-
PDF
- Split View
-
Views
-
Cite
Cite
Paola Y. Bertucci, A. Silvina Nacht, Mariano Alló, Luciana Rocha-Viegas, Cecilia Ballaré, Daniel Soronellas, Giancarlo Castellano, Roser Zaurin, Alberto R. Kornblihtt, Miguel Beato, Guillermo P. Vicent, Adali Pecci, Progesterone receptor induces bcl-x expression through intragenic binding sites favoring RNA polymerase II elongation, Nucleic Acids Research, Volume 41, Issue 12, 1 July 2013, Pages 6072–6086, https://doi.org/10.1093/nar/gkt327
- Share Icon Share
Abstract
Steroid receptors were classically described for regulating transcription by binding to target gene promoters. However, genome-wide studies reveal that steroid receptors-binding sites are mainly located at intragenic regions. To determine the role of these sites, we examined the effect of progestins on the transcription of the bcl-x gene, where only intragenic progesterone receptor-binding sites (PRbs) were identified. We found that in response to hormone treatment, the PR is recruited to these sites along with two histone acetyltransferases CREB-binding protein (CBP) and GCN5, leading to an increase in histone H3 and H4 acetylation and to the binding of the SWI/SNF complex. Concomitant, a more relaxed chromatin was detected along bcl-x gene mainly in the regions surrounding the intragenic PRbs. PR also mediated the recruitment of the positive elongation factor pTEFb, favoring RNA polymerase II (Pol II) elongation activity. Together these events promoted the re-distribution of the active Pol II toward the 3′-end of the gene and a decrease in the ratio between proximal and distal transcription. These results suggest a novel mechanism by which PR regulates gene expression by facilitating the proper passage of the polymerase along hormone-dependent genes.
INTRODUCTION
The regulation of specific patterns of gene expression is the main way by which cells respond to physiological and environmental signals. The first step in gene expression regulation is the control of the RNA polymerase II (Pol II)-dependent transcriptional response, which presents different points of exquisite control. In fact, most eukaryotic genomes are organized into a chromatin structure that generates high DNA package. This complex template acts as a barrier for Pol II transcription that provides several points for fine-tune regulation. The basic unit of chromatin is the nucleosome, composed of ∼147 bp of DNA wrapped in 1.67 left-handed superhelical turns around a histone octamer consisting of two copies each of the core histones H2A, H2B, H3 and H4 (1). Thus, the regulation of gene expression implies mechanisms that allow transcription factors (TFs) to gain access to chromatin packaged DNA (2). After DNA binding, activated TFs favor the recruitment of chromatin-modifying complexes that can either modify histone residues or use the energy of adenosine triphosphate (ATP) hydrolysis to alter the interaction between histones and DNA (3,4). Particularly, nuclear receptors (NRs) belong to a large and evolutionarily conserved superfamily of ligand-dependent TFs, which mediate the physiological effects of thyroid, retinoid and steroid hormones and play key roles in a wide variety of physiological and pathological processes (5,6). The classical mechanisms of NRs-dependent gene expression induction explains that after hormone interaction, the NR–ligand complex binds to specific hormone response elements (HREs) located in the promoter/enhancer region of target genes. On binding to DNA, NR–ligand complexes favor the formation of the pre-initiation complex (PIC) and transcription initiation (7) by provoking the recruitment of NRs co-activators as the histone acetyltransferases (HATs): CREB-binding protein (CBP), p300, SRC1/2/3 and the P300/CBP-associated factor (PCAF), among others (8). HAT enzymes reduce the net positive charge of the basic histones by acetylating specific histone residues and also create a dynamic platform for the recruitment of some ATP/dependent remodelers, as SWI/SNF and hNURF (9–11). Together, these remodelers open the chromatin structure by displacing or sliding the histone H2A/H2B dimers or the whole nucleosomes, exposing regulating sequences.
Genome-wide localization studies, performed with different NRs, have allowed the identification of cis-regulatory sites in previously unexplored regions of the genome, i.e far upstream sequences and 3′-end of target genes but surprisingly, mainly at intragenic regions (12–15). The role of these NRs-binding sites is still unknown and opens new questions regarding the way they operate and their functional relevance. In this sense, a new mechanism of action for NRs was recently proposed by Kininis et al. They found that after estradiol treatment, the estrogen receptor (ER) binds to target gene promoters and regulates the transition from initiation to elongation (16,17). This effect involves the ER-dependent recruitment of the positive elongation factor P-TEFb (conformed by multiple cyclin T subunits and the kinase Cdk9) to the promoter regions (18) and the consecuent displacement of negative elongation factors, leading to the transition of the paused Pol II to a productive elongation phase (16,19). The androgen receptor (AR) was also described as a putative regulator of transcription elongation by interacting with TFIIH and pTEFb at the promoter region of the prostate-specific antigen gene, enhancing transcription efficiency of its distal region on androgen induction (20). In this sense, both studies (16,20) contributed in understanding the mechanism of action of NRs by focusing on the regulation of transcription initiation and the transition to productive elongation. However, the role of steroid receptors (SRs) on the elongation process has not been fully addressed. In fact, these intragenic distal binding sites (15,21,22) would correspond to the largest part of the hormone-dependent gene regulatory network. Suggestively, different HATs and ATP-dependent chromatin remodelers associated to SRs effects on promoter regions have also been found regulating acetylation, eviction and methylation of nucleosomes in transcribed coding regions (23). Moreover, the modulation of intragenic chromatin conformation was associated with changes in Pol II elongation efficiency independently of transcription initiation regulation (20,24–26).
All together, these findings suggest that SRs may favor not only PIC formation and transcription initiation, as classically shown, and the transition from initiation to elongation step but also the Pol II elongation process itself by locally recruiting chromatin modifiers and elongation factors to specific intragenic sequences.
To evaluate this hypothesis, we analyzed progestin-dependent expression induction of the endogenous bcl-x gene in the human breast cancer cell line T47D. Bcl-x is an apoptosis regulator that codes for two different alternative splicing isoforms: Bcl-xL, which prevents apoptosis, and Bcl-xS, which induces cell death. The genomic structure of the human bcl-x gene contains three distinct promoters and only two coding exons separated each other by a long intron of ∼55 kb. This gene has been found to play a critical role in cell survival during tumor progression and chemotherapeutic resistance in mammary gland (27–29). In particular, bcl-x is induced by progestins in T47D (30) whose mechanism is still unknown.
