-
PDF
- Split View
-
Views
-
Cite
Cite
Yi-An Tu, Chia-Hung Chou, Po-Kai Yang, Chia-Tung Shun, Wen-Fen Wen, Po-Nien Tsao, Shee-Uan Chen, Jehn-Hsiahn Yang, Intentional endometrial injury enhances angiogenesis through increased production and activation of MMP-9 by TNF-α and MMP-3 in a mouse model, Molecular Human Reproduction, Volume 27, Issue 10, October 2021, gaab055, https://doi.org/10.1093/molehr/gaab055
- Share Icon Share
Abstract
There have been reports of improved pregnancy rates after performing intentional endometrial injuries, also known as endometrial scratching, in patients with recurrent implantation failure. In our previous study on intentional endometrial injury, we found an increased expression of matrix metalloproteinase (MMP)-3 following induced injuries to the mice endometrium. In the current study, we further examine whether the rise in MMP-3 could contribute to increased angiogenesis. Female C57B1/6 mice were obtained at 12 weeks of age, and intentional endometrial injuries were induced mechanically in the left uterine horns. Using the appropriate media, uterine-washes were performed on the injured and uninjured (control) horns of the harvested uteri. The uterine tissues were further processed for tissue lysates, histopathology and immunohistochemistry. The results show that intentional endometrial injuries caused an increase in secreted LPA in the injured horns, which were detected in the uterine-washes. In addition, LPA induced increased production of TNF-α in human endometrial epithelial cells (hEEpCs). Furthermore, TNF-α appeared to induce differential and cell-specific upregulation of the MMPs: MMP-3 was upregulated in the epithelial (hEEpCs), while MMP-9 was upregulated in the endothelial cells (human endometrial endothelial cells; hEEnCs). The upregulation of MMP-3 appeared to be necessary for the activation of MMP-9, whose active form stimulated the formation of vessel-like structure by the hEEnCs. The results of this study suggest that there may be enhanced angiogenesis following intentional endometrial injuries, which is mediated in part by TNF-α-induced and MMP-3-activated MMP-9 production.
Introduction
Although advances in ART have greatly improved the overall outcomes in patients with infertility, a problem that has resisted treatment with conventional IVF is that of recurrent implantation failure (RIF) (Coughlan et al., 2014). In addition, the implantation rate, which has remained stationary even with improved techniques in other aspects of IVF treatment, is still an incompletely understood component that limits the success rate of conventional IVF (Timeva et al., 2014). Initially demonstrated in rodents that intentional endometrial injury, also known as ‘endometrial scratching’, has been proposed as a method of enhancing decidualization and improving the chances of embryo implantation (Lejeune et al., 1982). The technique was later adapted to humans, in whom repeated implantation failures were seen (Barash et al., 2003; Gnainsky et al., 2010). In general, intentional endometrial injury is a relatively simple, low-risk and low-cost procedure, that is well-tolerated by patients; it usually consists of a controlled mechanical disruption of the endometrial linings prior to the transfer of embryos. As randomized controlled trials have shown, intentional endometrial injury performed in the luteal phase of the preceding cycle significantly improved the clinical pregnancy rates of women with history of multiple failed implantations (Vitagliano et al., 2018; Olesen et al., 2019; van Hoogenhuijze et al., 2019; Bar et al., 2020; van Hoogenhuijze et al., 2020). However, the debate on whether these procedures are beneficial in unselected patients undergoing IVF has remained inconclusive (Lensen et al., 2019; van Hoogenhuijze et al., 2019; Vitagliano et al., 2019; Nahshon et al., 2020). In addition, proponents of endometrial scratching have yet to clearly define a target group, for whom the benefits are repeatedly demonstratable.
The incomplete knowledge of the underlying mechanisms, that led to the higher pregnancy rates, has been another point of contention. Various pathways involved in inflammation, angiogenesis and cellular activations have been shown to be upregulated following endometrial injuries (Gnainsky et al., 2010, 2015). Specifically, increased expressions of tumor necrosis factor-α (TNF-α), interleukins, interferon-γ, vascular endothelial growth factor and hypoxia-induced factor 1α have been shown, while activation of the uterine natural killer cells and recruitment of monocytes that locally differentiate to dendritic cells have been observed (Siristatidis et al., 2014; Liang et al., 2015; Yu et al., 2019). Upregulation of the genes involved in blastocyst adhesion and endometrial remodeling, such as laminin α4, integrin α6 and matrix metalloproteinase-1 (MMP-1), has also been demonstrated (Li and Hao, 2009). Although many cellular and molecular mediators have been identified, it remains unclear how these mechanisms led to the improvements in implantation.
