-
PDF
- Split View
-
Views
-
Cite
Cite
Siwei Lv, Zengshun Lin, Junhui Shen, Laifu Luo, Qingguo Xu, Laigeng Li, Jinshan Gui, OsTCP19 coordinates inhibition of lignin biosynthesis and promotion of cellulose biosynthesis to modify lodging resistance in rice, Journal of Experimental Botany, Volume 75, Issue 1, 1 January 2024, Pages 123–136, https://doi.org/10.1093/jxb/erad367
- Share Icon Share
Abstract
Lignin and cellulose are two essential elements of plant secondary cell walls that shape the mechanical characteristics of the culm to prevent lodging. However, how the regulation of the lignin and cellulose composition is combined to achieve optimal mechanical characteristics is unclear. Here, we show that increasing OsTCP19 expression in rice coordinately repressed lignin biosynthesis and promoted cellulose biosynthesis, resulting in enhanced lodging resistance. In contrast, repression of OsTCP19 coordinately promoted lignin biosynthesis and inhibited cellulose biosynthesis, leading to greater susceptibility to lodging. We found that OsTCP19 binds to the promoters of both MYB108 and MYB103L to increase their expression, with the former being responsible for repressing lignin biosynthesis and the latter for promoting cellulose biosynthesis. Moreover, up-regulation of OsTCP19 in fibers improved grain yield and lodging resistance. Thus, our results identify the OsTCP19-OsMYB108/OsMYB103L module as a key regulator of lignin and cellulose production in rice, and open up the possibility for precisely manipulating lignin–cellulose composition to improve culm mechanical properties for lodging resistance.
Introduction
Lodging is a major problem in cereal crops that reduces the amount and quality of yield (Guo et al., 2021). Breeding for lodging-resistant, semi-dwarf cultivars has been widely adopted as an efficient approach for increasing yields in rice and wheat since the ‘green revolution’ in the 1960s (Khush, 1999; Peng et al., 1999). Semi-dwarf cultivars have shorter plant heights, which reduces lodging risk and allows for greater use of nitrogen fertilizers (Wang et al., 2021). However, excessive applications of fertilizer have negative impacts on the environment and on human health (Zhang et al., 2015). Moreover, shorter plant height limits the potential for further increasing grain yield due to shorter panicle length. Lodging resistance also depends on the structural features and the cell wall composition in mechanical tissues such as the sclerenchyma in rice (Zhang et al., 2021).
Cellulose and lignin are two major components of the secondary cell wall (SCW) in mechanical tissues (Zhang et al., 2021). The thickness and composition of the SCW affect the mechanical characteristics and lodging resistance of the culm (Liu and Li, 2016). Cellulose is a crystalline -1,4-glucan polymer that is synthesized on the plasma membrane by cellulose synthase complexes and incorporated into the cell wall (Tanaka et al., 2003). Lignin is a phenylpropanoid polymer that is derived from the polymerization of three monolignols, p-coumaryl alcohol, coniferyl alcohol, and sinapyl alcohol (Gui et al., 2011). Cellulose and lignin have different roles in conferring culm strength. Mutants with impaired cellulose biosynthesis have brittle culms, while mutants with reduced lignin biosynthesis have flexible culms (Y. Li et al., 2003, Li et al., 2009; Zhang et al., 2009, 2011; Wu et al., 2012; Song et al., 2013). Moreover, lignin and cellulose levels usually exhibit a reciprocal compensation pattern when either of them is disrupted (Hu et al., 1999; L. Li et al., 2003; Y. Li et al., 2003; Ambavaram et al., 2011). However, the mechanism of this reciprocal regulation is still unknown.
The formation of the SCW is regulated by a complex transcriptional network that is conserved in plants, but some transcription factors (TFs) have divergent functions in different species (Zhong et al., 2011; Hirano et al., 2013a, 2013b; Zhu and Li, 2021). For example, MYB103L, an ortholog of Arabidopsis MYB103, primarily regulates cellulose biosynthesis in rice, whereas MYB103 regulates syringyl lignin biosynthesis in Arabidopsis (Ohman et al., 2013; Yang et al., 2014; Ye et al., 2015; Wu et al., 2021). The Arabidopsis myb4 mutant accumulates more sinapate ester, and AtMYB4 is thought to be a transcriptional repressor of phenylpropanoid biosynthesis (Jin et al., 2000). MYB108 in rice, a homolog of AtMYB4, is involved in grass-specific p-coumaroylated and tricin lignin biosynthesis (Miyamoto et al., 2019). However, how MYB103L and MYB108 are regulated in rice is unclear.
