-
PDF
- Split View
-
Views
-
Cite
Cite
Martin Hetzer, Rudolf J. Schweyen, Manfred W. Mueller, DNA polymerization catalysed by a group II intron RNA in vitro, Nucleic Acids Research, Volume 25, Issue 9, 1 May 1997, Pages 1825–1829, https://doi.org/10.1093/nar/25.9.1825
- Share Icon Share
Abstract
The excised group II intron bI1 from Saccharomyces cerevisiae can act as a ribozyme catalysing various chemical reactions with different substrate RNAs in vitro. Recently, we have described an editing-like RNA polymerization reaction catalysed by the bI1 intron lariat that proceeds in the 3′→5′ direction. Here we show that the bI1 lariat RNA can also catalyse successive deoxyribonucleotide polymerization reactions on exogenous substrate molecules. The basic mechanism of the reaction involved interacting cycles between an alternative version of partial reverse splicing (lariat charging) and canonical forward splicing (lariat discharging by exon ligation). With an overall chain growth in the 3′→5′ direction, the 5′ exon RNAs (IBS1dN) were elongated by successive insertion of deoxyribonucleotides derived from single deoxyribonucleotide substitutions (dA, dG, dC or dT). All four deoxyribonucleotides were used as substrates, although with different efficiencies. Our findings extend the catalytic repertoire of group II intron RNAs not only by a novel DNA polymerization activity, but also by a DNA-DNA ligation capacity, supporting the idea that ribozymes might have been part of the first primordial polymerization machinery for both RNA and DNA.
Introduction
The discovery of RNAs with true enzymatic activities (ribozymes) (1,2) has provoked interest in exploring the catalytic potential of RNA. Meanwhile, various chemical reactions have been shown to be catalysed by members of the group I and group II intron ribozymes with substrates as different as RNA, DNA and even aminoacylated derivatives of RNA (3–13). These results have repeatedly been discussed in the light of the role of RNA in a presumed RNA world (10,12,14–19). Special interest has focused on RNA-catalysed polymerization activities of some introns, since such reactions might have been involved in the development of the first self-replicating systems (20).
Group II introns excise autocatalytically from pre-mRNAs through two separate transesterification reactions in vitro (21–24). In the first step, the 2′-OH group of the intron internal branch point adenosine attacks the 5′ splice site (5′ ss) to produce 5′ exon and intron-3′ exon intermediate RNA in lariat form. In the second step the 5′ exon attacks the 3′ ss resulting in exon ligation and release of the lariat intron RNA.
The released lariat RNA can catalyse the reversal of exon ligation (9) with different substrates, either composed of RNA, DNA or hybrid molecules containing both types of nucleic acids (11,12,25; Fig. 1A). Substrate specificity in reverse splicing is provided by intermolecular base pairing interactions (9,11). A sequence motif of 6 nt in the 5′ exon substrate RNA (IBS1, for intron binding site 1) that is complementary to an intron internal motif (EBS1, for exon binding site 1) (26) is important to activate the phosphorous atom 3′ adjacent to the IBS1 motif for nucleophilic attack by the 3′-terminal hydroxyl group of the lariat- IVS (intervening sequence) RNA (11). This transesterification reaction represents reversal of the exon ligation reaction and leads to a charged lariat (Fig. 1A). The 3′ exon sequences can be functionally substituted by deoxyribonucleotides, mononucleotides and even single phosphate groups (11). The charged lariat itself can be discharged (i.e. exon ligation) and the 3′ exon part is transferred to the 5′ exon (or a 5′ exon substitute), leading either to ligated exons or to recombined RNA/DNA molecules (11,12; Fig. 1A). On the basis of alternating charging and discharging reactions, the catalytic group II ribozyme repertoire exhibits different activities, such as a 3′-terminal transferase, RNA-DNA and DNA-RNA ligase, monophosphotransferase and general recombinase (11,12).
An alternative form of IVS charging has been reported for lariat bI1 RNA, derived from the first intron of the mitochondrial COB gene of Saccharomyces cerevisiae. In this case the phosphorous atom 5′ adjacent to the canonical 5′ ss (IBS1, GACAGpA) becomes transesterified by the 3′ oxygen atom of the lariat-IVS [‘(−1) charging’] (11). As a consequence, the 5′ exon RNA is dissected [5′ exon (−1)] and the 3′-terminal nucleotide (pA) is transferred to the 3′-terminus of the lariat-IVS (Fig. 1A). Interacting cycles of aberrant charging combined with canonical exon ligation with full-length 5′ exons lead to progressive addition of RNA monomers in the 3′→5′ direction (25).