In the present report, we discovered several intragenic progesterone receptor-binding sites (PRbs) in the bcl-x gene, which mediate hormone-dependent transcriptional induction in breast cancer cells. After hormone addition, the activated PR triggers the recruitment of three classes of co-activators to these intragenic binding sites: (i) the HATs -CBP and GCN5-, (ii) the ATP-dependent chromatin remodeler SWI/SNF and (ii) the P-TEFb elongation complex. This effect correlates with an increase in histone acetylation levels, a partial depletion in nucleosome occupancy, a re-distribution of the elongating Pol II torward the 3′-end of the gene, a decrease in the ratio between proximal and distal transcription and a change in the relative abundance between both alternative spliced bcl-x isoforms, favoring the accumulation of bcl-xL. In turn, we have found that the distribution of PRbs along the bcl-x gene would allow the loading of chromatin remodeling complexes, as well as elongation factors, both shown as key elements during gene induction by progestins. All together, these results suggest a novel mechanism, complementary to the classical model of NRs-dependent gene expression regulation, where PR would regulate the transcription levels of target genes by modulating the elongation process through intragenic binding sites.
MATERIALS AND METHODS
Plasmid constructions
The constructs p2.2hbcl-x-LUC; p3.5hbcl-x-LUC; p42hbcl-x-LUC and p58hbcl-x-LUC (expressing luciferase reporter gene under the control of SV40 minimal promoter plus hbcl-x fragment +2.2, +3.5, +42 and +58, respectively) were generated by subcloning the corresponding inserts into pGL3 reporter vector (Promega) into SacI/XhoI restriction sites.
Cell culture and transient transfection assays
T47D breast cancer cells were routinely grown in Roswell Park Memorial Institute medium (RPMI) 1640 medium supplemented with 10% Fetal Bovine Serum (FBS), 2 mM l-glutamine, 100 U/ml of penicillin and 100 µg/ml of streptomycin. For the experiments, cells were plated in RPMI medium without phenol red supplemented with 10% dextran-coated charcoal-treated FBS (DCC/FBS), and 48 h later medium was replaced by fresh medium without serum. After 24 h in serum-free conditions, cells were incubated with R5020 (10 nM) for different times at 37°C. For transient transfections, 4 × 105 T47D cells were co-transfected with 2 µg of each hbcl-x-LUC constructs and 2 µg of pCMV-LacZ using Lipofectamine 2000 (Invitrogen). After transfection, the cells were treated with R5020 for 36 h and then harvested in lysis buffer. Luciferase activity was measured with the Promega luciferase kit according to the manufacturer protocol and β-galactosidase as described before (31).
Chromatin immunoprecipitation assays
Chromatin immunoprecipitation (ChIP) assays were performed as previously described (32) using anti-PR (sc-7208), anti-CBP (sc-369), anti-GCN5 (sc-20698), anti-CDK9 (sc-484) and anti-total Pol II Clone 8WG16 (sc-899X), from Santa Cruz Biotechology. Anti-H2A and anti-H4 were supplied by Dr Stefan Dimitrov, Grenoble, France. Anti-H3 (ab1791), anti-H3K9ac (ab4441) and anti-H3K14ac (ab46984) from Abcam. Anti-H4K8ac (07-328), anti-H4K12ac (07-595), anti-H4panac (06-598) and anti-H3K18ac (07-354) from Upstate-Millipore. Anti-BAF57 (A300-810A) from Bethyl, and IgG (kch-504-250) from Diagenode, which was used as a negative control. An anti-total Pol II Clone 8WG16 (Covance) was used for Pol II ChIPs in 5,6-dichlorobenzimidazone-1-β-D-ribofuranoside (DRB)-treated assays. Specific ChIPs against Pol II-Ser2P were done as described previously (33) by using the specific antibody anti-Pol II-Ser2P Clone H5 (Covance-MMS-129R). Quantification of chromatin immunoprecipitation was performed by real-time polymerase chain reaction (PCR) using Roche Lightcycler (Roche) or DNA Engine Opticon™ System cycler (MJ Research). The fold enrichment of target sequence in the immunoprecipitated (IP) compared with input (Ref) fractions was calculated using the comparative Ct (the number of cycles required to reach a threshold concentration) method with the equation 2Ct(IP)–Ct(Ref). Each of these values were corrected by the human β-globin gene and referred as relative abundance over time zero. Primers sequences are available on request.
RNA interference experiments
siRNA GCN5 (sc-37946) and siRNA CBP (sc-29244) from Santa Cruz Biotechnology were transfected into the T47D cells using Lipofectamine 2000 (Invitrogen). After 48 h, the medium was replaced by fresh medium without serum. After one day in serum-free conditions, cells were incubated with R5020 (10 nM) or vehicle (ethanol) for different times at 37°C. The downregulation of CBP and GCN5 expression was determined by western blotting.
RNA extraction and reverse transcriptase–PCR
Total RNA was prepared and cDNA generated as previously described (34). Quantification of gene products was performed by real-time PCR. Each value calculated using the standard curve method was corrected by the human Glyceraldehyde 3-phosphate dehydrogenase (GAPDH) and expressed as relative RNA abundance over time zero. Primer sequences are available on request.
Coimmunoprecipitation assays
Cells were lysed in IP buffer (50 mM Tris–HCl, pH 7.4, 130 mM NaCl, 1 mM ethylenediaminetetraacetic acid, 1 mM ethylene glycol tetraacetic acid (EGTA), 5 mM MgCl, 1% Triton X-100 and 0.2 mg/ml of bovine serum albumin and protease inhibitors) and sonicated three times for 10 s at 20% output with a Branson 450 Sonifier. Cell extracts (1 mg protein) were incubated with protein A/G agarose beads (Oncogene) previously coupled with 3 µg of the corresponding antibodies or an unspecific control antibody. The immunoprecipitated proteins were eluted by boiling in sodium dodecyl sulfate—sample buffer. Inputs and IPs were analyzed by western blot using PR, CBP, GCN5 and CDK9 antibodies and the anti-cyclinT1 antibody (sc-8127X), from Santa Cruz Biotechology.
DNaseI hypersensitive assays
DNaseI hypersensitivesites (DHS) were identified as previously described (35). Digested DNA was used for deep sequencing using the Solexa Genome Analyzer.
Pol II elongation rate evaluation
T47D cells were treated with 100 µM DRB (Sigma) for 3 h. Then, cells were washed twice with phosphate-buffered saline, incubated in fresh medium in the presense of R5020 or vehicle for different times and total RNA was purifyed using the RNeasy kit (Qiagen). Reverse transcriptase (RT)–qPCR were performed as previously described with specific primers for proximal and distal regions of the bcl-x pre-mRNAs. Primer sequences are available on request.
Statistical analysis
Results were expressed as means ± standard error. Statistical analyses were performed with STATISTICA 6.0 (StatSoft, Inc.) and consisted of one- or two-way ANOVA followed by Tukey’s multiple comparisons tests. Differences were regarded as significant at P < 0.05. Before statistical analysis, data were tested for normality and homoscedasticity using Lilliefors and Bartlett’s tests, respectively. In some cases, log-transformed or root square-transformed data were used. In all cases, bars with different superscript letters are significantly different from each other.
