-
PDF
- Split View
-
Views
-
Cite
Cite
Weili Wang, Lanlan Meng, Jiaxin He, Lilan Su, Yong Li, Chen Tan, Xilin Xu, Hongchuan Nie, Huan Zhang, Juan Du, Guangxiu Lu, Mengcheng Luo, Ge Lin, Chaofeng Tu, Yue-Qiu Tan, Bi-allelic variants in SHOC1 cause non-obstructive azoospermia with meiosis arrest in humans and mice, Molecular Human Reproduction, Volume 28, Issue 6, June 2022, gaac015, https://doi.org/10.1093/molehr/gaac015
- Share Icon Share
Abstract
Meiosis is pivotal to gametogenesis and fertility. Meiotic recombination is a mandatory process that ensures faithful chromosome segregation and generates genetic diversity in gametes. Non-obstructive azoospermia (NOA) caused by meiotic arrest is a common cause of male infertility and has many genetic origins, including chromosome abnormalities, Y chromosome microdeletion and monogenic mutations. However, the genetic causes of the majority of NOA cases remain to be elucidated. Here, we report our findings of three Shortage in chiasmata 1 (SHOC1) bi-allelic variants in three NOA patients, of which two are homozygous for the same loss-of-function variant (c.231_232del: p.L78Sfs*9), and one is heterozygous for two different missense variants (c.1978G>A: p.A660T; c.4274G>A: p.R1425H). Testicular biopsy of one patient revealed impairment of spermatocyte maturation. Both germ-cell-specific and general Shoc1-knockout mice exhibited similar male infertility phenotypes. Subsequent analysis revealed comprehensive defects in homologous pairing and synapsis along with abnormal expression of DMC1, RAD51 and RPA2 in Shoc1-defective spermatocyte spreads. These findings imply that SHOC1 may have a presynaptic function during meiotic recombination apart from its previously identified role in crossover formation. Overall, our results provide strong evidence for the clinical relevance of SHOC1 mutations in patients with NOA and contribute to a deeper mechanistic understanding of the role of SHOC1 during meiotic recombination.
Introduction
Azoospermia, including obstructive azoospermia (OA) and non-obstructive azoospermia (NOA), refers to a complete lack of spermatozoa in the ejaculate. NOA affects ∼0.6% men in general and 10–20% infertile men (Wosnitzer et al., 2014). Three types of NOA have been identified according to variations in testicular histology: Sertoli cell-only syndrome, maturation arrest (MA) at a specific developmental stage (such as spermatogonia, spermatocyte or spermatid arrest), and hypospermatogenesis (Ezeh et al., 1998). Notably, the incidence of genetic disorders, such as chromosome abnormalities and Y chromosome microdeletion, is as high as 45% in patients with MA (Hung et al., 2007). However, the etiology of the large remaining percentage of infertile individuals remains unclear. In recent decades, a subset of single-gene variants has been identified as potentially responsible for MA, and these include DMC1 (He et al., 2018), STAG3 (Riera-Escamilla et al., 2019), MEI1 (Ben Khelifa et al., 2018), TEX11 (Yatsenko et al., 2015), TEX15 (Okutman et al., 2015), XRCC2 (Zhang et al., 2019b) and MEIOB (Gershoni et al., 2019). Considering that known genetic defects in more than 400 genes affect mouse fertility (Matzuk and Lamb, 2008), the discovery of the underlying genetic etiology of NOA remains a major task. Moreover, the clinical implications of the identified causative genes are still unclear owing to the small sample sizes involved in single center or sporadic reports.
Meiosis refers to the process comprising a single round of DNA replication of a diploid germ cell followed by two rounds of chromosome segregation to produce haploid gametes. To ensure proper segregation of homologous chromosomes with maternal and paternal origins during meiosis I, cells undergo a succession of coordinated molecular and cellular events, one of which is homologous recombination (Baudat et al., 2013). The process of homologous recombination is initiated by the formation of DNA double-strand breaks (DSBs), which is then proceeded by their repair, resulting in reciprocal exchange between homologous DNA segments in a process termed as crossover (Li et al., 2021; Wang et al., 2021). These recombination-mediated processes occur in physical and functional linkage with a highly conserved meiosis-specific structure, the synaptonemal complex (SC) (Zickler and Kleckner, 2015). A group of proteins termed ‘ZMM’ (ZIP1, ZIP2, ZIP3, ZIP4, MSH4, MSH5, MER3 and SPO16) is functionally related to the formation of crossovers and SCs in budding yeast, mammals and plants (Zhang et al., 2017, 2019a). Mutations in ZMM-related genes lead to defects in the formation of both crossovers and SCs, resulting in zipper-like structures between homologous pairs. However, it is still not clear how these ZMM proteins coordinate with other proteins or whether they have functions apart from SC assembly and crossover formation during meiotic recombination.
ZIP2 is an important meiotic protein found in yeast, and it has been identified as forming a complex with ZIP4 and SPO16 to promote the formation of crossovers (De Muyt et al., 2018). When ZIP2 is defective, the patterns of one recombination intermediate, the double Holliday junction, and the subsequent formation of normal SCs are disrupted, along with a remarkable reduction in crossovers. ZIP2 shows a high structural similarity (possesses a predicted XPF domain) to Arabidopsis Shortage in chiasmata 1 (SHOC1), human C9ORF84 and mouse SHOC1 (also named AI481877 or MZIP2), indicating that they are possible orthologs. SHOC1 mutant plants show impaired meiosis at diakinesis with reduced numbers of chiasmata, affecting interference-sensitive crossover formation (Macaisne et al., 2008). Spermatogenesis is arrested at metaphase I or zygotene stage in Shoc1 mutant mice with incomplete or complete ablation of SHOC1 (Guiraldelli et al., 2018; Zhang et al., 2018), indicating phenotypic divergence among Shoc1 mouse models. Recently, different degrees of meiotic arrest phenotypes were also observed in patients with bi-allelic SHOC1 loss-of-function variants (Krausz et al., 2020; Yao et al., 2021). Considering that SHOC1 is highly conserved between humans and mice, exploring the phenotypic properties and differences is particularly important for understanding the function of SHOC1 during meiosis.
Here, we performed a genetic study using whole-exome sequencing (WES) for 279 individuals with NOA, and bi-allelic SHOC1 variants were detected. We also generated mouse models of Shoc1 to understand its function during meiosis. Our results provide novel insights into the functional consequences of SHOC1 defects and reveal the crucial role of SHOC1 in meiotic recombination.
