-
PDF
- Split View
-
Views
-
Cite
Cite
Min Tan, Meng Wang, Xiao-Long Zhou, Wei Yan, Gilbert Eriani, En-Duo Wang, The Yin and Yang of tRNA: proper binding of acceptor end determines the catalytic balance of editing and aminoacylation, Nucleic Acids Research, Volume 41, Issue 10, 1 May 2013, Pages 5513–5523, https://doi.org/10.1093/nar/gkt252
- Share Icon Share
Abstract
Faithful translation of the genetic code depends on accurate coupling of amino acids with cognate transfer RNAs (tRNAs) catalyzed by aminoacyl-tRNA synthetases. The fidelity of leucyl-tRNA synthetase (LeuRS) depends mainly on proofreading at the pre- and post-transfer levels. During the catalytic cycle, the tRNA CCA-tail shuttles between the synthetic and editing domains to accomplish the aminoacylation and editing reactions. Previously, we showed that the Y330D mutation of Escherichia coli LeuRS, which blocks the entry of the tRNA CCA-tail into the connective polypeptide 1domain, abolishes both tRNA-dependent pre- and post-transfer editing. In this study, we identified the counterpart substitutions, which constrain the tRNA acceptor stem binding within the synthetic active site. These mutations negatively impact the tRNA charging activity while retaining the capacity to activate the amino acid. Interestingly, the mutated LeuRSs exhibit increased global editing activity in the presence of a non-cognate amino acid. We used a reaction mimicking post-transfer editing to show that these mutations decrease post-transfer editing owing to reduced tRNA aminoacylation activity. This implied that the increased editing activity originates from tRNA-dependent pre-transfer editing. These results, together with our previous work, provide a comprehensive assessment of how intra-molecular translocation of the tRNA CCA-tail balances the aminoacylation and editing activities of LeuRS.
INTRODUCTION
Aminoacyl-transfer RNA (tRNA) synthetases (aaRSs) perform a pivotal role in the decoding the genetic code by providing aminoacyl-tRNAs (aa-tRNAs) for protein biosynthesis (1,2). The aminoacylation reaction performed by aaRSs usually involves a two-step process in which the amino acid is first activated by adenosine triphosphate (ATP) to form an aminoacyl-adenylate (aa-AMP) intermediate and followed by transfer of the amino acid moiety of the aa-AMP to the 2′- or 3′- hydroxyl group of the terminal adenosine of tRNA to produce an aminoacyl-tRNA. These two steps are individual processes with tRNA not being required for amino acid activation of most aaRSs (2). Based on the conserved sequence and characteristic structural motifs, the 20 aaRSs can be classified into two families comprising 10 members each (3,4).
Leucyl-tRNA synthetase (LeuRS), together with isoleucyl-tRNA synthetase (IleRS) and valyl-tRNA synthetase (ValRS), belongs to the class Ia subgroup, which is characterized by an insertion domain located between the two halves of the Rossman fold and referred to as connective polypeptide 1 (CP1) (5–7). The CP1 domain, marked by a threonine-rich region and located ∼30 Å from the catalytic center for aminoacylation (8–10), is responsible for post-transfer editing. This process involves the hydrolysis of mis-charged tRNAs generated in the synthetic active site and subsequently transferred to the CP1 editing site (5,6,11–14). Furthermore, LeuRS and IleRS possess the pre-transfer editing ability to hydrolyze misaminoacyl-adenylate intermediates (9,15–19), which can occur in the absence or presence of tRNA (16–18). The synthetic domain performs tRNA-independent pre-transfer editing in addition to its internal tRNA aminoacylation activity (16,19). Entry of the tRNA CCA-tail into the CP1 domain is essential for tRNA-dependent pre-transfer editing (17) and results in the conformational change of LeuRS required for acceleration of hydrolysis of non-cognate aminoacyl-adenylates (11,20). The inter-domain communication between the synthetic and editing domains of LeuRS modulates this editing activity (21). The overall catalytic accuracy of aaRSs that is required to maintain the cellular function is achieved by the cooperation of pre- and post-transfer editing (17). Translational ambiguity arising from editing defects in aaRSs has profound functional consequences, including toxicity to bacteria and mammalian cells, and has been shown to give rise to serious pathological effects in mice (22–25).
The tRNA plays the central role in the catalytic processes of LeuRS, including aminoacylation, tRNA-dependent pre- and post-transfer editing reactions in species ranging from Escherichia coli (EcLeuRS) to humans (26–29). All of these functions of LeuRS rely on the intra-molecular translocation of tRNA CCA-tail between the synthetic and editing domains (20). The free tRNA CCA-tail preferentially binds to the CP1 domain and exhibits a regular helical conformation (corresponding to the low energy state) favoring binding to the discrete editing site under the condition that no amino acid was activated (20,30). In the aminoacylation conformation, the 3′ end of the tRNA CCA-tail distorts (corresponding to the high energy state) to bring the 2′-hydroxyl of the terminal ribose into the required position for attacking the aminoacyl-adenylate intermediate, suggesting that cis-elements of LeuRS are required to accomplish the translocation from the low energy state to the high energy state (20).
Our previous work shows that blocking entry of the tRNA CCA-tail into the CP1 abolishes both tRNA-dependent pre- and post-transfer editing (17). However, the mechanism by which the translocation of the tRNA CCA-tail into the synthetic domain modulates the catalytic functions of LeuRS remains to be elucidated.
In this study, we identified the key element involved in assisting the translocation of tRNA CCA into the synthetic domain and characterized the essential motif maintaining the uncharged tRNA in the synthetic domain. Mutations within these key residues disrupted the entry or binding of tRNA CCA-tail into the synthetic domain. As a consequence, post-transfer editing was severely reduced because tRNA cannot be charged with non-cognate amino acid. In contrast, the tRNA-dependent pre-transfer editing was significantly enhanced, resulting in increased overall editing activity. Taken together, our results indicated that the intra-molecular translocation of the tRNA CCA-tail balances the aminoacylation and tRNA-dependent editing functions of LeuRS.
MATERIALS AND METHODS
Materials
All chemicals were purchased from Sigma-Aldrich Inc. (St. Louis, MO, USA), unless otherwise noted. T4 polynucleotide kinase, T4 DNA ligase, RNasin (ribonuclease inhibitor), isopropyl β-d-thiogalactoside and all restriction endonucleases were obtained from Sangon Co. (Shanghai, China). All radioactive amino acids and ATP were purchased from PerkinElmer (Waltham, MA, USA). AN2690 was obtained from Milestone Pharmtech USA Inc. (New Brunswick, NJ, USA). GF/C and DE-81 filters were obtained from the Whatman Co. (Dassel, Germany). The gene-encoding tRNA nucleotidyltransferase and E. coli strain MT102 were from Architecture et Réactivité de l’ARN (Strasbourg, France).
Preparation of enzymes and RNA substrates
EcLeuRS was purified from an overproducing strain as previously described (10). The genes encoding the various mutants of EcLeuRS were constructed by the two-step polymerase chain reaction method, and the DNA sequences of all the mutants were confirmed by DNA sequencing. The EcLeuRS mutants were overproduced in E. coli as His-tagged proteins and purified on Ni-NTA Superflow column (Qiagen Inc., Germany), as described previously (13). E. coli tRNALeuGAG (EctRNALeu) with an accepting activity of 1500 pmol/A260 was prepared from an overproducing strain (31). [3H]Leu-EctRNALeu and [3H]-Ile-EctRNALeu were obtained using EcLeuRS-Y330D, as described previously (17).