In recent studies, deoxyribonucleotide substitutions have been used to analyse the role of certain 2′-OH groups in the catalysis of group II ribozymes (27,28). The experimental approach of the work presented here was designed to analyse the capacity of group II intron bI1 to catalyse polymerization reactions with DNA monomers. According to the RNA polymerization pathway (11), we speculated that 5′ exons with 3′-terminal deoxyribonucleotide substitutions should be processed at the (−1) position in the lariat-catalysed charging reaction, as well as in the exon ligation step. We show that the wild-type bI1 intron catalysed extension reactions at the 3′-terminus of different substrate RNAs with all four deoxyribonucleotides, dA, dT, dC and dG. Furthermore, in trimolecular splicing assays using two different substrates the bI1 lariat RNA generated chimeric RNA molecules with mixed deoxyribonucleotide insertions. These findings extend the catalytic repertoire of group II introns to include DNA-DNA ligation activities and, consequently, a new DNA polymerase function proceeding in the 3′→5′ direction. The presented data provide evidence for a role of ribozymes as part of the first primordial polymerization machinery for both RNA and DNA.
![Pathways for exon ligation (discharging) and reversal of exon ligation (charging). Excised intron RNAs (lariat-IVS) bind 5′ exon substrate molecules (grey and yellow boxes, respectively) containing an IBS1 sequence motif (5′-GACAGA) by base pairing with an intron internal EBS1 site (5′-CUGUCU). Phosphodiester bonds used in transesterifications are marked. (A) IVS-catalysed recombination. Lariat-IVS becomes charged with a 3′ exon sequence (blue box) by reversal of exon ligation [the authentic 3′ exon can be substituted by DNA, mononucleotides and even single phosphate (12)]. The charged lariat-IVS can again select different 5′ exon substrates, leading to recombinant molecules (yellow-blue) by exon ligation. (B) Predicted model for intron RNA-catalysed deoxynucleotide insertion. In an alternative version of IVS charging (−1 charging) the 3′ terminal nucleotide of the 5′ exon (shown for a dG residue) is transferred together with the 3′ exon to the 3′-OH group of the lariat-IVS, resulting in a 5′ exon (−1) molecule. Subsequent discharging by an unprocessed 5′ exon substrate terminating in a dG residue leads to insertion of one dG residue. Alternating cycles of (−1) charging and discharging results in progressive dG insertion in the 3′→5′ direction (25).](https://oup.silverchair-cdn.com/oup/backfile/Content_public/Journal/nar/25/9/10.1093_nar_25.9.1825/2/m_25-9-1825-fig001.gif?Expires=1750212280&Signature=tu3UtMergmVyzXeDfPu99TQIwta6N6Biyd7GjZxrjFMCxOcM2CCP-cibEKRq64cszkf45LB8JExBZXJ2wu9yRNEuWwq9jq1yt3DnA8pVG3qxzANIpOs70eDM2cY3pRi5EhIJTnEYehRxwzE1vOEw99eyGTNdwNnPrARycQt81cKBDO-DHnSEWTHHP60VsL9-YGZb42oSGsYO5-Rq0p8Cf1N97fRzKG1ApNnUkNkphxZBHuJyQgTk~GxiTBObc~5NCzdvXy6Cz5Ta1aUuFQnTuvw1mWd18sZLc7F1fgnILZU2fN9kn0MXyWrUDRCssTpjjtpdpu8uFMsd-XMA~xc-dA__&Key-Pair-Id=APKAIE5G5CRDK6RD3PGA)
Pathways for exon ligation (discharging) and reversal of exon ligation (charging). Excised intron RNAs (lariat-IVS) bind 5′ exon substrate molecules (grey and yellow boxes, respectively) containing an IBS1 sequence motif (5′-GACAGA) by base pairing with an intron internal EBS1 site (5′-CUGUCU). Phosphodiester bonds used in transesterifications are marked. (A) IVS-catalysed recombination. Lariat-IVS becomes charged with a 3′ exon sequence (blue box) by reversal of exon ligation [the authentic 3′ exon can be substituted by DNA, mononucleotides and even single phosphate (12)]. The charged lariat-IVS can again select different 5′ exon substrates, leading to recombinant molecules (yellow-blue) by exon ligation. (B) Predicted model for intron RNA-catalysed deoxynucleotide insertion. In an alternative version of IVS charging (−1 charging) the 3′ terminal nucleotide of the 5′ exon (shown for a dG residue) is transferred together with the 3′ exon to the 3′-OH group of the lariat-IVS, resulting in a 5′ exon (−1) molecule. Subsequent discharging by an unprocessed 5′ exon substrate terminating in a dG residue leads to insertion of one dG residue. Alternating cycles of (−1) charging and discharging results in progressive dG insertion in the 3′→5′ direction (25).