Materials and methods
Human participants
A cohort of 279 NOA-affected Chinese men were recruited from the Reproductive and Genetic Hospital of CITIC-Xiangya (Changsha, China). All participating individuals showed a normal karyotype (46; XY) without Y chromosome microdeletions. Semen analysis of the participating individuals was performed at least twice in accordance with the fifth WHO guidelines and revealed no spermatozoa within the standard volume as per the fifth WHO guidelines (Cooper et al., 2010). All participating individuals provided informed consent, and the study was conducted in accordance with the institutional ethics committees of the Reproductive Genetic Hospital of CITIC-Xiangya (LL-SC-2017) and Central South University (Changsha, China).
Whole-exome sequencing and bioinformatics analysis
Genomic DNA (gDNA) was isolated from peripheral blood samples using a QIAamp® DNA Blood Midi kit (Qiagen, Hilden, Germany) according to the manufacturer’s protocol. gDNA was subjected to WES performed by the Beijing Genome Institute at Shenzhen (BGI) on the HiSeq2000 sequencing platform (Illumina Inc., San Diego, CA, USA), as described previously (Zhu et al., 2016). WES data analysis was performed using the Genome Analysis Toolkit. Briefly, the WES raw reads after removing adaptors were aligned to the human genome (GRCh37/hg19) using the Burrows-Wheeler Aligner (Li and Durbin, 2009), followed by removal of the PCR duplicates and sorting using Picard (http://broadinstitute.github.io/picard/). Variant identification was performed using the Genome Analysis Toolkit package (McKenna et al., 2010) following the recommended best practices, including base recalibration variant calling with Haplotype Caller, variant quality score recalibration and variant annotation using the ANNOVAR software.
Candidate causative variants that matched the following criteria were selected: (i) frequency below 5% in public human databases (1000 Genomes variant database and gnomAD); (ii) predicted to be deleterious variants according to multiple bioinformatics tools; (iii) prioritized consideration of homozygous variants; and (iv) relevancy for infertile phenotype using comprehensive expression data (expression in testes) (GTEx Consortium, 2015), gene ontology terms (biological process associated with spermatogenesis) (Ashburner et al., 2000), and model organism data (a male infertility phenotype presented in animal models) (Eppig et al., 2015). The SHOC1 variants identified by WES were further validated by Sanger sequencing using the following primers: M1-F 5′-CAATTACAAGAATGGTGACCCAGA-3′, M1-R 5′-TACTGCCCTAATGTGGCAAAGC-3′; M2-F 5′-AGCTCCCATTATTAGGCAGATT-3′, M2-R 5′-GATGACAGTGTTAAGGACAATTTCT-3′; M3-F 5′-AGAAAATTGAAACCAAGGGAGA-3′, M3-R 5′-CTGGGTTGCAAATATTTCCTCT-3′.
Mouse models
Shoc1 conditional knockout (cKO) mice were generated using homologous recombination by CRISPR/Cas9-mediated genome editing technology in zygote carried out by Shanghai Model Organisms Center, Inc. (Shanghai, China). Briefly, Cas9 mRNA and single guide (sg) RNAs (sgRNA-1: CCCATTATCCACAGCTCAGTAGG, sgRNA-2: AACTCCATCTCACATATATTAGG) were obtained by invitro transcription. In the targeted allele, exon 5 of Shoc1 was flanked by loxP sites (referred to as Shoc1f). The donor vector was constructed through in-fusion cloning using an In-Fusion® HD Cloning Kit (TAKARA, 639650, Beijing, China). The donor vector contains a 3.0 kb 5'-homologous arm, a 0.7 kb loxP region and a 3.0 kb 3′-homologous arm. Then, Cas9 mRNA, gRNA and donor vector were microinjected into the fertilized oocytes of C57BL/6J mice (Strain Code: 219; Vital River Laboratories, Beijing, China) to obtain F0 generation mice. Positive F1 generation mice (Shoc1f/+) were achieved by mating F0 generation mice with C57BL/6J. The male germ cell-specific Shoc1 knockout mice (Shoc1 cKOStra8-Cre) and Sertoli cell-specific Shoc1 knockout mice (Shoc1 cKOAmh-Cre) were generated by breeding Shoc1f/f mice with Stra8-cre mice (a gift from Professor Wei Li, Institute of Zoology, Chinese Academy of Sciences, and the original strain is from the Jackson Laboratory [#008208, Bar Harbor, USA]) (Sadate-Ngatchou et al., 2008) or Amh-cre mice (purchased from Shanghai Model Organisms Center, Inc. [Shanghai, China], and the original strain is from the Jackson Laboratory [#007915, Bar Harbor, USA]) (Holdcraft and Braun, 2004). The founder mouse and its offspring were genotyped via PCR and Sanger sequencing of the tail gDNA using the following primers: P1 5′-CACCTCTCAACCAATCCTGTCC-3′, P2 5′-ATCTGTAGCCCCACCACTTGAAA-3′, used for detecting whether the loxP region is inserted (Supplementary Fig. S1a and b); P3 5′-CAACTCATGAACCACAAATAG-3′, P4 5′-GACAGTTCTCAGCAATCTT-3′, used for verifying the Cre recombinase activity in Amh or Stra8 expressed tissues (Supplementary Fig. S1a); Amh-F 5′-ATTTGCCTGCATTACCGGTCG-3′, Amh-R 5′-CAGCATTGCTGTCACTTGGTC-3′, used for detecting whether the Amh gene-directed Cre recombinase is positive; Stra8-F 5′-GTGCAAGCTGAACAACAGGA-3′, Stra8-R 5′-AGGGACACAGCATTGGAGTC-3′, used for detecting whether the Stra8 gene-directed Cre recombinase is positive. PCR products were analyzed by 2.0% agarose gel electrophoresis to determine band sizes. Moreover, we obtained a Shoc1 knockout (KO) mouse strain (Shoc1+/−) with exon 5 deletion from the offspring of Shoc1+/+, Shoc1f/+, Stra8-cre and Shoc1f/+, Amh-cre (Supplementary Fig. S1a). Adult mice (aged ≥ 6 weeks) were used in this study. All animal experiments were conducted in accordance with the guidelines approved by the Institutional Animal Care and Use Committee of the Central South University.
Histological and immunostaining analysis
For histological analysis, testes and epididymis were fixed in Bouin’s solution (Sigma-Aldrich, St. Louis, MO, USA) or 4% paraformaldehyde overnight at 4°C, and then embedded in paraffin blocks for sectioning. Subsequently, hematoxylin and eosin (H&E) staining was performed for histological analyses. Slides were immunostained as described previously (Wang et al., 2019). The sections were incubated with primary antibodies overnight at 4°C, followed by incubation with the secondary antibody. The nuclei were stained with 4,6-diamidino-2-phenylindole. Fluorescence signals generated as a result of the staining procedure were photographed using an Olympus IX51 fluorescence microscope (Olympus, Tokyo, Japan) and analyzed using the VideoTesT-FISH 2.0. The antibodies used in this study are listed in Supplementary Table S1.