In our previous study, we employed a mice model of intentional endometrial injury, where we identified a strong MMP-3 expression in the injured endometrium (Yang et al., 2019). MMPs are generally involved in the breakdown of extracellular matrices, such as during wound healing or tumorigenesis. Of interest, MMPs are also involved in the invasion of the stroma and decidua by the trophoblasts, which occurs after the blastocyst attaches to the uterine epithelium. Each of these steps requires the meticulous regulation of the extracellular matrix, of which the MMPs are often involved. Indeed, past studies have shown that MMP-2 is highly expressed in the subepithelial stroma during early implantation (Das et al., 1997; Zhao et al., 2002), while MMP-9 is expressed in the stroma and trophoblastic giant cells during the later phases of implantation (Martinez-Hernandez et al., 2011). MMP-3, which we identified in our previous study, was not universally found at the embryo-endometrial junction during implantation (Das et al., 1997; Chen et al., 2007). Due to the discrepant involvements of the MMPs reported in the previous studies, we designed the current study to elucidate whether the rise in MMP-3 from endometrial injury can be linked to increased angiogenesis, and whether it has a role in the interplay between the endometrial epithelium and endothelium during angiogenesis.
Materials and methods
Study animals and intentional endometrial injury
Female C57BL/6 mice were obtained at 12 weeks of age, at a weight of 25 g. The estrus cycle in mice can be divided into four stages: proestrus, estrus, metestrus and diestrus. Unless interrupted by pregnancies, these stages cycle continuously at a cycle duration of 4–5 days (Byers et al., 2012). After cycle synchronization, by methods of cohabitation and introduction of male mice urine, endometrial injury was induced during the peri-ovulation stage (proestrus and estrus), at which time, the vaginal opening is more permissive. The methods are detailed in our previous study (Yang et al., 2019). Briefly, after the mice were anesthetized by isoflurane (2-chloro-2-(difluoromethoxy)-1,1,1-trifluoro-ethane) inhalation, endometrial injuries were induced with 0.7-mm-diameter brushes that were inserted into the left uterine horn and mechanically rotated at 720°. The uninjured right horn from the uterus of the same mouse served as the control. The mice were sacrificed after set intervals, on Days 0, 1, 2, 3, 7 and 14 after endometrial injury. The intervals were chosen to assess the immediate effects on the endometrium following injury (Days 0, 1, 2 and 3) and the longer-term changes at the second and third cycles following endometrial injury (Days 7 and 14). After sacrifice, the uteri were dissected for histopathology, immunohistochemistry and tissue lysates (Fig. 1A). This study protocol was approved by the Institutional Animal Care and Use Committee (IACUC) at the College of Medicine, National Taiwan University (Approval no. 20180151). All experimental steps were performed according to the relevant guidelines and regulations.
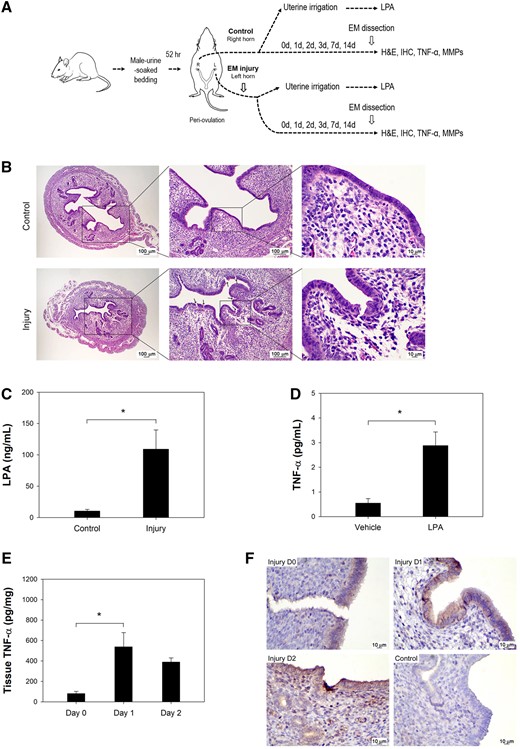
Endometrial injury induced LPA and TNF-α. (A) A diagram of the protocol used in the mouse experiments. (B) H&E staining of the mouse endometrium from an injured horn and control horn is shown (left panel, magnification 40×; middle panel, magnification 100×; right panel, magnification 400×). The presence of cracks in the luminal epithelium (arrows) was visible in the injured edematous endometrium. (C) LPA concentrations in the mouse uterine washing medium at 30 min post-injury showed much higher levels (P = 0.043). (D) TNF-α expression was more prominent in human endometrial epithelial cells (hEEpCs) treated with 10 µM LPA (P = 0.004). (E) Mouse endometrial TNF-α levels increased significantly on Days 1 and 2 post-injury (P = 0.030). (F) Immunohistochemistry demonstrated more prominent TNF-α stain in the endometrium on Days 0, 1 and 2 after injury than in uninjured endometrium (magnification 400×). Mouse isotype IgG was used as control. *Significant.
Hematoxylin and eosin (H&E) staining
The mice uteri were dissected immediately after sacrifice for histopathological evaluations. Dissected uteri were fixed in 4% paraformaldehyde and embedded in paraffin blocks. Paraffin blocks were sectioned at 5μm thickness and stained with H&E for histopathological evaluations under a light microscope.
