-
PDF
- Split View
-
Views
-
Cite
Cite
Tuuli Välineva, Jie Yang, Olli Silvennoinen, Characterization of RNA helicase A as component of STAT6-dependent enhanceosome, Nucleic Acids Research, Volume 34, Issue 14, 1 September 2006, Pages 3938–3946, https://doi.org/10.1093/nar/gkl539
- Share Icon Share
ABSTRACT
Signal transducer and activator of transcription 6 (STAT6) is a regulator of transcription for interleukin-4 (IL-4)-induced genes. The ability of STAT6 to activate transcription depends on functional interaction with other transcription factors and coactivators. We have characterized the mechanism of STAT6-mediated transcriptional activation by identifying STAT6 transcription activation domain (TAD) interacting nuclear proteins. The first of the identified proteins was coactivator protein p100, which regulates IL-4-induced transcription by connecting STAT6 with other transcriptional regulators. Here, we describe RNA helicase A (RHA) as a novel component of STAT6 transcriptosome. In vitro and in vivo experiments indicated that RHA did not directly interact with STAT6, but p100 protein was found to mediate the assembly of the ternary complex of STAT6-p100-RHA. In chromatin immunoprecipitation studies RHA together with p100 enhanced the binding of STAT6 on the human Igε promoter after IL-4 stimulation. RHA enhanced the IL-4-induced transcription, and the participation of RHA in IL-4-regulated transcription was supported by RNAi experiments. Our results suggest that RHA has an important role in the assembly of STAT6 transcriptosome. As RHA is also known to interact with chromatin modifying proteins, the RHA containing protein complexes may facilitate the entry of transcriptional apparatus to the IL-4 responsive promoters.
INTRODUCTION
Regulation of transcription is dependent both on general transcription factors as well as transcriptional activators and coactivators. These proteins are assembled into a nucleoprotein complex called the enhanceosome (1–3). In the enhanceosome transcriptional coactivators form multifunctional protein–protein complexes, which are assembled in a modular fashion, connect DNA-binding sequence-specific regulators to the basal transcription machinery, and facilitate chromatin remodelling and modifications (4,5).
Signal transducer and activator of transcription 6 (STAT6) has an important role in regulation of interleukin-4 (IL-4)-induced gene responses. IL-4 has pleiotropic effects on the immune system. It induces activated B lymphocytes to proliferate and to synthesize IgE and IgG1, and T cells to differentiate towards Th2 cells (6–8). IL-4 has also an important role in the pathogenesis of asthma and allergy. IL-4 stimulation results in activation of JAK1 and JAK3 tyrosine kinases, which in turn phosphorylate and activate STAT6 monomers. Phosphorylated STAT6 molecules dimerize and translocate into the nucleus, where they bind to the specific recognition sequences in the promoters of IL-4 responsive genes.
The ability of STAT6 to activate transcription is dependent on the cooperation with other transcription factors and coactivators on the IL-4 responsive promoters. STAT6 has been shown to cooperate with NF-κB, PU.1, IRF-4, BSAP and C/EBPβ transcription factors (9–14). The mechanisms of this interplay vary, e.g. C/EBPβ stabilizes the DNA-binding of STAT6, whereas NF-κB and PU.1 are required for transcriptional activation of STAT6. In addition, transcriptional coregulators for STAT6 have been identified, such as the CREB-binding protein (CBP), which acts as a coactivator for numerous transcription factors including all the STAT proteins (15–20). CBP/p300 stimulates the transcription of target genes by several mechanisms; CBP/p300 regulates chromatin remodelling through intrinsic histone acetyltransferase activity (21,22) and it also associates with p/CAF, another histone acetyltransferase (23). In addition, CBP/p300 can act as a coactivator by bridging transcription factors to basal transcription machinery (24–26). Also a CBP-associated protein NcoA-1, a member of the p160/steroid receptor coactivator family, has been shown to function as a coactivator for STAT6 (27).