Materials and Methods
RNA synthesis
Run-off transcripts from bI1 were synthesized in vitro with T3 RNA polymerase in the presence of [α-35S]UTP from EcoRIdigested plasmids Bluescript and bI1Δ24 as described (29). Pre-RNAs were gel purified and incubated in 40 mM Tris-HCl, pH 7.2, 60 mM MgCl2, 2 mM spermidine and 660 mM NH4Cl at 45°C for 60 min. The splicing product bI1 lariat-IVS RNA was quantitatively recovered from preparative polyacrylamide gels.
Synthetic oligoribonucleotides were synthesized on an Applied Biosystems DNA/RNA Synthesizer (392). 5′-End-labelling and purification were performed as described (11). Oligonucleotides: IBS1dA, 5′-AAAGGAAGACAGdA-3′; IBS1dG, 5′-AAAGGAAGACAGdG-3′; IBS1dT, 5′-AAAGGAAGACAGdT-3′; IBS1dC, 5′-AAAGGAAGACAGdC-3′; IBS1dGpA8, 5′-AAAGGAAGACAGdGAAAAAAAA-3′.
DNA polymerization and insertion reactions
For DNA polymerization 5′ terminally [γ-32P]ATP-labelled synthetic 5′ exon substrates IBS1dN (5 µM) labelled at the 5′-end and lariat-IVS bI1 (0.5 µM) were incubated in 40 mM Tris-HCl, pH 7.2, 60 mM MgCl2, 2 mM spermidine and 1.25 M NH4Cl at 45°C. Samples were taken for different times as indicated, analysed and quantified (PhosphorImager; Molecular Dynamics).
For DNA insertion synthetic 5′ exon substrates (IBS1dG) labelled at the 5′-end with [γ-32P]ATP and lariat-IVS bI1 were co-incubated as described above with substrate IBS1dGpA8 (1 µM). The products of the deoxyribonucleotide integration reaction were gel purified and subjected to alkaline hydrolysis or analysed by dideoxy sequencing.
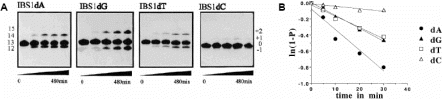
(A) Disproportionation of *pN7GACAGpdN RNA substrates (13mers) with single 3′-terminal deoxyribonucleotide substitutions (dN = dG, dA, dT or dC) in the presence of bI1 lariat intron RNA. Aliquots of 10 µM 32P-end-labelled 5′ exon derivatives *pN7GACAGpdN were incubated with 0.5 µM lariat-IVS in 40 mM Tris-HCl, pH 7.5, 60 mM MgCl2, 5 mM spermidine and 1.25 M NH4Cl, pH 7.5, at 45°C. Time is shown beneath each gel; numbers at the side of the gels indicate the corresponding length of reaction products. The input substrates were converted to labelled products one nucleotide shorter (−1) and to products extended in length by one or two nucleotides (+1 and +2). (B) Effect of different deoxyribonucleotide substitutions at the 5′ exon position (−1) on the the rate of substrate decrease. Rates were measured under reaction conditions described above. The natural logarithm of the fraction unreacted was plotted against time (P = fraction reacted at each time point).
Alkaline hydrolysis
Reaction products containing 5′-end-labels were incubated in hydrolysis buffer (0.5 M sodium bicarbonate/carbonate, pH 9.2) for 15 min at 90°C and analysed on denaturing 20% polyacrylamide gels.
