-
PDF
- Split View
-
Views
-
Cite
Cite
M. D. Gray, R. J. Cohen, A. M. S. Richards, J. A. Yates, D. Field, Co-propagation of maser emission at 1720 and 4765 MHz, Monthly Notices of the Royal Astronomical Society, Volume 324, Issue 3, June 2001, Pages 643–647, https://doi.org/10.1046/j.1365-8711.2001.04349.x
- Share Icon Share
Abstract
MERLIN observations are presented of OH 4765-MHz and OH 1720-MHz masers in the massive star-forming region W3(OH). Two of the three intense spots of maser emission at 4765 MHz are spatially coincident with two similar spots at 1720 MHz in both left-hand circular (LHC) and right-hand circular (RHC) polarizations, to an accuracy of 15 mas. The spots also overlap in velocity when allowance is made for Zeeman splitting of the 1720-MHz line. We conclude that we have found two examples of masers in different rotational levels of OH which are co-propagating through the same column of gas and experiencing competitive gain effects. The third 4765-MHz maser spot was found to have no overlapping counterpart amongst the 1720-MHz masers.
1 Introduction
Masers are potentially powerful diagnostic tools for probing the physical conditions in star-forming regions with unrivalled milliarcsec spatial resolution. Theoretical analysis of maser pumping and propagation indicates that the simultaneous detection of different maser transitions of a molecule arising from the same volume of gas, and amplified along the same line of sight, can enable very precise bounds to be placed on the local physical conditions, such as the temperature and density of the gas, the molecular abundance, the magnetic field and the velocity gradient along the line of sight Gray, Field & Doel (1992). However, it has proved very difficult to exploit this potential in practice, because of the problem of measuring the absolute positions of the different masers to a high enough accuracy to be satisfied that they are spatially coincident. Nevertheless there has been growing statistical evidence for an intimate association between OH 1720-MHz emission from the J = 3/2 ground rotational state, and 4765-MHz emission from the J = 1/2 excited rotational state. For example, the single-dish survey by Cohen, Masheder & Caswell (1995) showed that all maser sources detected at 4765 MHz were also known 1720-MHz emitters. Theoretical analysis explains physically why this association should occur Gray et al. (1992). The difficulty has been to verify experimentally that individual 1720- and 4765-MHz masers really coincide in space, and are not simply produced in different parts of an excited gas cloud.
The highest angular resolution for studying OH masers can be achieved using very long-baseline interferometry (VLBI). VLBI enables individual OH masers to be distinguished from each other, their spot sizes measured, and their relative positions determined to a precision of less than 1 mas. However, the absolute positional accuracy has been typically 0.1 arcsec or greater (e.g. Bloemhof, Reid & Moran 1992; Masheder et al. 1994), which is too coarse to address the question of spatial association precisely. The MERLIN interferometer network uses shorter baselines than VLBI, so the angular resolution is not as high, but the use of external phase-referencing allows absolute positions of masers to be measured to an accuracy of typically 10 mas, an order of magnitude better than for VLBI. Thus MERLIN observations have the accuracy to establish positional coincidence provided that the masers can be separated by the MERLIN interferometer beam. We have begun a programme of MERLIN phase-referenced observations of masers in W3(OH) and other sources to search for spatial coincidences between OH masers at 18- and 6-cm wavelength.
W3(OH) is a massive star-forming region which contains one of the strongest OH maser sources in the sky. The source is believed to contain a very young O-type star, which has produced an ultra-compact H ii region which is expanding into the surrounding molecular material. W3(OH) is a strong emitter in all the known OH maser transitions. Recent VLBI experiments have been carried out at 1.7 GHz (Garcia-Barreto 1988; Bloemhof et al. 1992; Masheder et al. 1994), 4.7 GHz (Baudry et al. 1988; Baudry & Diamond 1991), 6.0 GHz (Desmurs et al. 1998) and 13 GHz (Baudry & Diamond 1998), while quasi-thermal emission and absorption have been studied at other frequencies (for example, Baudry & Menten 1995). In the present paper we describe new MERLIN2 observations which demonstrate for the first time the close spatial association between OH masers at 1720 and 4765 MHz.