Western blots
The proteins of mouse testicular tissues were extracted using RIPA lysis buffer (P0013B, Beyotime Biotechnology, Shanghai, China), supplemented with protease inhibitor cocktail (P1006, Thermo Fisher Scientific, Carlsbad, CA, USA) and denatured at 100°C for 10 min. The denatured proteins were separated by SDS-PAGE and transferred onto a polyvinylidene difluoride membrane (IPVH00010, Millipore, MA, USA). Membranes were blocked in 5% non-fat milk for 2 h at 20–25°C, and incubated overnight at 4°C with the following primary antibodies: rabbit polyclonal anti-SHOC1 antibody (ABclonal, 1:1000, specifically binding mouse amino acids 440-642, Wuhan, China), and anti-GAPDH. Then, the membranes were washed three times in Tris-buffered saline with Tween 20 and incubated with secondary antibodies for 1 h at 20–25°C. After washing three times with Tris Buffered Saline with Tween 20 (TBST), the blots were visualized using an electrochemical luminescence western blotting kit (WP20005, Thermo Fisher Scientific) on a Tanon Chemical Imaging Analyzer (4600SF, Tanon, Shanghai, China).
Spermatocyte spreads and immunostaining
Mouse testicular tissue was macerated in phosphate-buffered saline (PBS) solution, followed by removal of the tunica albuginea. The seminiferous tubules were separated and transferred to hypotonic buffer (0.6 mol/l Tris pH 8.2, 500 µl; 0.5 mol/l sucrose, 1 ml; 0.17 mol/l trisodium citrate dihydrate, 1 ml; 0.5 mol/l EDTA, pH 8.0, 100 µl, 0.5 mol/l DTT, 50 µl; 0.1 mol/l PMSF, 100 µl; ddH2O, 7.25 ml) for 30 min. After being homogenized in 100 mMol sucrose buffer (pH 8.2), the cell suspension was gently spread onto slides with fixative buffer (1% PFA and 0.15% Triton X-100), followed by incubation in a moist box for 12 h at 20–25°C. Subsequently, the slides were air-dried, washed with PBST three times (PBS containing 0.1% Triton X-100), and stained for immunofluorescence analysis. The slides were blocked with 5% bovine serum albumin and incubated with the primary antibody overnight at 4°C. Slides were then washed and incubated with Alexa Fluor 488- or 555-conjugated secondary antibodies and observed using an Olympus IX51 fluorescence microscope (Olympus, Tokyo, Japan), then analyzed using the VideoTesT-FISH 2.0 software. Semi-quantitative analysis of the fluorescence signals was conducted using the ImageJ software (NIH Imaging program) (Schneider et al., 2012). The progression of meiosis I prophase was recognized by co-staining SYCP1 and SYCP3 in the spermatocyte chromosome spreads of adult mice (three mice for each genotype), and subsequently analyzed by calculating the percentage of spermatocytes at individual substages (leptotene, zygotene, pachytene and diplotene).
TUNEL assay
Apoptotic germ cells from Shoc1f/f mice and Shoc1 cKOStra8-Cre male mice were detected using a terminal deoxynucleotidyl transferase-mediated dUTP nick end labeling (TUNEL) assay using the DeadEnd™ Colorimetric TUNEL System (Promega, Madison, WI, USA) according to the manufacturer’s instructions. Digital images were captured using the Olympus IX51 fluorescence microscope (Olympus, Tokyo, Japan) and analyzed using VideoTesT-FISH 2.0 software.
Statistical analysis
Statistical analysis was performed by Student’s t-test or one-way ANOVA using GraphPad Prism version 5.01 (GraphPad Software; San Diego, CA, USA). Data are presented as the mean ± SEM. Differences were considered significant when the P-value was <0.05 (*), 0.01 (**) or 0.001(***).
Results
Bi-allelic SHOC1 variants were identified in men with NOA
To investigate potential genes associated with NOA, WES and bioinformatics analyses were performed according to our established protocol (Tan et al., 2019). We identified three NOA-affected men from three unrelated Chinese families harboring bi-allelic SHOC1 variants (GenBank: NM_173521), accounting for 1.08% (3/279) of the cohort (Fig. 1a, Table I and Supplementary Table S2). Testicular biopsy tissues were obtained from individual F2: II-1. H&E staining revealed testicular impairment of spermatocyte maturation arrest (Fig. 1b). Individuals F1: II-2 and F2: II-1, from two unrelated consanguineous families, were shown to harbor the same homozygous frameshift variant (c.231_232del: p.L78Sfs*9) in SHOC1 (Fig. 1c). Individual F3: II-1, from a non-consanguineous family, harbored the compound heterozygous missense variants c.1978G>A: p.A660T and c.4274G>A: p.R1425H. These identified SHOC1 variants were rare or absent from public human databases, including the 1000 Genomes Project and gnomAD (v.2.1.1, 141 456 samples). These SHOC1 missense variants were also predicted to be deleterious via multiple bioinformatics tools, including PolyPhen-2, SIFT, MutationTaster and CADD (Table I). Subsequent Sanger sequencing confirmed that individuals F1: II-2 and F2: II-1 harbored the homozygous SHOC1 frameshift variant (c.231_232del: p.L78Sfs*9) (Fig. 1c), and individual F3: II-1 harbored the compound heterozygous missense variants (c.1978G>A: p.A660T and c.4274G>A: p.R1425H). Co-segregation analysis was not performed because of the lack of available DNA samples from the family members.