The transcription activation domain (TAD) of STAT6 is located in the C-terminus of the protein. TADs are the most divergent domains among different STATs, and are capable of functioning as independent transactivators (28,29). To investigate the mechanism of STAT6-mediated transcriptional activation, we have analyzed STAT6-TAD interacting nuclear proteins, and identified novel, putative components of the STAT6 enhanceosome. Our previous studies led to the identification of staphylococcal nuclease (SN)-like domain containing protein p100 as a STAT6 interacting protein and an important regulator of IL-4-induced transcription (30). Here, we report identification of another novel member of the STAT6 transcriptosome, RNA helicase A (RHA). RHA possesses several functions in modulation of gene expression. RHA participates in many aspects of RNA processing and particularly catalyzes the unwinding of both double-stranded RNA and DNA (31–33). In addition, RHA functions as a transcriptional coactivator by bridging RNA Polymerase II to CBP (26). Our results show that RHA functions as a coactivator in STAT6-mediated transcriptional activation of IL-4-regulated genes. RHA connects to STAT6 via p100 protein, and it participates in the assembly of the STAT6-dependent enhanceosome on the human Igε promoter.
MATERIALS AND METHODS
Cell culture and transfections
HeLa cells and COS-7 cells were grown as previously reported (30). Transfection of HeLa cells was performed using the calcium phosphate precipitation method. COS-7 cells were transfected by electroporation with a Bio-Rad gene pulser at 260 V/960 μF.
Plasmids
pSG5-p100-Flag, pSG5-p100-SN-Flag, pSG5-p100-TD-Flag, GST-p100-SN, GST-p100-TD, pCIneo-C-termHA-STAT6, GST-St6-TAD (amino acids 642–847) and Igε-reporter plasmids have been described earlier (30,34). pCIneo-N-termHA-STAT6 was generated by PCR using primers containing the HA-epitope sequence inserted into the N-terminus of STAT6. IL-4R-reporter plasmid (IL-4R-STAT6x3-luc) contains three copies of STAT6 response element from IL-4-receptor gene promoter (35). It was constructed by cloning annealed oligonucleotides, sense, 5′-p-AGCTTAGCTTCTTCATCTGAAAAGAGCTTCTTCATCTGAAAAGAGCTTCTTCATCTGAAAAGG-3′ and antisense 5′-p-TCGACCTTTTCAGATGAAGAAGCTCTTTTCAGATGAAGAAGCTCTTTTCAGATGAAGAAGCTA-3′, into HindIII and SalI sites of pfLUC plasmid. pGEX-RHA1 (amino acids 1–262), -2 (amino acids 255–664), -3 (amino acids 649–1077) and -4 (amino acids 1064–1270), pcDNA3-HA/RHA and pcDNA3-HA/RHAmATP plasmids were kindly provided by Dr T. Nakajima (26,36).
GST-fusion proteins
GST-fusion proteins were expressed in Escherichia coli and purified with GST affinity beads (glutathione–Sepharose 4B, Pharmacia).
COS-7 cell lysates
Transfected COS-7 cells were lysed in NP-40 lysis buffer (50 mM Tris–HCl, pH 7.5, 150 mM NaCl, 1 mM EDTA, 0.5% NP-40, 10% glycerol and 50 mM sodium fluoride) supplemented with PMSF and aprotinin. Protein concentrations of the lysates were measured using the Bio-Rad Protein Assay system (Bio-Rad Laboratories, Hercules, CA).
In vitro translation
35S-labeled proteins were prepared by in vitro translation using TNT T7/T3 Coupled Reticulosyte Lysate System (Promega) according to manufacturer's instructions.
GST pulldown assays
Purified GST-fusion proteins were incubated with COS-7 cell lysates or in vitro translated proteins diluted in NP-40 lysis buffer (50 mM Tris–HCl, pH 7.5, 150 mM NaCl, 1 mM EDTA, 0.5% NP-40, 10% glycerol and 50 mM sodium fluoride). After washes with NP-40 lysis buffer, the bound proteins were eluted from beads, separated by SDS–PAGE and analyzed by immunoblotting with mouse monoclonal anti-Flag M2 (Sigma) or mouse monoclonal anti-HA (clone 16B 12; BabCO) antibodies, or by autoradiography.