Dideoxy sequencing of polymerization product
*pN7GACAGpdG2dG1A8 22mer (cf. Fig 3A) was gel extracted and tagged by virtue of the 3′-terminal OH group to the 5′ phosphate group of an EcoRI site-containing a DNA adapter molecule in the presence of T4 RNA ligase (38). The 3′-OH group of an adapter-specific primer was used to initiate cDNA synthesis, followed by CRTC (controlled ribonucleotide tailing of cDNA ends) (39) and covalent ligation of a KpnI site-containing DNA adapter to the 3′-OH group of the elongated cDNA product in the presence of T4 DNA ligase. PCR amplification was performed with adapter-specific primers. Dideoxy sequencing after a cloning step, utilizing restriction sites derived from the DNA adapters (KpnI and EcoRI), was performed on an automated DNA sequencer (LI-COR L4000; VBC Sequencing Service) as described (39).
Results and Discussion
In a first step towards group II intron-catalysed DNA polymerization, we synthesized oligoribonucleotides (13mers) containing single deoxyribonucleotide substitutions (IBS1dN; dN = dA, dG, dC or dT) at the 3′-terminal IBS1 motif (*pN7GACAGdN). The four different 5′ exon substrates were assayed in the presence of gel-purified bI1 lariat RNA under splicing conditions at 45°C (Fig. 2A). All four 32P-5′-end-labelled input molecules (*pN7GACAGpdN) were progressively converted into products 1 nt shorter at the 3′-end [12mer (−1), *pN7GACAG], indicating that all four deoxyribonucleotides were accepted for the (−1) charging reaction. Additionally, products extended by one or more deoxyribonucleotides in length were observed in all four assays [e.g. 14mer (+1), *pN7GACAGdA2dA1].

The lariat-IVS is regenerated in the reaction and can catalyse multiple cycles of alternative charging and accurate discharging. As can be seen with substrate IBS1dA, at least three cycles could be detected, resulting in 3 nt extensions. According to the disproportionation, the ratio of extension products (+dN) to dissected [IBS1(−1)] products was expected to be GACAG+dN/GACAG = 1. When compared with the RNA polymerization reaction (25), more of the dissected product [IBS1(−1)] was detected using deoxyribonucleotide substitutes; 0.7 in the case of substrates with non-authentic IBS1/EBS1 base pairing (e.g. *pN7GACAGdG and *pN7GACAGdT) compared with wild-type *pN7GACAGdA (0.5). One explanation could be that the discharging reaction (2′-H) is slower than the (−1) charging reaction (2′-OH), resulting in accumulation of *pN7GACAG product. We cannot determine if this 2′-H effect is due to reduced binding affinity caused by IBS1/EBS1 duplex destabilization or if the 2′-OH group at the terminal IBS1 nucleotide directly affects the chemical rate of the discharging reaction. In previously described RNA extension reactions catalysed by lariat bI1 (25), extension products of up to six ribonucleotides were detected in the case of (GACAGrA). The corresponding deoxyribonucleotide substitution (dA) used in this study resulted in three deoxyribonucleotide extensions. In all other cases (T, G and C) ribo- and deoxyribonucleotide extensions were in the same range (∼+1 to +2). From this we conclude that the 2′-H substitution at the 5′ exon terminus has only a moderate effect on the efficiency of the charging and discharging reactions and seems not to be directly involved in catalysis. This is consistent with results derived from group II intron aI5c ribozyme-catalysed cleavage reactions with DNA substrates, where the 2′-OH group at position (−1) made only a minor contribution to the rate of chemical cleavage (30).

Intron-catalysed insertion of deoxyribonucleotides. (A) 32P-5′-End-labelled 5′ exon *pN7GACAGpdG (13mer; 5 µM) was co-incubated with non-labelled pN7GACAGpdGpA8 (21mer; 10 µM) in the presence of 1 µM lariat-IVS for different times as indicated. Numbers at the side of the gel indicate the respective length of fragments. (B) Alkaline hydrolysis of reaction products. Integrated deoxyribonucleotides in labelled products (*pN7GACAGpdGA8 and *pN7GACAGpdGpdGA8) resulted in OH-resistance. In contrast, product *pN7GACAGpA8 (20mer) generated in exon ligation performed by 5′ exon (−1) molecule gave a complete OH-ladder. (C) Dideoxy sequencing. Reaction products labelled with 32P and corresponding in size to a 22mer (*pN7GACAGpdGpdGA8) were subjected to dideoxy sequencing as described in Materials and Methods (38,39).