2 MERLIN observations
The 1720-MHz observations used in this work were carried out between 1993 December 02 17:25 ut and 1993 December 04 05:17 ut. Eight telescopes of MERLIN (Thomasson et al. 1993) were used, giving 27 baselines, the MkII-Lovell baseline being discarded because of confusion. The reference antenna was the MkII telescope at Jodrell Bank. In addition to the target, observations were made towards a bandpass calibrator (3C 84), and a phase calibrator (0224+671) with observations of the latter source interleaved with observations of the target. Tabulated values for a primary flux calibrator (3C 286) were used to set the flux of 3C 84 at 27.37 ± 0.13 Jy at 1720 MHz. All three sources were observed in the same spectral configuration: dual circular polarization with 128 channels covering a bandwidth of 125 kHz, giving a channel separation of 977 Hz (0.170 km s−1). The band centre (the boundary of channels 64 and 65) was set to a local standard of rest (LSR) velocity of −45.0 km s−1.
The 4765-MHz observations were carried out between 1995 March 15 17:52 ut and 1995 March 17 06:04 ut. This experiment used six MERLIN antennas, resulting in 15 baselines. The reference antenna was again the MkII telescope. The observed continuum calibrators, and in particular the phase calibrator 0224+671, were the same as those used at 1720 MHz. The primary flux calibrator was 0552+398; the flux was set at 6.0 ±0.3 Jy. The spectral configuration for the 4765-MHz observations was dual circular polarization with 64 channels in a bandwidth of 250 kHz, giving a channel separation of 3.91 kHz (0.245 km s−1). The band centre (the boundary of channels 32 and 33) was set to a LSR velocity of −45.0 km s−1. All three sources were observed in the same spectral configuration.
The absolute positional accuracy of the phase calibrator, 0224+671, is less than 2 mas, from astrometric VLBI observations (Sovers et al. 1988), but is not a limiting factor for these observations. Larger errors in absolute position result from the fact that although the external phase calibrator corrects the phase at the target, removing the zeroth-order phase error, there is a residual first-order error dependent on the gradient of the phase. This error, proportional to the separation of the target and phase calibrator positions, limits the absolute positions to about 10 mas. This is further discussed in Section 4.
3 Data reduction
After initial editing, to remove interference and instrumental problems, the uv data were processed using the Astronomical Image Processing System (aips, Greisen 1994). Basic steps in the reduction were a correction for the parallactic angle, flux calibration, bandpass calibration and phase calibration, allowing initial generation of images of the target in LHC and RHC polarizations. Accurate absolute positions for the reference feature at each frequency were determined at this stage, using the aips task jmfit. Positions at epoch B1950 were used throughout the data reduction process. Some positions were later precessed to epoch J(2000) for comparison with the work of other authors (see Section 6). In all cases, the reference feature was chosen to be the brightest spatial feature in the brightest spectral channel in order to obtain the maximum signal-to-noise ratio. At 4765 MHz, the reference feature was in channel 32, whilst at 1720 MHz it was in channel 62 for 1720R, channel 67 for 1720L. The accurate positions were determined at this stage because the initial images were produced using calibration tables based on the phase calibrator, that is, prior to any self-calibration which can cause small shifts in the positions of feature maxima. The 4765-MHz reference feature is known to be unresolved by a 25-mas beam, and perhaps as low as a 15-mas beam (Baudry et al. 1988). However, at 1720 MHz, the reference feature has been shown to be complex in 1720L by Masheder et al. (1994) with components 2–3 mas in size, separated by angles of up to 10 mas. Therefore, for both polarizations at 1720 MHz, positions were derived from images based on a single clean-component. This procedure minimizes the effect of the weaker sources within the beam. Following position fitting, images of the maser reference features at each frequency (1720-MHz LHC, 1720-MHz RHC and 4765 MHz) were used as models to self-calibrate the data, with five iterations required to construct the phase and amplitude models which were then applied to all the data in each frequency channel.