ATP/pyrophosphate (PPi) exchange, tRNA charging and deacylation
The ATP-PPi exchange assay was performed as previously described (10). Aminoacylation activity of EcLeuRS was measured at 37°C in a reaction mixture containing 100 mM Tris–HCl (pH 7.8), 30 mM KCl, 12 mM MgCl2, 0.5 mM Dithiothreitol (DTT), 4 mM ATP, 20 μM EctRNALeu, 40 μM [3H]Leucine (15 Ci/mmol) and 20 nM EcLeuRS (10). Aminoacylation kinetic parameters of EcLeuRS for tRNALeu were determined in the presence of 0.5–30 μM tRNALeu. Assays of hydrolytic editing activity of EcLeuRS and its mutants were performed at 37°C in 100 mM Tris–HCl (pH 7.5), 30 mM KCl, 12 mM MgCl2, 0.5 mM DTT and 1 μM [3H]Ile-tRNALeu (300 μCi/μmol), and the reaction was initiated with 5 nM enzyme. Hydrolysis of Leu-tRNALeu (10 μM) was carried out in the same reaction buffer, except that the reaction was performed at 30°C to reduce spontaneous hydrolysis of Leu-tRNALeu.
Adenosine monophosphate (AMP) formation
AMP formation by EcLeuRS was measured as previously described (16). The reaction mixture (10 µl) contained 100 mM Tris–HCl (pH 7.8), 30 mM KCl, 12 mM MgCl2, 5 mM DTT, 5 U/ml pyrophosphatase, 3 mM ATP, 20 nM [α-32P]ATP (3000 Ci/mmol) and 15 mM norvaline (Nva), in the presence or absence of 5 µM tRNALeu. The reaction was initiated by the addition of 0.2 µM EcLeuRS or its mutants and incubated at 37°C. For Leu (5 mM) mis-editing, the concentration of enzyme was 1 µM. Aliquots (1.5 µl) were quenched with 6 µl of 200 mM sodium acetate (pH 5.0) and subsequently spotted (1.5 µl) in duplicate on polyethyleneimine cellulose plates pre-washed with water. Separation of aminoacyl-[32P]AMP, [32P]AMP and [32P]ATP was performed by developing thin layer chromatography (TLC) plates in 0.1 M ammonium acetate and 5% acetic acid. Plates were visualized by phosphorimaging, and data were analyzed using Multi Gauge V3.0 software (FUJIFILM). The gray densities of [32P] AMP spots were compared with the gray density of known [32P]ATP concentrations. Rate constants were calculated based on the slope of the graphical presentation of [32P]AMP formation plotted against time.
Aminoacylation and deacylation assays with non-radioactive Nva and α-amino butyrate
The tRNALeu was labeled at the 3′-terminal nucleotide using the exchange reaction catalyzed by tRNA nucleotidyl transferase, which was purified from E. coli overproduction strain in our laboratory as described previously (29). Reactions contained 50 mM Tris–HCl (pH 8.0), 12 mM MgCl2, 0.5 mM DTT, 5 µM tRNALeu, 5 µM ATP, 20 nM [α-32P]ATP (3000 Ci/mmol) and 3 µM tRNA nucleotidyl transferase. After incubation at 37°C for 5 min, yeast pyrophosphatase was added to a final concentration of 10 U/ml, and the reaction mixture was incubated for an additional 2 min before quenching by phenol extraction. The [32P]-labeled tRNALeu was then precipitated in −20°C and purified by polyacrylamide gel electrophoresis. Ligation of unlabeled Leu, Nva or α-amino butyrate (ABA) to [32P]-labeled tRNALeu was measured in aminoacylation assays, which were identical to those described above, except that 1 mM Leu, 25 mM Nva or 100 mM ABA was used. After aminoacylation, all reactions were stopped, and products were digested by placing 1 µl of reaction mixture in a buffer containing 40 mM sodium acetate (pH 4.5), 300 mM NaCl, 2 mM ZnSO4 and 5 U/µl S1 nuclease and incubating for 20 min at 37°C. Digested products were separated by TLC and quantified by phosphorimaging analysis as previously described (16).
Filter binding assays for determination of the KD for tRNALeu
The radiolabeled E. coli [32P] tRNALeu (50 nM, 45 000 cpm) was incubated in 50 μl of binding buffer [50 mM HEPES-KOH (pH 6.8), 30 mM KCl, 12 mM MgCl2] in the presence of increasing concentrations of LeuRS proteins for 15 min at 4°C. Samples were filtered through nitrocellulose membrane (Milipore, 0.22 μm) previously equilibrated in washing buffer [50 mM potassium phosphate (pH 5.5), 50 mM MgCl2]. Filters were then washed two times with 0.3 ml of washing buffer. Filters were air dried before radioactive quantification. Data were analyzed using GraphPad PRISM software.
RESULTS
R418 is required for the entry of tRNA CCA-tail into the synthetic active site
EcLeuRS consists of 860 amino acid residues. The CP1 domain is inserted into the synthetic active domain (G225–Y415). The side chain of R418, which forms part of the highly conserved 416-RLRDWGVSRQRYWG-429 motif, is located in the synthetic domain (20). In the editing conformation, R418 was close to the CP1 domain and pointing to the tRNA with a distance of 7.3 Å from the phosphate group of C74 in tRNA (Figure 1A) (20). R418 re-orientates to make base-specific hydrogen bonds with the discriminator base A73 of tRNA in the aminoacylating conformation (Figure 1B). These results indicated that R418 played a role in the translocation of tRNA from the CP1 domain to the synthetic domain. Substitution of R418 to Ala decreased the aminoacylation efficiency (kcat/KM) to 14% of that of wild-type (WT) enzyme (Table 1). In addition, substitution of R418 with acidic Glu or Asp almost abolished tRNA charging activity (Table 1 and Figure 1C). These results indicated that the electrical charge at position 418 was critical for aminoacylation activity, and this hypothesis was confirmed by the observation that aminoacylation activity was significantly recovered by the polar and uncharged R418Q or R418N mutations compared with the negatively charged R418E or R418D forms (Table 1, Figure 1C and Supplementary Table S1).
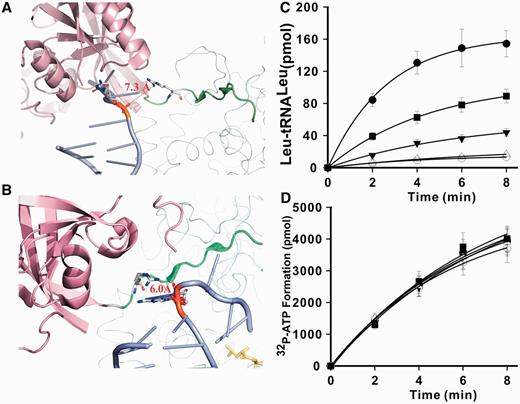
Effect of R418E substitutions on aminoacylation and amino acid activation. (A) Structure of EcLeuRS in complex with tRNALeu (light blue) in the editing conformation (PDB entry 4ARI) (20). The conserved R416–G429 motif was shown in green. R418 and C74 of tRNA were highlighted in the stick model with their distance labeled. The cartoon model of the editing domain (CP1) was in light pink, and the ribbon model of the synthetic was in cyan. (B) Detailed view of the spatial relationship between R418 (stick model) and tRNA (light blue with A73 in the stick model) in the aminoacylation conformation (PDB entry 4AQ7) (20). Cartoon model of the CP1 domain was in light pink. The synthetic domain was in ribbon model with cyan color. The conserved R416–G429 motif was shown in green. The analogue of aminoacyl-adenylate: Leu-AMS, which indicates the synthetic active center, was highlighted in yellow in stick model. The distance between R418 and A73 of tRNA was indicated. (C) Aminoacylation activities of WT EcLeuRS and its mutants. (D) Leu (5 mM) activation by WT EcLeuRS and its mutants. Symbols in C and D were as follows: EcLeuRS (black circle), -R418E (white circle), -R418D (white up-pointing triangle), -R418N (black square) and -R418Q (black down-pointing triangle).
