-
PDF
- Split View
-
Views
-
Cite
Cite
Shuiping Liu, Lu Chen, Yintao Zhang, Ying Zhou, Ying He, Zhen Chen, Shasha Qi, Jinyu Zhu, Xudong Chen, Hao Zhang, Yongchao Luo, Yunqing Qiu, Lin Tao, Feng Zhu, M6AREG: m6A-centered regulation of disease development and drug response, Nucleic Acids Research, Volume 51, Issue D1, 6 January 2023, Pages D1333–D1344, https://doi.org/10.1093/nar/gkac801
- Share Icon Share
Abstract
As the most prevalent internal modification in eukaryotic RNAs, N6-methyladenosine (m6A) has been discovered to play an essential role in cellular proliferation, metabolic homeostasis, embryonic development, etc. With the rapid accumulation of research interest in m6A, its crucial roles in the regulations of disease development and drug response are gaining more and more attention. Thus, a database offering such valuable data on m6A-centered regulation is greatly needed; however, no such database is as yet available. Herein, a new database named ‘M6AREG’ is developed to (i) systematically cover, for the first time, data on the effects of m6A-centered regulation on both disease development and drug response, (ii) explicitly describe the molecular mechanism underlying each type of regulation and (iii) fully reference the collected data by cross-linking to existing databases. Since the accumulated data are valuable for researchers in diverse disciplines (such as pathology and pathophysiology, clinical laboratory diagnostics, medicinal biochemistry and drug design), M6AREG is expected to have many implications for the future conduct of m6A-based regulation studies. It is currently accessible by all users at: https://idrblab.org/m6areg/

M6AREG systematically covers, for the first time, data on the effect of m6A-centered regulation on both disease development and drug response, explicitly describes the molecular mechanism underlying each type of regulation and fully references the collected data by cross-linking to existing databases.
INTRODUCTION
As the most prevalent internal modification in eukaryotic RNAs, N6-methyladenosine (m6A) is widely known to play essential roles in cellular proliferation, metabolic homeostasis, embryonic development, and so on (1–6). With the rapid increase in research interest in m6A, its crucial roles in the occurrence and progression of various diseases, including cardiovascular disease (7), influenza (8), gastroenteritis (9), liver fibrosis (10), diabetes (11) and cancer (12), are gaining more and more attention. Moreover, various types of m6A regulation are found to affect the responses of a drug in its corresponding disease via mediating the expression of target genes (13–15). In other words, the regulation of disease development and drug response by m6A has emerged as one of the most promising directions in recent years (7–19), and various studies have been conducted to uncover the molecular mechanism underlying regulation (mediated by the regulators of methyltransferase (writers), demethylase (erasers) and m6A-binding proteins (readers) (20–22).
The above-mentioned studies have accumulated valuable data for researchers in the diverse directions of (i) pathology and pathophysiology clarifying the essential role of m6A modifications in the occurrence/progression of disease (23–26), (ii) clinical laboratory diagnostics promoting the discovery of new therapeutic targets (27–31) and diagnostic/prognostic biomarkers (32–37) and (iii) medicinal biochemistry and drug design facilitating the discovery of m6A’s mechanisms in determining drug sensitivity and the design of new drug and drug combinations (38–42). Therefore, it is essential to have a database that provides data on the effect of m6A-centered regulation on disease development and drug response, together with the molecular mechanisms underlying each type of regulation.
So far, a variety of popular m6A-related databases have been developed (43–52). Some focus on describing the genome-wide landscape of RNA modification, variants and a variant's effect on post-transcriptional regulation [such as RMDisease (43), RMBase (44) and RMVar (45)]. Some others aim to show the m6A modification sites of RNAs based on sequencing experiments and transcriptome-wide prediction [such as m6A-Atlas (46), WHISTLE (47), m6Avar (48) and SRAMP (49)]. The remaining databases provide the validated or predicted targets of m6A regulators based on low-/high-throughput studies [such as MeT-DB (50) and M6A2Target (51)]. The majority of these existing databases have been frequently accessed and highly cited due to their considerable contributions to the needs of research communities (44–50). However, to date, there is no database available to provide data of m6A-centered regulation of disease development and drug response. Moreover, such a database is needed to systematically describe the molecular mechanisms underlying each type of regulation.
