-
PDF
- Split View
-
Views
-
Cite
Cite
Lena Norrbrand, Mikael Grönkvist, Stylianos Kounalakis, Kjartan Halvorsen, Ola Eiken, Metabolic Demands and Kinematics During Level Walking in Darkness With No Vision or With Visual Aid, Military Medicine, Volume 188, Issue 7-8, July/August 2023, Pages e2010–e2017, https://doi.org/10.1093/milmed/usac327
- Share Icon Share
ABSTRACT
Uniformed services commonly perform foot-borne operations at night, while using visual aid in terms of night vision goggles (NVG). During slow-level walking, complete lack of visual input alters kinematics and markedly increases the metabolic demand, whereas the effect on kinematics and energy expenditure of restricting the peripheral visual field by wearing NVG is still unknown. The purpose was to evaluate whether metabolic demands and kinematics during level walking are affected by complete darkness with and without visual aid.
Eleven healthy men walked on a treadmill (inclination: +2.3°, velocity: 4 km/h) with full vision in a lighted laboratory (Light), and in complete darkness wearing either a blindfold (Dark), or restricting the visual field to about 40° by wearing monocular (Mono) or binocular (Bino) NVG. Oxygen uptake (|$\dot{\text{V}}$|O2) was measured to evaluate metabolic demands. Inertial measurement units were used to estimate kinematics, and the outcome was validated by using a motion capture system. Ratings of perceived exertion, discomfort, and mental stress were evaluated after each condition using a Borg ratio scale. Physiologic and kinematic variables were evaluated using repeated measures analysis of variance (ANOVA), whereas ratings were evaluated using non-parametric Friedman ANOVA.
|$\dot{\text{V}}$|O2 was 20% higher in the Dark (1.2 ± 0.2 L/min) than the Light (1.0 ± 0.2 L/min) condition. Nominally, |$\dot{\text{V}}$|O2 in the Mono (1.1 ± 0.2 L/min) and Bino (1.1 ± 0.2 L/min) conditions fell in between those in the Light and Dark conditions but was not statistically different from either the Light or the Dark condition. Step length was shorter in the Dark (−9%, 1.22 ± 0.16 m) and Mono (−6%, 1.27 ± 0.09 m) conditions than in the Light condition (1.35 ± 0.11 m), whereas the Bino (1.28 ± 0.08 m) condition was not statistically different from either the Light or the Dark condition. The three conditions with no or limited vision were perceived more physically demanding, more uncomfortable, and more mentally stressful than the Light condition, and the Dark condition was perceived more mentally stressful than both NVG conditions.
The study confirms that complete lack of visual cues markedly reduces the mechanical efficiency during level walking, even under obstacle-free and highly predictable conditions. That |$\dot{\text{V}}$|O2 and step length values for the NVG conditions fell in between those of the Light and Dark conditions suggest that both foveal and peripheral vision may play important roles in optimizing the mechanical efficiency during level walking.
INTRODUCTION
Several studies have shown that during walking, an individuals’ preferable step length,1 frequency,2 and hence speed3–5 are gauged so as to optimize the mechanical efficiency. In young and middle-aged men, the optimal economy of level walking typically occurs at a speed between 4.4 and 4.8 km/h, whereas faster or slower speed increases the metabolic demand in relation to covered distance.4,5
Visual feedback is important for postural stability6,7 and locomotion.8 Impaired or limited vision makes the walker more cautious of maintaining balance and results in shorter step length,9–11 slower pace,10,11 and increased mediolateral step width and step variability.9,12 Furthermore, when walking blindfolded, at slow and steady pace on a treadmill, the metabolic demand was increased by ∼20%, attributable to reduced stride length, as well as increased step width and vertical clearance of the feet.13 If the energy expenditure would still be increased with restricted peripheral visual field is however unknown.
Individuals engaged in foot-borne operations in complete darkness are typically personnel from the uniformed services that undertake surveillance, security, and rescue operations, while using visual aid in terms of night vision goggles (NVG). Since NVG devices markedly limit the peripheral visual field,14,15 and hence the optical flow,16 that may impact the foot trajectory during walking,10 we reasoned that it would be of interest to evaluate the metabolic demand during walking at moderate speed in darkness with NVG.
Hence, the present aims were to evaluate if the oxygen uptake (|$\dot{\text{V}}$|O2) and kinematics would change when walking in darkness with or without NVG. To establish whether a stereoscopic foveal vision would affect these variables, we used both monocular (Mono) and binocular (Bino) NVG as visual aid. We hypothesized that the metabolic demand would increase because of a changed movement pattern during walking in complete darkness with or without NVG.