Kinetic constants of EcLeuRS and its mutant in the aminoacylation reaction at 37°C
LeuRS . | KM of tRNA (µM) . | kcat (s−1) . | kcat/KM (s−1 µM−1) . | kcat/KM (relative) . | Loss . |
---|---|---|---|---|---|
WT | 2.7 ± 0.32 | 4.8 ± 0.54 | 1.78 | 1 | |
-R418E | 4.3 ± 0.36 | 0.44 ± 0.056 | 0.10 | 5.6 × 10−2 | 18 |
-R418D | 3.9 ± 0.41 | 0.4 ± 0.061 | 0.11 | 6.2 × 10−2 | 16 |
-R418A | 4.5 ± 0.58 | 1.2 ± 0.22 | 0.27 | 0.15 | 6.7 |
-E292R | 2.7 ± 0.3 | 0.65 ± 0.03 | 0.25 | 0.14 | 7.4 |
-R416E | 4.5 ± 0.51 | 2.3 ± 0.33 | 0.51 | 0.26 | 3.8 |
-E292R/R416E | 5.1 ± 0.43 | 4.7 ± 0.61 | 0.92 | 0.52 | 1.9 |
-R416E/R418E | 4.4 ± 0.47 | 0.13 ± 0.026 | 0.03 | 1.7 × 10−2 | 59 |
LeuRS . | KM of tRNA (µM) . | kcat (s−1) . | kcat/KM (s−1 µM−1) . | kcat/KM (relative) . | Loss . |
---|---|---|---|---|---|
WT | 2.7 ± 0.32 | 4.8 ± 0.54 | 1.78 | 1 | |
-R418E | 4.3 ± 0.36 | 0.44 ± 0.056 | 0.10 | 5.6 × 10−2 | 18 |
-R418D | 3.9 ± 0.41 | 0.4 ± 0.061 | 0.11 | 6.2 × 10−2 | 16 |
-R418A | 4.5 ± 0.58 | 1.2 ± 0.22 | 0.27 | 0.15 | 6.7 |
-E292R | 2.7 ± 0.3 | 0.65 ± 0.03 | 0.25 | 0.14 | 7.4 |
-R416E | 4.5 ± 0.51 | 2.3 ± 0.33 | 0.51 | 0.26 | 3.8 |
-E292R/R416E | 5.1 ± 0.43 | 4.7 ± 0.61 | 0.92 | 0.52 | 1.9 |
-R416E/R418E | 4.4 ± 0.47 | 0.13 ± 0.026 | 0.03 | 1.7 × 10−2 | 59 |
Kinetic parameters were determined using the tRNA charging assay described under ‘Materials and Methods’ section in the presence of 0.5–30 µM tRNALeu. All rates represented the average of three assays with the standard deviations indicated.
Kinetic constants of EcLeuRS and its mutant in the aminoacylation reaction at 37°C
LeuRS . | KM of tRNA (µM) . | kcat (s−1) . | kcat/KM (s−1 µM−1) . | kcat/KM (relative) . | Loss . |
---|---|---|---|---|---|
WT | 2.7 ± 0.32 | 4.8 ± 0.54 | 1.78 | 1 | |
-R418E | 4.3 ± 0.36 | 0.44 ± 0.056 | 0.10 | 5.6 × 10−2 | 18 |
-R418D | 3.9 ± 0.41 | 0.4 ± 0.061 | 0.11 | 6.2 × 10−2 | 16 |
-R418A | 4.5 ± 0.58 | 1.2 ± 0.22 | 0.27 | 0.15 | 6.7 |
-E292R | 2.7 ± 0.3 | 0.65 ± 0.03 | 0.25 | 0.14 | 7.4 |
-R416E | 4.5 ± 0.51 | 2.3 ± 0.33 | 0.51 | 0.26 | 3.8 |
-E292R/R416E | 5.1 ± 0.43 | 4.7 ± 0.61 | 0.92 | 0.52 | 1.9 |
-R416E/R418E | 4.4 ± 0.47 | 0.13 ± 0.026 | 0.03 | 1.7 × 10−2 | 59 |
LeuRS . | KM of tRNA (µM) . | kcat (s−1) . | kcat/KM (s−1 µM−1) . | kcat/KM (relative) . | Loss . |
---|---|---|---|---|---|
WT | 2.7 ± 0.32 | 4.8 ± 0.54 | 1.78 | 1 | |
-R418E | 4.3 ± 0.36 | 0.44 ± 0.056 | 0.10 | 5.6 × 10−2 | 18 |
-R418D | 3.9 ± 0.41 | 0.4 ± 0.061 | 0.11 | 6.2 × 10−2 | 16 |
-R418A | 4.5 ± 0.58 | 1.2 ± 0.22 | 0.27 | 0.15 | 6.7 |
-E292R | 2.7 ± 0.3 | 0.65 ± 0.03 | 0.25 | 0.14 | 7.4 |
-R416E | 4.5 ± 0.51 | 2.3 ± 0.33 | 0.51 | 0.26 | 3.8 |
-E292R/R416E | 5.1 ± 0.43 | 4.7 ± 0.61 | 0.92 | 0.52 | 1.9 |
-R416E/R418E | 4.4 ± 0.47 | 0.13 ± 0.026 | 0.03 | 1.7 × 10−2 | 59 |
Kinetic parameters were determined using the tRNA charging assay described under ‘Materials and Methods’ section in the presence of 0.5–30 µM tRNALeu. All rates represented the average of three assays with the standard deviations indicated.
Theoretically, the reduced aminoacylation activity could originate from either the aminoacylation reaction or the interaction with tRNA. As R418 was far from the catalytic active center characterized by the two conserved peptides KMSK and HIGH (Figure 1A and B), we proposed that mutations at R418 would not directly affect the catalytic center. The observation of intact amino acid activation activity among all the mutants compared with the WT enzyme supported this hypothesis (Figure 1D). Taking into account the observations from crystal structures (showing the proximity of oppositely charged tRNA and R418 in both the editing and aminoacylating conformations) and our biochemical data, we speculated that R418 functioned as a cis-element assisting in the translocation of the tRNA CCA-end between the synthetic and editing domains through interaction with tRNA. Mutations at R418, especially Glu or Asp, could disturb the interaction with tRNA through charge repulsion, preventing the entry of the tRNA CCA-tail into the synthetic active site and consequently, severely reducing aminoacylation activity.
Critical involvement of the salt bridge between E292 and R416 in aminoacylation
The mechanism by which EcLeuRS maintains the tRNA CCA-tail in the synthetic domain before its aminoacylation is unclear. In our previous work, we found that EcLeuRS could be spontaneously cleaved between E292 and A293 in the CP1 domain and that E292 was important for the aminoacylation activity (32,33). Recently reported crystal structures for EcLeuRS reveal that the CP1 domain is directly involved in positioning the tRNA for aminoacylation. E292 of the CP1 domain forms hydrogen bonds with the phosphate of A73 and the 2′-OH of U72 of the tRNALeu (20). E292 also forms a salt bridge with R416 at a distance of 2.8 Å (Figure 2A) (20). Furthermore, R416 makes the same base-specific hydrogen bonds with the tRNA discriminator A73 as R418. Formation of the E292–R416 salt bridge is required to facilitate translocation of the 3′ end of the tRNA from the editing site to the synthetic site. After aminoacylation reaction, this salt bridge is required to be disrupted for facilitating translocation of aminoacyl-tRNA out of the synthetic site. Thus, we investigated the role of this dynamic E292–R416 salt bridge in the aminoacylation reaction of EcLeuRS.