In the case of 4765 MHz, the LHC and RHC images were very similar, so the Stokes I clean components were used as the only model, and the polarization-specific data reduction was terminated at this stage. The extreme similarity of LHC and RHC spectra in the 4.7 GHz lines is exactly as expected, since the Lande g-factors for the corresponding transitions are zero to a very good approximation. The data were then imaged for all spectral channels, using the last calibration table generated by the self-calibration process. The circular Gaussian restoring beams had FWHM diameters of 130 mas for the observations at 1720 MHz; 40 mas for 4765 MHz. Generation of this full image cube included a Hanning smoothing algorithm, which degraded the velocity resolution by a factor of two. Significant maser emission features, defined as above the 5-σ level, were identified using the aips task sad, channel by channel, until a complete table of such features was generated.
Maser spots, consisting of several sequential features, persisting over at least a minimum number of adjacent channels, were identified by the fortran code, happy, described in detail elsewhere (Wright et al., in preparation). happy normally required three sequential features, with each successive feature pair separated by 1 MERLIN beam width or less, to define a spot. Three spots were found at 4765 MHz. At 1720 MHz, there were 25 identified spots in LHC, and 32 in RHC, but only four of these were sufficiently close to the 4765-MHz spots to warrant further investigation of overlaps. Details of the three 4765, and four selected 1720-MHz maser spots appear in Table 1. It was found that the original reference features formed the brightest component of spot ‘C’ at 4765 MHz, and spot ‘D’ at 1720 MHz in both hands of polarization. The spots containing the reference features are indicated by asterisks in Table 1. The naming of the spots ‘A’, ‘B’, and ‘C’ follows the convention used by Baudry et al. (1988).
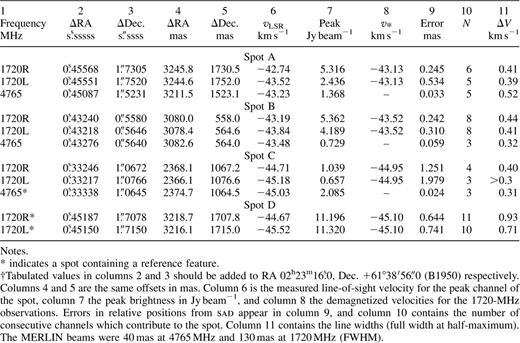
Characteristics of strong 4765- and 1720-MHz OH maser spots in W3(OH) (B1950 positions
Spot offsets from the image phase-centre, taken to be the flux-weighted mean offsets with averaging over all the contributing features in a spot, returned by the aips task sad, were then used to calculate the position of each spot relative to the reference feature. The combination of these relative positions, and the accurate position of the reference feature at each frequency (see above) were then used to derive the absolute positions of all spots. If peak channel offsets were used instead of the offsets from flux-weighted averaging, spot positions were altered by about 2 mas or less.
4 Absolute positions of maser spots
The absolute positions of the maser spots are shown in Table 1. It can be seen that within each of the spots B and C the absolute positions at all three frequencies overlap to within the MERLIN beams. These overlaps are secure to within the absolute accuracy limits imposed by the angular separation between the target source and the phase calibrator, which are in this case 5 mas at 5 GHz (Garrington et al. 1997) and 15 mas at 1.7 GHz (Muxlow, private communication). At 1.7 GHz, the errors based on the signal-to-noise ratios, R, of the reference features are significantly smaller with values, σ, of about 6 mas from the formula where θ is the FWHM of the MERLIN beam, and N the number of antennas in use.