Co-immunoprecipitation
Transfected HeLa or COS-7 cells were resuspended in NP-40 lysis buffer (50 mM Tris–HCl, pH 7.5, 150 mM NaCl, 1 mM EDTA, 0.5% NP-40, 10% glycerol and 50 mM sodium fluoride) supplemented with PMSF and aprotinin. Protein concentrations of the lysates were measured using the Bio-Rad Protein Assay system (Bio-Rad Laboratories, Hercules, CA). The cell lysates were immunoprecipitated with rabbit polyclonal anti-STAT6 (Santa Cruz Biotechnology), anti-Flag M2 (Sigma), anti-HA antibody (clone 16B 12; BabCO) or as a control mouse monoclonal anti-c-Myc antibody (Roche). The immunoprecipitated proteins were separated by SDS–PAGE and detected by blotting with anti-Flag M2 antibody (Sigma), anti-STAT6 (Santa Cruz Biotechnology) or anti-HA antibody (clone 16B 12; BabCO).
Chromatin immunoprecipitation
HeLa cells were starved and stimulated with IL-4 (10 ng/ml) for 4 h. Chromatin immunoprecipitation (ChIP) experiments were carried out as described previously (37) using anti-STAT6 (Santa Cruz Biotechnology), anti-Flag (Sigma) or anti-HA (clone 16B 12; BabCO) antibodies. Immunoprecipitated DNA was analyzed for human Igε promoter by quantitative RT–PCR using QuantiTect SYBR Green PCR Kit (Qiagen) according to the manufacturer's instructions. The following primers were used: 5′-TGGGCCTGAGAGAGAAGAGA-3′ and 5′-AGCTCTGCCTCAGTGCTTTC-3′. The results were normalized against the total input DNA.
Reporter gene assays
HeLa cells were transfected on six-well plates with 500 ng of reporter plasmid, 200 ng of pCMV-β-galactosidase plasmid, 200 ng of STAT6-wt plasmid and different expression plasmids as indicated in the figures. One day after transfection, cells were starved overnight in DMEM containing 1% FBS and treated or left untreated with 10 ng/ml of recombinant human IL-4 (PeproTech EC Ltd, London, GB). Cells were lysed in Reporter Lysis Buffer (Promega, Madison, WI) and luciferase activity was measured using the Luciferase Assay System (Promega, Madison, WI) according to manufacturer's instructions. The luciferase values were normalized against β-galactosidase activity of the lysates.
RNAi
siRNAs were generated using the Ambion SilencerTM siRNA Construction Kit, according to the manufacturer's protocol. Sense and antisense primers corresponding to the following target sequences were used: RHA I 5′-AACCACACAGGTTCCCCAGTT-3′ (corresponding to nt 1331–1351 of human RHA mRNA), RHA II 5′-AAGGAAAGCAACTGGGCTATA-3′ (corresponding to nt 2764–2784 of human RHA mRNA). Primers were extended with the sequence 5′-CCTGTCTC-3′ at the 3′ end. Transfection of cells was performed as follows: HeLa cells were plated in 24-well tissue-culture plates at a density of 3–5 × 104 cells per well and incubated for 24 h before transfection. Cotransfection of 100 ng of Igε-reporter, 50 ng of β-galactosidase and 60 pmol of siRNA were performed with Oligofectamine (Invitrogen). Twenty-four hours after transfection cells were either stimulated with 10 ng/ml of IL-4 or left untreated. Cells were lysed in Reporter Lysis Buffer (Promega, Madison, WI) 48 h after transfection. Luciferase expression was determined using the Luciferase Assay System (Promega, Madison, WI) and the results were normalized against β-galactosidase activity of the lysates.
RESULTS
Interaction between RHA and STAT6
To identify possible coregulators of STAT6, the TAD of STAT6 was expressed as a glutathione S-transferase (GST) fusion protein (GST-St6TAD) and used to purify interacting nuclear proteins from Ramos B cells, as explained by Yang et al. (30). The band corresponding to a 140 kDa protein was recovered and subjected to in-gel trypsin digestion. The molecular masses of the digested peptides were measured by matrix-assisted laser desorption ionization time-of-flight (MALDI-TOF) mass spectrometry. The program ProFound was used to compare the mass maps obtained against theoretical tryptic peptide mass maps in the OWL protein sequence database. The comparison resulted in identification of the 140 kDa protein as human RNA helicase A protein.