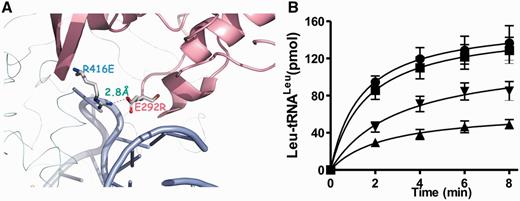
Effects of the E292-R418E salt bridge on aminoacylation activity. (A) E292 and R418 formed a salt bridge in the aminoacylation conformation. The picture was representation of part of the structure of the EcLeuRS complex with tRNA (light blue) in the aminoacylation conformation (PDB entry 4AQ7) (20). The CP1 domain was in cartoon model with light pink color. The synthetic domain was in ribbon model with cyan color. Both E292 and R416 were highlighted in stick model with index, and their distance was also labeled. (B) Aminoacylation of 20 µM tRNA by WT EcLeuRS and its mutants. Symbols were as follows: EcLeuRS (black circle), -E292R/R416E (black square), -E292R (black up-pointing triangle) and -R416E (black down-pointing triangle).
First, E292R or R416E substitutions were introduced to disrupt the salt bridge by charge repulsion. E292R severely decreased the kcat value to 0.65 s−1, ∼12.5% of that of the WT enzyme (4.8 s−1), while having no effect on KM value of tRNA (Table 1). R416E decreased the kcat value to ∼50% of that of WT enzyme and increased the KM value of tRNA by 100%. The overall catalytic efficiency (kcat/KM) of EcLeuRS-R416E was ∼25% of that of the WT EcLeuRS (Table 1). However, both mutations had no effect on amino acid activation activity (Supplementary Figure S1). These results indicated the important role of the E292–R416 salt bridge in aminoacylation. As both E292 and R416 form hydrogen bonds with A73 of the tRNA, we could not exclude the possibility that the reduced aminoacylation activity resulted from the effect on interactions with the tRNA. Regeneration of the salt bridge by combining E292R and R416E substitutions resulted in significant recovery of the aminoacylation activity (Table 1 and Figure 2B). The kcat value of EcLeuRS-E292R/R416E was identical to that of WT enzyme (4.7 s−1 versus 4.8 s−1). However, the KM value of the tRNA was ∼2-fold greater than that of the WT enzyme and similar to that of EcLeuRS-R416E (Table 1). We also combined the R418E substitution with E292R and R416E, alone and in together in the form of two double mutants R416E/R418E, E292R/R418E and a triple mutant E292R/R416E/R418E. The aminoacylation efficiency of E292R/R418E was ∼33% of that of E292R (0.09 s−1µM−1/0.025 s−1µM−1) (Supplementary Table S1). The catalytic efficiency of the R416E/R418E double mutant was significantly reduced (59-fold) with a kcat value (0.13 s−1) reduction to only 3% of that of the WT EcLeuRS (Table 1). Consistently, R418E replacement decreased the aminoacylation activity of the E292R/R416E mutant by ∼8-fold owing to a reduction in kcat (Table 1).
Taken together, these results showed that the salt bridge formed between positions 292 and 416 was important for the aminoacylation activity. We proposed that this potential interaction maintained the free tRNA in the synthetic domain before aminoacylation. Disruption of the salt bridge by E292R or R416E resulted in translocation of the tRNA from the synthetic site to a low energy state and concomitantly reduced aminoacylation activity. The aminoacylation activity was further reduced by a combination of E292R or R416E with R418E.
The effect of tRNA translocation on tRNA-dependent pre-transfer editing
These present mutants enabled us to further evaluate the effect of tRNA intra-molecular translocation on the editing activity of LeuRS. Total editing activity, including tRNA-independent, tRNA-dependent pre-transfer and post-transfer editing, is reflected by the rate of AMP formation in the presence of non-cognate amino acids and assayed by TLC (17). The contribution of tRNA-independent pre-transfer editing comprised <10% of the total editing activity in EcLeuRS (17). Our previous work suggested that the formation of mis-charged tRNA is the rate-limiting step of post-transfer editing in AMP accumulation. This process occurs in parallel with aminoacylation, and reduced aminoacylation activity in turn decreases post-transfer editing activity (21). All of the mutants (E292R, R416E and R418E) exhibited decreased aminoacylation activity, suggesting impaired post-transfer editing activity. To further support this hypothesis, we investigated a post-transfer editing mimicking reaction using the editing specificity altered T252A mutant (EcLeuRS-T252A) (13), which aminoacylates tRNA with Leu and subsequently hydrolyzes cognate Leu-tRNALeu owing to its enlarged aminoacyl moiety binding site in the CP1 domain (Figure 3A). Therefore, EcLeuRS-T252A mis-edits cognate Leu in a manner similar to WT EcLeuRS proofreading non-cognate Nva through the post-transfer editing cycle. In the TLC-based AMP formation assay with cognate Leu, the tRNA-dependent AMP formation rate of T252A was 1.1 s−1 (Table 2 and Figure 3C and D). T252A was combined with R416E and R418E, respectively. EcLeuRS-T252A/R416E exhibited an identical aminoacylation activity to EcLeuRS-R416E, which was higher than that of EcLeuRS-T252A (Supplementary Table S1). However, R418E further decreased the tRNA charging activity of EcLeuRS-T252A by almost 5-fold (Supplementary Table S1). The Leu-tRNALeu hydrolysis capacity of EcLeuRS-T252A/R418E was unchanged compared with that of EcLeuRS-T252A (Figure 3A), while that of EcLeuRS-T252A/R416E was severely reduced (Figure 3A). These results explain why R416E rescues the tRNA charging activity of EcLeuRS-T252A. Consistently, the R416E mutation almost abolished the tRNA-dependent editing activity of EcLeuRS-T252A towards Leu. The kobs of EcLeuRS-T252A/R416E for AMP formation in Leu mis-editing was 0.039 s−1, approximately 3.6% of that for EcLeuRS-T252A (kobs=1.1 s−1) (Table 2 and Figure 3B and D). The Leu mis-editing activity of EcLeuRS-T252A/R418E was approximately 14.3% of that for EcLeuRS-T252A, consistent with the decreased effect of the R418E mutation on the aminoacylation activity of EcLeuRS-T252A (Table 2, Figure 3C and D and Supplementary Table S1). These results strongly indicated that post-transfer editing of R416E and R418E was reduced to variable extents due to different mechanisms. Furthermore, we proposed that the E292R replacement also reduced post-transfer editing activity due to its reduced tRNA charging activity.