Herein, a database named ‘m6A-centered regulations of disease development and drug response (M6AREG)’ is therefore introduced. First, a systematic literature review on m6A’s regulation of disease development and drug response was conducted. The development of various diseases and the response data of drugs that were reported to be regulated by the corresponding m6A were systematically collected into the M6AREG database. Second, the molecular mechanisms underlying each type of regulation discussed above were then retrieved from the literature. The target RNAs regulated by the identified m6A regulators and their corresponding pathways were systematically collected. In particular, their regulation profiles (including m6A modification pattern, up-/down-regulation of expression and the cell processes regulated) were provided. Finally, because some xenobiotics were reported to interact with and mediate a particular m6A regulator (53–55), a number of xenobiotics regulating disease development and drug response through the mediation of certain m6A regulators were also provided. M6AREG data were also fully cross-linked to available databases. Since the data provided in M6AREG (https://idrblab.org/m6areg/) are valuable for diverse directions, it is expected to have great implications for the future conduct of m6A-based regulation studies.
FACTUAL CONTENT AND DATA RETRIEVAL
Data collection for m6A-centered regulation
Data on m6A-centered regulation of disease developments and drug responses were collected based on the following procedure. First, a large number of diseases, drugs and the m6A regulators were retrieved from ICD-11 (56), DrugBank (57), TTD (58), NCBI Gene (59), UniProt (60) and HGNC (61). Second, the data about m6A-centered regulations of disease development and drug response were collected by a comprehensive literature review in PubMed (59) using the keywords: ‘m6A + disease’, ‘m6A + drug’, ‘m6A Regulator Name + disease’, ‘m6A Regulator Name + drug’, ‘m6A + Disease Name’, ‘m6A + Drug Name’, ‘Regulator Name + Disease Name’, ‘Regulator Name + Drug Name’, etc. As a result, the development of 165 classes of diseases (such as diabetes, lung cancer and rheumatoid arthritis) and the response data of 70 drugs (such as cisplatin, sorafenib and tamoxifen) which were regulated by 31 regulators (such as FTO, METTL3 and ALKBH5) were collected in M6AREG. Third, all identified publications were reviewed and their detailed regulation information was manually collected, which included cell lines, animal models, targets and pathways. In addition, a total of 93 xenobiotics that regulated disease development and drug response via affecting m6A regulators were also collected.
The m6A regulators and their biological function
The m6A modification is an important RNA methylation without affecting the nucleotide sequence, which is achieved by m6A regulators (14). Such regulators include writers (e.g. METTL3, METTL14 and METTL16), erasers (e.g. FTO and ALKBH5) and readers (e.g. YTHDC1-2, IGF2BP1-3 and SND1), which have been reported to play key biological roles in many epigenetic directions, such as the mRNA life cycle and the cellular/developmental/disease process (62).