Initially GST pulldown assay method was used to investigate, whether the detected interaction between STAT6 and RHA was direct or mediated via bridging factors. The bacterially produced GST-fusion proteins were purified using glutathione–Sepharose beads. The purity and the quantity of the fusion proteins were then analyzed with SDS–PAGE and protein staining. Equal amounts of fusion proteins bound to glutathione beads were then used for binding assays with either ectopically expressed or in vitro translated proteins. Protein complexes were separated by SDS–PAGE. GST-fusion proteins containing different RHA fragments (GST-RHA1, 2, 3, 4) (26,36) were used in the interaction assays. Fragment 1 (amino acids 1–262) consists of the N-terminal basic region, in which there are two double-stranded RNA binding domains. Fragments 2 (amino acids 255–664) and 3 (amino acids 649–1077) form the central core of the enzyme, and contain the helicase motifs. ATP binding domain is situated in the fragment 2. C-terminal fragment 4 (amino acids 1064–1270) contains an RGG-rich region, which is capable of binding single-stranded nucleic acids (38). GST-RHA fusion proteins and as a negative control GST recombinant proteins were produced in bacteria and affinity purified. GST and GST-fusion proteins were incubated with COS-7 cell lysates expressing HA-tagged STAT6, or with in vitro translated STAT6. After SDS–PAGE, an analysis of the RHA-bound proteins was performed. Anti-HA western blotting or autoradiography failed to detect any interaction between GST-RHA-fusion proteins and the expressed or in vitro translated STAT6 (Figure 1A–C). Interaction between RHA and STAT6 was also studied reciprocally: purified GST-STAT6-TAD and GST recombinant proteins were incubated with lysates expressing HA-tagged full-length RHA or with in vitro translated RHA. The results in Figure 1E and F show that RHA did not interact with GST-STAT6-TAD.
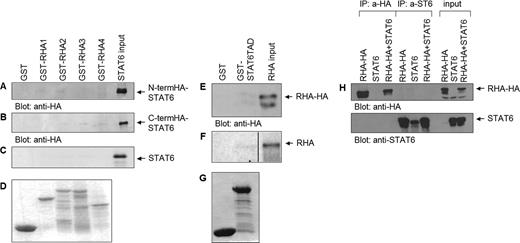
RHA does not directly interact with STAT6 in vitro or in vivo. (A) GST and GST-RHA fragments 1–4 were incubated with cell lysates ectopically expressing N-terminally HA-tagged STAT6, (B) C-terminally HA-tagged STAT6 or (C) in vitro translated STAT6. The bound proteins were subjected to SDS–PAGE and analyzed by western blotting with anti-HA antibody or autoradiography. (D) Expression level of GST-fusion proteins. (E) GST and GST-STAT6-TAD were incubated with cell lysates ectopically expressing HA-tagged RHA or (F) in vitro translated RHA. The bound proteins were subjected to SDS–PAGE and analyzed by western blotting with anti-HA antibody or autoradiography. (G) Expression level of GST-fusion proteins. (H) COS-7 cells were transfected with plasmids encoding HA-tagged full-length RHA and STAT6 either separately or together. Cell extracts were immunoprecipitated with anti-HA or anti-STAT6 antibodies and immunoblotted with anti-HA or with anti-ST6 antibody. Input lanes show 10% of the total protein used in immunoprecipitation experiments.
Co-immunoprecipitation method was used to analyze whether RHA-STAT6 complex can be detected in vivo. COS-7 cells were transfected with HA-epitope tagged RHA and STAT6 either separately or together. Immunoprecipitation from total cell lysates was performed using anti-HA or anti-STAT6 antibodies. After SDS–PAGE and western blotting, the presence of RHA and STAT6 was detected using anti-HA and anti-STAT6 antibodies. As shown in Figure 1H, RHA did not immunoprecipitate with STAT6. Taken together these results indicate that RHA and STAT6 do not interact directly, and suggest that the initially detected interaction is mediated by bridging proteins.
Interaction between RHA and p100
Previously we have shown that p100 interacts with STAT6 both in vitro and in vivo and functions as an adapter protein for RNA pol II and CBP (30,37). Therefore, we wanted to investigate whether RHA-STAT6 interaction could be mediated by p100.