![Effect of R416E and R418E substitutions on the editing of Leu by EcLeuRS-T252A. (A) Hydrolysis 10 µM [3H]Leu-tRNALeu by 10 nM EcLeuRS mutants at 30°C. (B) AMP formation assay of EcLeuRS-T252A/R416E in the absence (left) and presence (right) of 5 µM tRNALeu with 5 mM Leu. (C) AMP formation assay of EcLeuRS-T252A (left) and -T252A/R418E (right) in the presence of 5 µM tRNALeu and 5 mM Leu. (D) The concentration of AMP produced during Leu editing by the mutants from panel (B) and (C) plotted versus time to fit a polynomial linear equation. The slope of the curve represented the kobs for AMP formation given in Table 2. Symbols in (A) were as follows: no enzyme (black circle), EcLeuRS-T252A (black square), -T252A/R418E (black up-pointing triangle), -T252A/R416E (black down-pointing triangle), and symbols in (D) are as follows: EcLeuRS-T252A with tRNA (black circle), -T252A/R418E with tRNA (black square), -T252A/R416E without tRNA (black up-pointing triangle), -T252A/R416E with tRNA (black down-pointing triangle).](https://oup.silverchair-cdn.com/oup/backfile/Content_public/Journal/nar/41/10/10.1093_nar_gkt252/2/m_gkt252f3p.jpeg?Expires=1750574571&Signature=vsdqD27cTBOKgcXohamFJsSQ-EplM9P2VEASit3MVo6WhmSJjGn5ITTaMwDVJZW9yXEOXfvgfGzFsowz02H5i3g9fLu9M1KHBF9JdSRuSgy38JxnJM4~BFLbr3FB09hA7eCUIlJCgIrndBgP3Mhlkdo1bf5Rf~D9hfAvCtuU3Q7zfCreNveOrnw~FYF-elxxVSqzimW4pSwh2ROVwUzbPWv5WK0-6ZVXZ1Dami8oEt5Sm-XaJBT9L311Tlnp7~IE-TkizNAtr4ym3LCiF6Ks4DsQK-F1NRR~RJqcwNfJANazjaX9CnM6VPnbAPersXPQCfIktNfPKzksWGDIyKI1Fw__&Key-Pair-Id=APKAIE5G5CRDK6RD3PGA)
Effect of R416E and R418E substitutions on the editing of Leu by EcLeuRS-T252A. (A) Hydrolysis 10 µM [3H]Leu-tRNALeu by 10 nM EcLeuRS mutants at 30°C. (B) AMP formation assay of EcLeuRS-T252A/R416E in the absence (left) and presence (right) of 5 µM tRNALeu with 5 mM Leu. (C) AMP formation assay of EcLeuRS-T252A (left) and -T252A/R418E (right) in the presence of 5 µM tRNALeu and 5 mM Leu. (D) The concentration of AMP produced during Leu editing by the mutants from panel (B) and (C) plotted versus time to fit a polynomial linear equation. The slope of the curve represented the kobs for AMP formation given in Table 2. Symbols in (A) were as follows: no enzyme (black circle), EcLeuRS-T252A (black square), -T252A/R418E (black up-pointing triangle), -T252A/R416E (black down-pointing triangle), and symbols in (D) are as follows: EcLeuRS-T252A with tRNA (black circle), -T252A/R418E with tRNA (black square), -T252A/R416E without tRNA (black up-pointing triangle), -T252A/R416E with tRNA (black down-pointing triangle).
Observed rate constants for AMP synthesis at 37°C by EcLeuRS and its mutants in the presence of Leu
LeuRS . | AMP formation kobs (s−1) . | |
---|---|---|
−tRNA . | +tRNA . | |
-T252A | (1.5 ± 0.2) × 10−2 | 1.1 ± 0.1 |
-T252A/R416E | (1.7 ± 0.2) × 10−2 | (3.9 ± 0.6) × 10−2 |
-T252A/R418E | (1.3 ± 0.2) × 10−2 | (1.4 ± 0.2) × 10−1 |
LeuRS . | AMP formation kobs (s−1) . | |
---|---|---|
−tRNA . | +tRNA . | |
-T252A | (1.5 ± 0.2) × 10−2 | 1.1 ± 0.1 |
-T252A/R416E | (1.7 ± 0.2) × 10−2 | (3.9 ± 0.6) × 10−2 |
-T252A/R418E | (1.3 ± 0.2) × 10−2 | (1.4 ± 0.2) × 10−1 |
Rates for AMP formation were determined in the TLC assay described under ‘Materials and Methods’ section. All rates represented the average of three assays with the standard deviations indicated.
Observed rate constants for AMP synthesis at 37°C by EcLeuRS and its mutants in the presence of Leu
LeuRS . | AMP formation kobs (s−1) . | |
---|---|---|
−tRNA . | +tRNA . | |
-T252A | (1.5 ± 0.2) × 10−2 | 1.1 ± 0.1 |
-T252A/R416E | (1.7 ± 0.2) × 10−2 | (3.9 ± 0.6) × 10−2 |
-T252A/R418E | (1.3 ± 0.2) × 10−2 | (1.4 ± 0.2) × 10−1 |
LeuRS . | AMP formation kobs (s−1) . | |
---|---|---|
−tRNA . | +tRNA . | |
-T252A | (1.5 ± 0.2) × 10−2 | 1.1 ± 0.1 |
-T252A/R416E | (1.7 ± 0.2) × 10−2 | (3.9 ± 0.6) × 10−2 |
-T252A/R418E | (1.3 ± 0.2) × 10−2 | (1.4 ± 0.2) × 10−1 |
Rates for AMP formation were determined in the TLC assay described under ‘Materials and Methods’ section. All rates represented the average of three assays with the standard deviations indicated.
In editing Nva, EcLeuRS-R418E and EcLeuRS-E292R exhibited significantly enhanced total editing activity compared to the WT enzyme (Figure 4 and Table 3). The observed rate constants (kobs) of EcLeuRS-R418E and EcLeuRS-E292R for AMP formation were 7.4 s−1 and 9.3 s−1, respectively, while that of WT EcLeuRS was 3.3 s−1(Table 3). The editing activity of EcLeuRS-E292R/R418E was also higher than that of WT EcLeuRS (6.5 versus 3.3 s−1) (Table 3). Above results showed that decreased aminoacylation activity resulted in reduced post-transfer editing activity (Table 2 and Figure 3C). None of these mutants affected tRNA-independent pre-transfer editing (Table 3). Thus, we hypothesized that the mutations of R418E and E292R, both alone and in combination, significantly increased tRNA-dependent pre-transfer editing, leading to enhanced total editing activity. EcLeuRS-R416E had a similar total editing activity to that of the WT enzyme (kobs: 3.1 versus 3.3 s−1) (Table 3 and Figure 4), indicating that R416E also increased the tRNA-dependent pre-transfer editing to a level just compensating for its decreased post-transfer editing activity. Interestingly, R416E decreased the enhanced tRNA-dependent editing activity of EcLeuRS-E292R and -R418E. The tRNA-dependent AMP formation rate catalyzed by EcLeuRS-E292R/R416E, -R416E/R418E and -E292R/R416E/R418E fell to 2.5, 2.3 and 2.1 s−1, respectively (Table 3). Although the T252A mutation led to altered editing specificity, it had little effect on the editing of Nva, which was similar to that of WT enzyme. In addition, EcLeuRS-T252A/R418E (7.7 s−1) and -T252A/R416E (2.2 s−1) also had similar total editing activity compared with EcLeuRS-R418E (7.4 s−1) and -R416E (3.1 s−1), respectively (Supplementary Table S2 andSupplementary Figure S2).
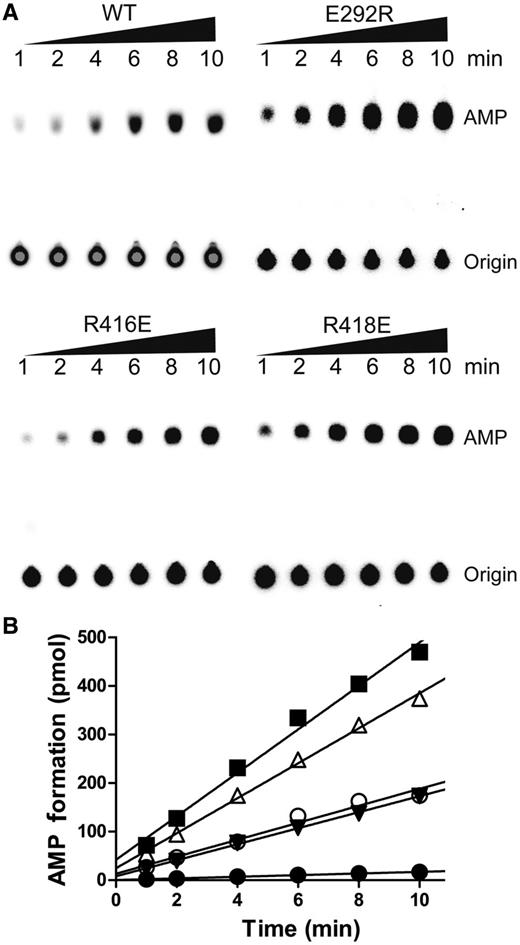
AMP formation assay in the presence of Nva. (A) TLC-based AMP formation assay of mutants EcLeuRS-E292R, -R416E and -R418E (0.2 µM each) in the presence of 5 µM of tRNALeu and 15 mM of Nva. (B) Graphical representation of AMP formation; kobs of AMP formation was calculated from the slope and reported in Table 3. Symbols were as follows: no tRNA (black circle), EcLeuRS with tRNA (white circle), -E292R with tRNA (black square), -R418E with tRNA (white up-pointing triangle) and -R416E with tRNA (black down-pointing triangle).