RESULTS
Characterization of intragenic PR-binding sites on bcl-x gene
Genome-wide distribution of PRbs detected by ChIP-seq performed in human T47D cells treated during 60 min with the synthetic progestin R5020 (10−8 M) showed that 31% of PRbs are located in introns and 4% in exons, whereas the rest does not overlap with protein coding regions (22). One example of intragenic PRbs is found in the bcl-x gene (bcl2l1), one of the genes induced by progestins in T47D cells (36). To confirm that bcl-x is a direct target of progestins, we determined bcl-x mRNA levels on hormone addition in the presence or absence of RNA and protein synthesis inhibitors. Although induction of bcl-x transcripts was blocked in the presence of the transcription inhibitor α-amanitin (α-Am), cycloheximide (Cyclohex) did not influence bcl-x induction (Figure 1A), demonstrating that it is not dependent on protein synthesis. In agreement with a direct effect of progestins on bcl-x expression, we found four clusters of intragenic PRbs located at: +2.2, +3.5, +42 and +58 kb as counted from the more active bcl-x promoter (P1A) (Figure 1B). The first three sites are placed in the long intronic region, whereas the +58 kb site localizes at the end of the last exon. To validate the recruitment of PR to the bcl-x intragenic sites, we perfomed ChIP–qPCR experiments in T47D cells treated or not treated with R5020 for 60 min, focusing on the intragenic PR-binding sites but also on the promoters and enhancer regions located up to 3.5 kb upstream the transcriptional start site (TSS) (Figure 1B and C). We found that PR is recruited to the +2.2, +3.5, +42 and +58 sites, and that recruitment was higher close to the 3′-end of the gene. No recruitment of PR was observed either in the promoter regions (P1A, AB, P1B or P2) or in the enhancer region (R1–R5). ChIP-seq results have also shown no PR recruitment up to 10 kb upstream of the bcl-x promoter (22). Interestingly, a fraction of the PR recruited to bcl-x was phosphoryled at Ser-294, suggesting that it was activated on hormone addition (Supplementary Figure S1A) (34).
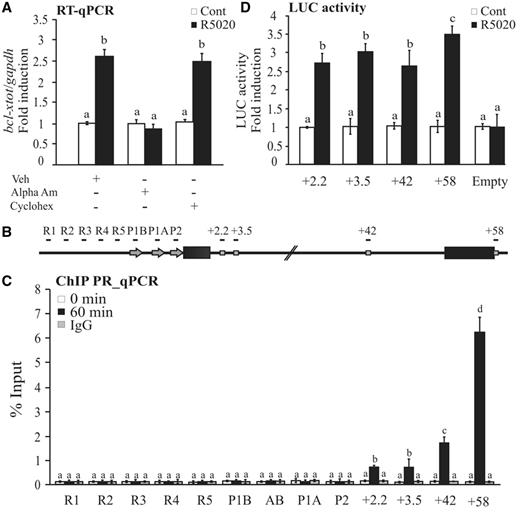
Progestin-dependent bcl-x expression. (A) T47D cells were treated or not treated with R5020 for 6 h in the presence or absence of α-amanitin or cycloheximide, and total RNA was prepared. cDNA was generated and used as template for real-time PCR with bcl-x-specific primers. The values were normalized with gapdh expression. The histograms show the mean ± standard deviation (SD) of three experiments performed in triplicate. Different superscript letters are significantly different from each other (P ≤ 0.05). (B) Scheme of the human bcl-x gene structure: R1–R5 sites indicate TSS upstream regions; P1A, P1B or P2 indicate promoter regions and +2.2, 3.4, +42 and +58 indicate PRbs. Immunoprecipitation with normal rabbit IgG (IgG) was used as control. (C) Recruitment of PR to the TSS upstream regions, promoter regions and PRbs. T47D cells were treated with R5020 for 60 min and subjected to ChIP assays with α-PR. The histograms show the mean ± SD of three experiments performed in triplicate. Different superscript letters are significantly different from each other (P ≤ 0.05). (D) T47D cells were transiently transfected with luciferase reporter constructs containing the sequence encompassing the different PRbs. After transfection, the cells were treated with R5020 for 36 h, and luciferase activity was measured. The values represent the mean and standard deviation from three experiments performed in duplicate. Different superscript letters are significantly different from each other (P ≤ 0.05).
To explore the kinetics of recruitment, we perfomed ChIP–qPCR experiments at different times after hormone addition. Initially, at 1 and 5 min, PR was recruited similarly to all sites, but after 30 min, a different pattern of binding was detected, with more PR recruited to the sites closer to the 3′-end of the gene (Supplementary Figure S1B). Thus, on progestin stimulation, activated PR is rapidly recruited to intragenic PRbs, but it remains bound to each of them for different times.
To assess the capacity to mediate hormone responsiveness of the intragenic PRbs, DNA fragments encompassing each of the PRbs at +2.2, +3.5, +42 and +58 were subcloned into luciferase reporter vector and transfected into T47D cells, which were then treated or not treated with R5020. Our results showed that the four intragenic elements significantly responded to the hormone induction, indicating that they contain sequences responsible for the hormone-dependent bcl-x expression behaving as bona fide HREs (Figure 1D).
Histone acetyltransferases CBP and GCN5 are needed for bcl-x induction and are recruited to its intragentic PRbs via association with PR
Acetylation of histones is often associated to gene activaton, and many NR co-activators exhibit HAT activities as, for example, CBP and GCN5 (8). Thus, we transfected T47D cells with specific siRNAs against these two HATs and then treated them with R5020. Bcl-x expression was measured by RT–qPCR, whereas the protein levels of CBP and GCN5 with either control or specific siRNAs were determined by western blotting (Figure 2A). Quantitative evaluation of CBP and GCN5 protein levels yielded a degree of depletion of 80 and 50%, respectively; under these conditions, hormone-dependent bcl-x induction was significantly compromised (Figure 2A, top). However, knockdown of PCAF, a different HAT that has been found also associated to NRs (37,38) did not show any effect on bcl-x expression regulation (unpublished observations). Accordingly, overexpression of the histone deacetylases HDAC1 and HDAC2 also prevented hormone-dependent bcl-x induction (Supplementary Figure S2). Moreover, ChIPs assays performed using specific antibodies for CBP and GCN5 showed that both HATs are recruited to the intragenic PRbs on 60 min of hormone treatment to an extent correlated to the previously observed binding of PR (compare Figures 1C and 2B). Together these results suggest that CBP and GCN5 histone acetylases are involved as direct mediators of the PR-dependent bcl-x transcription regulation.