We used co-immunoprecipitation method to investigate whether RHA and p100 form a complex. COS-7 cells were transfected with HA-epitope tagged RHA and Flag-tagged p100 either separately or together. RHA was immunoprecipitated from the total cell lysates with anti-HA, or as a negative control, with anti-Myc antibody, and the presence of p100 was detected after SDS–PAGE and western blotting with anti-Flag antibody. p100 was found to immunoprecipitate with RHA (Figure 2A) but p100 was not detected in anti-HA immunoprecipitations from cells transfected only with RHA or p100. Stripping and reprobing of the same membrane with anti-HA shows equal amounts of RHA in different samples. Neither p100 nor RHA was detected from the control sample precipitated with anti-Myc or from non-transfected control sample (COS-7 cell 0-lysate). To study the interactions with endogenous STAT6, HeLa cells were transfected with RHA, with or without p100-Flag. STAT6 was immunoprecipitated from total cell lysates with anti-STAT6 antibody, and the presence of RHA was detected by anti-HA immunoblotting. As shown in Figure 2B, RHA did not coimmunoprecipitate with STAT6 in cells transfected with only RHA. However, RHA was detected in the STAT6 immunocomplex in cells ectopically expressing p100 protein, indicating that p100 protein could function as a bridging factor between RHA and STAT6 in vivo.
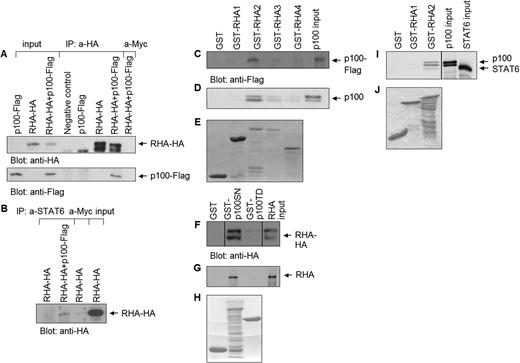
p100 interacts directly with RHA in vitro and in vivo, and bridges RHA to STAT6. (A) COS-7 cells were transfected with plasmids encoding HA-tagged full-length RHA and Flag-tagged p100. Cell extracts were immunoprecipitated with anti-HA or as a negative control with anti-Myc antibody. Immunodetection was performed with anti-Flag antibody, and re-immunodetection with anti-HA antibody. Input lanes show 10% of the total protein used in immunoprecipitation experiments. (B) HeLa cells were transfected with HA-tagged full-length RHA and Flag-tagged p100. Cell extracts were immunoprecipitated with anti-STAT6 or as a negative control with anti-Myc, and immunoblotted with anti-HA antibody. Input lane shows 20% of the total protein used in immunoprecipitation experiment. (C) GST and GST-RHA fragments 1–4 were incubated with overexpressed, Flag-tagged p100, or (D) in vitro translated p100. The bound proteins were subjected to SDS–PAGE and analyzed by western blotting with anti-Flag antibody or autoradiography. (E) Expression level of GST-fusion proteins. (F) GST, GST-p100-SN and GST-p100-TD were incubated with overexpressed, HA-tagged RHA or (G) in vitro translated RHA. The bound proteins were subjected to SDS–PAGE and analyzed by western blotting with anti-HA antibody or autoradiography. (H) Expression level of GST-fusion proteins. (I) GST and GST-RHA fragments 1 and 2 were first incubated with in vitro translated p100 and after stringent washes with in vitro translated STAT6. The bound proteins were subjected to SDS–PAGE and analyzed by autoradiography. (J) Expression level of GST-fusion proteins.
In order to define, which domains of p100 and RHA interact, GST pulldown assays were performed. GST-fusion proteins containing two different p100 fragments, GST-p100-SN and GST-p100-TD, were used. GST-p100-SN fusion protein contains the four SN-like domains and GST-p100-TD the single TD-domain of p100. GST-fusion proteins were incubated with overexpressed HA-tagged RHA or with in vitro translated RHA. As shown in Figure 2F and G, RHA interacted specifically with GST-p100-SN, but not with GST or GST-p100-TD. Similar experiments were performed reciprocally: bacterially produced GST-RHA fragments were incubated with Flag-tagged, overexpressed or in vitro translated p100. The results show that p100 interacts specifically with GST-RHA2 (Figure 2C and D).
To confirm the assembly of STAT6-p100-RHA ternary protein complex, GST pulldown experiments were performed with GST-RHA2 or GST-RHA1 as a negative control, and in vitro translated STAT6 and p100. GST-fusion proteins were first incubated with p100, and after stringent washes (NP-40 lysis buffer with 300 mM NaCl) with STAT6. The results in Figure 2I show that both the higher migrating p100 as well as the lower migrating STAT6 were detected in GST-RHA2 pulldown but not with GST-RHA1 pulldown.