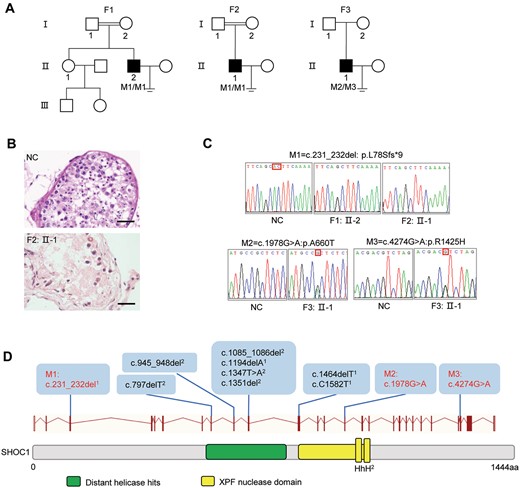
Bi-allelic SHOC1 variants in three Chinese men with NOA. (A) Pedigrees of families. Double horizontal lines represent consanguineous unions. Black-filled squares indicate male individuals with NOA. (B) Histologic section of the testes of patient F2: II-1 and normal control (NC) showing spermatogenesis up to the spermatocyte cell stage. (C) Sanger sequencing chromatograms of SHOC1. Homozygous SHOC1 frameshift variant (NM_173521: c.231_232del) and compound heterozygous missense variants (NM_173521: c.1978G>A; NM_173521: c.4274G>A) were confirmed. (D) Structure of the SHOC1 protein and positions of SHOC1 variants in this study and previous studies. Black variants, variants identified from other studies; red variants, variants identified in this study. 1Variants identified in the study of Yao et al. (2021). 2Variants identified in the study of Krausz et al. (2020). F, family; M, mutation.
SHOC1 variants . | M1 . | M2 . | M3 . |
---|---|---|---|
cDNA alteration | c.231_232del | c.1978G>A | c.4274G>A |
Protein alteration | p.L78Sfs*9 | p.A660T | p.R1425H |
Variant type | Frameshift | Missense | Missense |
rs ID | rs777595871 | rs755742775 | rs533026166 |
1000 Genomes Project | 0 | 0 | 0.000399361 |
East Asians in gnomAD | 0.0003853 | 0.0002178 | 0.0009526 |
All individuals in gnomAD | 0.00002870 | 0.00003377 | 0.00003191 |
SIFT | NA | Damaging | Damaging |
PolyPhen-2 | NA | Probably damaging | Possibly damaging |
MutationTaster | NA | Disease causing | Disease causing |
CADD | NA | 27.3 | 34 |
SHOC1 variants . | M1 . | M2 . | M3 . |
---|---|---|---|
cDNA alteration | c.231_232del | c.1978G>A | c.4274G>A |
Protein alteration | p.L78Sfs*9 | p.A660T | p.R1425H |
Variant type | Frameshift | Missense | Missense |
rs ID | rs777595871 | rs755742775 | rs533026166 |
1000 Genomes Project | 0 | 0 | 0.000399361 |
East Asians in gnomAD | 0.0003853 | 0.0002178 | 0.0009526 |
All individuals in gnomAD | 0.00002870 | 0.00003377 | 0.00003191 |
SIFT | NA | Damaging | Damaging |
PolyPhen-2 | NA | Probably damaging | Possibly damaging |
MutationTaster | NA | Disease causing | Disease causing |
CADD | NA | 27.3 | 34 |
NA, not available; M1–3, mutations 1–3; SHOC1, shortage in chiasmata 1.
NCBI reference sequence number of SHOC1 is NM_173521.
Variants with CADD values greater than 4 are considered to be deleterious.
SHOC1 variants . | M1 . | M2 . | M3 . |
---|---|---|---|
cDNA alteration | c.231_232del | c.1978G>A | c.4274G>A |
Protein alteration | p.L78Sfs*9 | p.A660T | p.R1425H |
Variant type | Frameshift | Missense | Missense |
rs ID | rs777595871 | rs755742775 | rs533026166 |
1000 Genomes Project | 0 | 0 | 0.000399361 |
East Asians in gnomAD | 0.0003853 | 0.0002178 | 0.0009526 |
All individuals in gnomAD | 0.00002870 | 0.00003377 | 0.00003191 |
SIFT | NA | Damaging | Damaging |
PolyPhen-2 | NA | Probably damaging | Possibly damaging |
MutationTaster | NA | Disease causing | Disease causing |
CADD | NA | 27.3 | 34 |
SHOC1 variants . | M1 . | M2 . | M3 . |
---|---|---|---|
cDNA alteration | c.231_232del | c.1978G>A | c.4274G>A |
Protein alteration | p.L78Sfs*9 | p.A660T | p.R1425H |
Variant type | Frameshift | Missense | Missense |
rs ID | rs777595871 | rs755742775 | rs533026166 |
1000 Genomes Project | 0 | 0 | 0.000399361 |
East Asians in gnomAD | 0.0003853 | 0.0002178 | 0.0009526 |
All individuals in gnomAD | 0.00002870 | 0.00003377 | 0.00003191 |
SIFT | NA | Damaging | Damaging |
PolyPhen-2 | NA | Probably damaging | Possibly damaging |
MutationTaster | NA | Disease causing | Disease causing |
CADD | NA | 27.3 | 34 |
NA, not available; M1–3, mutations 1–3; SHOC1, shortage in chiasmata 1.
NCBI reference sequence number of SHOC1 is NM_173521.
Variants with CADD values greater than 4 are considered to be deleterious.
Notably, SHOC1 is the human ortholog of Saccharomyces cerevisiae ZIP2 (8.7% similarity) and Arabidopsis thaliana SHOC1 (19.2% similarity) using the HHpred method (https://toolkit.tuebingen.mpg.de), which are essential for the formation of Class I crossovers during meiosis. Coincidently, bi-allelic SHOC1 mutations were reported to cause meiotic arrest and NOA during the period of our study (Krausz et al., 2020; Yao et al., 2021), which has strengthened our speculation that SHOC1 could be the cause of infertility in the probands examined here. Interestingly, the homozygous SHOC1 frameshift variant (c.231_232del: p.L78Sfs*9) in individuals F1: II-2 and F2: II-1 was also found in a previously reported patient with compound heterozygous variants in SHOC1 (c.C1582T and c.231_232del) (Fig. 1d). Given the recurring frameshift variant (c.231_232del) from our cohort, we compared the single nucleotide polymorphisms (SNPs) on chromosome 9 (where SHOC1 is located) using the WES data from individuals F1: II-2 and F2: II-1 and observed a high overlap of SNPs located 1 Mb upstream/downstream of SHOC1 (92/103, 89.32%, >10 sequencing depth) (Supplementary Table S3), suggesting a potential founder effect.