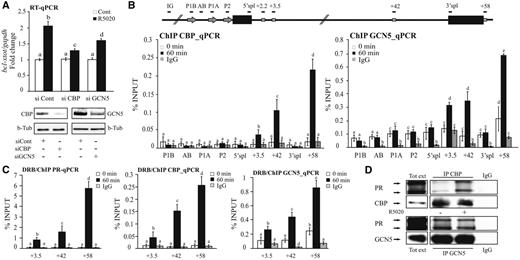
Role of CBP and GCN5 on progestin-dependent bcl-x induction. (A) T47D cells treated or not treated with R5020 were transfected with control, CBP or GCN5 siRNAs, then total RNA and proteins were extracted. The relative levels of bcl-x expression were determined by RT–PCR and normalized to gapdh expression. The degree of depletion of CBP and GCN5 was monitored by western blot using specific antibodies. For each condition, β-tubulin was used as loading control. The histograms show the mean ± SD of three experiments performed in duplicate. The different superscript letters are significantly different from each other (P ≤ 0.05). (B) T47D cells were treated with R5020 for 60 min and subjected to ChIP assays with α-CBP- and α-GCN5-specific antibodies. Recruitment of CBP (left) and GCN5 (right) to PRbs, promoter regions and TSS upstream regions of the bcl-x gene is shown. Immunoprecipitation with normal rabbit IgG (IgG) was used as control. The graphics show the mean ± SD of three experiments performed in duplicate. The different superscript letters are significantly different from each other (P ≤ 0.05). (C) Effect of transcription elongation on PR (left), CBP (middle) and GCN5 (right) recruitment to the +3.5, +42 and +58 PRbs. T47D cells pre-incubated with 50 µM DRB were treated with R5020 for 60 min and subjected to ChIP assays with α-PR, α-CBP and α-GCN5 antibodies. Immunoprecipitation with normal rabbit IgG (IgG) was used as control. The graphics show the mean ± SD of three experiments performed in duplicate. The different superscript letters are significantly different from each other (P ≤ 0.05). (D) T47D cells treated or not treated with R5020 for 1 h were lysed and immunoprecipitated with α-CBP- and α-GCN5-specific antibodies or with normal rabbit IgG (IgG). The immunoprecipitates were analyzed by western blotting with α-PR, α-CBP and α-GCN5 as indicated.
As HATs may also be recruited to the chromatin by the elongating Pol II (39–42), we re-analyzed the recruitment of CBP and GCN5 in T47D but not in cells pre-treated with the elongation inhibitor DRB (43), which inhibits the activity of the kinase subunit CDK9 of the P-TEFb complex (44). Bcl-x induction after R5020 addition was blocked by DRB in a dose-dependent manner (Supplementary Figure S3). Thus, we performed ChIP assays to analyze the recruitment of PR, CBP and GCN5 in cells pretreated with 50 μM of DRB for 1 h and then incubated with R5020 in the presence of the inhibitor. Recruitment of PR, CBP and GCN5 to the intragenic PRbs was not affected by the presence of DRB (Figure 2C), suggesting that recruitment of PR and the two HATs is independent of RNA Pol II elongation. Moreover, co-IP experiments performed with protein extracts from T47D cells treated or not treated with R5020, showed that both HATs interact with PR, and that the association is hormone dependent for CBP but not for GCN5 (Figure 2D). Thus, likely the HATs are recruited to the intragenics PRbs of bcl-x via an interaction with PR.
Changes in chromatin accessibility in the distal intragenic PRbs
Several studies have shown that nucleosomes constitute a strong barrier for elongating polymerases. Therefore, we next wondered whether changes in the chromatin structure could be involved on PR-dependent regulation of bcl-x expression. To assess this question, we performed ChIP experiments using specific antibodies recognizing histones H2A, H3 and H4. Our results show a significant decrease (between 20 and 40%) on histone H2A, H3 and H4 content after hormone addition, at the intragenic sites: +3.5, +42 and +58 (Figure 3A). The degree of histones H3 and H4 removal from the intragenic PRbs was similar to the displacement of H2A/H2B dimers (Figure 3A, compare left with middle and right), suggesting that whole nucleosomes loose interaction with DNA rather than just the dimers of H2A–H2B. The presence of DRB did not block histone displacement, indicating that this process seems to be PR dependent rather than a consequence of histone eviction associated to the elongating activity of the Pol II (Figure 3B). Analysis of histone levels along the bcl-x gene before and after hormone addition showed that hormone-dependent chromatin remodeling was enriched in the PRbs surrounding regions (Figure 3C), suggesting that the strong binding of activated PR triggers these changes in nucleosome occupancy. In this sense, histone displacement was not observed at the promoter P1A (Figure 3A and C). Moreover, the analysis of read profiles obtained from ChIP-seq of H4 showed that histone displacement is most clearly seen toward the 3′-end of the gene (Figure 3D) correlating with the enhanced binding of the receptor, as well as GCN5 and CBP recruitment (Figures 1C and 2B).
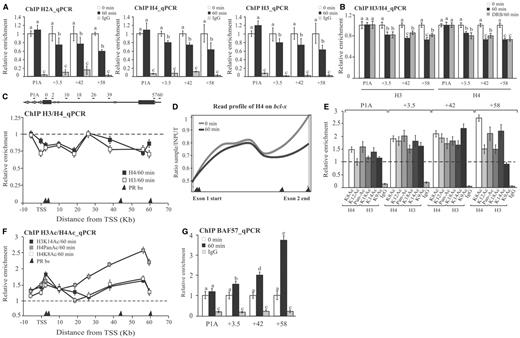
Histone acetylation and chromatin remodeling. (A) T47D cells were treated with R5020 for 60 min and subjected to ChIP assays with α-H2A, H4 and H3 antibodies. The precipitated DNA fragments were subjected to PCR analysis to test for the presence of sequences corresponding to +3.5, +42 and +58 PRbs and the promoter P1A. Immunoprecipitation with normal rabbit IgG (IgG) was used as control. The histograms show the mean ± SD of three experiments. Bars with different superscript letters are significantly different from each other (P ≤ 0.05). (B) Effect of transcription elongation on histone H3 and H4 levels at P1A and the +3.5, +42 and +58 PRbs. T47D cells pre-incubated with 50 µM DRB were treated with R5020 for 60 min and subjected to ChIP assays with H4 and H3 antibodies. Immunoprecipitation with normal rabbit IgG (IgG) was used as control. The graphics show the mean ± SD of three experiments performed in duplicate. The different superscript letters are significantly different from each other (P ≤ 0.05). (C) T47D cells were treated with R5020 for 60 min and subjected to ChIP assays with H4 and H3 antibodies. The precipitated DNA fragments were subjected to PCR analysis along bcl-x. Primers positions are indicated in the scheme above the figure. The histograms show the mean ± SD of three experiments. The dashed line indicates the relative levels of histones H3 and H4 in the absence of hormone. Black triangles at the absice indicates PRbs location. (D) T47D cells were treated with R5020 for 60 min and subjected to ChIP-seq analysis using α-H4 and H3 antibodies (22). The read profiles obtained in the presence and in the absence of hormone in the bcl-x gene, are shown. Black triangles at the absice indicates PRbs location. (E) T47D cells were treated with R5020 for 60 min and subjected to ChIP assays with antibodies against specific acetylated marks: H4K8ac, H4K12ac, pan-acetylated H4 (Pan-Ac), H3K18ac, H3K14ac and H3K9ac. The precipitated DNA fragments were subjected to PCR analysis to test for the presence of sequences corresponding to +3.5, +42 and +58 PRbs and the promoter P1A. The histograms show the mean ± SD of three experiments performed in duplicate. (F) T47D cells were treated with R5020 for 60 min and subjected to ChIP assays with H3K14ac; pan-acetylated H4 (Pan-Ac) and H4K8ac antibodies. The precipitated DNA fragments were subjected to PCR analysis along bcl-x. Primers positions are indicated in C. The histograms show the mean ± SD of two experiments. The dashed line indicates the basal levels of the different modifications obtained in the absence of hormone. Black triangles at the absice indicates PRbs location. (G) T47D cells were treated with R5020 for 60 min and subjected to ChIP assays with α-BAF57 (a member of the SWI/SNF complex). Recruitment of BAF57 to the +3.5, +42 and +58 PRbs and the promoter P1A was evaluated by RT–PCR. Immunoprecipitation with normal rabbit IgG (IgG) was used as control. The histograms show the mean ± SD of three experiments performed in duplicate. Bars with different superscript letters are significantly different from each other (P ≤ 0.05).