METHODS
Subjects
Eleven healthy males (age: 36 ± 14 years, height: 182 ± 7 cm, body mass: 78 ± 13 kg) participated in the study. Subjects were informed about the experimental protocol before giving their consent to participate. The study protocol was approved by the regional Human Ethics Committee at the Karolinska Institute in Stockholm.
Equipment
Treadmill
Walking was conducted on a treadmill (Nordic Sportsmaster, Nesbru, Norway) set at the lowest inclination (+2.3°). The treadmill was equipped with protective railing, and the subject wore a loose-fitting safety harness attached to the ceiling to avoid injury in case of tripping.
NVG
In two of the conditions, head-mounted NVGs were used, either a Mono (mass: ∼600 g) or Bino (∼950 g) (ITL Optronics Ltd, Israel). Because of the complete visual overlap of the two light-amplifying tubes of the Bino, both the Mono and Bino NVGs restricted the visual field to 40°.17 In the Mono condition, the contralateral eye was covered (blinded) by a patch, ensuring that the subject was relying on monoscopic foveal vision.
|$\dot{\text{V}}$|O2, walking economy, ventilation, and heart rate
|$\dot{\text{V}}$|O2 and ventilation (|$\dot{\text{V}}$|E) were measured using a portable metabolic cart (K4b2, Cosmed, Rome, Italy), and heart rate (HR) was monitored telemetrically (Polar Electro Oy, Kempele, Finland). Data were averaged over the entire 10-minute walking period. To assess walking economy, |$\dot{\text{V}}$|O2 was expressed in relation to the body mass and distance covered, i.e., mL/(kg· km).
Ratings of perceived exertion, discomfort, and mental stress
Ratings of perceived exertion (RPE), discomfort, and mental stress were evaluated after each walking trial with a category ratio scale (0 to 1018).
Inertial measurement units
For evaluation of kinematic measurements during all conditions, inertial measurement units (x-IMU, X-Io Technologies Ltd, Bristol, UK) were used. Data were sampled at 131 Hz, stored on an SD card in each IMU, and subsequently saved in a computer. The two IMUs were mounted at the sacrum, and lateral aspect of the left shank, just above the ankle.
Optoelectronic motion capture system
For evaluation of kinematic measurements, an optical motion capture system (ProReflex, Qualisys AB, Gothenburg, Sweden) was used to detect positions of the reflective markers (tracked at 128 Hz). Nine markers were taped to the breathing mask and the skin at the sacrum, vertebra C7, and unilaterally (left side) at the lateral side of the wrist, the lateral malleolus, the distal part of the femur, the distal part of the humerus, the acromion, and the iliac crest. Optical motion capture data were collected during the Light and Dark conditions. For the Mono and Bino conditions, optical motion capture data were not collected since the camera units include infrared stroboscopes, which would interfere with the NVG. The marker data served the purpose of reference motion capture for validation of the IMU-based motion capture. The two IMUs and the optical motion capture system were synchronized using a “clapper.”
Procedures
After instrumentation, the subject was familiarized with the speed (4 km/h) of the treadmill, both fully sighted in a lighted laboratory and blindfolded in complete darkness. Before the experiments, each subject was also familiarized with the NVG devices, which were adjusted so as to optimize visual acuity and comfort. During the experiments, the subject walked at 4 km/h on a treadmill during 10-minute trials, each walking trial (condition) being separated by a 10-minute period, during which he was resting seated while being re-instrumented. The four different conditions were fully sighted in light (Light), complete darkness using blindfold (Dark), or monocular (Mono) or binocular (Bino) NVG. For the individual subject, all conditions were performed during 1 day, and the order was alternated among subjects in a counter-balanced manner.
Data Analysis and Comparison of Kinematic Measurements
Data analyses of kinematic measurements
For the kinematic analysis, 1-minute data were extracted from 3 minutes into the trial, corresponding to approximately 60 gait cycles. The data were divided into separate gait cycles, starting and ending with the initial contact of the left foot, as determined from the peak in the acceleration signal of the IMU at the distal left shank. The orientation of the body segments was determined from the rotation of the IMUs, removing the mean from the gyroscope data and then integrating using the trapezoidal method. The reference orientation of the segment with respect to the vertical was obtained by using data from a short sequence where the subject was standing still. The orientation of the IMU, and hence of the segment to which it was attached, was calculated with respect to this reference orientation.