Immunohistochemistry (IHC)
The slides were rehydrated in phosphate buffered saline (PBS) for 15 min and were inhibited by endogenous peroxidase at room temperature for 10 min using 3% H2O2/methanol. For blocking, the slides were immersed in 5% non-fat milk/PBS at room temperature for 30 min. Slides were incubated with specific antibodies from Santa Cruz Biotechnology (Santa Cruz, sc, CA, USA) and Bioss Antibodies (Bioss Inc., bs, MA, USA) for 16 h at 4°C. The specific antibodies that were employed were as follows: TNF-α antibody (sc-52746; diluted to 2µg/ml), MMP-3 antibody (sc-21732; diluted to 4µg/ml), MMP-9 antibody (sc-393859; diluted to 2µg/ml), CD34 antibody (sc-74499; diluted to 2µg/ml), VEGFR2 antibody (bs-10412R; dilution of 1:400, diluted to 2.5µg/ml), and mouse isotype IgG (sc-2025; diluted to 8µg/ml). The peroxidase-conjugated secondary antibodies were added at room temperature for 1 h. The slides were developed by immersion in 0.06% 3,3'-diaminobenzidine tetrahydrochloride, and counterstained with Gill's Hematoxylin V.
Determining lysophosphatidic acid (LPA) levels
After dissection of the mice uteri, the injured and uninjured horns from each mouse were separately irrigated with 200 µl of 1× PBS containing heparin (100 U/ml). These uterine-washes were centrifuged at 3000 rpm for 10 min, and the supernatants were assayed for LPA with the mouse LPA ELISA Kit (MBS3806268, MyBioSource, San Diego, CA, USA).
Determining tissue concentrations of MMPs
The dissected uteri were separated into injured and uninjured horns. The endometrial tissues were grounded in liquid nitrogen for extraction of total proteins, using a cell lysis buffer (Thermo Fisher Scientific, Waltham, MA, USA). Concentrations of total protein were quantified using Bio-Rad Protein Assays (Life Science Research, Hercules, CA, USA).
Human endometrial tissues
Human endometrial tissues were isolated from women who had received hysteroscopic surgery for submucosal myomas. All patients (n = 6, Table I) were premenopausal (mean age 33 years, range 31–36 years), had regular periods and were not receiving hormone contraception. They were all in the mid-to-late proliferative phase (i.e. Days 8–12 by history taking) at surgery. Surplus endometrial tissues were collected for experimental use. This study protocol was approved by the National Taiwan University Hospital Research Ethics Committee, and informed consent was obtained from all women before surgery (NTUH-REC no. 201708038RINC). All methodologies were carried out in accordance with the approved guidelines.
Patient . | Age . | Menstruation regularity . | Intervala (days) . | Durationb (days) . | Diagnosis . | Symptoms . | Day of surgeryc . | Hormone medication use . |
---|---|---|---|---|---|---|---|---|
1 | 32 | Regular | 30 | 7 | Myoma | Anemia | 12 | No |
2 | 31 | Regular | 27 | 5 | Myoma | Anemia | 9 | No |
3 | 35 | Regular | 29 | 6 | Myoma | Abnormal uterine bleeding | 10 | No |
4 | 31 | Regular | 28 | 7 | Myoma | Anemia | 11 | No |
5 | 33 | Regular | 29 | 6 | Myoma | Abnormal uterine bleeding | 10 | No |
6 | 36 | Regular | 27 | 4 | Myoma | Primary infertility | 8 | No |
Patient . | Age . | Menstruation regularity . | Intervala (days) . | Durationb (days) . | Diagnosis . | Symptoms . | Day of surgeryc . | Hormone medication use . |
---|---|---|---|---|---|---|---|---|
1 | 32 | Regular | 30 | 7 | Myoma | Anemia | 12 | No |
2 | 31 | Regular | 27 | 5 | Myoma | Anemia | 9 | No |
3 | 35 | Regular | 29 | 6 | Myoma | Abnormal uterine bleeding | 10 | No |
4 | 31 | Regular | 28 | 7 | Myoma | Anemia | 11 | No |
5 | 33 | Regular | 29 | 6 | Myoma | Abnormal uterine bleeding | 10 | No |
6 | 36 | Regular | 27 | 4 | Myoma | Primary infertility | 8 | No |
Intervals indicate average days between each menstrual period.
Durations refer to average duration of bleeding in each menstrual period.
Day of the menstrual cycle on the day of surgery derived from the date of the last menstrual period by history taking.
Patient . | Age . | Menstruation regularity . | Intervala (days) . | Durationb (days) . | Diagnosis . | Symptoms . | Day of surgeryc . | Hormone medication use . |
---|---|---|---|---|---|---|---|---|
1 | 32 | Regular | 30 | 7 | Myoma | Anemia | 12 | No |
2 | 31 | Regular | 27 | 5 | Myoma | Anemia | 9 | No |
3 | 35 | Regular | 29 | 6 | Myoma | Abnormal uterine bleeding | 10 | No |
4 | 31 | Regular | 28 | 7 | Myoma | Anemia | 11 | No |
5 | 33 | Regular | 29 | 6 | Myoma | Abnormal uterine bleeding | 10 | No |
6 | 36 | Regular | 27 | 4 | Myoma | Primary infertility | 8 | No |
Patient . | Age . | Menstruation regularity . | Intervala (days) . | Durationb (days) . | Diagnosis . | Symptoms . | Day of surgeryc . | Hormone medication use . |
---|---|---|---|---|---|---|---|---|
1 | 32 | Regular | 30 | 7 | Myoma | Anemia | 12 | No |
2 | 31 | Regular | 27 | 5 | Myoma | Anemia | 9 | No |
3 | 35 | Regular | 29 | 6 | Myoma | Abnormal uterine bleeding | 10 | No |
4 | 31 | Regular | 28 | 7 | Myoma | Anemia | 11 | No |
5 | 33 | Regular | 29 | 6 | Myoma | Abnormal uterine bleeding | 10 | No |
6 | 36 | Regular | 27 | 4 | Myoma | Primary infertility | 8 | No |
Intervals indicate average days between each menstrual period.