Next, we investigated whether recruitment of CBP and GCN5 to the intragenic PRbs correlates with changes in histone acetylation. We performed ChIP assays using specific antibodies against either pan-acetylated H4 or individual acetylated lysines: K8 and K12 of H4 and K18, K14 and K9 of H3. The results were normalized to the levels of histones H3 and H4 in each case (Figure 3E) and show that after hormone induction, the acetylation of histones H3 and H4 is increased between 1.5- and 2.7-fold at the +3.5, +42 and +58 intragenic PRbs, whereas only a moderate increase is observed at the promoter region P1A (Figure 3E). Both CBP and GCN5 participate in the acetylation at intragenic PRbs, as we observed increased levels of H3K9ac and H4K8ac that are exclusively placed by GCN5 and CBP, respectively (Figure 3E). Moreover, we detected a moderate increase in H3K14ac that could be catalyzed by another HAT (9). We also observed increased levels of H4K12ac (Figure 3E), a modification that it cannot be produced by neither by GCN5 nor CBP (45), indicating that other HATs, as hKAT1/(HAT1), hKAT5/(TIP60) or hKAT7/HBO1/MYST2 could also be involved in the histone acetylation process occurring in the bcl-x intragenic PRbs. Furthermore, according to ChIP assays performed against H3K14ac, H4panac and H4K8ac analyzed along bcl-x, histone acetylation also seems to be mainly increased at the 3′-region of the gene (Figure 3F).
As shown previously, some chromatin remodelers, such as SWI/SNF (BAF and P/BAF complexes) and NURF, contain subunits that recognized specific histone acetylation marks and, therefore, cooperate in their binding to target chromatin (9,46). ChIP experiments showed a significant hormone-dependent recruitment of BAF57, a subunit of the BAF complex to the +3.5, +42 and +58 PRbs, to a level correlated to the extent of PR and HAT recruitment (Figure 3G).
As the recruitment of the SWI/SNF chromatin remodeling enzymes is one of the mechanisms likely to disrupt chromatin at many DHS (47–49), we next asked whether specific binding of PR along with chromatin remodelers to the intragenic PRbs changes the DHS pattern after hormone induction. In this sense, we searched for DHS at the bcl-x gene by analyzing a DNase I hypersensitive sites sequencing (DNase I-seq) assay performed in T47D cells treated or not treated for 60 min with R5020 (22). Results showed an increase in DHS at the intragenic PRbs at +42 and +58 on hormone addition (Figure 4, top and bottom). The regions over the intragenic PRbs at +2.5 and +3.5 were hypersensitive to DNase I before hormone treatment and became less sensitive to nucleases in the presence of R5020 (Figure 4). Thus, the promoter distal PRbs of bcl-x showed a clear chromatin remodeling after hormone treatment in correlation with their higher binding of PR, HATs and BAF. This different behavior of PRbs located closer to the 3′ of the gene compared with those sited more proximal to promoter regions together with the nucleosomes displacement detected earlier in the text suggests that the more accessible chromatin conformation induced by PR at these sites would favor the passage of the elongating RNA Pol II along bcl-x, mainly at the 3′-end region.

Increase in DHS signal at the intragenic PRbs after hormone induction. T47D cells were treated with R5020 for 60 min and subjected to DNAse-seq analysis (35). Top and middle: the profiles of DHS signals obtained in the presence and in the absence of R5020, as well as the PRbs obtained by ChIP-seq (22) in the bcl-x gene are shown. Bottom: Quantification of the DHS reads associated with the +2.5, +3.5, +42 and +58 PRbs. The window used in each PRbs was ±50 bp.
To test this hypothesis, we then directly monitored the hormone-dependent Pol II distribution along bcl-x gene. Analysis of the read profile obtained from a ChIP-seq showed that the Pol II is stalled in the 5′-end of the gene before hormone addition (Figure 5A, top). Hormone induction promoted accumulation of Pol II toward the 3′-end of the gene [(22) and Figure 5A, top]. Box plots representing the density of Pol II at 5′ (exon1) and 3′ (exon2) bcl-x regions confirmed the statistical significance of this finding (Figure 5A, bottom). Similar results were obtained by using quantitative ChIP–qPCR. The magnitude of Pol II increase was ∼2-fold at the 3′-region of the gene (Figure 5B). As expected, the increase in Pol II levels was abolished by the inhibitor DRB (Figure 5C), ruling out the possibility of an internal Pol II recruitment as a consequence of the activated PR binding to the intragenic PRbs. Thus, the binding of the activated PR would affect the internal Pol II distribution toward the 3′-end rather than the recruitment of new Pol II molecules to the promoter region.