The biological function of writers, erasers and readers
As shown in Figure 1, m6A methyltransferase complexes are composed of the catalytic core METTL3, the RNA support element METTL14, the stabilizer WTAP and various adaptors [e.g. RBM1515B, ZC3H13, GBLL1 and VIRMA (63,64)]. m6A modifications are reversible and dynamic, since they can be removed by erasers, such as FTO and ALKBH5. Both writers and erasers regulating the level of a target’s m6A modification by methylation or demethylation play a critical role in the development of different classes of diseases (such as cancer, diabetes and rheumatoid arthritis) and drug response by targeting both coding and non-coding RNA (65–68). Taking the oscillatory shear stress-induced proatherogenic process as an example (shown in Figure 2), the expression of a writer METTL3 is up-regulated, which induces METTL3-dependent m6A hypermethylation of two targets (NLRP1 and KLF4), and then results in the elicitation of atherogenic responses, such as inflammation and cell adhesion (69). Another example of a writer is METTL14, which is reported to be remarkably down-regulated in trastuzumab-resistant cancer cells compared with their parental HER2-positive breast cancer cells. Some mechanistic studies reveal that it decreases the expression of FGFR4 by m6A modification (as shown in Figure 2), and FGFR4 could phosphorylate GSK-3β and stimulate β-catenin/TCF4 signaling to drive anti-HER2 resistance (70,71). Moreover, the first identified m6A demethylase (eraser), FTO, is found to play critical roles in leukemogenesis and drug response. It promotes leukemic oncogene-mediated cell transformation and leukemogenesis, and inhibits all-trans-retinoic acid-induced leukemia cell differentiation. Mechanistic studies show that FTO performs its oncogenic role by reducing the m6A level in mRNA transcripts of ASB2 and RARA, and then decreasing their expression (72).
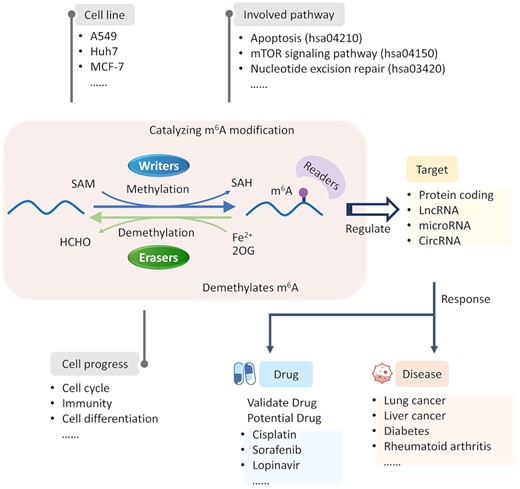
Function and corresponding mechanism of m6A writers and erasers in various cellular processes. The m6A modification is reversible and dynamic, which can not only be introduced into various target RNAs using the core methyltransferase complex (METTL3/METTL14/WTAP) and other adaptors, but can also be removed by the demethylases. These m6A-regulated RNAs which are involved in disease occurrence/progression and drug response were collected in M6AREG.
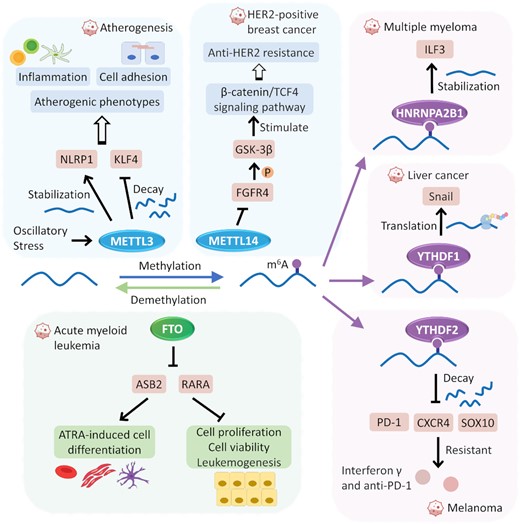
Representative examples describing the roles and corresponding molecular mechanisms of each m6A regulator in disease development and drug response. The m6A writer METTL3 can induce hypermethylation of NLRP1 and KLF4, and then up-regulate NLRP1 while downregulating KLF4 to elicit an atherogenic responses such as inflammation and cell adhesion. METTL14 decreases FGFR4 expression which could phosphorylate GSK-3β and stimulate β-catenin/TCF4 signaling to drive anti-HER2 resistance. FTO, as an eraser, promotes leukemogenesis and inhibits all-trans-retinoic acid-induced leukemia cell differentiation via reducing the m6A level in mRNA transcripts of ASB2 and RAR. The m6A readers, HNRNPA2B1, YTHDF1 and YTHDF2 individually promote multiple myeloma progression via stabilizing ILF3 mRNA, upregulating key transcription factors of the epithelial–mesenchymal transition in cancer via increasing translation of Snail, and sensitize melanoma cells to interferon γ and anti-PD-1 by causing the decay of PD-1, CXCR4 and SOX10.