After the orientation of the IMU/segment was identified, the displacement of the center of the IMU could be estimated. To eliminate the drift, the accelerometer data were first transformed into a static, spatial frame of reference, and a linear trend was removed from each cycle of accelerometer data. The accelerations were then integrated twice.
Validation of kinematic measurements
IMU data were compared to optical motion capture data for four subjects in the Light and Dark conditions. The vertical movement of a point at sacrum has previously been shown to give reasonable approximation of vertical displacement of center of mass,19 and hence the sacrum marker and IMU were considered a proxy for the center of mass. To validate the data, Pearson’s correlation between the two data sets was calculated, and covariance was calculated for each pairwise comparison. Mean values from IMU data corresponded well with optical marker data for the vertical displacement of center of mass (r = 0.99, covariance = 7, Table IA) and were in reasonable agreement for left foot clearance (r = 0.87, covariance = 2, Table IB). Therefore, IMU measurements of vertical displacement of center of mass and left foot clearance were compared for all subjects between conditions. Additionally, IMU measurements of gait frequency, variability of gait frequency (interquartile range), and calculated step length for the left foot were compared between conditions.
Individual Optical Marker Data and IMU Data for (A) Vertical Displacement (cm), (B) Left Foot Clearance (cm), in Four Subjects during Walking with Full Vision in the Lighted Room (L), and in the Dark Room Wearing a Blindfold (D)
. | L-Marker . | L-IMU . | D-Marker . | D-IMU . |
---|---|---|---|---|
A. | ||||
Vertical displacement (cm) | ||||
S4 | 2.4 ± 0.2 | 2.8 ± 0.3 | 3.2 ± 0.3 | 3.6 ± 0.4 |
S6 | 4.0 ± 0.3 | 4.5 ± 0.5 | ||
S10 | 3.5 ± 0.3 | 3.9 ± 0.5 | 4.9 ± 0.4 | 5.0 ± 0.6 |
S12 | 3.3 ± 0.2 | 3.9 ± 0.4 | 5.1 ± 0.3 | 5.2 ± 0.4 |
B. | ||||
Left foot clearance (cm) | ||||
S4 | 15.9 ± 0.2 | 15.6 ± 0.5 | 15.7 ± 0.4 | 15.7 ± 1.1 |
S6 | 16.3 ± 0.4 | 14.6 ± 2.3 | ||
S10 | 17.0 ± 0.5 | 16.9 ± 0.9 | 18.0 ± 0.3 | 17.7 ± 0.8 |
S12 | 18.6 ± 0.3 | 18.6 ± 0.6 | 18.5 ± 0.8 | 17.2 ± 1.3 |
. | L-Marker . | L-IMU . | D-Marker . | D-IMU . |
---|---|---|---|---|
A. | ||||
Vertical displacement (cm) | ||||
S4 | 2.4 ± 0.2 | 2.8 ± 0.3 | 3.2 ± 0.3 | 3.6 ± 0.4 |
S6 | 4.0 ± 0.3 | 4.5 ± 0.5 | ||
S10 | 3.5 ± 0.3 | 3.9 ± 0.5 | 4.9 ± 0.4 | 5.0 ± 0.6 |
S12 | 3.3 ± 0.2 | 3.9 ± 0.4 | 5.1 ± 0.3 | 5.2 ± 0.4 |
B. | ||||
Left foot clearance (cm) | ||||
S4 | 15.9 ± 0.2 | 15.6 ± 0.5 | 15.7 ± 0.4 | 15.7 ± 1.1 |
S6 | 16.3 ± 0.4 | 14.6 ± 2.3 | ||
S10 | 17.0 ± 0.5 | 16.9 ± 0.9 | 18.0 ± 0.3 | 17.7 ± 0.8 |
S12 | 18.6 ± 0.3 | 18.6 ± 0.6 | 18.5 ± 0.8 | 17.2 ± 1.3 |
Values are mean ± SD recorded during minute three.