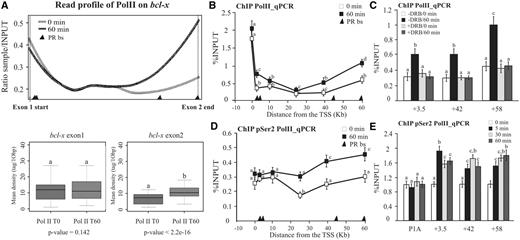
Occupancy of intragenic PRbs after hormone induction increased RNA pol II loading to the 3′-end of the bcl-x gene. (A) T47D cells were treated with R5020 for 60 min and subjected to ChIP-seq analysis using α-RNA pol II antibody (22). Top: the read profiles obtained in the presence and in the absence of hormone in the bcl-x gene are shown. Black triangles at the absice indicate PRbs location. Bottom: after hormone induction, increased loading of RNA pol II polymerase is detected at the 3′-end of the bcl-x gene. Quantification of the ChIP-seq reads associated with the bcl-x exon 1 (right) and 2 (left) in the presence and in the absence of hormone is shown. The P-value is indicated at the bottom of each box plot. (B) T47D cells were treated with R5020 for 60 min and subjected to ChIP assays with α-RNA Pol II antibody. The precipitated DNA fragments were subjected to PCR analysis along bcl-x. The histograms show the mean ± SD of three experiments. Different superscript letters are significantly different from each other (P ≤ 0.05). Black triangles at the absice indicates PRbs location. (C) Effect of transcription elongation on Pol II distribution at the +3.5, +42 and +58 PRbs. T47D cells pre-incubated with 50 µM DRB were treated with R5020 for 60 min and subjected to ChIP assays with α-RNA Pol II antibody. Immunoprecipitation with normal rabbit IgG (IgG) was used as control. The graphics show the mean ± SD of three experiments performed in duplicate. The different superscript letters are significantly different from each other (P ≤ 0.05). (D) T47D cells were treated with R5020 for 60 min and subjected to ChIP assays with α-RNA Pol II-Ser2P antibody. The precipitated DNA fragments were subjected to PCR analysis along bcl-x. The histograms show the mean ± SD of three experiments. Different superscript letters are significantly different from each other (P ≤ 0.05). Black triangles at the absice indicate PRbs location. (E) Time course of RNA Pol II-Ser2P recruitment: T47D cells were treated with R5020 for 0, 5, 30 and 60 min and subjected to ChIP assays using α-RNA Pol II-Ser2P PR antibody. The precipitated DNA fragments were subjected to PCR analysis to test for the presence of sequences corresponding to P1A and the three PRbs: +3.5, +42 and +58 PRbs. The histograms show the mean ± SD of three experiments performed in duplicate. Different superscript letters are significantly different from each other (P ≤ 0.05).
Taking into account that the phosphorylation of the carboxy-terminal domain (CTD) of the largest Pol II subunit in the Ser2 residues is a good marker of active elongation, we performed ChIP experiments against the Pol II-Ser2P to cofirm that hormone treatment affects the elongating polymerase. Figure 5D shows that on progestin addition, the levels of the Pol II-Ser2P increased toward the 3′-end of the gene. Moreover, a time-course curve of the Pol II-Ser2P at P1A and the +3.5, +42 and +58 PRbs showed a similar profile to the time course previously observed for the PR recruitment to these internal PRbs (compare Figure 5E with Supplementary Figure S1B). These results highlight the role of progestins affecting the elongation rate.
P-TEFb is recruited to the intragenic PRbs on hormone addition
As phosphorylation of Pol II-Ser2 is carried out by the positive elongation factor P-TEFb, which is known to be recruited by the SRs to target genes (16,19,20), and given that bcl-x hormone-dependent induction is affected by the inhibition of the P-TEFb subunit CDK9 (Supplementary Figure S3), we then tested whether this elongation factor is recruited to the bcl-x PRbs. We performed ChIP assays with a specific antibody recognizing CDK9 in T47D cells treated with R5020 for 60 min. Consistently, we found hormone-dependent recruitment of the CDK9 kinase to the intragenic PRbs with a similar pattern to that observed previously for PR and the two HATs (Figure 6A). Moreover, CDK9 was recruited even when its activity was impaired by the presence of DRB (Figure 6B), supporting a PR-mediated recruitment. Indeed, the P-TEFb subunits, CDK9 and cyclinT1, co-precipitate with PR in response to the hormone in this cell line (Figure 6C). As P-TEFb favors the elongation process by phosphorylation of Pol II Ser2 and by displacing negative elongation factors, intragenic PR-mediated recruitment of this factor would favor progesterone enhancement of transcription elongation.
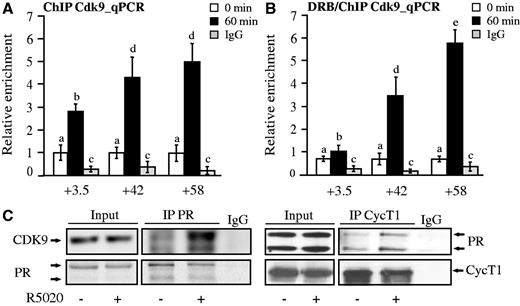
The elongation complex pTEFb is recruited along with PR to the bcl-x intragenic PRbs. (A) T47D cells were treated with R5020 for 60 min and subjected to ChIP assays with α-Cdk9 antibody. The precipitated DNA fragments were subjected to PCR analysis to test for the presence of sequences corresponding to +3.5, +42 and +58 PRbs. Immunoprecipitation with normal rabbit IgG (IgG) was used as control. The histograms show the mean ± SD of three experiments. Different superscript letters are significantly different from each other (P ≤ 0.05). (B) T47D cells pre-incubated with 50 µM DRB were treated with R5020 for 60 min and subjected to ChIP assays with α-Cdk9 antibody. The precipitated DNA fragments were subjected to PCR analysis to test for the presence of sequences corresponding to +3.5, +42 and +58 PRbs. Immunoprecipitation with normal rabbit IgG (IgG) was used as control. The histograms show the mean ± SD of three experiments. Different superscript letters are significantly different from each other (P ≤ 0.05). (C) T47D cells treated or not treated with R5020 for 1 h were lysed and immunoprecipitated with α-PR or with normal rabbit IgG (IgG). The immunoprecipitates were analyzed by western blotting with α-Cdk9, α-CyclinT1 and α-PR as indicated. Blots correspond to one representative experiment (n = 3).
Involvement of intragenic PRbs on the transcription elongation and alternative splicing of bcl-x
Changes in the Pol II rate or processivity on endogenous genes in response to a particular stimulus is technically difficult to determine. However, there is a way to asses Pol II processivity by evaluating with specific primers the relative abundance of a pre-mRNAs in a proximal versus a distal region by RT–qPCR, assuming that most pre-mRNAs are co-transcriptional intermediates (50) (Figure 7A). We used this approach to analyze Pol II processivity on bcl-x in T47D cells treated with R5020 for 15, 30 and 60 min (Figure 7B, left). Results show that the proximal/distal ratio for bcl-x gene decreases after hormone addition, indicating a hormone effect on Pol II elongation activity. The observed change in the proximal/distal ratio was detected already after 15 min of hormone treatment and reached the maximum after 30 min.