Once RNA has been m6A methylated, the m6A writers (including YTHDFs, YTHDCs, IGF2BPs, eIF3, HNRNPs and FMRPs) could bind to the methylation site and play a specific role in RNA nuclear export, stabilization, splicing, translation and decay (73,74). As shown in Figure 3, due to such characteristics, great diversity in the m6A modification pattern and expression regulation (up-/down-) toward target RNAs was exhibited, which resulted in different regulation mechanisms in diseases and drug responses (75,76). As illustrated in Figure 2, the m6A reader HNRNPA2B1 is up-regulated in multiple myeloma and negatively correlated with a favorable prognosis. It promotes multiple myeloma progression (such as promoting cell proliferation and inhibiting cell apoptosis) by stabilizing the mRNA of ILF3 and AKT3 (77). Another example of a reader is YTHDF2, which causes the decay of PD-1, CXCR4 and SOX10, and then sensitizes melanoma cells to interferon γ and anti-PD-1 (76). As a subtype of YTHDF, YTHDF1 mediates m6A-increased translation of Snail that is a key transcription factor of the epithelial–mesenchymal transition, which has been considered as a negative prognostic factor for the overall survival rate of liver cancer patients (78).
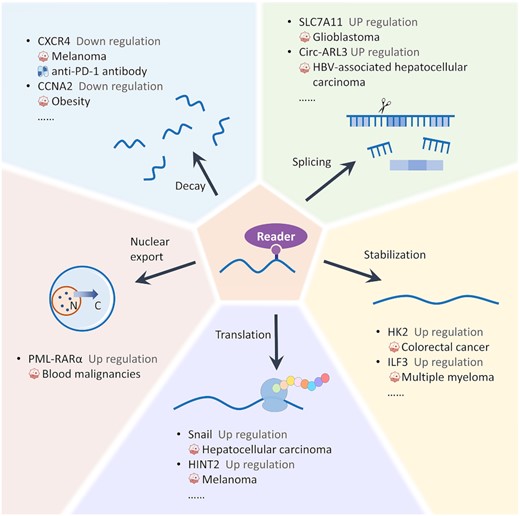
Different roles and mechanisms of m6A readers in various cell processes. Once the RNAs have been modified, different m6A writers will bind to the modification sites and perform diverse functions (RNA nuclear export, stabilization, translation, splicing and decay), which results in the up-/down-regulation of target genes. All RNAs that are involved in the regulation of disease occurrence/development and drug response of m6A writers were collected and explicitly described in M6AREG.
The description and statistics of regulators in M6AREG
For each m6A regulator shown in M6AREG, the detailed descriptions on its general information were provided online, which included regulator name, synonyms, gene name, sequence, protein family, biological function, regulator type, a full list of potential target genes of the regulator and other molecular information associated with the external links to NCBI Gene (59), UniProt (60), etc. The potential targets for a certain m6A regulator were discovered based on the transcriptomic studies collected from GEO (79), such as RIP seq, CLIP-seq, eCLIP-seq, PARCLIP-seq, iCLIP-seq and RNA-seq. For each regulator and its targets, detailed information such as gene name, experimental method, fold change, cell line and the external links to GEO (79), NCBI Gene (59), UniProt (60), etc. was also described. A diagram of the mechanism of each m6A regulator was also provided in the regulator page of the M6AREG database, which included representative information of its role in m6A modification, interactions with other regulators, target genes, etc. A full list of experimentally validated disease development and drug responses that were mediated by this regulator was provided, and detailed information, such as the m6A regulation pattern, in vivo/in vitro model and pathway, was also provided.