Certain minor effects have been ignored in our data reduction strategy. First, we ignore the effects of proper motion between the two epochs of observation: the elapsed time is only 15 months, in which time proper motions in excess of are highly unlikely. At an assumed source distance of 2.2 kpc, each km s−1 of transverse velocity would introduce a shift of only 0.12 mas in this time (Bloemhof et al. 1992). Also because of the relatively short interval between observations compared with the 7.5 yr in Bloemhof et al. (1992), we can ignore the second order epoch corrections used in that work; these would produce positional uncertainties of <0.15 mas. Positional uncertainties of this magnitude do not change any of the conclusions of this work.
The OH 1720-MHz masers listed in Table 1 all occur in pairs, which are positionally coincident to within our errors of measurement, and which we argue are produced by Zeeman splitting (Section 6). In order to facilitate comparison between 1720-MHz masers and 4765-MHz masers, which do not show Zeeman splitting, we calculated for each 1720 MHz pair the unshifted or degmagnetized velocity corresponding to no Zeeman splitting to be the mid-point velocity between the LSR velocities of the LHC and RHC components at 1720 MHz in Table 1. The LSR and demagnetized velocities are based on flux-weighted averages over all the features contributing to each spot, the same scheme used for calculating the positions. Again, the difference introduced by using velocities of the peak channel for each spot were small compared to the velocity resolution. There is no more accurate demagnetization method available for 1720 MHz unless the magnetic hyperfine quantum numbers of the inverted levels are known. Calculations for full Zeeman multiplets at 1720 MHz, and other frequencies, appear in Gray & Field (1995), and further discussion of the Zeeman effect at 1720 MHz appears in Section 6.
5 Discussion of maser overlaps
The positions of spots ‘B’ and ‘C’ at all three frequencies overlap to well within the positional errors. The overlaps are already fairly secure, as the measured sizes of the 4765-MHz spots in these regions are comparable to the largest position errors, those at 1720 MHz resulting from angular separation of the phase callibrator from the target (see Section 4). To obtain absolute position accuracy down to the size of 1720-MHz spots, smaller than 1.5 mas in region ‘B’ (Masheder et al. 1994), new observations are required in which the angular separation of target and phase calibrator must be <1°. Better uv coverage to detect extended emission, and so measure the true cloud extent is needed. Higher sensitivity is also required as, on average, the sensitivity limited number of phase calibrators for a given target will fall as the square of the maximum allowed target-to-calibrator separation. To confirm the overlap of maser spots, on scales smaller than the sizes of the individual spots at 1720 MHz, requires observations with higher angular resolution, ideally of order 1 mas, so phase-referenced VLBI is required.
We already know from Masheder et al. (1994) that the 1720L spot is smaller than 1.5 mas in region ‘B’. The 4765-MHz spot in this region might break up into components in a higher resolution experiment. Baudry et al. (1988) set upper size limits (see Section 3), and later work (Baudry & Diamond 1991) showed that there are 4765-MHz spots at a velocity of −43.5 km s−1 which are sufficiently small to appear on a transatlantic baseline, and these might be in region ‘B’. In region ‘C’, the 4765 MHz maser spot has a measured size of 34 mas (Baudry), but the size of the 1720 MHz spots is unknown. This region was not detected in 1720 MHz LHC polarization by Masheder et al. (1994) although the 1993 flux densities would have made it detectable. We can only say that either this spot brightened considerably between 1989 and 1993, or the 1720L spot size in this region is considerably larger than in region ‘B’, and the emission was resolved out by the global interferometer. In both the ‘B’ and ‘C’ regions the correlation between the demagnetized 1720 MHz velocities and the velocities of the 4765 MHz spots is good: well within the available resolution of ∼0.3 km s−1 at 4765 MHz.
5.1 Possible overlaps with 6- and 13-GHz masers
In order to compare our maser positions at 1720 and 4765 MHz with data at 6 GHz (Desmurs et al. 1998) and at 13.44 GHz (Baudry & Diamond 1998), we adopted the method of Aoki et al. (Aoki et al. 1993) to process our coordinates from the B1950 epoch to J2000. This method was also used by Baudry & Diamond (1998).
