified. These results are in concordance with experimental animal studies as well as with clinical studies linking elevated magnesium levels to beneficial effects on VC and mortality [ 5–10 , 19–21 ]. Thus, magnesium seems to influence molecular processes associated with VC and it may be prudent to more carefully observe serum magnesium levels also in patients suffering from renal failure in order to detect a possible protective effect of this mineral against VC also in clinical situations. Controlled interventional studies are, however, needed to better understand its role.
E.O is a scientific advisor for Fresenius Medical Care, Turkey. J.P.-D. is a consultant to Fresenius Medical Care Deutschland GmbH. S.S. and M.P. are employees of Fresenius Medical Care Deutschland GmbH. All other authors have no conflicts of interest.
Funding. This study was supported by Fresenius Medical Care Deutschland GmbH, Germany.
Conflict of interest statement . None declared.
Comments