All in all, 14 m6A writers were reported to be involved in the development of 57 diseases by targeting 317 RNAs in 469 cell lines, and two m6A erasers were found to participate in the development of 49 diseases by targeting 164 RNAs in 335 cell line models. The studies on mechanisms showed that m6A writers and erasers regulated different cellular processes (such as the cell cycle and immunity) by regulating 89 and 64 signaling pathways (such as apoptosis, mTOR signaling and nucleotide excision repair), respectively. These regulators greatly affected the efficacies of a variety of drugs (such as cisplatin and sorafenib). Moreover, 15 m6A readers were identified to participate in the development of 41 diseases by targeting 217 RNAs in 391 cell lines, which were actively involved in 73 signaling pathways and significantly affected the responses of a variety of drugs.
For an m6A-based database, it is key to provide a quantitative description on the up-/down-regulation of the m6A target genes. The RNA-seq data were therefore incorporated into this newly developed database. First, a systematic review was conducted in GEO (79) to retrieve the regulator-related RNA-seq data by searching keywords such as ‘m6A Regulator Name’, ‘m6A Regulator Name + RNA sequencing’, ‘m6A Regulator Name + RNA seq’, and so on. The comparative data that studied the gene expression variations by knocking out, knocking down or overexpressing certain regulators were collected, which led to a total of 236 GEO datasets. Second, these newly collected datasets were carefully reviewed, and those datasets without a clearly stated data pre-processing method or of <2 samples for the control/case group were excluded from our analysis (80,81). As a result, a total of 144 datasets were identified for subsequent differential expression analyses. Third, the ‘raw read count’ and ‘TPM’ datasets were analyzed using the DESeq2 and limma packages, respectively (82–84), and the ‘RPKM’ and ‘FPKM’ datasets were transformed to ‘TPM’ and then analyzed using the limma package (85). The genes identified as significantly differentially expressed (fold change >1.5 and P-value <0.05) were considered as regulated by the studied regulator (86,87). Finally, the differential expression patterns of 33 889 target genes that were regulated by at least one regulator were collected, and the quantitative description of such a regulated expression patten was provided in the regulator page and target page of the M6AREG database (as illustrated in Figure 4). Detailed information such as experimental conditions, cell line and species was also described.
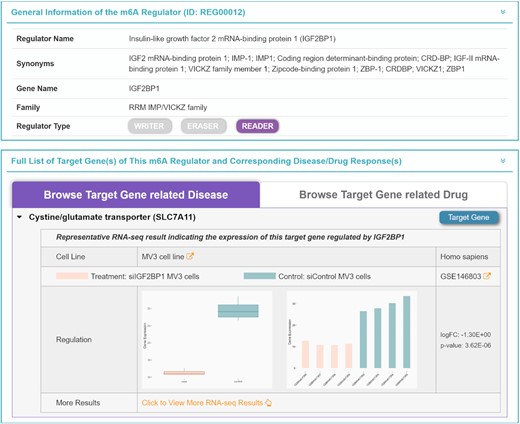
Quantitative description of the regulation pattern of target genes by m6A regulators. For a target gene and corresponding m6A regulator, the differential gene expression of the target gene in cells with treatment (knockout, knockdown and overexpression) and control cells was provided in the form of a bar chart and boxplot together with fold change and P-values, which were based on the RNA-seq data collected from the GEO. Detailed information such as cell line, species and experimental condition was also explicitly described. Pink, target gene expression of the cells with treatment; blue, target gene expression of the control cells.