Teosinte branched1/cycloidea/proliferating cell factor (TCP) proteins are a class of plant-specific transcription factors that play important roles in various aspects of growth and development (Martin-Trillo and Cubas, 2010; Manassero et al., 2013; Nicolas and Cubas, 2016). TCP family genes are divided into two clades (class I and II), which frequently have opposite effects on growth and development (Martin-Trillo and Cubas, 2010). Among them, AtTCP14 and AtTCP15 encode Class-I TCP transcription factors that act redundantly in the regulation of Arabidopsis development, including cell-cycle regulation (Li et al., 2012), seed germination (Resentini et al., 2021), leaf development (Kieffer et al., 2011), floral organ morphogenesis (Lucero et al., 2015; Gastaldi et al., 2020), plant height (Daviere et al., 2014), thermal morphogenesis (Ferrero et al., 2019), and trichome formation (Camoirano et al., 2020). In rice, OsTCP19 and OsTCP5 are each homologs of both AtTCP14 and AtTCP15 that might function redundantly in regulating plant growth and development. OsTCP19 is up-regulated in response to cold, drought, and salt stress (Sharma et al., 2010), and might mediate abiotic stress responses (Mukhopadhyay and Tyagi, 2015). OsTCP19 has also been identified as a genomic locus that affects nitrogen use efficiency by regulating the expression of nitrogen utilization genes (Liu et al., 2021).
In this study, we discovered a novel role of OsTCP19 in rice in coordinating the inhibition of lignin biosynthesis and the stimulation of cellulose biosynthesis. OsTCP19 expression in fibers confers strong lodging resistance and high grain yield. Our study reveals a molecular module that modulates lignin and cellulose biosynthesis in rice culms, thereby providing a potential new strategy for improving culm mechanical characteristics for lodging resistance in crops.
Materials and methods
Plant materials and growth conditions
Oryza sativa subsp. japonica variety Zhonghua 11 (ZH11) was used as the wild type in this study. The full-length coding sequence of OsTCP19 (LOC_Os06g12230) was amplified from ZH11 and cloned into the pEASY-Blunt cloning vector. After sequencing, OsTCP19 was inserted into the pCAMBIA1300::UbiP-VP64 and pCAMBIA1300::UbiP-EAR vectors to fuse it with the VP64 motif (tetrameric repeats of VP16) and the EAR4 motif (tetrameric repeats of EAR), respectively, at the C-terminal. For cell-specific expression, OsTCP19 from pEASY-OsTCP19 and PdDUF579-9P-35Smini from pCAMBIA2300::PdDUF579-9P-35Smini-GUS were subcloned into pCAMBIA1301 to obtain the pCAMBIA1300::PdDUF579-9P-35Smini-OsTCP19 vector. For the binding assay, OsTCP19 was cloned into a binary pHB vector to generate the 35S::OsTCP19-3Flag construct. For the subcellular localization assay, OsTCP19 was cloned into a binary pm-rk vector to generate the 35S::OsTCP19-mCherry construct, which was introduced into Agrobacterium tumefaciens and then transformed into tobacco (Nicotiana benthamiana). In addition, the sgRNA target site of MYB108 (LOC_ Os09g36730) was designed (http://skl.scau.edu.cn/targetdesign/), assembled into sgRNA expression cassettes, and then mobilized into CRISPR/Cas9 binary vectors (Ma et al., 2015). All the primers used in this study are listed in Supplementary Table S1. The constructs were introduced into rice calli via Agrobacterium tumefaciens EHA105 to regenerate transgenic rice plants (Yukoh et al., 1994). The plant materials were grown either in a phytotron under a 12/12 h photoperiod (~200–250 μmol m–2 s–1) at constant 28 °C and 60% relative humidity, or in a nitrogen-rich paddy field at the CAS Center for Excellence in Molecular Plant Sciences, Shanghai (39.937128°N, 121.122304°E).