Individual Optical Marker Data and IMU Data for (A) Vertical Displacement (cm), (B) Left Foot Clearance (cm), in Four Subjects during Walking with Full Vision in the Lighted Room (L), and in the Dark Room Wearing a Blindfold (D)
. | L-Marker . | L-IMU . | D-Marker . | D-IMU . |
---|---|---|---|---|
A. | ||||
Vertical displacement (cm) | ||||
S4 | 2.4 ± 0.2 | 2.8 ± 0.3 | 3.2 ± 0.3 | 3.6 ± 0.4 |
S6 | 4.0 ± 0.3 | 4.5 ± 0.5 | ||
S10 | 3.5 ± 0.3 | 3.9 ± 0.5 | 4.9 ± 0.4 | 5.0 ± 0.6 |
S12 | 3.3 ± 0.2 | 3.9 ± 0.4 | 5.1 ± 0.3 | 5.2 ± 0.4 |
B. | ||||
Left foot clearance (cm) | ||||
S4 | 15.9 ± 0.2 | 15.6 ± 0.5 | 15.7 ± 0.4 | 15.7 ± 1.1 |
S6 | 16.3 ± 0.4 | 14.6 ± 2.3 | ||
S10 | 17.0 ± 0.5 | 16.9 ± 0.9 | 18.0 ± 0.3 | 17.7 ± 0.8 |
S12 | 18.6 ± 0.3 | 18.6 ± 0.6 | 18.5 ± 0.8 | 17.2 ± 1.3 |
. | L-Marker . | L-IMU . | D-Marker . | D-IMU . |
---|---|---|---|---|
A. | ||||
Vertical displacement (cm) | ||||
S4 | 2.4 ± 0.2 | 2.8 ± 0.3 | 3.2 ± 0.3 | 3.6 ± 0.4 |
S6 | 4.0 ± 0.3 | 4.5 ± 0.5 | ||
S10 | 3.5 ± 0.3 | 3.9 ± 0.5 | 4.9 ± 0.4 | 5.0 ± 0.6 |
S12 | 3.3 ± 0.2 | 3.9 ± 0.4 | 5.1 ± 0.3 | 5.2 ± 0.4 |
B. | ||||
Left foot clearance (cm) | ||||
S4 | 15.9 ± 0.2 | 15.6 ± 0.5 | 15.7 ± 0.4 | 15.7 ± 1.1 |
S6 | 16.3 ± 0.4 | 14.6 ± 2.3 | ||
S10 | 17.0 ± 0.5 | 16.9 ± 0.9 | 18.0 ± 0.3 | 17.7 ± 0.8 |
S12 | 18.6 ± 0.3 | 18.6 ± 0.6 | 18.5 ± 0.8 | 17.2 ± 1.3 |
Values are mean ± SD recorded during minute three.
Statistical Analysis
Physiologic and kinematic variables were evaluated using repeated measures ANOVA, followed by Tukey’s post-hoc test to evaluate overall differences between conditions. As a measure of effect size, the Cohen’s d was calculated dividing the difference between sample means by the “pooled” standard deviation. Values of 0.20, 0.50, and above 0.80 were considered as small, medium, and large, respectively. Ratings of RPE, discomfort, and mental stress were evaluated using non-parametric Friedman ANOVA, and for specific comparisons between modes, a Wilcoxon matched pair test. The α-level of significance was set a priori at .05. Values of physiological variables are presented as means ± SD, whereas ordinal data (ratings) are presented as medians (range).
RESULTS
|$\dot{\text{V}}$|O2, Walking Economy, |$\dot{\text{V}}$|E, and HR
There was an overall difference between conditions for |$\dot{\text{V}}$|O2 (F (3, 30) = 6.9, P = 0 .001), with subjects exhibiting 20% higher (P < 0.001, d = 0.94) |$\dot{\text{V}}$|O2 in the Dark than the Light condition (Fig. 1A). Nominally, |$\dot{\text{V}}$|O2 values in the Mono and Bino conditions fell in between those of the Light and Dark conditions. There was, however, no statistically significant difference between the Mono and Light conditions (P = 0.27, d = 0.47) or between the Bino and Light conditions (P = 0.14, d = 0.56). Neither was there any difference between the Dark and Mono conditions (P = 0.06, d = 0.53), between the Dark and Bino conditions (P = 0.13, d = 0.46), or between the Bino and Mono conditions (P = 0.98, d = 0.09).
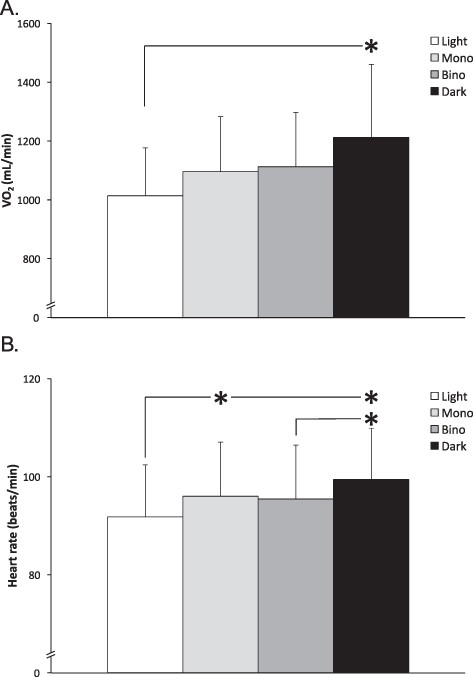
The (A) |$\dot{\text{V}}$|O2 (mL/min) and (B) HR (beats/min), during walking fully sighted in light (Light), in complete darkness using monocular (Mono) or binocular (Bino) NVG, or in complete darkness using blindfold (Dark). Values are mean ± SD. * indicate a statistical difference between conditions.