In the comparison with 13.44-GHz masers, the spots denoted ‘B’ and ‘C’ in this work clearly do not overlap with any 13.44-GHz component; they lie much too far to the south, as found previously by Baudry & Diamond (1998) (see fig. 5 of that work). The same is true of the 4765-MHz ‘A’ spot. This process of elimination leaves only the 1720-MHz spots ‘A’ and ‘D’ as possible overlap partners for the 13-GHz masers. The absolute positional accuracy in Baudry & Diamond is ±10 mas in RA, and ±20 mas in Dec., so we considered overlaps possible for separations of up to 18 mas in RA and 25 mas in Dec., after adding in quadrature the errors at 13 GHz, and the 1720-MHz data from the present work. The only possible overlap which has sufficiently close spatial superposition and a small velocity shift is spot ‘A’ from this work in 1720L overlapping with the same hand of polarization from spot 10 in table 1 of Baudry & Diamond (1998). The separation is 17.9 mas in RA and 1.3 mas in Dec., but the demagnetized velocities differ by 0.67 km s−1, so we discount this as a real association.
At 6 GHz, the absolute accuracy of the data (Desmurs et al. 1998) is considerably poorer, at ∼200 mas in each axis, so the criterion for a possible overlap was taken to be a position match within 200 mas, which is an order of magnitude larger than the MERLIN error, and larger than the MERLIN beam, combined with a velocity match within the MERLIN velocity resolution. The overlapping spot groups ‘B’ and ‘C’, and the 4765 MHz component of spot ‘A’ had no possible overlap partners. There were therefore no cases of overlap detected between 4765-MHz masers and 6-GHz masers at either 6030 MHz or 6035 MHz.
Only two possible cases of overlap between 1.7 and 6-GHz masers were found, both involving spot ‘A’ from the present work. The first case is a potential overlap between ‘A’, and spot E from table 1 in Desmurs et al. (1998) in both hands of polarization. The positional coincidence is 200 mas, at the limit of acceptability. The demagnetized velocities differ by 0.32 km s−1. The other possibility is an overlap between our spot ‘A’, and the component B’ at 6030 MHz from table 2 of Desmurs et al. (1998). The LHC spots were separated by 124 mas and the RHC spots by 146 mas, while the demagnetized velocities differ by 0.38 km s−1. These possible overlaps remain tentative because of the large absolute positional uncertainties associated with the 6-GHz data.
6 Physical interpretation
An association of 1720- and 4765-MHz emission in star forming regions has been predicted for some time by Gray et al. (1992). The required conditions are based on a predominantly radiative pumping scheme which must include far infrared line overlap. Higher temperatures are also required compared to conditions which will produce 1720-MHz amplification alone. Unfortunately, we have no size information for the 1720R spot in region ‘C’, but using just the peak brightnesses in this region, we find the 4765-MHz maser peak is twice as bright as at 1720R. This suggests conditions similar to those in fig. 12 of Gray et al. (1992) but with a somewhat shorter overlap scale and/or higher velocity gradient in order to enhance the 4765 MHz gain coefficient relative to 1720 MHz (fig. 6 of Gray, Doel & Field 1991). Approximate conditions for this column of gas would be a kinetic temperature of 125 K, velocity shift of 0.36 km s−1, molecular hydrogen number density of and an OH concentration of 10 parts per million. The amplifying column would need to be longer than 2 × 1012 m.
In regions ‘A’, and ‘D’, where we see dominant 1720-MHz emission, without accompanying 4765-MHz masers, the physical conditions are probably more like those giving rise to fig. 8 of Gray et al. (1992), that is, kinetic temperature = 50–75K, velocity shift of 1.3 to 2 km s−1, molecular hydrogen number density of and a similar OH concentration to the overlapping region. The amplifying column could be considerably shorter, requiring only ∼
to achieve full saturation. It is more difficult to produce conditions which generate isolated 4765 MHz masers, but densities and temperatures considerably above those required for the 1720/4765 overlap region are likely in order to quench ground-state inversions, and maintain high populations in the F2,
state.