Gene expression analysis
The third basal internode tissues of 2-month-old plants were collected for qRT-PCR analysis. Total RNA was extracted from the tissues using a plant RNA kit (Omega Bio-tek). About 2 μg of total RNA of each sample was reverse-transcribed using a TransScript One-Step gDNA Removal and cDNA Synthesis SuperMix kit (TransGen Biotech). Gene-specific primers were designed for PCR amplification (Supplementary Table 1). Quantitative PCR was performed using SYBR Green (TransStart Tip Green qPCR SuperMix) with a QuantStudio 3 Real-Time PCR System (Applied Biosystems). The gene expression data were normalized using the 2–ΔΔCT method with three biological replicates, based on the rice housekeeping genes UBIQUITIN1 (LOC_Os03g13170) and ACTIN1 (LOC_Os03g50885), which are among the most stable reference genes (Gui et al., 2014; Pabuayon et al., 2016).
Measurement of mechanical properties
Breaking force and extensibility of 3-month-old rice culms were measured using a mechanical testing machine (HY-0580, http://www.hengyiyiqi.com/en/home.asp). The cross-section area of the third internode was calculated as (a1b1−a2b2)π/4, where a1 and a2 represent the outer and inner diameters of the major axis, respectively, while b1 and b2 represent the outer and inner diameters along the minor axis, respectively. We then placed the samples between the grips of the machine and applied a tensile force until they broke. The breaking force was recorded as the maximum force required for breaking the samples. Extensibility was expressed as an elongation ratio of the distance extended at breaking versus the distance between the grips (Gui et al., 2011). Tensile strength was calculated as the breaking force per unit cross-section area.
The lodging index was measured for rice plants at 3-month-old. The breaking strength of the third internode was measured by a three-point bending test using the HY-0580 machine. The distance between the two fulcrum points (D) was 5 cm and the force applied to break the internode (F) was recorded. The breaking strength (BS, g cm) was then calculated as BS = D×L/4. The bending moment (BM, g cm) was calculated as the product of the length (L) and fresh weight (FW) of the tissues from the third internode to the top of the panicle: BM = L×FW. The lodging index (LI) was then calculated as the ratio of the bending moment to the breaking strength: LI = BM/BS.
Lodging was defined as any deviation of the stem from the vertical position by more than 45°. Lodging occurrence was calculated as the ratio of lodged plants to total plants in each 1 × 3 m field plot at maturity.
Histochemical staining
Phloroglucinol-HCl staining was performed as described by Gui et al. (2011). Briefly, cross-sections of 3-month-old rice culms were stained with 1% phloroglucinol (w/v) in 12% HCl for 5 min and immediately observed under a microscope (Olympus BX53). For cellulose staining, paraffin-embedded tissues were sectioned at 14 μm thickness and stained with a 0.05% aqueous solution of calcofluor (Fluorescent Brightener 28; Sigma) for 2 min and visualized under the Olympus BX53 fluorescence microscope using a GFP filter set.
Cell-wall component analysis
Samples were collected, dried at 65 °C, turned into powder using a ball-mill, and then filtered through a 60-mesh sieve in preparation for determination of alcohol-insoluble cell-wall residues (AIRs) as described by Gui et al. (2020). Crystalline cellulose content was determined as described by Foster et al. (2010b). Briefly, 2 mg of each dried AIR sample was treated with 2 M trifluoroacetic acid (TFA) at 121 °C for 90 min. The insoluble precipitate was collected and hydrolysed with Updegraff reagent (acetic acid:nitric acid:water, 8:1:2 v/v) at 100 °C for 30 min. After being washed with H2O and acetone, the precipitate was incubated with 72% sulfuric acid at room temperature for 1 h. The crystalline cellulose content was determined by anthrone assay. The acetyl bromide-soluble lignin content was determined according to Foster et al. (2010a). Briefly, 1 mg of each dried AIR sample was treated with freshly made acetyl bromide solution (25% v/v acetyl bromide in glacial acetic acid) at 50 °C for 3 h. After being cooled on ice, the treated sample was mixed with 2 M NaOH, 0.5 M fresh hydroxylamine hydrochloride, and acetic acid. The lignin content was measured using a microplate reader (Varioskan Flash, Thermo Scientific).