The m6A-centered regulation of disease development
Dynamic m6A modification is reported to be involved in multiple biological processes by affecting gene expression of multiple levels including tissue development, self-renewal and differentiation of stem cells, circadian rhythm, heat shock, metabolism, metastasis and sex determination (15). With the advances in technology, increasing evidence showed that m6A modification plays crucial roles in the occurrence/progression of various disease (7–12). Recent literature indicated that m6A modification could be a new molecular tool to understand the occurrence and progression of diseases, which is regulated by many m6A regulators (24,88,89), and an increasing number of m6A regulators and their corresponding target gene were identified to have clinical implications in either diagnosis/prognosis or therapy for various diseases (24,90,91).
To obtain such m6A-centered regulation data, the disease-specific regulation data of both molecules and pathways were systematically reviewed and explicitly described in M6AREG. A total of 165 diseases were identified to be regulated by m6A modification according to the latest International Classification of Diseases (56). In particular, a total of 747 RNA molecules (e.g. mRNA, lncRNA, miRNA and cirRNA) and 78 pathways (physiological/pathological) that were regulated by 30 regulators (e.g. METTL3, FTO and YTHDF2) were collected. First, general information on each disease related to an m6A regulator and target genes (such as autophagy-related protein, cyclin-dependent kinase, microRNA and lncRNA) were provided. Second, as illustrated in Figure 5, the involved cellular processes of each target gene were described, which included cell growth, cell cycle, cell migration, cell proliferation and macrophage infiltration. Third, the regulation mechanisms of target genes in m6A-centered disease responses were also collected, which included the m6A regulators involved, up-/down-regulation of target genes and regulated pathways (e.g. PI3K–Akt signaling, TNF signaling and JAK–STAT signaling). Such data on m6A-centered regulation were essential for understanding of the mechanism underlying target genes in m6A-centered disease response. Fourth, besides the involved cellular processes and regulation mechanism data, in vitro and in vivo disease models were also illustrated in M6AREG. As described in Figure 5, a total of 200 cell lines of various diseases together with 23 model organisms were collected and provided in this newly developed database. Fifth, the comprehensive information for each target gene can be accessed by clicking the ‘Target Info’ button, which includes target name, synonyms, gene name, chromosomal location, functions and links to existing databases (59–61). Meanwhile, the comprehensive data of m6A regulators can be accessed by clicking the ‘Regulator Info’ buttons, and both the pathways altered by the m6A regulators and the corresponding in vitro models were directly linked to the KEGG (92) and Cellosaurus (93) databases.
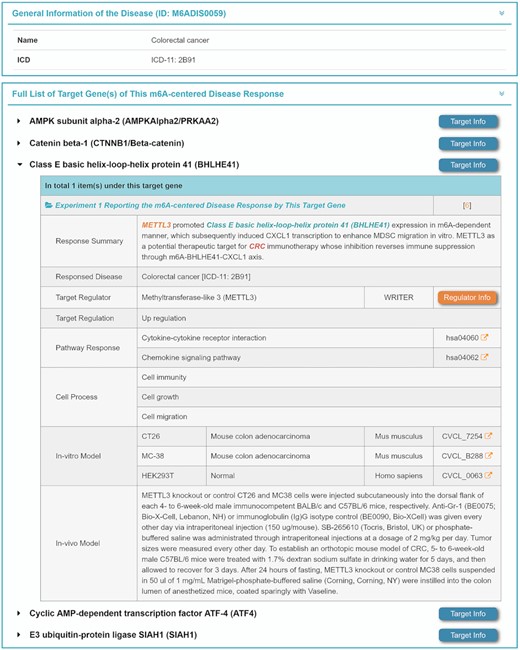
Regulation of target genes and pathways by m6A regulators in disease occurrence and progression. The detailed mechanism underlying m6A-centered disease responses is shown, which included a summary of disease responses, the m6A regulators involved, up-/down-regulation of target genes, regulated pathways, cellular processes and in vitro/in vivo disease models used. Extended descriptions can be accessed by clicking the corresponding differently colored buttons.