The nature of the extended deoxyribonucleotides was confirmed by trimolecular experiments with lariat-IVS and two exogenous substrate RNAs (Fig. 3A). The first molecule (21mer, N7GACAGpdGA8) contained the IBS1 motif 5′ adjacent to a run of eight adenosine residues (A8). This artificial ligated exon substrate provides two potential target sites for nucleophilic attack by the lariat-IVS; the original ligation junction and the (−1) position within the IBS1 motif. Co-incubation of non-labelled N7GACAGpdGA8 with lariat-IVS and an excess of 32P-5′-end-labelled 13mer (*pN7GACAGpdG) yielded oligonucleotidylic acids with the electrophoretic mobility of a 22mer (*pN7GACAGpdG2dG1A8). This product is consistent with the DNA integration activity involving (−1) charging and subsequent discharging. Other products can be explained as the result of different charging/discharging combinations. For instance, the 20mer *pN7GACAGpA8 seemed to be generated by correct charging and subsequent ligation to (−1) *pN7GACAG molecules. Previously we have shown that 5′ exons lacking the terminal IBS1 nucleotide are indeed able to perform the second step of splicing (25).
When the reaction products were subjected to alkaline hydrolysis the inserted deoxyribonucleotides resulted in hydrolysis-resistant bands corresponding to their predicted integration site 3′ of the IBS1 GACAGdG motif (Fig. 3B). A more direct confirmation of the nature of the polymerization product (*pN7GACAGpdG2dG1A8, 22mer) was by dideoxy sequencing (Fig. 3C).
Both successive addition of all four deoxyribonucleotides at the 3′-terminus of the *pN7GACAGpdN substrates (Fig. 2) and insertion of deoxyribonucleotides within the substrate *pN7GACAGpdGA8 (Fig. 3) are consistent with the predicted model for DNA polymerization as illustrated in Figure 1B. From this we propose that the described extension reaction with deoxyribonucleotide substitutions at the 3′-end of IBS1-containing substrate RNAs uses the same set of transesterification steps predicted for the RNA polymerization reaction and proceeds in the 3′→5′ direction.
The presented data extend previous findings on bI1-catalysed forward and reverse exon ligation reactions with RNA and DNA analogues (12,25). Mörl et al. (12) demonstrated that a 5′ exon entirely composed of DNA was able to perform exon ligation to a 3′ exon RNA. The efficiency of this reaction was reduced 18-fold compared with reactions with ribonucleotide exons (12). In further studies they showed that bI1 lariat RNA could also cleave single-strand DNA substrates with an internal IBS1 sequence motif.
According to our experimental data, the 3′-terminal deoxyribonucleotide exerts a nucleophilic attack on the 3′ splice site of a lariat-IVS charged with a 3′ exon DNA, which is the first description of DNA-DNA ligation performed by a group II intron.
Lariat-catalysed reactions with DNA substrates may have implications for their catalytic role in vivo. It has been suggested that excised group II introns can directly bind and at least partially reverse splice into single-stranded DNA and thereby possibly migrate into new genetic locations (12,31,32). It is tempting to speculate that mobile group II introns might have additional functions in cellular or mitochondrial metabolism by circumventing the rigid specificity for only one type of nucleic acid. Since an accessible IBS1 motif, consisting of six ribo- or deoxyribonucleotides, seems to be the only requirement for a molecule to become an ‘exon’, there should be many possible cleavage or recombination sites within group II-containing genomes.
Taken together, these findings emphasize the catalytic flexibility of group II introns as potential recombinogenic mediators between the RNA and DNA worlds. Polymerization reactions catalysed by introns (including those for group I introns) in general have been widely interpreted in terms of participation in primordial nucleotide synthesis and replication (15,17,33,34). Our results suggest that such ‘primitive polymerases’ might have possessed rather broad substrate specificities, producing mixed RNA-DNA molecules composed of interspersed ribo- and deoxyribonucleotides (Fig. 1B). The generation of ribozymes with an increased amount of DNA could have been beneficial, since it confers greater stability to hydrolysis (35,36). It has been shown that DNA-containing ribozymes do not necessarily have reduced catalytic properties; even complete deoxymetalloenzymes generated in vitro could ligate DNA substrates (37). We suggest that a potential role of intron RNAs as the first ‘polymerases’ in the RNA world was to assist in the gradual progression of genetic templates from single-stranded RNA to DNA.
Comments