Shoc1 deficiency results in male infertility in mice
To further validate our findings and characterize the function of SHOC1 during spermatogenesis, we built Shoc1 knockout mouse models. Shoc1 is expressed during murine embryonic development, and a previous study reported embryonic lethality when completely deleting SHOC1 by targeting exons 2–20 (Guiraldelli et al., 2018). To overcome the obstacle of embryonic lethality and to determine whether Shoc1 deletion could induce germ-cell-autonomous defects, we generated a mouse model carrying a Shoc1 cKO allele (Shoc1f) using CRISPR/Cas9 technology. The sites of the frameshift mutation in human (exon3: c.231_232) corresponded to c.418_419 on exon 5 of mouse. We wanted to maximumly mimic this mutation in mouse under the condition of a high knockout efficiency, so we chose to abolish the whole exon 5 in mouse. Theoretically, the deletion of exon 5 will result in frameshift of the reading frame, leading to a premature translation stop at amino acid 90. This truncated peptide should not be functional because the two conserved helix-hairpin-helix (HhH)2 domains (amino acids 925–1156) were deleted. Importantly, Sertoli cell-specific Shoc1 cKO mice (Shoc1 cKOAmh-Cre) exhibited normal reproductive function with normal testicular development, spermatogenesis and fertility (Supplementary Fig. S2a and b), indicating that deletion of SHOC1 in Sertoli cells did not impair meiosis. In contrast, male germ cell-specific cKO mice (Shoc1 cKOStra8-Cre) displayed male infertility with significantly decreased testis weight (Fig. 2a–c). This suggests that the defect in spermatogenesis is germ cell dependent. Moreover, we obtained Shoc1 KO (Shoc1–/–) mice using the Shoc1 KO mouse strain (Shoc1+/−) (See Materials and Methods, Supplementary Fig. S1a). Surprisingly, Shoc1–/– offspring mice conformed to the Mendelian ratio (Supplementary Table S4), suggesting that Shoc1–/– embryos were not required for embryonic development. As the phenotypes caused by SHOC1 complete deletion were described in a previous study (Supplementary Fig. S2a and b) (Zhang et al., 2018), we used the germ cell-specific Shoc1 cKO mice (Shoc1 cKOStra8-Cre) for further investigation of SHOC1 functions during meiosis.
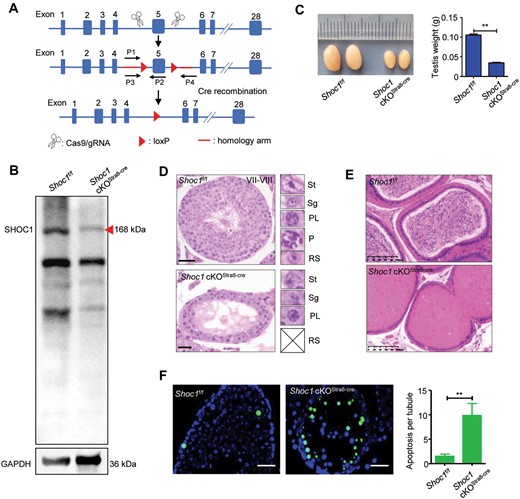
Shoc1 cKOStra8-Cre male mice exhibited NOA phenotype. (A) Red arrowheads show loxP sites on the Shoc1 cKOStra8-Cre targeting vector before and after exon 5. Red lines indicate homologous arms, and black arrows indicate where the primers were used for genotyping anneal. (B) Western blot analysis of Shoc1f/f and Shoc1 cKOStra8-Cre testis lysates. Red arrowhead shows dramatically decreased expression of the full-length SHOC1 protein. (C) Testes from Shoc1f/f and Shoc1 cKOStra8-Cre male mice (n = 3) at 10 weeks showing Shoc1 cKOStra8-Cre testes were significantly smaller than those from the Shoc1f/f testes (**P < 0.01, right). (D) Histology of Shoc1f/f and Shoc1 cKOStra8-Cre mouse testes. The insets on the right indicate different types of germ cells. No post-meiotic germ cells were observed in the Shoc1 cKOStra8-Cre mouse testes. P, pachytene spermatocyte; PL, pre-leptotene spermatocyte; RS, round spermatid; Sg, spermatogonia; St, Sertoli cell. (E) Histology of the cauda epididymis of Shoc1f/f and Shoc1 cKOStra8-Cre male mice. The absence of sperm was visualized in the cauda epididymis of Shoc1 cKOStra8-Cre male mice. Scale bar = 50 µm. (F) TUNEL assay on testes from Shoc1f/f and Shoc1 cKOStra8-Cre male mice. Green, TUNEL-positive cells. Nuclei were counterstained with DAPI (blue). Scale bar = 50 µm. cKO, conditional knockout.
We tested Shoc1 KO efficiency in Shoc1 cKOStra8-Cre mice by western blotting. The results showed that the protein level of SHOC1 was dramatically reduced in the testes of Shoc1 cKOStra8-Cre mice compared with Shoc1f/f mice (Fig. 2b, Supplementary Fig. S3). Histological analysis showed that the seminiferous tubules of Shoc1 cKOStra8-Cre mice lacked haploid round spermatids and elongated spermatids, indicating a meiosis arrest (Fig. 2d), and the same as observed in the Shoc1–/– mice (Supplementary Fig. S4a and b). We also stained seminiferous tubules for the mid-pachytene marker histone H1t. The results showed that there were almost no H1t-positive germ cells in Shoc1 cKOStra8-Cre mice (Supplementary Fig. S5), reflecting an arrest of spermatogenesis before mid-pachytene of meiosis I. Histological analysis of the epididymis also confirmed that there were no mature spermatozoa in Shoc1 cKOStra8-Cre mice (Fig. 2e). TUNEL analysis revealed a significantly increased apoptosis rate of primary spermatocytes in sections of Shoc1 cKOStra8-Cre testes compared to Shoc1f/f testes at the age of postnatal day 30 (Fig. 2f). Together, SHOC1 deletion could cause male infertility by arresting the spermatogenesis before mid-pachytene of meiosis I.
SHOC1 is required for homologous chromosome pairing and synapsis
During meiosis I prophase (consisting of leptotene, zygotene, pachytene and diplotene), changes in chromosome configurations and structure accompany recombination events. The pairing and synapsis of homologous chromosomes are mediated by the SC, where the two lateral elements are connected by the central elements SYCP1, SYCE1, SYCE3 and other promoting factors (Gao and Colaiacovo, 2018; Fan et al., 2021). We first stained the chromosome spreads of spermatocytes derived from Shoc1 cKOStra8-Cre and Shoc1f/f mice with SYCP1 and SYCP3 (a lateral element of SC). The linear co-staining of these proteins indicated full synapsis of autosomal bivalents in Shoc1f/f spermatocytes during pachytene. However, full assembly of SYCP1 was never seen in Shoc1 cKOStra8-Cre spermatocytes, indicating extensive asynapsis induced by SHOC1 deficiency (Fig. 3a). Abnormal pairing configurations of chromosomes were detected in Shoc1 cKO zygotene-like spermatocytes, including links between non-homologous chromosomes and the formation of multivalent chromosomes (Fig. 3b). Significantly reduced pairings between homologues were also observed in ∼40.5% (47/116) of the cKO spermatocyte spreads, characterized by casually distributed univalent, indicating a failure in homologous recognition and/or connection (Fig. 3c). We also noticed that although co-staining with SYCP3 suggested that SYCP1 was recruited to the axial elements on some homologous chromosomes, these co-staining axial elements were scarcely linked together and the homologous chromosomes remained separate (Fig. 3b). Moreover, the centromeric ends (CREST-positive, Fig. 3c) of the bivalents, which usually show delayed synapsis in normal spermatocytes (Roig et al., 2010), were more frequently unsynapsed in SHOC1 deficiency mice. These findings indicated that SHOC1 defect could lead to synapsis failure due to turbulence of homologous paring before entering pachytene stage and the massive unsynapsed regions are more likely to be asynaptic (where SC did not assemble at all) rather than desynaptic (where SC had formed but subsequently disassembled). According to the analysis of specific stages through meiotic I prophase (Fig. 3d), the meiosis of Shoc1 deficient spermatocytes was arrested at a zygotene-like stage because of synapsis defects.