The Zeeman structure of the 1720-MHz transition can be quite complex because it is a satellite transition with different energy shifts, for a given magnetic field, in the upper and lower states. The result is the generation of a maximum of nine dipole-allowed components, of which the six that may become circularly polarized (the σ components) may be relevant in this work. These six components break down into three Zeeman pairs, both members of each pair having identical Einstein A-values. The two members of each pair are shifted in frequency or velocity to either side of the centre frequency of the transition by shifts of equal magnitude. In taking the midpoint velocity between the LHC and RHC components as the demagnetized velocity in Section 4, we have assumed that the LHC and RHC components are members of the same Zeeman pair. When the Doppler shift has been applied, the shifts relating to the three 1720-MHz pairs are 0.055, 0.170 and 0.285 km s−1 mG−1, so we would expect the members of a pair to have velocity separations of double these values. Of these three, the pair with the smallest splitting has the largest Einstein A-value. Of course, in a maser region, only a subset of these magnetic hyperfine transitions may be inverted, depending on the parameters of the pumping scheme. If we assume that the observed circularly polarized Zeeman pairs correspond to the strongest magnetic component at 1720 MHz, (splitting of 0.11 km s−1 mG−1) the derived magnetic field in the bright D region is This is not significantly smaller than the field derived for the same region in Masheder et al. (1994) (8.1 mG) so there is no detectable change in the magnetic field strength. With the same assumptions, the magnetic field in region C is 4.7 mG, and is 6.2 mG in both the A and B regions. The field in all regions appears to be in the same sense. To look for the effects of blended multiple components in the 1720-MHz data, we attempted to measure asymmetries in the lineshapes of individual LHC and RHC features. No clear asymmetries were found. Further investigation of this problem requires future work with both higher velocity resolution and higher sensitivity.
7 Conclusions
We conclude that, within the experimental errors, there are two regions in W3(OH) where 1720-MHz and 4765-MHz OH masers are co-propagating through the same column of gas. Further observations (a phase-referenced VLBI experiment) are required with better spatial resolution, better uv coverage, and with high spectral resolution, and a phase reference source within 1° of the target to image the extent of the emitting regions and thus confirm the co-propagation of different maser transitions to an accuracy which is no larger than the sizes of the 1720-MHz spots (of order 1 mas).
The present MERLIN result provides the first clear observational evidence of masers at different frequencies co-propagating and undergoing competitive gain processes. Where spot sizes have been measured by other authors, the 4765-MHz maser spots in the two co-propagation regions are considerably larger than those at 1720 MHz. Owing to the small number of features in W3(OH) with closely associated 1720- and 4765-MHz emission, we conclude that co-propagation is not common, but we cannot estimate precisely how uncommon it is without more examples. The present data are part of a more comprehensive MERLIN survey of OH masers in W3(OH) at all 1.7-GHz and 4.7-GHz frequencies. Results of overlaps within the 1.7-GHz group will be presented elsewhere (Gray et al., in preparation). These results will allow us to investigate and analyse quantitatively via modelling, the physical conditions, saturation state and competitive gain effects in the co-propagating region.
No overlaps were found between 4765-MHz masers and other masers at 6035 MHz, 6030 MHz or 13.44 GHz. This is in accord with the detailed composite mapping of masers at several OH maser frequencies in Baudry & Diamond (1998), which included 4765 MHz, but no 1720-MHz data. A small number of tentative overlaps between one 1720-MHz spot and 6-GHz and 13.44-GHz data were found, but much better absolute positions are required at 6 GHz before most of these can be confirmed or rejected as likely cases of co-propagation. The 1720/13.44 GHz possibility looks very unlikely already, as it is near the extreme allowed separation in one axis, and has a velocity shift which does not suggest overlap.
Acknowledgments
MDG acknowledges the award of a University Research Fellowship by the Royal Society.
References