The m6A-centered regulation of drug responses
In the course of drug therapy, the clinical treatment effect on a patient can be seriously affected by the lack of alternative drugs and mutations in drug response-related genes (94). An increasing number of studies showed that the m6A modification plays a vital role in drug response of various diseases, which could be regulated by various m6A regulators and multiple target genes modulated by the same regulator (15,73,95). The m6A regulators altered drug responses by modulating drug–target interaction and drug-mediated cell death signaling. On the one hand, m6A modification interfered with drug efficacy that was mediated by a multidrug efflux transporter, drug-metabolizing enzyme and drug target (96–98). On the other hand, alterations of the m6A modification can inhibit drug-mediated cell death by inducing DNA damage and modulating its repair capacity (73). A number of studies indicated that m6A regulators or their corresponding target genes have potential as drug–effect biomarkers in disease (99,100), and diverse xenobiotics could bind to m6A regulators and modify their activities, resulting in regulation of disease progression or drug response (53,54).
As shown in Figure 6, a total of 70 drugs were identified to be regulated by 21 regulators, which were verified by in vitro/in vivo experiments. General information on each drug [such as drug name, synonym, clinic status, structure, formula, International Chemical Identifie (InChI) and InChIKey] is provided, and a list of m6A target genes associated with this drug response are described in M6AREG. For each m6A target gene, the summary of the target-regulated drug response, response disease, involved regulator, target regulation, pathway response, cellular process and in vitro/in vivo models are also described (illustrated in Figure 6). Moreover, the additional description of each target, m6A regulator and disease relevant to this drug can be accessed through clicking ‘Target Info’, ‘Regulator Info’ and ‘Disease Info’, respectively. All in all, a total of 146 experimentally verified targets, 36 pathways (physiological/pathological), 46 cellular processes, 269 cell lines and 75 in vivo animal models were found to be involved in identifying drug response alterations which were mediated by m6A modification, which are all provided in M6AREG. Based on those data provided on M6AREG’s drug page, the user can readily retrieve a list of m6A targets that were involved in the therapeutic effects of the corresponding drug described.
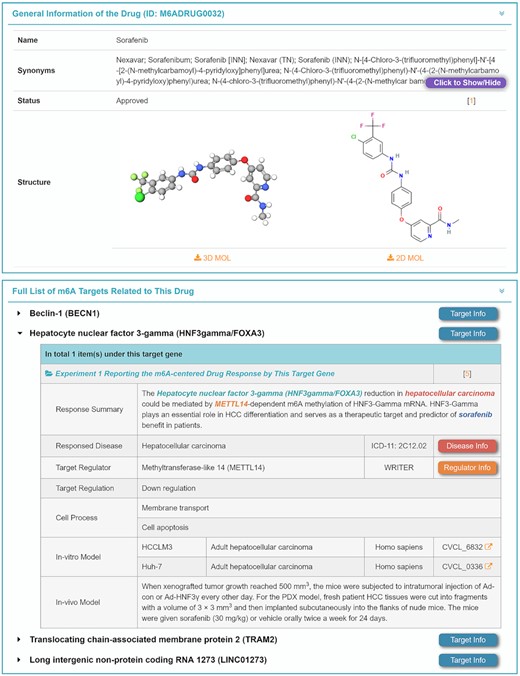
A typical page describing the m6A-centered regulations of drug responses. General information on the drug and its m6A targets are shown. For each target, the experimentally validated mechanisms are explicitly described. The regulation data are linked to their cell line or animal models, and extended information (such as each involved disease, target gene and regulated pathway) can also be retrieved by clicking the corresponding differently colored buttons.