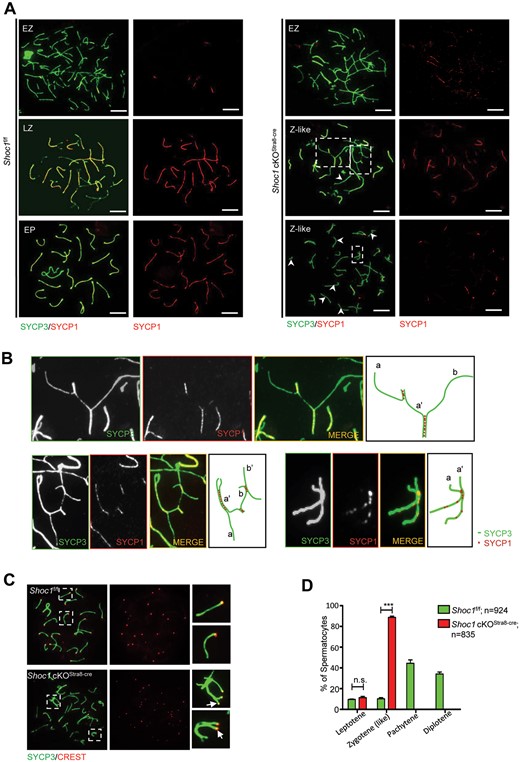
Spermatocytes of Shoc1 cKOStra8-Cre male mice displayed a zygotene-like arrest with severe synaptic defects. (A) Staining of the synapsed chromosome marker SYCP3 (green) with SYCP1 (red) on the chromosome spreads of spermatocytes derived from adult Shoc1f/f and Shoc1 cKOStra8-Cre males. Scale bar = 10 µm. EP, early-pachytene; EZ, early-zygotene; LZ, late-zygotene; Z-like, Zygotene-like. (B) Higher magnification of the panel in A. The right panel shows the asynapsed chromosomes. a–a’ and b–b’ represent homologous chromosomes. a’–b represents the pairing of non-homologous chromosomes. (C) CREST staining on the chromosome spreads of pachytene spermatocytes marks centromeres. White arrows indicate the unsynapsed pericentric ends. (D) Stage analysis of spermatocytes at meiosis I prophase from Shoc1f/f and Shoc1 cKOStra8-Cre mice. Random spermatocyte spreads from meiosis I prophase were analyzed from adult mice. A total of 924 spermatocytes from 3 Shoc1f/f mice and 835 cells from 3 Shoc1 cKOStra8-Cre mice were quantified. cKO, conditional knockout.
Meiotic DSBs failed to repair in Shoc1 cKO male mice
Meiotic recombination starts with the genetically programmed formation of DSBs in leptotene (Li et al., 2021). The phosphorylated form of the histone variant H2AX (γH2AX) is recruited to these DSBs (Fernandez-Capetillo et al., 2003). Single-stranded DNA (ssDNA) tails are resected and protected by the ssDNA-interacting complex comprising MEIOB, SPATA22 and the preformed RPAs (RPA1/2/3) (Luo et al., 2013) and are subsequently replaced by DNA strand exchange proteins (DMC1 and RAD51) to search for a homologous target (Neale and Keeney, 2006). This is followed by synapsis and highly regulated repair to promote the formation of at least one crossover per bivalent (Souquet et al., 2013).
Similar to the pattern of γH2AX in Shoc1f/f spermatocytes at leptotene and zygotene stages, the γH2AX marked chromatin domains surrounding DSBs in Shoc1-deficient spermatocytes at leptotene and zygotene-like stages (Fig. 4a). In the pachytene and diplotene Shoc1f/f spermatocytes, γH2AX staining localized exclusively to the XY body, which is the unpaired region of the X and Y chromosomes (Fig. 4a). However, the Shoc1 deficient spermatocytes never reached the pachytene stage and the γH2AX signal was retained on the chromosomes, indicating an accumulation of unrepaired DSBs (Fig. 4a). We then analyzed DSB formation by detecting RPA2 levels. Notably, the RPA2 foci of Shoc1-deficient spermatocytes were significantly higher than those of Shoc1f/f spermatocytes at the early zygotene stage, indicating an increase in DSBs (Fig. 4b). We then detected the foci of the DNA strand exchange proteins on the chromosomes. In control spermatocytes, the foci of DMC1 and RAD51 peaked at the early zygotene stage and dramatically decreased from late zygotene to pachytene, where sporadic expression was mainly retained on the XY chromosomes at pachytene (Fig. 4c and Supplementary Fig. S6). Shoc1-deficient spermatocytes contained comparable DMC1 and RAD51 foci to Shoc1f/f spermatocytes at the early zygotene stage (Fig. 4c and Supplementary Fig. S6). However, the zygotene-like Shoc1-deficient spermatocytes showed significantly higher levels of DMC1 and RAD51 foci compared with the late-zygotene control spermatocytes, and higher levels than the early- zygotene spermatocytes (Fig. 4c and Supplementary Fig. S6). The increases in DMC1 and RAD51 foci in Shoc1-deficient spermatocytes may have been caused by an increase in DSB production, as indicated by the elevated RPA foci, or by a delay in DSB repair.