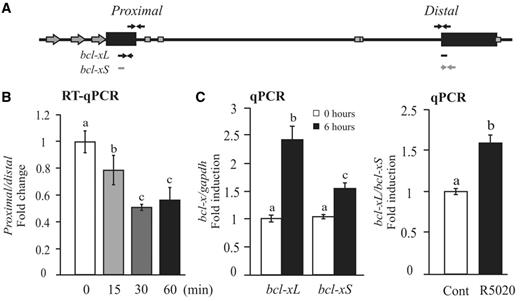
PR-dependent Pol II processivity and bcl-x splicing. (A) Scheme of the human bcl-x gene structure: arrows indicate the sequences that hibridize with the different primers used in these assays. (B) T47D cells were treated or not treated with R5020 for 15, 30 and 60 min, and the proximal/distal ratio of mRNA bcl-x gene was evaluated. The histograms show the mean ± SD of three experiments performed in duplicate. The different superscript letters are significantly different from each other (P ≤ 0.05). (C) T47D cells were treated or not treated with R5020 for 6 h, and total RNA was prepared. cDNA was generated and used as template for real-time PCR with bcl-xL and bcl-xS-specific primers (left). Right: the ratio between bcl-xL and bcl-xS was calcultated. The values were normalized with gapdh expression. The histograms show the mean ± SD of three experiments performed in triplicate. Different superscript letters are significantly different from each other (P ≤ 0.05).
Taking into account that changes in Pol II processivity influence RNA processing (24,25), and that bcl-x alternative splicing is affected by changes in Pol II elongation efficiency (51), we analyzed the hormone-dependent regulation of bcl-x alternative spliced isoforms. The results showed that both bcl-xL and bcl-xS transcript levels significantly increased on R5020 addition (Figure 7B, middle); however, the relative abundance between each other favors bcl-xL accumulation (right). These results add more evidences supporting the hypothesis of a progestin-dependent regulation of Pol II elongation.
A progesterone effect on Pol II elongation activity was also observed in the endoplasmic reticulum to nucleus signaling factor 1 (ern1) gene (Supplementary Figure S4D). The ern1 gene like bcl-x contains intragenic PRbs and is also upregulated in the presence of hormone (Supplementary Figure S4A). On the other hand, other induced hormone-dependent genes, such as transforming growth factor α (tgfα) (Supplementary Figure S4B) and the insulin-like growth factor 1 receptor (igf1r) (Supplementary Figure S4C), show a proximal/distal pre-mRNA ratio that either does not change or even increases after hormone, respectively (Supplementary Figure S4E and F, respectively).
The recent progress in DNA sequencing technology allowed us to increase the coverage 10-fold and to identify 28.474 intragenic PRbs on 60 min of hormone treatment (P < 10−5). Combining the results of the ChIP-seq of PR with the gene expression data obtained by RNA-seq, we found that the internal PRbs are significantly enriched in upregulated genes compared with genes that do not respond to hormone (3.2 versus 1 PRbs/gene, respectively) (Supplementary Table S1). Interestingly, intragenic PRbs also showed a preference for downregulated genes with 2 PRbs/gene. Thus, we conclude that internal PRbs are accumulated in hormone-regulated genes, suggesting a more general mechanism than originally expected.
DISCUSSION
The study of how NRs control transcription has been largely focused on the regulation of PIC formation and transcription initiation. However, the putative role of NRs on the regulation of subsequent transcriptional steps has recently been enabled by using new generation sequencing techniques (16). Particularly, ChIP-seq analysis showed that NRs bind to sites located outside and sometimes at great distances of promoters, mainly at intragenic regions (14,16,52). However, the functional relevance and the mechanism of action of these internal NRbs have not been conclusively clarified.
Induction of bcl-x transcription correlates with the binding of PR to intragenic sites and the recruitment of active CBP and GCN5
The classical model of SRs-mediated gene activation proposes that Pol II recruitment and PIC formation to SRs target promoters is favored by an open chromatin structure, as demonstrated by high sensitivity to DNase cleavage observed on hormone treatment (53,54). It is assumed that this localized relaxed chromatin conformation is generated by SR-mediated recruitment of chromatin-modifying complexes that are common SR-co-factors during gene activation or induction (8,55–58).
In this work, we found that progestin-dependent bcl-x transcriptional induction in the breast cancer cell line T47D is controlled by binding of PR to several intragenic binding sites instead of the classicaly described promoter PRbs. In response to hormone treatment, the activated PR recruits CBP and GCN5 HATs to these sites, as was already demostrated at target promoters. Interestingly, the recruitment of these CBP and GCN5 is independent of transcription elongation, as it cannot be inhibited by DRB. CBP and GCN5 binding was acompanied by a local increase in histones H3 and H4 acetylation and was necessary for the hormone induction of bcl-x, as interfering either CBP or GCN5 expression precluded activation of the gene. These results, together with the co-immunoprecipitation of PR with both CBP and GCN5, support the hypothesis of a PR-dependent recruitment of chromatin modifiers to these intragenic regions. In fact, simultaneously with the recruitment of these HATs, accumulation of the ATP-dependent chromatin remodeler SWI/SNF and reduction in nuclesome occupancy in the bcl-x intragenic PRbs were observed, suggesting a change in the chromatin structure toward a more open state. Histone displacement was not exclusively restricted to the internal PRbs but also to surrounding regions. This effect correlates with the presence of other PRbs with lower levels of PR recruited than those observed at +2.5, +3.5, +42 and +58 described here (22). These weaker PRbs are mainly localized in the 3′-region of bcl-x intron and may contribute to the PR-dependent effect on Pol II elongation mainly at this region of the gene. PR-dependent remodeling of chromatin organization was previously demonstrated at promoter regions as a complex and necessary prerequisite for hormonal induction in a diversity of biological models (59–62). For example, two consecutive cycles of chromatin remodeling involving activated protein kinases, HATs and ATP-dependent chromatin modifiers have been described on hormonal induction of the mouse mammary tumor virus (MMTV) promoter (63).
Supporting our observations, local recruitment of chromatin remodelers and a decrease in histone density were found at four AR intronic sites located ≥90 kb downstream from the TSS of the fkbp51 locus in VCaP and LNCaP prostate cancer cells, in response to the comercial androgen R1881 (64). Thus, SRs-associated histone acetylation and chromatin remodeling do not seem to occur only at promoter regions but also at distal downstream enhancers.
It has also been described that the glucocorticoid receptor exerts long-range regulation of fkbp51 transcription through distal sites located ∼100 kb from the TSS of the gene (65). Distal SRbs would facilitate the formation of transcriptional chromatin loops in certain genes, favoring the occurrence of protein–protein interactions among TFs bound to widely separated regulatory elements. The concept that the pre-assembled higher-order structures of chromatin can create boundaries for long-range interactions was also reported by Cullen et al. (66), who described the juxtaposition of distal ER-binding sites and proximal promoter regions of the prolactin gene in response to estrogens. Although we cannot discard the PR-dependent formation of chromatin loops in the bcl-x locus and their role on Pol II activity, ChIPs-seq assays did not reveal PR or Pol II enrichment around the promoter and 5′-end regions of the gene, both events expected if a promoter loop is formed. Furthermore, the observed Pol II accumulation toward the 3′-end of the gene in response to hormone treatment suggests a local effect of PR rather than a promoter-directed regulation.