Durations refer to average duration of bleeding in each menstrual period.
Day of the menstrual cycle on the day of surgery derived from the date of the last menstrual period by history taking.
Isolation of human endometrial epithelial cells (hEEpCs)
The purification of hEEpCs has been previously described (Chen et al., 2016; Chou et al., 2020). Briefly, the endometrial tissues were washed with 50 ml Dulbecco's modified Eagle's medium (DMEM, Invitrogen Technologies, Waltham, MA, USA) and treated with 2.5 ml of 0.1% Type II collagenase in DMEM at 37°C for 1.5 h. The collagenase-digested endometrial tissues were separated by filtration through a 100-μm nylon cell strainer (Becton Dickinson, Franklin Lakes, NJ, USA). Isolation of hEEpCs was performed using a 40-μm nylon cell strainer (Becton Dickinson). The hEEpCs cells remaining on the 40-μm nylon strainer were cultured in phenol-red-free DMEM/F-12 that contained 10% charcoal-stripped fetal bovine serum. Cell purity was assessed by flow cytometry for cytokeratin 8 (Santa Cruz Biotechnology, Dallas, TX, USA) and was determined to be greater than 92%.
Isolation of human endometrial endothelial cells (hEEnCs) and stromal cells
After separation of the hEEpCs, the hEEnCs were isolated from the cell suspension that passed the 40-μm nylon cell strainer. The cell suspension was washed in 5 ml of Hanks' balanced salt solution that contained 10% fetal calf serum (Invitrogen). The cells were incubated, under gentle agitation, for 30 min in 1 ml of cold M199 medium (Invitrogen) that contained 1µg/ml of mouse anti-human PECAM-1 (CD31) antibody (sc-13537; Santa Cruz Biotechnology). This was followed by the addition of 50 μl of Dynabeads™ M-280 Sheep Anti-Mouse IgG (10 mg/ml), and incubation for a further 30 min. Magnetic isolation of hEEnCs from unbounded stromal cells was performed using an MPC-1 magnet (Dynal®, Oslo, Norway). The hEEnCs were re-suspended and cultured in endothelial cell growth medium (Cell Applications, San Diego, CA, USA). The unbounded stromal cells were collected, re-suspended and cultured in phenol-red-free DMEM/F-12 that contained 10% charcoal-stripped fetal bovine serum. Cell purities of the hEEnCs and the stromal cells were both >95% when assessed by flow cytometry for CD31 and vimentin (Dako, Deerfield, IL, USA), respectively.
Effects of LPA on TNF-α expression in hEEpCs
The hEEpCs were cultured on 6 cm dishes (1×106/dish) for 16 hours. After the cells had attached and achieved 80% confluence, they were washed twice with PBS. The hEEpCs were cultured in 2 ml serum-free DMEM/F-12, and treated with vehicle (20 μl 1% BSA, Sigma Aldrich, St. Louis, MS, USA) or LPA (10µM; Cat. no. L7260, Sigma Aldrich) for 24 h. The cell culture media were collected and centrifuged at 1000g for 5 min at 4°C to remove cell debris. The levels of TNF-α were quantified by ELISA.
Quantification of TNF-α and MMPs using ELISA
The levels of TNF-α, MMP-1, -2, -3 and -9 and active MMP-9 in the tissue lysates and cell culture media were determined using these enzyme immunoassay kits: mouse TNF-α Quantikine ELISA kit (MTA00B), mouse MMP-1 ELISA kit (Cusabio, Houston, TX, USA), mouse MMP-2 Quantikine ELISA kit (MMP200), mouse MMP-3 Quantikine ELISA kit (MMP300), mouse MMP-9 Quantikine ELISA kit (MMPT90), human TNF-α Quantikine ELISA kit (DTA00C), human MMP-3 Quantikine ELISA kit (DMP300), human MMP-9 Quantikine ELISA kit (DMP900), and human active MMP-9 Fluorokine E kit (F9M00). All kits were obtained from R&D Systems (Minneapolis, MN, USA).