To conclude, these results indicate that RHA interacts directly with p100. The interaction is mediated by fragment 2 (amino acids 255–664) of RHA and SN domains of p100. The results also suggest that p100 protein functions as a bridging factor between STAT6 and RHA.
In vivo binding of STAT6, p100 and RHA complex to the Igε promoter
To confirm that RHA is recruited to STAT6-dependent IL-4-inducible Igε promoter, chromatin immunoprecipitation assays were performed. Cells were transfected with p100-Flag, RHA-HA or empty vector, stimulated with IL-4 or left untreated. Immunoprecipitation of cross-linked chromatin was performed with anti-STAT6, anti-Flag or anti-HA antibodies. DNA was extracted and analyzed for the STAT6 binding site containing region of human Igε promoter.
As shown in Figure 3, IL-4 stimulation increased the binding of endogenous STAT6 to the promoter. Ectopic expression of RHA alone did not affect the STAT6 promoter binding, but expression of p100 together with RHA enhanced the promoter binding of STAT6 after IL-4 stimulation. This result confirms that a ternary complex between RHA, p100 and STAT6 is formed on Igε promoter after IL-4 stimulation, and suggests, that RHA participates in the assembly of STAT6 enhanceosome through p100.
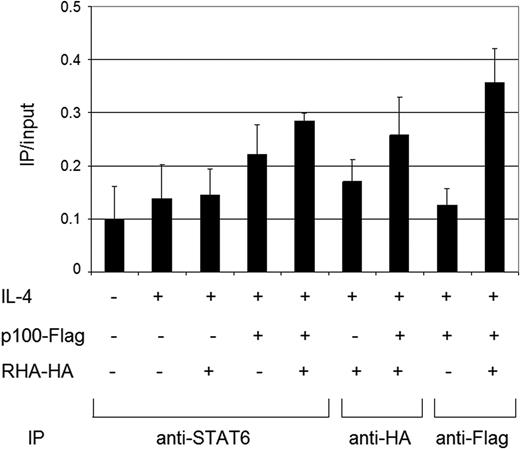
In vivo binding of STAT6, p100 and RHA complex to the Igε promoter HeLa cells were transfected with plasmids encoding p100, RHA or empty vector as indicated in the figure. Cells were treated with IL-4 for 4 h or left untreated. Immunoprecipitation of cross-linked chromatin was performed with anti-STAT6, anti-Flag or anti-HA. DNA was extracted and analyzed for the region of human Igε promoter that contains a STAT6 binding site by quantitative RT–PCR. The results were normalized against the total input DNA.
The functional role of RHA in STAT6-mediated transcriptional activation
p100 as well as RHA have been reported to function as transcriptional regulators. p100 functions as a coactivator for EBNA2, STAT5 and STAT6, but has also been reported to inhibit c-Myb-dependent promoter activation (39–41). RHA cooperates with CBP and acts as a positive regulator in the transcriptional activation via the cAMP responsive factor CREB. The helicase activity of RHA, which is dependent on the ATP binding activity, is required for mediating the target gene activation (26).
The functional role of RHA in STAT6-mediated transcriptional activation was investigated with the luciferase reporter gene assay. HeLa cells were cotransfected with STAT6-dependent Igε- or IL-4R-promoter containing luciferase reporter genes together with increasing amounts of RHA or mutant form of RHA, which is defective in ATP-binding and helicase activity (Figure 4A and B). IL-4 stimulation resulted in Igε- or IL-4R-reporter stimulation. The basal activity of the reporter was not affected, but RHA expression enhanced the IL-4-stimulated reporter gene activity in a dose dependent manner. The ATP-binding mutant of RHA inhibited IL-4-induced Igε- and IL-4R-reporter gene expression. The mutant form of RHA was not defective in binding to p100, suggesting that the interaction is not dependent on the helicase/ATP-binding activity of RHA (data not shown).
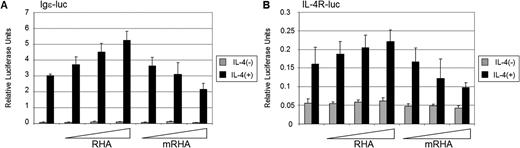
RHA enhances STAT6-dependent transcriptional activation HeLa cells were transfected with either Igε- (A) or IL-4R-luciferase reporter (B), β-galactosidase, STAT6 and increasing amounts of either RHA or ATP-binding mutant RHA (mRHA). Twenty-four hours after transfection cells were starved and either stimulated with IL-4 or left untreated. Cells were lysed 48 h after transfection and luciferase expression was determined. The results were normalized against β-galactosidase activity of the lysates.