Likewise, when expressed in relation to the body mass and distance covered, the |$\dot{\text{V}}$|O2 differed between conditions (F (3, 30) = 6.0, P = 0.001). |$\dot{\text{V}}$|O2 was 20% higher in the Dark (235 ± 37 mL/[kg· km]), P < 0.001, d = 1.32) than the Light condition (196 ± 18 mL/[kg· km]), and hence the walking economy was impaired during the Dark condition. There was no difference between the Light and the Mono (212 ± 22 mL/[kg· km]), P = 0.25, d = 0.78) or between the Light and the Bino (215 ± 16 mL/[kg· km]), P = 0.15, d = 1.08) conditions. Neither was there any difference between the Dark and Mono conditions (P = 0.06, d = 0.73), between the Dark and Bino conditions (P = 0.10, d = 0.70), or between the Mono and Bino conditions (P = 0.99, d = 0.13).
There was also an overall difference between conditions for |$\dot{\text{V}}$|E (F (3, 30) = 7.1, P < 0.001), where the |$\dot{\text{V}}$|E was 22% higher during the Dark (31 ± 6 L/min, P < 0.001, d = 1.12) than the Light condition (26 ± 3 L/min). Yet, there was no difference between the Light and Mono conditions (27 ± 4 L/min, P = 0.57, d = 0.49) or between the Light and Bino conditions (28 ± 5 L/min, P = 0.26, d = 0.53). The |$\dot{\text{V}}$|E was greater during the Dark than the Mono condition (P = 0.02, d = 0.78), whereas there was no difference between the Dark and Bino conditions (P = 0.06, d = 0.56) or between the Mono and Bino conditions (P = 0.94, d = 0.16).
Similarly, there was an overall difference between conditions for HR (F (3, 30) = 10.3, P < 0.001), with subjects exhibiting 8% higher (P < 0.001, d = 0.66) HR in the Dark than the Light condition and 5% higher (P = 0.02, d = 0.40) HR in the Mono than Light condition (Fig. 1B). HR in the Bino condition was not different from that in the Light condition (P = 0.06, d = 0.34). HR was higher in the Dark than the Bino condition (P = 0.03, d = 0.35) but did not differ between the Dark and Mono conditions (P = 0.08, d = 0.30).
Kinematics
There was an overall difference between conditions for gait frequency (F (3, 30) = 6.3, P = 0.002) and step length (F (3, 30) = 8.2, P < 0.001), with a higher frequency (0.93 ± 0.14 Hz, P = 0.001, d = 0.85), and thus shorter step length (P < 0.001, d = 0.89, Fig. 2A), in the Dark than the Light condition (0.83 ± 0.07 Hz). Nominally, the gait frequency in the Mono and the Bino conditions was in between those in the Light and the Dark conditions but did not differ between the Light and the Mono (0.88 ± 0.07 Hz, P = 0.15, d = 0.73) or Bino (0.87 ± 0.06 Hz, P = 0.32, d = 0.59) conditions. The step length was 6% shorter in the Mono than Light condition (P = 0.03, d = 0.74) but did not differ between the Bino and Light conditions (P = 0.09, d = 0.62). The gait frequency (P = 0.07, d = 0.51) and the step length (P = 0.09, d = 0.48) did not differ between the Dark and the Bino condition, nor did the gait frequency (P = 0.17, d = 0.42) or step length (P = 0.25, d = 0.36) differ between the Mono and Dark conditions.