Observed rate constants for AMP synthesis at 37°C by EcLeuRS and its mutants in the presence of Nva
LeuRS . | AMP formation kobs (s−1) . | |
---|---|---|
−tRNA . | +tRNA . | |
WT | (3.3 ± 0.40) × 10−1 | 3.3 ± 0.51 |
-R418E | (3.2 ± 0.50) × 10−1 | 7.4 ± 1.10 |
-E292R | (3.6 ± 0.32) × 10−1 | 9.3 ± 0.60 |
-E292R/R418E | (3.1 ± 0.27) × 10−1 | 6.5 ± 0.72 |
-R416E | (3.5 ± 0.56) × 10−1 | 3.1 ± 0.36 |
-E292R/R416E | (3.4 ± 0.43) × 10−1 | 2.5 ± 0.34 |
-R416E/R418E | (3.7 ± 0.38) × 10−1 | 2.3 ± 0.26 |
-E292R/R416E/R418E | (3.2 ± 0.41) × 10−1 | 2.1 ± 0.35 |
LeuRS . | AMP formation kobs (s−1) . | |
---|---|---|
−tRNA . | +tRNA . | |
WT | (3.3 ± 0.40) × 10−1 | 3.3 ± 0.51 |
-R418E | (3.2 ± 0.50) × 10−1 | 7.4 ± 1.10 |
-E292R | (3.6 ± 0.32) × 10−1 | 9.3 ± 0.60 |
-E292R/R418E | (3.1 ± 0.27) × 10−1 | 6.5 ± 0.72 |
-R416E | (3.5 ± 0.56) × 10−1 | 3.1 ± 0.36 |
-E292R/R416E | (3.4 ± 0.43) × 10−1 | 2.5 ± 0.34 |
-R416E/R418E | (3.7 ± 0.38) × 10−1 | 2.3 ± 0.26 |
-E292R/R416E/R418E | (3.2 ± 0.41) × 10−1 | 2.1 ± 0.35 |
Rates for AMP formation were determined in the TLC assay described under ‘Materials and Methods’ section. All rates represented the average of three assays with the standard deviations indicated.
Observed rate constants for AMP synthesis at 37°C by EcLeuRS and its mutants in the presence of Nva
LeuRS . | AMP formation kobs (s−1) . | |
---|---|---|
−tRNA . | +tRNA . | |
WT | (3.3 ± 0.40) × 10−1 | 3.3 ± 0.51 |
-R418E | (3.2 ± 0.50) × 10−1 | 7.4 ± 1.10 |
-E292R | (3.6 ± 0.32) × 10−1 | 9.3 ± 0.60 |
-E292R/R418E | (3.1 ± 0.27) × 10−1 | 6.5 ± 0.72 |
-R416E | (3.5 ± 0.56) × 10−1 | 3.1 ± 0.36 |
-E292R/R416E | (3.4 ± 0.43) × 10−1 | 2.5 ± 0.34 |
-R416E/R418E | (3.7 ± 0.38) × 10−1 | 2.3 ± 0.26 |
-E292R/R416E/R418E | (3.2 ± 0.41) × 10−1 | 2.1 ± 0.35 |
LeuRS . | AMP formation kobs (s−1) . | |
---|---|---|
−tRNA . | +tRNA . | |
WT | (3.3 ± 0.40) × 10−1 | 3.3 ± 0.51 |
-R418E | (3.2 ± 0.50) × 10−1 | 7.4 ± 1.10 |
-E292R | (3.6 ± 0.32) × 10−1 | 9.3 ± 0.60 |
-E292R/R418E | (3.1 ± 0.27) × 10−1 | 6.5 ± 0.72 |
-R416E | (3.5 ± 0.56) × 10−1 | 3.1 ± 0.36 |
-E292R/R416E | (3.4 ± 0.43) × 10−1 | 2.5 ± 0.34 |
-R416E/R418E | (3.7 ± 0.38) × 10−1 | 2.3 ± 0.26 |
-E292R/R416E/R418E | (3.2 ± 0.41) × 10−1 | 2.1 ± 0.35 |
Rates for AMP formation were determined in the TLC assay described under ‘Materials and Methods’ section. All rates represented the average of three assays with the standard deviations indicated.
Collectively, E292R, R416E and R418E substitutions disrupted the entry of the tRNA CCA-tail into the synthetic active site, leading to significantly enhanced tRNA-dependent pre-transfer editing activity. R416E eliminated the increased pre-transfer editing by E292R and R418E, suggesting that R416E had an additional effect on tRNA-dependent pre-transfer editing.
Mutation of R416E affected the interaction with tRNA when its CCA-tail binds in the CP1 domain
Our previous work showed that tRNA-dependent pre-transfer editing of LeuRS is a process triggered by tRNA to accelerate the hydrolysis of non-cognate aminoacyl-adenylate (17). The binding of the tRNA CCA-tail to the CP1 domain is a prerequisite of this editing function. The side chain of Y330 is in close proximity (2.1 Å) to the phosphate of the tRNA A76. Y330D substitution results in charge repulsion of the phosphate of A76 and prevents entry of the tRNA CCA-tail into the CP1 domain (17). As a consequence, both tRNA-dependent pre- and post-transfer editing were abolished. The crystal structure of EcLeuRS indicates that the distance between R416 and the C74 phosphate is approximately 4.3 Å when tRNA binds in the CP1 domain (Figure 5A) (20). Such a short distance between two moieties with same charge is enough to induce repulsion and therefore. We proposed that R416E affected the interaction between tRNA and the enzyme in the editing conformation. R416E almost abolished the hydrolysis activity towards Leu-tRNALeu by EcLeuRS-T252A. Consistently, R416E exhibited 4-fold decrease in deacylation activity towards Ile-tRNALeu (Figure 5B). As R416 was far from the active site of the CP1 domain (Figure 5A), we speculated that the negative effect of R416E on hydrolysis of cognate or non-cognate aminacyl-tRNAs was due to its interaction with tRNA. In contrast, there was a 7.3 Å distance between R418 and the C74 phosphate (Figure 1A). Such a distance guaranteed that the R418E substitution had little effect on the binding of tRNA in the CP1 domain. Therefore, R418E replacement led only to a slight decrease in deacylation activity (Figure 5B). E292 was invisible in the editing conformation of EcLeuRS (20). According to the structure of Thermus thermophilus LeuRS, E292 is located at a 17 Å distance from the closest nucleotide of tRNA in the editing conformation (Supplementary Figure S3) (7), which may explain why the E292R mutation also had no effect on aminoacyl-tRNA hydrolysis (Figure 5B).