Semiquantitative assessment of angiogenesis and neovascularization by IHC
Insitu quantification of the microvessel density is frequently used as an index of angiogenesis. Angiogenesis was assessed immunohistochemically, using the anti-CD34 antibodies, and neovascularization was assessed by staining for vascular endothelial growth factor (VEGF) receptor-2 (VEGFR2). The number of positively stained cells were counted under high power fields (HPFs, 400× magnification). Singular immune-positive endothelial cells or cells in independent endothelial clusters were included in the cell counts. Immune-positive endothelial cells that lined vessels with tunica media or nonspecific immune-positive areas without obvious endothelial structures were disregarded. The quantified number of CD34 and VEGFR2-positive cells was then compared between groups. Five pairs of uteri (control and injured horns) from five different mice were stained with anti-CD34 and VEGFR2. One representative section from each uterine horn was submitted for cell count. For each section, five HPFs were randomly selected for quantification of immune-positive cells. In summary, five HPFs from five different mice were quantified in each group, with a final total of 25 HPFs per group.
Specific recombinant proteins and chemical regulators
UK356618 (Sigma), an MMP-3 inhibitor, was used to assess the functions of MMP-3. Recombinant human TNF-α protein (rhTNF-α; 210-TA; R&D Systems) was used to elicit the endometrial response in human endometrium cell cultures. Recombinant human MMP-9 protein (rhMMP-9; 911-MP; R&D Systems), in proenzyme form, was used to validate the role of MMP-9 in angiogenesis. Amino-phenyl mercuric acetate (APMA, Sigma) was used to activate rhMMP-9 from the latent pro-collagenase form. In short, these recombinant proteins and their chemical regulators acted as singular controls in the proposed angiogenesis cascade, in order to elucidate the roles of the MMPs in the injury response of the human endometrial cells in vitro.
Conditioned medium (CM)
Attached hEEpCs (1×106 cells in 6 cm dish) were first washed with PBS twice and cultured in 3 ml serum-free DMEM/F-12 for 24 h as serum starvation. The culture media were then replaced with 3 ml serum-free M199 media plus either vehicle (10 µl ddH2O) or rhTNF-α (1 ng/ml) for another 24 h. The culture media were then collected, centrifuged at 4°C 1000g for 5 min for cell debris removal, then used as vehicle CM or TNF-α protein CM. The vehicle CM and TNF-α protein CM were then cultured with hEEnCs for another 24 h, after which the concentrations of MMP-3 and MMP-9 were quantified using ELISA, and the hEEnCs were assessed for tube formations.
hEEnCs tube formation assay
hEEnCs (1×104 cells in 100 μl) were plated onto a layer of Cultrex® Reduced Growth Factor Basement Membrane Extract (RGF-BME, R&D Systems) in 96-well plates, and incubated at 37°C for 4 h. Photographs of the tube-like structures were taken under 100× magnification. The number of tube-like structures per HPF (400×) was quantified for each of the different culture conditions.
Statistical analysis
The number of mice or human subjects per group that were involved in each part of the experiments is different, so the numbers are independently reported in each section of the results and figures. For comparisons between two independent groups, the Mann–Whitney U-test was employed; for two groups with linked samples, the Wilcoxon-Signed Rank test was employed; and for multiple groups comparisons, the Kruskal–Wallis test followed by the Tukey test was employed. Significance level was set at P < 0.05. Statistical Program for Social Sciences (SPSS version 12; SPSS Inc., Chicago, IL, USA) was used for the calculation of the P-values.
Results
Relationship between endometrial injury, LPA production and expression of TNF-α
On Day 0, histopathology showed microscopic injuries in the mouse endometrium. Under HPF, there was the presence of cracks and discontinuities in the luminal epithelium, and local hemorrhages in the edematous, sub-epithelial stroma (Fig. 1B). Higher concentrations of LPA were detected in the uterine-washes from the injured uterine horns, as compared with the uterine-washes from the uninjured horns (Fig. 1C, n = 5 pairs of uterine horns from 5 mice, P = 0.043). To clarify the relationship between LPA and TNF-α, LPA was added to primary cultures of hEEpCs. The LPA-treated hEEpCs demonstrated higher levels of TNF-α compared with untreated hEEpCs from the same patients (Fig. 1D, n = 6 paired samples from six patients, P = 0.004). A temporal relationship between the secretion of LPA and production of TNF-α was seen in vivo, whereby higher levels of TNF-α was detected in the mice endometrial lysates on post-injury Day 1 (Fig. 1E, n = 3 mice per time-point, P = 0.030 for comparison to Day 0 values), following the elevated LPA in uterine-washes on Day 0. Immunohistochemistry also demonstrated stronger staining for TNF-α in the endometrium of the injured uterine horns (Fig. 1F).
Endometrial injury induces expression of MMPs
Levels of MMPs in the endometrial tissue lysates from the injured and control horns on post-injury Days 0, 1, 2, 3, 7 and 14 mice were examined and compared. The concentrations of MMP-1 and MMP-2 on Days 1, 2, 3, 7 and 14 mice were not significantly different from their concentrations on Day 0. In contrast, MMP-3 concentrations were significantly higher on Days 2 and 3 (Fig. 2A, n = 5 mice per time-point for a total of 30 mice for the whole experiment, P = 0.004 for comparisons of Days 0 and 2 and P = 0.013 for comparisons of Days 0 and 3). In contrast, MMP-9 was only significantly higher on Day 7 (P = 0.013, for comparison to Day 0). Between Days 2 and 14, immunohistochemistry of the mice uteri showed similar changes in situ, with more prominent staining of MMP-3 in the injured luminal epithelium of the endometrium and MMP-9 in the injured epithelium and also in the sub-epithelium (Fig. 2B).