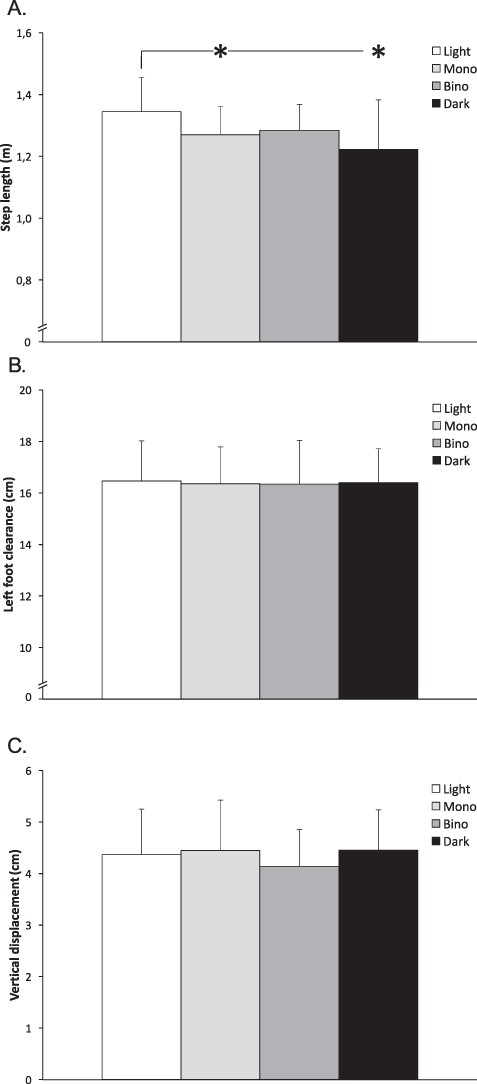
The mean (A) step length (n = 11) and (B) foot clearance for the left foot (n = 11), and (C) vertical displacement of center of mass (n = 9) during walking fully sighted in light (Light), in complete darkness using monocular (Mono) or binocular (Bino) NVG, or in complete darkness using blindfold (Dark). Values are mean ± SD. * indicate a statistical difference between conditions.
The variability in gait frequency did not differ between conditions (Light: 0.022 ± 0.011 Hz, Dark: 0.032 ± 0.019 Hz, Mono: 0.026 ± 0.016 Hz, Bino: 0.023 ± 0.008 Hz, F (3, 30) = 2.2, P = 0.11). There were no differences between conditions for maximal foot clearance (F (3, 30) = 0.1, P = 0.95, Fig. 2B) or for vertical displacement of center of mass (n = 9; F (3, 24) = 1.0, P = 0.40, Fig. 2C).
Ratings of Perceived Exertions, Discomfort, and Mental Stress
All three conditions with limited or no vision were perceived harder than the Light condition (Dark: P = 0.008, Mono: P = 0.01, Bino: P = 0.01, Fig. 3A). The Mono and Bino conditions were not different from each other (P = 0.69) or from the Dark condition (Mono: P = 0.51, Bino: P = 0.72).
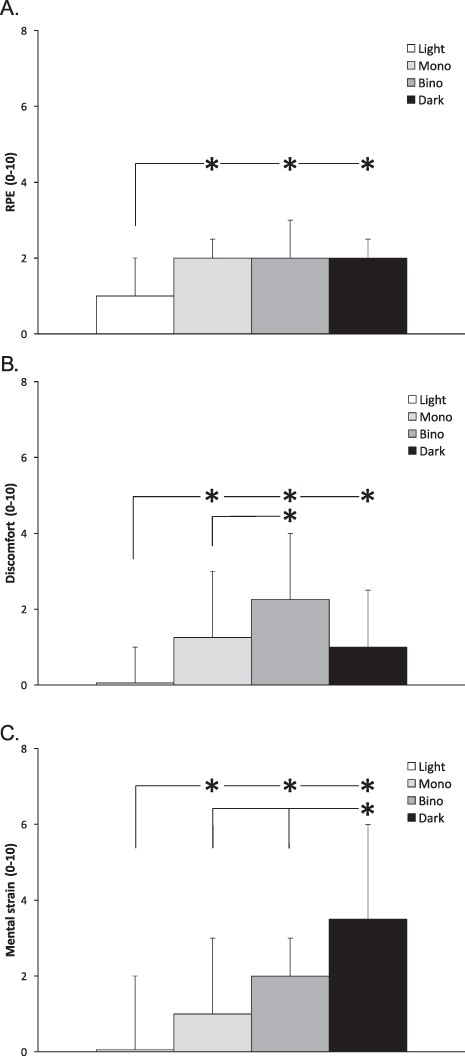
Ratings of (A) perceived exertion (RPE), (B) discomfort, and (C) mental strain during walking fully sighted in light (Light), in complete darkness using monocular (Mono) or binocular (Bino) NVG, or in complete darkness using blindfold (Dark). Median values are presented, and the error bars indicate the maximum value for each condition. * indicate a statistical difference between conditions.
All three conditions with no or limited vision were perceived more uncomfortable than the Light condition (Dark: P < 0.05, Mono: P = 0.008, Bino: P = 0.005, Fig. 3B). The Bino condition was perceived more uncomfortable than the Mono condition (P = 0.008), whereas there was no difference between the Bino and Dark conditions (P = 0.06) or between the Mono and Dark conditions (P = 0.61).