Moreover, it is well known that some of the leading causes of drug efficiency include the mutation/altered expression of target proteins, deregulated drug transporters and altered drug metabolism, and the m6A regulators are reported to play a vital role in affecting the drug response by acting on the related molecules (73,101–105). Thus, the potential drug responses mediated by a specific m6A regulator are collected into M6AREG. First, three types of critical proteins (drug targets, transporters and metabolic enzymes) were identified from experimentally verified targets of m6A regulators. Second, the drugs targeting these three types of critical proteins were collected. As a result, a total of 4258 drugs including 593 approved drugs were found to interact with these types of targets that were regulated by m6A modification. All drugs can be accessed at the bottom of the regulator page, which provides the drug name, drug clinical status and mechanism leading to the drug response. Detailed information can be retrieved by clicking the button ‘Drug Info’.
M6AREG data standardization, access and retrieval
To facilitate users accessing and analyzing the M6AREG data, the collected raw data were carefully cleaned up and then systematically standardized. The standardizations included (i) all M6AREG diseases were standardized based on the latest version of the International Classification of Disease (56); (ii) all genes, RNAs, proteins, pathways and in vitro models in M6AREG were standardized and cross-linked to popular databases (such as NCBI Gene, HGNC, miRbase, Ensembl, UniProt, KEGG and Cellosaurus); and (iii) M6AREG drugs were cross-linked to a variety of well-established databases (such as TTD, PubChem and DrugBank). All data in the M6AREG database can be viewed, accessed and downloaded online without a login requirement by all users.
CONCLUSION
Epigenetic modifications (e.g. histone modifications, DNA methylation and RNA modifications) regulate gene expression without changing the DNA sequence and play pivotal roles in diseases and drug responses (106–108). RNA methylations are considered as a significant epigenetic modification, and those widely studied include N6-methyladenosine (m6A), 2′-O-methyladenosine (m6Am), N1-methyladenosine (m1A), 5-methylcytosine (m5C) and pseudouridine (ψ), whose dynamic changes can affect the fate of target RNAs and play critical roles in various bioprocesses. Similar to m6A modification, most of these methylation types are modulated by three types of regulators (writer, eraser amd reader), and play an important role in affecting the stability, translation, alternative splicing, nuclear export and secondary structure of RNAs. Moreover, different methylation types show diversity in preferred target RNAs and modification sites (109–111). Since the methylations account for >50% of 170 different types of naturally occurring modifications in RNAs (110), it is necessary to develop a system-wide method/tool to facilitate their efficient detection, as well as to explore the molecular mechanism of RNA methylation in target RNAs and their corresponding roles in disease development and drug response.
All in all, the M6AREG is unique in (i) providing the data of m6A-centered regulation on disease development and drug responses, (ii) explicitly describing the molecular mechanisms underlying each regulation and (iii) fully referencing the collected data by cross-linking to existing databases. Since the accumulated data in the M6AREG are valuable for researchers in diverse disciplines such as pathology and pathophysiology, clinical laboratory diagnostics, and medicinal biochemistry and drug design, this new database is expected to have great implications for the future conduct of m6A-based regulation studies. M6AREG is now freely accessible and fully downloadable by all users without any login requirement at: https://idrblab.org/m6areg/
FUNDING
Funded by the Natural Science Foundation of Zhejiang Province [LQ17H160009 and LR21H300001]; National Natural Science Foundation of China [81802371 and 81872798]; Leading Talent of ‘Ten Thousand Plan’ of the National High-Level Talents Special Support Plan of China; Fundamental Research Fund of Central University [2018QNA7023]; Key R&D Program of Zhejiang Province [2020C03010]; Chinese ‘Double Top-Class’ Universities [181201*194232101]; Start-up Grant of HZNU [4125C5021720421]; China Scholarship Council [201908330151]; Westlake Laboratory (Westlake Laboratory of Life Science and Biomedicine); Alibaba-Zhejiang University Joint Research Center of Future Digital Healthcare; Alibaba Cloud; and Information Tech Center of Zhejiang University.
Conflict of interest statement. None declared.
REFERENCES
Author notes
The authors wish it to be known that, in their opinion, the first four authors should be regarded as Joint First Authors.
Comments