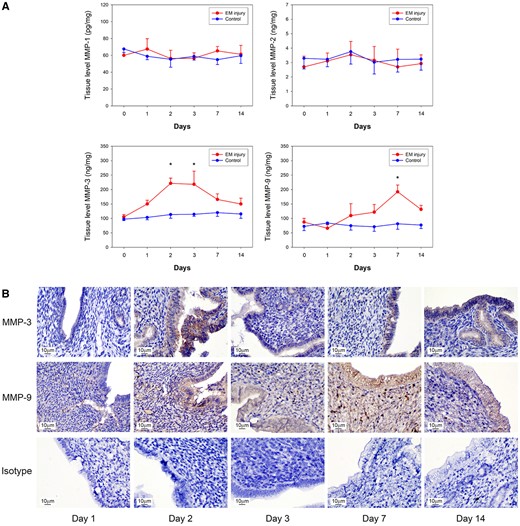
Temporal and spatial changes in MMP expression in mouse model. (A) MMP-3 concentrations were significantly increased on post-injury Days 2 and 3 (P = 0.004 and 0.013, respectively), while MMP-9 concentrations were increased on Day 7 (P = 0.013), compared to that on Day 0. MMP-1 and MMP-2 remained unchanged after injury. (B) Immunohistochemistry revealed prominent MMP-3 and MMP-9 expression from post-injury Days 2–14 after endometrial injury. Mouse isotype IgG was used as control. *Significant.
TNF-α as an inducer of MMP and the effect on angiogenesis
To test whether the increased expression of MMP-3 and MMP-9 were induced by TNF-α, TNF-α (1 ng/ml) was added to cultures of hEEpCs, hEEnCs and endometrial stromal cells. The results showed that following the addition of TNF-α to the hEEpCs, there was an upregulation of MMP-3 (Fig. 3A, n = 6 paired samples from six patients, P = 0.004, for comparison to the addition of vehicle), but this effect was not seen in the hEEnCs or in the endometrial stromal cells. In contrast, MMP-9 was upregulated in the hEEnCs, following the addition of TNF-α (Fig. 3A, n = 6 paired samples from six patients, P = 0.010, for comparison to the addition of vehicle), but this was not seen in the hEEpCs or in the endometrial stromal cells. In the mouse model (Fig. 3B), there were more angiogenesis and neovascularization of the endometrium in the injured horns on Day 14, when compared with the control horns. This was seen by the significantly greater number of CD-34-positive (n = 5 pairs of uterine horns from five mice, P = 0.00001) and VEGFR2-positive cells (n = 5 pairs of uterine horns from five mice, P = 0.00001) (Fig. 3B, lower panel). A similar picture was seen with immunohistochemistry, as there were more prominently stained cells on the representative photographs (Fig. 3B, upper panel). CD34 is an endothelial progenitor cell marker, while VEGFR2 is a marker of functional capillaries, endothelial migration and proliferation (Miettinen et al., 2012).
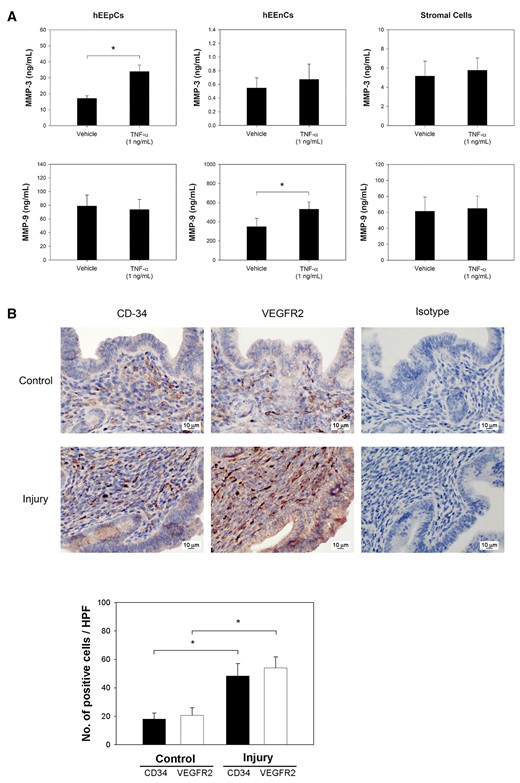
In vitro human cell-specific expressions of MMP-3 and MMP-9 induced by TNF-α. (A) MMP-3 concentrations were significantly induced by TNF-α in hEEpCs (P= 0.004), and MMP-9 concentrations were significantly induced by TNF-α in human endometrial endothelial cells (hEEnCs) (P= 0.010). (B) Immunohistochemistry with anti-CD34 and VEGFR2 antibodies of mouse endometrium demonstrated increased positive cells (brown staining) in the injured uterine horn in situ, compared to that in the non-injured control horn on post-injury Day 14. Sections stained with mouse isotype IgG were provided for reference. Using immunohistochemistry for semiquantitative assessment, significantly higher average numbers of CD34 (P= 0.00001) and VEGFR2 positive cells (P= 0.00001) were observed in the injured uterine horn. *Significant.