Finally, all three conditions with limited or no vision were more mentally stressful than the Light condition (Dark: P = 0.003, Mono: P = 0.005, Bino: P = 0.005, Fig. 3C). The Dark condition was more stressful than the Mono (P = 0.02) and Bino (P < 0.05) conditions. There was no difference between the two NVG conditions (P = 0.21).
DISCUSSION
The purpose was to compare the metabolic demand and kinematics during level walking, in a fully-sighted (Light) condition, in a blinded (Dark) condition, and in complete darkness with visual aid in terms of Mono and Bino NVG. In accordance with our hypothesis, |$\dot{\text{V}}$|O2 and HR were higher, and step length was shorter, in the Dark than the Light condition, with values from the Mono and Bino conditions in between those of the Dark and Light conditions. Overall, the results showed that all three conditions with limited or no vision were perceived more strenuous, uncomfortable, and mentally stressful than the Light condition.
That the blinded condition resulted in a 20% increase of the metabolic energy demand is in close agreement with our previous investigation.13|$\dot{\text{V}}$|O2 in the NVG conditions did not differ significantly from either that of the Light or the Dark condition. With only 11 participants in the present study, it can be argued that the study lacked sufficient statistical power to conclusively establish a |$\dot{\text{V}}$|O2 difference between NVG conditions and the Light or the Dark conditions. However, since nominal values of |$\dot{\text{V}}$|O2, walking economy, |$\dot{\text{V}}$|E, and HR for the NVG conditions, all fell in between those of the Light and Dark conditions, it is hard to interpret the results in any other way than that the energy demanding alteration induced by temporary blinding the subjects was not fully restored by introducing NVG. Since usage of NVG entails good foveal visual input but causes loss of peripheral visual cues, the results indicate that mechanical efficiency is affected by loss of peripheral vision. Both the Mono and the Bino NVG restrict the visual field to 40°,14,15,17 suggesting that optimization of mechanical efficiency requires a visual field exceeding 40°. It cannot be excluded that other characteristics of the NVG images may have impaired spatial orientation and/or balance and therefore prevented an optimization of the mechanical efficiency. Thus, NVGs may affect not merely the colors of the images but also their contrast and clarity and may, on occasions, add high-frequency noise in the form of scintillations.15
The present subjects showed some individual strategies to adapt to the conditions with limited or no vision, but a shorter step length appeared to be a general precaution to secure balance, which confirms earlier reports on gait effects of limited vision.9,13 While a natural behavior in conditions of limited vision may be to also walk at a slower pace,10,20 increased cadence, to keep up with the treadmill speed,2 was one consequence of the present set-up. Since the metabolic demand will increase if walking at a non-preferable step length,1 it can be estimated that approximately half of the increased energy expenditure in the Dark condition was attributable to the 9% reduction in stride length.21 That the use of NVG did not fully restore the reduced step length (Mono: −6%) indicates that loss of peripheral vision also affects the walking kinematics.
The question arises whether other kinematic alterations than the stride length might have contributed to the increased metabolic demand in the Dark condition and the tendency for ditto in the Mono and Bino conditions. Any insecurity during walking with closed or blindfolded eyes may also be reflected in increased step width and step variability,9,13,22 which may increase the metabolic demand.23 In the present experiments, the step variability in the for-aft direction, i.e., the gait frequency variability, was neither significantly affected by blindfolding nor by NVG and thus did not seem to have a major impact on the metabolic demand.
The vertical displacement of the center of mass during walking at 4 km/h with full vision has been reported to reach 2.4-2.7 cm.24,25 Hence, it is possible that the mean vertical displacement of the center of mass in the present study, i.e., 4.1-4.5 cm, was somewhat overestimated in all conditions, whereas the comparison between conditions should still be valid. Reduced vertical displacement of the center of mass in response to an imposed increase of the cycle frequency has been reported both during full vision treadmill walking25 and during running.26 Yet, despite a substantially increased cycle frequency in the present Dark condition, no difference in vertical displacement of the center of mass was observed. Likewise, Eiken et al.13 reported of no difference in hip vertical displacement between trials with and without vision. Collectively, present and previous13 results suggest that eliminating vision appears to alter the walking strategy so that the vertical displacement of center of mass is kept at a similar level despite increased cadence. The influence of such change in gait kinematics on energy expenditure remains to be settled.