Recent genome-wide studies have shown that nucleosomes downstream of paused polymerases act as physical barriers to polymerase elongation. Therefore, we propose that this local chromatin relaxation triggered by the activated PR bound to the intragenic PRbs would be involved in controling Pol II elongation along bcl-x gene.
Chromatin remodeling complexes and P-TEFb recruited to intragenic sites could facilitate transcription elongation
A connection between histone acetylation and transcription elongation was also suggested by the observation that acetylated histones regulate the recruitment of the positive elongator factor P-TEFb (67,68). Binding of P-TEFb would not only induce Pol II activation through the CDK9-dependent specific phosphorylation of the CTD but also counteract negatively acting elongation factors that keep the Pol II stalled near promoters (69–71).
As far as the SR mechanism of action is concerned, the regulation of pre-loaded Pol II activity has been described as the predominant genomic outcome of ER (16). In the absence of estrogens, a large number of ER target promoters are Pol II pre-loaded with minimal occupancy over the coding body, a phenomenon referred as pausing or promoter proximal enrichment of Pol II (PPEP). On hormone addition, ER binding to those promoters triggers the recruitment of P-TEFb favoring Pol II dispausing toward productive elongation (15). Even more, in a recent work, it was demonstrated that recruitment of the ER/P-TEFb complex to a well-know transcriptional pausing site located at ∼1.7 kb downstream of the TSS of c-myb gene (72) enables Pol II to resume transcriptional elongation (17). These results reinforce the idea that control of elongation in addition to transcription initiation are relevant mechanisms by which SRs control gene expression. In this sense, we propose that PR-dependent recruitment of P-TEFb to the intragenic bcl-x PRbs would mantain an elongating Pol II state by regulating the enzyme activity per se and/or by displacing negative elongation factors bound to DNA. Accordingly, activated PR not only induces bcl-x expression but also causes an increase in distal over proximal transcription.
Taking into account that elongation rate and processivity are functionally distinct and at least partially separable phenomena in vivo, and that most so-called elongation factors do not affect the rate at which Pol II travels down the gene (73), the combined recruitment of PR with CBP, GCN5 and P-TEFb to the different intragenic PRbs could explain, at least partially, that the increase in the Pol II abundance to the 3′ gene region and in the generation of full-length bcl-x transcripts would be consequence of combinatorial and complementary effects on the Pol II elongation steps. Thus, modulation of the productive elongation could be a mechanism by which PR induces transcription of target genes containing intragenic PRbs. In a first attempt to extend our findings, we searched for genes that had similar characteristics to bcl-x gene, comparable length and encompassing intragenic instead of classical promoter/enhancer PRbs. Out of three genes found, one of them, the ern1 gene showed hormone-dependent regulation similar to bcl-x; thus, taking into account the limitation in the number of genes analyzed, further studies are required to clarify the generality of this mechanism. However, results from the ChIP-seq of PR combined with the data from RNA-seq assays allow us to propose that internal PRbs are accumulated in up- and downregulated genes. Thus, more genes would follow the same trend as bcl-x, making the mechanism of gene activation mediated by intragenic PRbs a more general mechanism than originally expected. Second, these sites would also be relevant in hormone-dependent downregulation. How the specificity is acquired is a question that requires more investigation, but the existence of different pools of PR in the cell or the chromatin context of the PRbs in up- and downregulated genes are two interesting hypothesis that would explain, in part, our observations.
Finally, several questions arise from this work: what is the real outcome of P-TEFb recruitment to these intragenic sites? What is the relevance of these intragenic PRbs on other transcriptional steps? Are these sites able to directly recruit factors involved in pre-RNA processing? Here, we have also demonstrated that progestins are able to regulate the relative abundance between two bcl-x isoforms that are products of two alternative 5′-splice sites. This effect could be consequence of the increase in Pol II elongation efficiency triggered by the activated PR. In this sense, it was demonstrated that the decrease of Pol II elongation efficiency by ultraviolet irradiation correlates with an increase in the bcl-xS/bcl-xL ratio favoring apoptosis (51), suggesting that Pol II elongation activity would be directly associated with the alternative splicing of this gene. On the other hand, PR could also locally stimulate the recruitment of splicing factors on triggering chromatin remodelling as was found for the SRs co-activators CAPER, which act as splicing factors associated to hormone receptors (74,75).
In summary, intragenic PRbs distributed along bcl-x intron would act as ‘filling stations’ needed to refill the elongating polymerase with factors required for its proper travel along the gene and affecting the processing of bcl-x pre-RNAs (Figure 8).
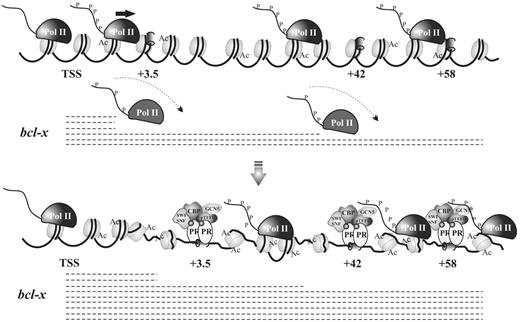
Schematic model of PR-dependent bcl-x transcription regulation. In the absence of hormone, the bcl-x gene presents a basal transcriptional activity characterized by the presence of some RNA Pol II molecules transcribing the entire gene, whereas they are commonly from the DNA template before reaching the 3′-end. On R5020 addition, PR is recruited to the intragenic PRbs along with the HATs CBP y GCN5 and the positive transcription elongation factor P-TEFb, favoring Pol II elongation. A higher number of Pol II molecules now are able to complete full-length transcription.
FUNDING
Ministerio de Educación y Ciencia (MEC), Spain [BFU2011-28587]; Consejo Nacional de Investigación Científica y Tecnológica [PIP112-200801-00859]; Agencia Nacional de Programación Científica y Tecnológica, [Préstamo BID-PICT2011-1321]; University of Buenos Aires, Argentina. A.R.K.; L.R.V. and A.P. are members of the CONICET-Argentina. G.P.V. is a recipient of the I3 Program. Funding for open access charge: Waived by Oxford University Press.
Conflict of interest statement. None declared.
ACKNOWLEDGEMENTS
The authors thank Dr M. Monte, School of Sciences, University of Buenos Aires for the HDACs vectors, Dr J. Valcarcel for useful help with the manuscript. P.Y.B. obtained a short-term scholarship from the UICC. Conceived and designed the experiments: P.Y.B., G.P.V. and A.P. Performed the experiments: P.Y.B., A.S.N., L.R.V., M.A., C.B. and R.Z. Analyzed the data: P.Y.B., G.C., D.S.; A.R.K., M.B., G.P.V., and A.P. Contributed reagents/materials/analysis tools: A.R.K., M.B., G.P.V. and A.P. Wrote the article: P.Y.B., G.P.V. and A.P.
REFERENCES
Author notes
The authors wish it to be known that, in their opinion, the first two authors should be regarded as joint First Authors.
Comments