We also performed knockdown experiments using two different siRNAs directed against RHA in Igε-reporter gene assays (Figure 5). IL-4 induced strong transcriptional activation in the control cells that were not treated with siRNA or treated with siRNA directed against scrambled sequence. As expected, depletion of STAT6 by siRNA completely abrogated Igε reporter activation. Also both siRNAs directed against RHA caused a marked inhibition of Igε reporter activation. In control experiments the RHA siRNAs reduced the amount of RHA protein by ∼60%. Taken together, these results suggest that RHA is a coactivator in STAT6-mediated transcription, and this function is dependent on its helicase activity.

RHA siRNA inhibits Igε-reporter gene expression HeLa cells were transfected with Igε-luciferase reporter, β-galactosidase and siRNAs directed against RHA (RHA I and II), STAT6, luciferase or scrambled sequence (scr). Twenty-four hours after transfection cells were either stimulated with IL-4 or left untreated. Cells were lysed 48 h after transfection and luciferase expression was determined. The results were normalized against β-galactosidase activity of the lysates.
DISCUSSION
The assembly of transcription apparatus at eukaryotic promoters involves a large number of transcription factors and coregulators. These proteins both modify chromatin structures and recruit the basal transcription machinery to the DNA. STAT6 is a signal transducer and transcription activator that is activated by IL-4 induced tyrosine phosphorylation and mediates most of the IL-4- and IL-13-induced gene responses (6). The mechanisms by which STAT6 activates gene transcription are still largely unknown. Identification of the transcriptional coregulators of STAT6 and the composition of STAT6 transcriptosome is an important step in solving the events leading to transcriptional activation of IL-4-regulated genes including the allergy mediator Igε.
In this study we identified RHA as a novel component of STAT6 enhanceosome. RHA is considered to participate in regulation of transcription by several mechanisms, which involve both bridging functions and helicase functions. RHA is detected in RNA polymerase II (RNA pol II) holoenzyme complexes and it interacts directly with RNA pol II (26). RHA has also been reported to interact with several other nuclear proteins necessary for transcriptional activation, including CBP/p300, topoisomerase IIα, breast cancer specific tumor suppressor protein BRCA1 and survival of motor neurons (SMN) complex (26,42–44). RHA is able to bind both DNA and RNA, but it has been suggested to be mainly pre-RNA and mRNA-binding protein. RHA has stronger affinity towards single-stranded than double-stranded DNA substrates, suggesting that RHA might have a transient role in DNA-binding during transcript formation (45). The centrally located catalytic core domain of RHA contains a DExH motif and a group of seven helicase motifs. The unwinding activity driven by ATP is localized to the core domain, where the ATP binding site is situated (38). It is expected, that RHA induces local changes in chromatin structure of promoter DNA, promoting the entry of the transcriptional apparatus (26).
The interaction between RHA and STAT6 was mediated by p100 protein. p100 (SND1, Tudor-SN) was initially identified as a transcriptional coactivator for Epstein–Barr virus nuclear antigen 2 (EBNA2) (39), and subsequently been shown to interact with other transcription factors such as TFIIE, c-Myb, STAT5 and STAT6 (30,39–41). p100 consists of four SN-like domains and a single TD-domain. SN-like domains of p100 resemble staphylococcal nucleases, small calcium-dependent enzymes that hydrolyze both DNA and RNA (46). However, SN-like domains of p100 lack catalytic activity, but instead are implicated to function as interaction domains. The first subdomain in the SN-like domains forms an oligonucleotide/oligosaccharide-binding (OB)-fold structure. However, it is still not clear, whether p100 can directly bind to DNA. Instead, SN-like domains have been shown to function as protein interaction domains: p100 has been reported to interact with c-Myb and Pim-1 serine/threonine kinase through sequences located in SN-like domains (41,47). p100 also interacts both with STAT6-TAD, STAT5TAD, RNA Pol II and CBP through the SN-like domains (30,37,40). Here, we show that the SN-like domains of p100 also mediate the interaction between STAT6 and RHA. Thus, the SN-like domains appear to have evolved as important docking elements in the assembly of transcription complexes, and multiplication of the SN-like domains has provided possibilities for several simultaneous protein–protein interactions. The TD-domain of p100 is a hybrid SN-like domain, in which the OB-domain has been replaced by a domain found in Drosophila melanogaster Tudor protein (46). The tudor domain of p100 has been suggested to function in RNA or protein binding and RNA metabolism and/or transport (48). Our recent results support this notion and indicate that the TD domains are important for pre-RNA processing (Yang et al., unpublished).