Another common precaution during walking with no or limited vision that possibly augments energy expenditure is increased distance to the ground during the swing phase. Thus, in a study by Graci et al.,10 circumferential-peripheral visual occlusion resulted in greater minimum foot clearance, and in our previous study, blindfolding increased minimum foot clearance by about 20%.13 In the present experiment, we measured maximal foot clearance, the increase of which has been reported to increase the metabolic demand.27 Yet, the present results showed similar foot clearance in all conditions suggesting that it did not substantially contribute to the increased |$\dot{\text{V}}$|O2 in the Dark condition.
One aim of the present study was to evaluate if the stereoscopic vision during the Bino condition would be advantageous compared with wearing the Mono NVG. The results of |$\dot{\text{V}}$|O2, HR, and step length were virtually identical in the Mono and Bino conditions, suggesting that stereoscopic vision was not a key determinant of walking economy. The subjects in the present study could maintain a steady gait focusing straightforward without being concerned with obstacles, whereas persons with loss of peripheral visual field commonly use safety strategies, such as biomechanical adjustments to avoid tripping on obstacles.28,29 Perhaps, in a more unpredictable high-fidelity environment, the effects of reduced visual field with or without stereoscopic vision would have a greater impact on the walking economy. It is therefore warranted to investigate the effects of wearing Mono and Bino NVG on energy expenditure and kinematics in field experiments. However, since the camera-based motion capture system technique is not feasible in a field environment, one prerequisite for performing such study is to establish an alternative method for kinematic analysis. On the basis of the present IMU and motion capture system results, it appears that the use of IMUs, being a wearable technology, holds promise also for field conditions.
A limitation with the present study may be that the walking speed (4 km/h) was slightly slower than the range (4.4-4.8 km/h) that healthy young and middle-aged men typically spontaneously select during level walking.4,5 However, even at considerably slower walking speed (3.3 km/h), blindfolding reduces step length by 10-11%.13 As discussed above, another study limitation is the small number of participants, restricting the statistical power. However, since the results were very consistent in that, for all variables, values obtained in the Mono and Bino conditions nominally fell in between those of the Dark and Light conditions, it appears highly likely that the altered gait and impaired walking economy induced by complete lack of vision were not fully restored by wearing NVG in darkness. Together, the results from the present and previous13 study underline the importance of vision for optimizing the gait mechanical efficiency, even when the walking is performed at slow-to-moderate constant pace on an obstacle-free horizontal surface under highly predictable conditions. Future studies should investigate whether women exhibit similar responses as men during level walking in darkness with and without visual aid.
CONCLUSION
In conclusion, all three conditions with limited or no vision were perceived physically harder, more uncomfortable, and more mentally stressful than the Light condition. |$\dot{\text{V}}$|O2 and HR were higher, and step length was shorter in the blindfolded (Dark) than the fully-sighted (Light) condition, and the Mono and Bino visual aid did not fully abolish the changes of the blinded condition. From an operational perspective, the impacts of impaired vision and use of NVG on gait and metabolic demand, needs to be evaluated in high-fidelity experiments, investigating their effects in varying terrain, at various gait speeds.
ACKNOWLEDGMENTS
The authors would like to thank Björn Johannesson for assisting in the design of the experimental setup.
FUNDING
This work was supported by the Swedish Armed Forces (No: AF 922: 0906). Open access funding provided by KTH Royal Institute of Technology.
CONFLICT OF INTEREST STATEMENT
The authors declare that the research was conducted in the absence of any commercial or financial relationships that could be construed as a potential conflict of interest.
DATA AVAILABILITY
Data supporting the study findings can be requested from the corresponding author (L.N.), but are not publicly available since they contain information that could compromise the privacy of the research participants.
CLINICAL TRIAL REGISTRATION
None declared.
ETHICAL REVIEW BOARD
The study protocol was approved by the regional Human Ethics Committee at the Karolinska Institute in Stockholm.
INSTITUTIONAL ANIMAL CARE AND USE COMMITTEE
Not applicable.
INDIVIDUAL AUTHOR CONTRIBUTION STATEMENT
O.E. conceived the study and all authors were involved in the study design and data collection. L.N., S.N., and K.H. analyzed the data, and all authors contributed to the data interpretation. L.N., K.H., and O.E. drafted the manuscript, and all authors revised the draft and approved the final manuscript.
INSTITUTIONAL CLEARANCE
Not applicable.
REFERENCES
Author notes
Preliminary results are presented at the Nordic Military Sport leaders Conference, September 2014.
The views expressed in this material are exclusively those of the authors and do not reflect the official policy of any domestic authority or the like.