TNF-α-induced angiogenesis in hEEnCs are mediated through MMP-3 and MMP-9
The hEEnCs formed tube-like structures when incubated in the presence of TNF-α CM (Fig. 4A, left panel). Quantified results showed that this effect (Fig. 4A, right panels, n = 6 endometrial samples from 6 patients, P = 0.002) was downregulated in the presence of UK356618, a potent and selective enzyme inhibitor of MMP-3 (Fig. 4A, right panel, n = 6 endometrial samples from six patients, P = 0.014). The suppressive effects of UK356618 disappeared with the addition of APMA-activated rhMMP-9 (Fig. 4A, right panel, n = 6 endometrial samples from six patients, P = 0.043), but not with inactive rhMMP-9 (Fig. 4A, right panel, n = 6 endometrial samples from six patients, P = 0.014). This suggests that MMP-3 is responsible for the activation of MMP-9, whose active form induces the formation of vessels. This activation of MMP-9 by MMP-3 was further confirmed by assaying for total and active MMP-9 under different combinations of vehicle, TNF-α, TNF-α with UK356618, or TNF-α with DMSO (the vehicle of UK356618). TNF-α increased the total and active MMP-9 levels (Fig. 4B, P = 0.003 for total MMP-9; Fig. 4C, P = 0.0001 for active MMP-9; n = 6 endometrial samples from six patients). The TNF-α-induced increase in total MMP-9 was unaffected by UK356618, while a decrease was seen in active MMP-9 levels with the addition of UK356618 (Fig. 4C, n = 6 endometrial samples from six patients, P = 0.044). Concentrations of MMP-3 were also increased following the addition of TNF-α, but were unaffected by the further addition of UK356618 (Fig. 4D, n = 6 endometrial samples from six patients, P = 0.0008).
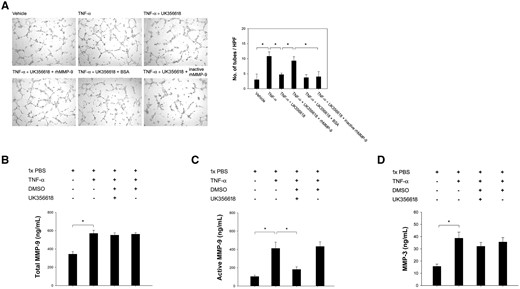
In vitro tube formation assay and the regulation of MMP-3 and MMP-9. (A) Tube formation assay demonstrated enhanced angiogenesis of hEEnCs after the addition of rhTNF-α-treated hEEpCs culture medium (P = 0.002). This enhanced angiogenesis was blocked by UK356618, an MMP-3 inhibitor (P = 0.014). This was mitigated by the addition of active rhMMP-9 (P = 0.043), but not of inactive rhMMP-9 (P = 0.014). BSA: bovine serum albumin. (B, C, D) Levels of total and active MMP-9 (P=0.003 and P = 0.0001, respectively) and MMP-3 (P = 0.044) were significantly increased after the stimulation with TNF-α, and the level of active MMP-9 is downregulated after the addition of UK356618 (P = 0.0008). DMSO, Dimethyl sulfoxide. *Significant.
Discussion
The known tissue repair paradigm, which proceeds from coagulation, inflammation and angiogenesis to tissue remodeling, has provided insights on how to achieve functional renewal via exploitation of the body’s natural responses to injuries. Some examples of this are renewed hair growth (Sun et al., 2009) and vaginal rejuvenation (Salvatore et al., 2015; Paraiso et al., 2020), which were found to follow controlled injuries. It is theorized that low-level tissue damage causes mesenchymal–epithelial transition (Owusu-Akyaw et al., 2019), tissue-specific stem cell activation (Gargett et al., 2016; Lucas et al., 2016) and neovascularization of the wound surfaces (Yang et al., 2019), which all contribute to functional renewal. Our study investigates the known tissue repair paradigm, as applicable to the endometrium, to further our understanding of the mechanisms that underlie the proposed improvements following endometrial scratching.
This study was designed to investigate the mechanisms specifically related to tissue angiogenesis. A mouse model was employed to explore the effects of intentional endometrial injury in vivo, while the relevant mechanisms were then verified in human endometrial cell cultures in vitro. We hypothesized that endometrial injury produces localized hemorrhage, and maybe platelet activation, which lead to production of TNF-α and MMPs, which in turn promotes angiogenesis. Specifically, we found that the cascade was initiated with the production of LPA, likely from platelet sources (Eichholtz et al., 1993; Sano et al., 2002). LPA is a well-known stimulatory molecule with growth-factor-like activities and immune activities (Chen et al., 2010; Beltrame et al., 2019). High levels of LPA stimulate the production of TNF-α, itself an important inflammatory cytokine that mediates the formation of new tissue after tissue injuries (Eisinger et al., 2018).