The interaction between p100 and RHA is mediated by RHA fragment 2 (amino acids 255–664), which is located in the central catalytic core of the enzyme. This fragment contains several helicase motifs and the ATP binding domain; a K/R 417 substitution in this domain abrogates the helicase activity. The same region has previously been reported to interact with the 200 kDa subunit of RNA pol II (26) and BRCA1 (43), while the interaction between RHA and CBP is mediated by the N-terminal region (amino acid 1–250) of RHA. Both CBP and BRCA1 activate transcription when they are linked to the RNA pol II holoenzyme via RHA. BRCA1 is suggested to function as a transcriptional coactivator by interacting with specific DNA binding transcription factors to transmit a regulatory signal to the RNA pol II holoenzyme via RHA. BRCA1 mutants, which have a reduced ability to bind to RHA, are observed in breast cancer. It has been suggested, that weaker interaction between RHA and BRCA1 decreases the transcriptional activity of BRCA1, leading to the development of breast cancer (43).
RHA was also found to function as coactivator for two different IL-4-regulated gene promoters, Igε and IL-4R. Mutation of the ATP binding lysine in RHA did not affect the interaction with p100, but abolished the coactivator function. Thus, the helicase activity of RHA appears to be required for the transcriptional effect. Previously, RHA has been shown to enhance the transactivation of NF-κB p65 in helicase activity-dependent manner (49). Our results are consistent with a model where RHA functions in STAT6 transcriptosome as a chromatin remodelling factor. RHA interacts also with other chromatin modifying proteins including CBP (26) and topoisomeraseIIα (42), and recruitment of these proteins to the promoter may facilitate the entry of transcription activation complexes to the initiation site.
RHA is involved in transcription activation complexes composed of several different transcription factors and coregulators, and may therefore be an essential coregulator for a variety of transcriptional pathways. The specificity may be determined by the interacting proteins and the composition of the transcription activation complex. This study provides a new insight into the mechanisms of STAT6-mediated transcriptional activation. Our results suggest that RHA acts as a coactivator for STAT6 in IL-4 induced STAT6-mediated transcription. In addition to its function as a bridging factor, the ATPase/helicase activity of RHA is also important for transactivation. Interestingly, chromatin immunoprecipitation assays indicated that the formation of ternary complex between p100, RHA and STAT6 resulted in enhanced promoter binding of STAT6, thus suggesting that the ternary protein complex may facilitate the unwinding of the chromatin structure and accessibility of the promoter. The role of p100 on RHA promoter recruitment was further investigated by RNAi experiments. Knockdown of p100 reduced but did not abolish the promoter binding of RHA (data not shown) suggesting that also other coactivator proteins such as CBP are likely to function in the recruitment of the promoter complex. In pulldown and co-immunoprecipitation assays RHA was found to interact directly with p100 protein. Both RHA and p100 have been implicated in regulation of gene expression through several mechanisms such as coupling transcription with RNA processing or DNA replication (39). Therefore, it is possible that this interaction may also serve other, yet unknown functions in these processes.
ACKNOWLEDGEMENTS
The authors thank Dr T. Nakajima for kindly providing reagents and helpful discussions, and Merja Lehtinen and Paula Kosonen for excellent technical assistance. This work was supported by grants from Medical Research Council of Academy of Finland, Medical Research Fund of Tampere University Hospital, Finnish Foundation for Cancer Research, Tampere Tuberculosis Foundation, Sigrid Juselius Foundation, National Natural Science Foundation of China (30300070), Tianjin Municipal Science and Technology Commission (043802811), NCET-04–0245 and Specialized Fund for the Doctoral Program of Higher Education (20040062003). Funding to pay the Open Access publication charges for this article was provided by the Academy of Finland.
Conflict of interest statement. None declared.
Comments