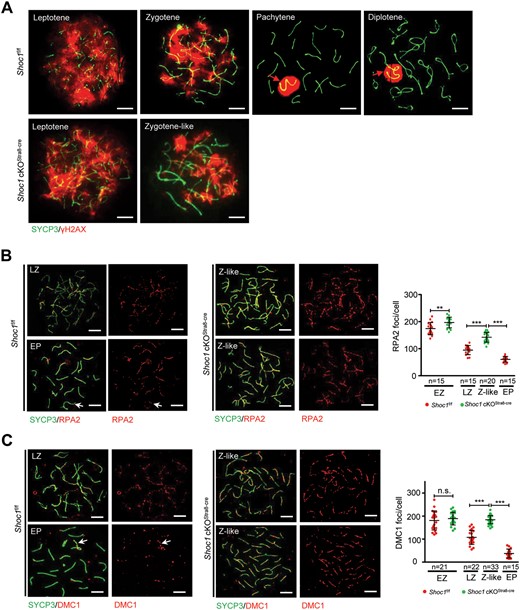
Spermatocytes of Shoc1 cKOStra8-Cre male mice exhibited defective meiotic recombination. (A) Representative chromosome spreads from adult Shoc1f/f and Shoc1 cKOStra8-Cre spermatocytes stained with SYCP3 (green) and γH2AX (red) antibodies. Scale bars = 10 µm. Red arrows indicate the sex chromosomes. (B) Chromosome spreads of adult Shoc1f/f and Shoc1 cKOStra8-Cre spermatocytes immunostained for SYCP3 (green) and RPA2 (red). Representative images are presented. Scale bar = 10 µm. Quantification of the foci numbers of RPA2 in adult Shoc1f/f and Shoc1 cKOStra8-Cre spermatocytes are shown on the right. EP, early-pachytene; EZ, early-zygotene; LZ, late-zygotene; Z-like, zygotene-like. (C) Detection of recombinase DMC1 in chromosome spreads of adult Shoc1f/f and Shoc1 cKOStra8-Cre spermatocytes by immunostaining with antibodies against SYCP3 (green) and DMC1 (red). Representative images are presented. The white arrow indicates XY chromosomes. Scale bar = 10 µm. Quantification of the foci numbers of DMC1 in adult Shoc1f/f and Shoc1 cKOStra8-Cre spermatocytes are shown on the right. Statistical analysis was performed using two-tailed Student’s t-tests: **P < 0.01; ***P < 0.001; n.s., not significant. Error bars indicate SEM. n: number of spermatocytes analyzed from three independent experiments. Spermatocyte spreads for these experiments were obtained from three mice for each genotype. cKO, conditional knockout.
Single end invasion causes the displacement of one strand of the homologue, resulting in the formation of D-loop (Szostak et al., 1983). The functional ssDNA-interacting complex (MEIOB-SPATA22-RPAs) could protect this D-loop and subsequent recombination intermediates (Xu et al., 2017; Ribeiro et al., 2021). We noticed that the RPA2 signal of Shoc1f/f spermatocytes was reduced at the late-zygotene stage and the pachytene stage (Fig. 4b). The difference between DMC1 and RAD51 is that RPA2 maintains a fair level of expression on the autosomes and XY chromosomes in the pachytene stage, indicating that the RPA-related complex may still function in the second end capture after strand invasion and stabilize the middle recombination intermediates for crossover insurance (Ribeiro et al., 2021). However, the zygotene-like Shoc1-deficient spermatocytes showed higher levels of RPA2 than the late-zygotene and early pachytene spermatocytes of the control (Fig. 4b). These results indicate that the RPA-related complex may have accumulated at the unformed/unstable middle recombination intermediates in collaboration with the functions of DMC1 and RAD51 due to the depletion of SHOC1. Together, these results showed failure of DSB repair and abnormal recruitment of DMC1, RAD51 and RPA2 to DSBs in Shoc1-deficient spermatocytes. Our results indicated that SHOC1 might play two distinct roles in meiotic recombination: a necessary role in homologous target and synapsis at early stages and an important role in promoting crossover formation at later stages (Fig. 5).

A schematic diagram showing a proposed model of SHOC1 in meiotic recombination during meiotic I prophase. SHOC1 may be recruited to the resected single strand along with the ssDNA-interacting complex (comprising MEIOB, SPATA22 and RPAs) and the DNA strand exchange protein (including RAD51 and DMC1), which is required for the homology search and synapsis. Subsequently, SHOC1 may also function in the protection of recombination intermediates (such as D-loop and unligated dHJ) from dissociation. The deletion of SHOC1 in Shoc1 cKOStra8-Cre could result in synaptic defects (such as pairing of non-homologous chromosomes) and failure of crossover formation during meiotic I prophase. cKO, conditional knockout; CO, crossover; DSB, DNA double-strand break; dHJ, double Holiday junction; NCO, non-crossover; SDSA, synthesis dependent strand annealing.
Discussion
In this study, we identified a homozygous frameshift variant and two novel missense variants of SHOC1 in males from three unrelated Chinese families. We also uncovered a possible founder effect of the frameshift variant (c.231_232del: p.L78Sfs*9), which has now been identified in three unrelated Chinese families (two in this study and one in a previous study) (Yao et al., 2021). Therefore, we propose that a complete screening of SHOC1 might be beneficial for the clinical evaluation of NOA men. Our findings also highlight the essential role of SHOC1 during homologous pairing and synapsis in mice, apart from its established role in crossover formation.
Based on the histological and pathological analysis in recent studies, an early MA (spermatocyte arrest) subtype of NOA was indicated in humans with SHOC1 loss-of-function mutations, suggesting that microsurgical testicular sperm extraction may be unnecessary for these patients. Interestingly, the nature of meiotic arrest exhibited heterogeneity among SHOC1 mutant patients. Three patients demonstrated severe zygotene arrest with no formation of the XY body (Yao et al., 2021), which was similar to our germ-cell specific cKO model and to KO models arrested at a zygotene-like stage (Zhang et al., 2018). However, a previous study showed that ∼4.2% of spermatocytes in one patient with a homozygous SHOC1 deletion variant (c.797delT; p.L266Qfs*6) could form the XY body, thus passing through the pachytene checkpoint and developing further into metaphase I, as observed in the hypomorph Shoc1 model (Yao et al., 2021). These subtle phenotypic variances may be caused by the degree of damage to functional SHOC1 truncation and/or isoform formation in vivo, or by differences in individual tolerance for variants due to epigenetics or other protective mechanisms.