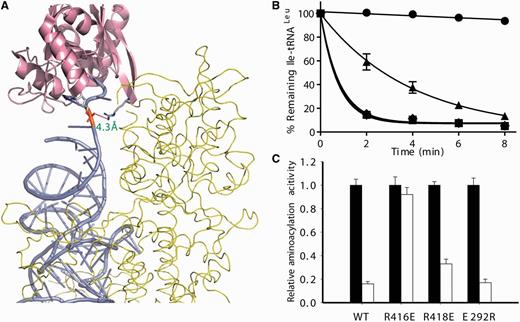
R416E mutation affects the binding of tRNA in the CP1 domain. (A) The orientation of R416 and C74 of tRNA in the editing conformation. The picture was representation of part of the crystal structure of EcLeuRS complex with tRNA in the editing conformation (PDB entry 4ARI) (20). R416 and C74 of tRNA were highlighted in the stick model, and their distance was labeled. Cartoon model of the editing domain (CP1) (light pink) and ribbon model of the synthetic and C-terminal domains (yellow). (B) Hydrolysis of 1 µM 3H-Ile-tRNALeu by EcLeuRS and its mutants. (C) The effect of AN2690 on the aminoacylation activity of EcLeuRS and its mutants. For each enzyme, the initial activity in the presence of 100 µM AN2690 (white) was normalized to that without AN2690 (black). Symbols in (B) are as follows: no enzyme (black circle), EcLeuRS (black square), -R416E (black up-pointing triangle), -R418E (black down-pointing triangle), -E292R (black diamond suit).
AN2690, a boron-containing compound, forms a stable adduct with the 2′- and 3′-oxygen atoms of the tRNA 3′-terminal adenosine and the CP1 domain, thus trapping the tRNA CCA-tail (30). This inhibitory mechanism depends on the precise interaction between tRNA and CP1 domain. AN2690 (100 µM) inhibited >80% of the charging activity of WT EcLeuRS (Figure 5C), which was consistent with previous reports (17,34). The tRNA charging activity of EcLeuRS-E292R and -R418E was also inhibited by AN2690 (100 µM) (5- and 3-fold reductions, respectively) (Figure 5C). However, EcLeuRS-R416E was resistant to AN2690 (Figure 5C). The distance between R416 and the AN2690 binding site in the CP1 domain determined that R416E could not directly affect the binding of AN2690 in the CP1 domain, implying that the resistance of the R416E replacement to AN2690 was due to its interaction with tRNA. We also measured the tRNA dissociation constants (KD) of the mutants by filter binding assays. The KD of EcLeuRS-R416E for tRNA increased 5-fold, while that of EcLeuRS-R418E increased only 2-fold and that of EcLeuRS-E292R remained unchanged compared with that of WT EcLeuRS (Table 4).
LeuRS . | KD (μM) . |
---|---|
WT | 0.20 ± 0.041 |
-R416E | 1.03 ± 0.224 |
-R418E | 0.39 ± 0.073 |
-E292R | 0.19 ± 0.054 |
LeuRS . | KD (μM) . |
---|---|
WT | 0.20 ± 0.041 |
-R416E | 1.03 ± 0.224 |
-R418E | 0.39 ± 0.073 |
-E292R | 0.19 ± 0.054 |
KD values were determined by filter binding assays in the presence of concentrations of LeuRS ranging between 0.1 and 15 µM. All rates represent the average of three assays with the standard deviations indicated.
LeuRS . | KD (μM) . |
---|---|
WT | 0.20 ± 0.041 |
-R416E | 1.03 ± 0.224 |
-R418E | 0.39 ± 0.073 |
-E292R | 0.19 ± 0.054 |
LeuRS . | KD (μM) . |
---|---|
WT | 0.20 ± 0.041 |
-R416E | 1.03 ± 0.224 |
-R418E | 0.39 ± 0.073 |
-E292R | 0.19 ± 0.054 |
KD values were determined by filter binding assays in the presence of concentrations of LeuRS ranging between 0.1 and 15 µM. All rates represent the average of three assays with the standard deviations indicated.
Altogether, these results strongly indicated that the R416E mutation affected the binding of the tRNA CCA-tail to the CP1 domain, whereas R418E and E292R had little or no effect on this interaction. We speculated that the effect of R416E on the interaction between tRNA and CP1 domain in the editing conformation was the mechanism underlying inhibition of the enhanced tRNA-dependent editing activity by E292R and R418E (Table 3). These results also indicated that the enhanced tRNA-dependent pre-transfer editing by E292R or R418E mutations relied on the precise binding of tRNA CCA-tail in the CP1 domain.
Effect of E292, R416 and R418 mutations on the aminoacylation fidelity
To investigate the effect of E292, R416 and R418 mutations on the fidelity of aminoacylation, we measured the steady-state rate of these mutants for formation of Nva-tRNALeu and ABA-tRNALeu with ABA, which is a compound smaller than Nva and also is activated by LeuRS (29). We used [32P] tRNALeu and TLC to separate the digestion products of nuclease S1 and monitored the rates of aminoacyl-tRNA formation. The rate of Leu-tRNALeu formation was also measured for comparison with the results established with the radiolabeled amino acid. The rates of Leu-tRNALeu formation (kobs) by EcLeuRS-E292R and -R418E were 0.13 s−1 and 0.15 s−1, respectively, whereas EcLeuRS-R416E exhibited a higher Leu-tRNALeu formation rate (kobs = 0.61 s−1). The kobs of the WT enzyme was 6.41 s−1. These values were consistent with the steady-state kinetics measured with [3H]Leu, showing that E292R and R418E mutations decreased the aminoacylation activity more significantly than the R416E mutation (Table 1). When tested in the presence of Nva or ABA, only mutant EcLeuRS-R416E formed detectable amounts of Nva- and ABA-tRNALeu, although the rates only reached 0.15 s−1 and 0.071 s−1, respectively (Table 5 and Supplementary Figure S4). The corresponding values for the editing deficient EcLeuRS-Y330D were 2.89 s−1 and 1.36 s−1, respectively. The aminoacylation activity of EcLeuRS-R416E was ∼20% of that of-Y330D. However, the efficiency of mis-charged tRNA formation by EcLeuRS-R416E was ∼3–5% of that by EcLeuRS-Y330D. These results suggested that the preserved editing activity of EcLeuRS-R416E, which comprised predominantly tRNA-dependent pre-transfer editing, could, to some extent, maintain the catalytic fidelity. No detectable Nva- or ABA-AMP was formed by the mutants EcLeuRS-E292R and EcLeuRS-R418E or the WT EcLeuRS.
LeuRS . | kobs(s−1) . | ||
---|---|---|---|
Leu (1 mM) . | Nva (25 mM) . | ABA (100 mM) . | |
WT | 6.41 ± 0.46 | nd | nd |
-R416E | (6.1 ± 1.4) × 10−1 | (1.5 ± 0.03) × 10−1 | (7.1 ± 0.8) × 10−2 |
-Y330D | 2.96 ± 0.22 | 2.89 ± 0.26 | 1.36 ± 0.16 |
-E292R | (1.3 ± 0.6) × 10−1 | nd | nd |
-R418E | (1.5 ± 0.33) × 10-1 | nd | nd |
LeuRS . | kobs(s−1) . | ||
---|---|---|---|
Leu (1 mM) . | Nva (25 mM) . | ABA (100 mM) . | |
WT | 6.41 ± 0.46 | nd | nd |
-R416E | (6.1 ± 1.4) × 10−1 | (1.5 ± 0.03) × 10−1 | (7.1 ± 0.8) × 10−2 |
-Y330D | 2.96 ± 0.22 | 2.89 ± 0.26 | 1.36 ± 0.16 |
-E292R | (1.3 ± 0.6) × 10−1 | nd | nd |
-R418E | (1.5 ± 0.33) × 10-1 | nd | nd |
Aminoacylation rates were determined using [32P]-labeled tRNAs, S1 digestion and TLC analysis.
The values represented the mean value of at least two independent experiments with the standard deviations indicated.
nd, not determined (because activity was too low for reliable detection).