Members of the MMP family, also involved in the healing process during the later remodeling phase, were also found to be important players in this process. In our previous study, we noted the involvement of MMP-3, an angiogenesis protein, in endometrial injuries (Yang et al., 2019). In the current study, we further demonstrated that MMP-3 may be solely produced by the epithelial component of the endometrium after TNF-α stimulation, while MMP-9 was produced by the endothelial component. These induced MMP-3 and MMP-9 interact, whereby the MMP-3 activates the upregulated pro-MMP-9, which then directly stimulates angiogenesis. These findings provided a possible link that explains how endometrial injury can lead to a subsequent activation of angiogenesis.
Although our results reinforced the importance of MMPs in the healing process, these results are not completely compatible with the results from embryo implantation studies. We did not find an increased expression of MMP-2 after injury (Fig. 2A), which had been reported to participate in the early implantation process (Das et al., 1997; Zhao et al., 2002). In addition, although our study suggests a significant role for MMP-3 in the healing process of the endometrium, MMP-3 has not been mentioned in any significant role from studies on the peri-implantation endometrium (Das et al., 1997). However, this may be reconciled by the common pathway through MMP-9, which appears to be involved in both endometrial healing and blastocyst implantation (Lee et al., 2005). Known activators of MMP-9 include MMP-2 (Fridman et al., 1995), MMP-3 (Ogata et al., 1992; Hahn-Dantona et al., 1999) and MMP-13 (Knauper et al., 1997; Fridman et al., 2003). Perhaps it is this common pathway through MMP-9 activation, that is responsible for the purported benefits of endometrial scratching.
The limitations of this study are as follows. The current invivo endometrial injury model was established in mice, and we cannot ascertain that the endometrial injuries in mice accurately reflect those sustained by humans after endometrial scratching (Boretto et al., 2017). For example, there might be physiological differences in injury severity between those caused by a biopsy pipette in humans and the injuries that were presented in this study. Furthermore, the physiological responses may be different, as the mouse uterus only contains a single layer of epithelium, while the human endometrium is multi-layered. Lastly, early embryo implantation may be a process that is too intricate to be assessed using conventional laboratory techniques, and finer techniques, such as those provided by the microfluidic culture model (Abbas et al., 2020), may be needed to detect more minute changes in mediators. And finally, embryo implantation is a complicated process with multiple contributing factors. Although we postulate that the improved angiogenesis in recently injured endometrium is a contributing factor for implantation success, we concede that we have yet to identify a clinical target group for this treatment. Perhaps a subset of RIF patients with low endometrial immune activation or non-immunological RIF may be the main beneficiaries, as suggested by a recent article (Rahmati and Ledee, 2020). However, more research is needed to answer these questions.
Conclusion
Intentional endometrial injury induces LPA secretions, which stimulate the production of TNF-α in endometrial tissues. TNF-α induces increased expression of MMP-9 in the endothelial cells of the endometrium and promotes the formation of MMP-3 in the epithelial cells of the endometrium. The production of MMP-3, in turn, activates the MMP-9 in the endothelial cells. Activation of MMP-9 enhances angiogenesis in the endometrium, which may facilitate embryo implantation (Fig. 5).
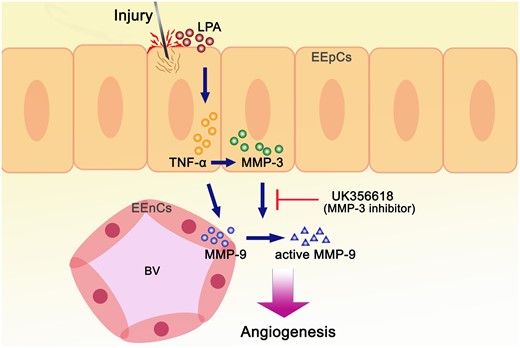
Schematic summary of the mechanisms involved in endometrial injury. Endometrial injury induces LPA, which stimulates TNF-α and MMP-3 secretion in the epithelial cells, which subsequently increases secretion and activation of MMP-9 in the endometrial endothelial cells leading to angiogenesis. The induced angiogenesis was blocked by UK356618, but could be induced with the direct addition of active rhMMP-9.
Data availability
Data are available on request.
Acknowledgements
The authors would like to express their thanks to the staff of National Taiwan University Hospital-Statistical Consulting Unit (NTUH-SCU) for statistical consultation and analyses.
Authors’ roles
Y.-A.T., C.-H.C. and P.-K.Y. were responsible for writing the manuscript. Y.-A.T., S.-U.C., C.-T.S., W.-F.W. and J.-H.Y. contributed to the interpretation of data for the work. C.-H.C., C.-T.S. and W.-F.W. were responsible for execution and analysis of the study. P.-K.Y., P.-N.T. and S.-U.C. were responsible for editing of the manuscript and revising it critically. J.-H.Y. participated in the study design and the final revision of the article.
Funding
This study was supported by the research grant MOST 107-2314-B-002-158-MY3 from the Ministry of Science and Technology of the Republic of China (Taiwan), and the research grants NTUH 108-S4094 and NTUH 109-S4467 from the National Taiwan University Hospital.
Conflict of interest
All authors report no conflict of interest.