Phenotypic divergence among Shoc1 mouse models has also been observed (Macaisne et al., 2008; Guiraldelli et al., 2018; Zhang et al., 2018). A mouse model replacing exons 2–20 of Shoc1 with a Neo-PGK-gb2 cassette showed embryonic lethality in homozygous mutant mice (Guiraldelli et al., 2018). However, replacing exons 2–4 of Shoc1 with a neomycin-resistance cassette generated a hypomorph mouse model showing lower expression of truncated, but functional, SHOC1, resulting in a moderate phenotype presented by a reduced number of crossovers and meiotic arrest at metaphase I. In this study, a Sertoli-cell and male germ-cell specific Shoc1 cKO mouse model, as well as a derived general Shoc1 KO mouse model, were obtained by deleting the coding exon 5 using the CRISPR/Cas9 method. As a result, the germ cell-specific Shoc1 cKO mouse model and the Shoc1 KO mouse model exhibited similar spermatogenetic defects, while the Sertoli-cell-specific Shoc1 cKO mouse model maintained normal fertility. Our results indicated that SHOC1 mainly functions in gametogenesis without causing other developmental problems, and that spermatogenetic defects are dominated by SHOC1 deletion in male germ cells. Shoc1 KO mice and germ-cell cKO mice with dramatic SHOC1 reduction exhibited similar defective phenotypes, in which meiosis was arrested at a zygotene-like stage with severe defects in SC assembly and DSB repair. Our results were similar to those of another Shoc1 KO mouse model with a 7-bp deletion in exon 5 (Zhang et al., 2018), with the exception that the synaptic defects seemed much more significant in our models. Thus, the severity of meiotic defects may correlate with the deletion method of Shoc1, and under some rare circumstances, a shorter form of SHOC1 could still exert partial function.
SHOC1 has a well-defined predicted XPF domain. Most XPF-related proteins are conserved endonucleases responsible for branched DNA structure recognition and processing. SHOC1, however, preferentially binds branched DNA molecules but lacks endonuclease activity (Macaisne et al., 2008; Guiraldelli et al., 2018). Human SHOC1 is the ortholog of yeast ZIP2, which belongs to the ZMM protein group. The main function of the ZMM proteins is to promote crossover formation by protecting the recombination intermediates (Shinohara et al., 2008). Previous studies have revealed the role of mammalian ZIP2 (SHOC1) as a crossover facilitator or stabilizer along with two other ZMM proteins, ZIP4 (mammalian TEX11) and SPO16 (Guiraldelli et al., 2018; Zhang et al., 2019a). Interestingly, in budding yeast, ZIP2 was also shown to localize to the sites of synapsis initiation to promote SC formation (Chua and Roeder, 1998; Arora and Corbett, 2019), but the underlying mechanism has not been identified. In our study, we showed that SHOC1 deficiency caused extensive defects in homologous pairing and effects on the homology search-related proteins (such as DMC1, RAD51 and RPA2), as well as asynapsis. DMC1 and RAD51 are bacterial RecA homologues and may facilitate in the homology search process, after which DNA strand invasion exchange produces a linkage between the two homologs (Yoshida et al., 1998), while the RPA-related proteins modulate their activity (Hinch et al., 2020). Therefore, these findings indicate that SHOC1 may also be involved in homology search-related activities in the early stages of meiotic recombination, soon after DSB formation. This speculation is also motivated by the observation that mammalian SHOC1 had strong co-localization with DMC1 at the zygotene and pachytene stages, and the number of SHOC1 foci was highly reduced in Dmc1-deleted spermatocytes (Guiraldelli et al., 2018). Interestingly, SHOC1 foci were significantly increased in spermatocytes null for HOP2 (Guiraldelli et al., 2018), a gene suggested to enhance the catalytic activities of DMC1 and/or RAD51 (Chen et al., 2004; Petukhova et al., 2005), implying that SHOC1 might enhance the function of DMC1 and/or RAD51 when they have decreased catalytic efficiency. This could also explain the increased DMC1 and/or RAD51 foci in Shoc1-deficient mice observed in our study. In a mutant with defective DSB repair, ZIP2 co-localized with proteins involved in DSB formation and processing (Chua and Roeder, 1998). Based on these observations, we can infer that SHOC1 may be involved in DMC1 and/or RAD51-mediated catalytic activities. However, whether SHOC1 affects the early steps of meiotic recombination by ensuring the catalytic efficiency of DMC1 and/or RAD51 or by enhancing the target strand exchange activities in cooperation with RPAs remains to be tested in the future. Moreover, our results uncovered that more DSBs were incurred due to SHOC1 defects, which was also shown in ZMM mutants from S. cerevisiae (Mu et al., 2020). During meiosis, DSB formation must be controlled by a network of feedback circuits to mitigate the risk of genome instability, and SC assembly plays an essential role in this process (Keeney et al., 2014). The increased DSBs during SHOC1 deficiency might be caused by the lack of a negative feedback loop mediated by assembled SCs that inhibits DSB formation.
In summary, our findings report a recurrent SHOC1 variant and novel SHOC1 variants in humans with NOA. Moreover, we found that SHOC1 is a meiosis-specific gene responsible for SC assembly and DSB repair through homologous recombination, and revealed a possible presynaptic role of SHOC1 in aiding the process of homology search and/or strand exchange during meiotic recombination. Overall, this study provides a more detailed mechanistic understanding of SHOC1 from the perspective of genetics and biochemistry and will update its clinical significance for diagnostic purposes.
Supplementary data
Supplementary data are available at Molecular Human Reproduction online.
Data availability
The data underlying this article are available in the article and in its online supplementary material.
Acknowledgements
We would like to thank the families for participating and supporting this study.
Authors’ roles
Y.-Q.T., Cha.T. and W.W. conceived and designed the study. L.M., H.Z., H.N. and J.D. were involved in the patients care and evaluation. L.M., J.H., L.S., Y.L., Cha.T. and W.W. performed the experiment and analyzed the data. M.L. supplied technical support. L.M., X.X. and Che.T. supplied bioinformatics support. G.L. and G.L. participated in the project supervision. Cha.T. and W.W. wrote the manuscript. L.M., Y.-Q.T., Cha.T. and W.W. critically reviewed the manuscript and all authors read and approved the final manuscript.
Funding
This work was supported by the National Key Research and Developmental Program of China (2018YFC1004901 to Y.-Q.T.), the National Natural Science Foundation of China (81971447 and 82171608 to Y.-Q.T., 82101961 to Cha.T.), a key grant from the Prevention and Treatment of Birth Defects from Hunan province (2019SK1012 to Y.-Q.T.), Hunan Provincial Grant for Innovative Province Construction (2019SK4012), research grants from CITIC-Xiangya (YNXM-202004 and YNXM-202006) and the Hunan Provincial Natural Science Foundation of China (2020JJ5993).
Conflict of interest
The authors declare no conflict of interest.
References
Author notes
Chaofeng Tu and Yue-Qiu Tan contributed equally to this work.