LeuRS . | kobs(s−1) . | ||
---|---|---|---|
Leu (1 mM) . | Nva (25 mM) . | ABA (100 mM) . | |
WT | 6.41 ± 0.46 | nd | nd |
-R416E | (6.1 ± 1.4) × 10−1 | (1.5 ± 0.03) × 10−1 | (7.1 ± 0.8) × 10−2 |
-Y330D | 2.96 ± 0.22 | 2.89 ± 0.26 | 1.36 ± 0.16 |
-E292R | (1.3 ± 0.6) × 10−1 | nd | nd |
-R418E | (1.5 ± 0.33) × 10-1 | nd | nd |
LeuRS . | kobs(s−1) . | ||
---|---|---|---|
Leu (1 mM) . | Nva (25 mM) . | ABA (100 mM) . | |
WT | 6.41 ± 0.46 | nd | nd |
-R416E | (6.1 ± 1.4) × 10−1 | (1.5 ± 0.03) × 10−1 | (7.1 ± 0.8) × 10−2 |
-Y330D | 2.96 ± 0.22 | 2.89 ± 0.26 | 1.36 ± 0.16 |
-E292R | (1.3 ± 0.6) × 10−1 | nd | nd |
-R418E | (1.5 ± 0.33) × 10-1 | nd | nd |
Aminoacylation rates were determined using [32P]-labeled tRNAs, S1 digestion and TLC analysis.
The values represented the mean value of at least two independent experiments with the standard deviations indicated.
nd, not determined (because activity was too low for reliable detection).
DISCUSSION
The mechanism by which AN2690 inhibits LeuRS activity suggested that the free 3′ end of tRNA has to sample the editing site before aminoacylation reaction (30). Recently reported crystal structures for EcLeuRS revealed that the tRNA CCA-tail adopts a regular conformation to bind in the CP1 domain (20), which corresponds to a low energy state. To perform the aminoacylation, the tRNA CCA-tail is required to translocate to the synthetic site. In that conformation, the tRNA and enzyme complex is in a high energy state with regard to the distorted tRNA CCA-tail and constrained enzyme. Four flexibly linked LeuRS domains, the CP1 domain, leucine-specific domain, ZN1 module and the C-terminal domain, exhibit correlated rotation to facilitate the translocation of tRNA (20).
The side chain of R418 points to the tRNA CCA-tail in both the editing and aminoacylation conformations, indicating that R418 is in coupled motion with the translocation of the tRNA acceptor stem. In the present work, we showed that R418 was extremely important for aminacylation activity. R418A mutation decreased the aminoacylation efficiency by 6.7-fold. Introduction of the acidic residues Glu or Asp at R418 almost abolished the tRNA charging activity with 16- and 18-fold reductions in catalytic efficiency, respectively. However, EcLeuRS-R418Q or -R418N exhibited significantly higher aminoacylation activity than R418E or R418D. Based on the observation of tertiary structure and our mutagenesis study, we propose that R418 functions as a cis-element to promote the translocation of the tRNA CCA-tail to the synthetic domain through its positive charge. Mutations of R418, especially negatively charged Glu and Asp, disrupted the entry of the tRNA acceptor into the synthetic active site, resulting in diminished or almost complete abolition of aminoacylation activity.
Although R416 is in a similar orientation compared with R418 in both the aminoacylation and editing conformations, it affects the aminoacylation activity in a different manner. In the aminoacylation conformation, E292 and R416 form a strong salt bridge with a 2.8 Å distance at the surface between the CP1 and synthetic domains (20). We further showed that this salt bridge, rather than the two individual residues, was important for aminoacylation. Single site mutation (E292R or R416E) disrupted the salt bridge through charge repulsion, leading to 7.4- and 3.8-fold reductions in aminoacylation efficiency, respectively. Regeneration of the salt bridge by combining E292R and R416E in the double mutant EcLeuRS-E292R/R416E resulted in almost complete recovery of the charging activity (Table 1 and Figure 2B). The tertiary structural study also reveals that disruption of this salt bridge is required to facilitate translocation of charged tRNA from the synthetic site to the editing site for checking the aminoacyl moiety (20). Thus, we propose that this salt bridge is an important part of frame that maintains the tRNA acceptor stem in the synthetic domain before its aminoacylation. Without this salt bridge, the tRNA would prefer to transform to its lower energy state with the CCA-tail binding in the editing domain before being charged with an amino acid, resulting in diminished aminoacylation activity.
In the editing activity assay, E292R and R418E mutations increased the total editing activity by 2- and 3-fold (Table 3), respectively; however, EcLeuRS-R416E exhibited a similar total editing activity compared with EcLeuRS. A post-transfer editing mimicking assay, which is based on the altered editing specificity of EcLeuRS-T252A, showed that all of these mutants exhibited reduced post-transfer editing activity (Figure 3). R416E decreased post-transfer editing through restriction of both the formation and subsequently the hydrolysis of mis-charged tRNA. The decline in post-transfer editing of EcLeuRS-R418E and -E292R arose from their severely reduced tRNA activity. Previous pre-steady-state kinetic assays showed that rapid transfer of amino acids to tRNA in EcLeuRS is coincident with its robust post-transfer editing activity, whereas direct hydrolysis of the aminoacyl-adenylate intermediate is inhibited (35). Here, we show that restricted tRNA charging by EcLeuRS-E292R and -R418E significantly enhanced tRNA-dependent pre-transfer editing activity, leading to increased total editing activity. Both E292R and R418E mutations resulted in preferential binding of the tRNA CCA-tail to the editing domain, which was shown in our previous work to activate the tRNA-dependent pre-transfer editing capability of EcLeuRS (17,21). Greater binding of the tRNA CCA-tail in the CP1 domain in the presence of E292R or R418E results in greater direct hydrolysis of mis-activated amino acids, thus contributing to the enhanced tRNA-dependent pre-transfer editing. The R416E mutation has a similar effect on tRNA translocation as E292R. However, EcLeuRS-R416E has an identical editing activity to WT EcLeuRS. Furthermore, the R416E mutation blocked the enhanced tRNA-dependent pre-transfer editing of EcLeuRS-E292R or -R418E. These results indicate that R416E also affects tRNA-dependent pre-transfer editing in a manner different from that in EcLeuRS-E292R or -R418E. The crystal structure of EcLeuRS revealed a distance of only 4.3 Å between R416 and the C74 phosphate in the editing conformation. Such a distance is sufficient to allow the formation of interactions between two molecules with opposite charges. R416E substitution decreases the hydrolysis of aminoacyl-tRNA and leads to insensitivity to AN2690. Both of these reactions require precise interactions between the tRNA CCA-tail and the CP1 domain. Furthermore, EcLeuRS-R416E increased the KD for tRNA by 5-fold. According to these results, we propose that the R416E mutation results in repulsion of the tRNA, thus preventing optimal binding of the tRNA CCA-tail in the CP1 domain.
Altogether, our data show that the equilibrium of the tRNA acceptor stem between the editing and synthetic domains determines the relative efficiencies of the aminoacylation and editing reactions in a process analogous to the balance between the Chinese Yin and Yang. Interestingly, this system provides the opportunity for more efficient elimination of aa-tRNAs produced by a mutated synthetic active site. In the event of a mutation also affecting the amino acid-charging specificity, such mechanism would help the cell to limit the synthesis of mis-charged tRNA and thereby to survive until the occurrence of a new mutation that would suppress the effect of the first mutation.
FUNDING
Funding for open access charge: National Key Basic Research Foundation of China [2012CB911001]; Natural Science Foundation of China [30930022 and 31130064]; Committee of Science and Technology in Shanghai [12JC1409700] and Exchange Program and Programme International de Coopération Scientifique from CNRS [3606].
Conflict of interest statement. None declared.
REFERENCES
Author notes
The authors wish it to be known that, in their opinion, the first two authors should be regarded as joint First Authors.
Comments