RNA-sequencing analysis
The third basal internode tissues of 2-month-old plants were collected for RNA-sequencing (RNA-seq) analysis. RNA isolation, cDNA library preparation, and sequencing were performed by Personal Gene (Nanjing, China). The 150 bp paired-end of each sample was sequenced using an Illumina NovaSeq 6000 with three biological replicates. Low-quality reads were removed from raw reads using the NGS QC Toolkit. After quality control, the clean reads were mapped to the Nipponbare reference genome (http://rice.uga.edu/) using HISAT2. Gene expression counts were generated using HTseq with default parameters and the expression level was calculated as fragments per kilobase per million reads (FPKM). Differentially expressed genes (DEGs) were determined using the R package DESeq2 with a cut-off of P-value <0.05 and |log2(fold-change)| ≥1. The gene ontology (GO) analysis and the Kyoto Encyclopedia of Genes and Genomes (KEGG) pathway analysis of DEGs were performed using the topGO and KAAS software, respectively.
EMSAs
The coding sequence of OsTCP19 was amplified and cloned into the pET-28b recombinant vector, and fused with a His6 tag at the C terminus (Novagen). The OsTCP19-His protein was then expressed in the E. coli BL21 (DE3) strain (Invitrogen) and purified using Ni-NTA resin (Qiagen). The promoter fragments of MYB108 (–1709 to –1483 bp upstream of ATG) and MYB103L (–2372 to –2205 bp upstream of ATG) and their respective mutated promoter fragments (generated by site-directed mutation) were obtained by PCR amplification with Cy5-labeled fluorescent primer or unlabeled competition primers. The OsTCP19 protein was incubated with the MYB108/MYB103L probe in binding buffer (20 mM Tris pH 7.5, 10 mM MgCl2, 1 mM DTT, 100 μg ml–1 sperm DNA, 40 μg m l–1 BSA, 5% glycerol) for 20 min at room temperature. The protein–DNA mixtures were separated on a 6% (w/v) native PAGE and imaged using a Typhoon FLA 9000 imager (GE Healthcare).
ChIP-PCR assays
Transgenic OsTCP19-3Flag and wild-type seedlings at 3-week-old were used for ChIP-PCR assays using a Magna ChIP A/G Kit (Millipore). The cells were lysed and the chromatin was sonicated to fragment the genome DNA. The DNA–protein mixture was incubated with anti-Flag antibody (Abmart) and then conjugated to protein A/G-Magna Beads (Millipore) to immune-precipitate the DNA–protein complex. The enriched complex was then reversely cross-linked to release the DNA fragments and dissolved in distilled H2O. Wild-type rice was processed in parallel as a negative control. The bound DNA fragments were analysed using real-time quantitative PCR. The expression levels were obtained by normalizing against the input.
Dual-luciferase reporter assays
Rice protoplasts were isolated according the method described by Gui et al. (2016). The 2.4 kb MYB103L promoter and 3.5 kb MYB108 promoter were each cloned into the pGreen II 0800-LUC reporter vector. The OsTCP19 coding sequence was cloned into each of the pCAMBIA1300::UbiP, pCAMBIA1300::UbiP-VP64, and pCAMBIA1300::UbiP-EAR effector vectors. The protoplasts were co-transfected with the effector and reporter plasmids and incubated for ~12–16 h at 25 °C in the dark. The LUC activities were measured in three replicates using a Dual-Luciferase Reporter Assay System (Promega), and are presented as the ratio of firefly to Renilla LUC (LUC/REN).
Immunolocalization assays
For immunolocalization, the third basal internode tissues of 2-month-old PdDUF579-9P-35Smini::OsTCP19-3Flag transgenic plants were fixed and embedded using a microwave-accelerated acetone-fixation and paraffin-embedding protocol (Tang et al., 2006). Immunolocalization was performed as described by Song et al. (2010) using the anti-Flag primary antibody (Abmart) and the goat anti-mouse secondary antibody linked to alkaline phosphatase (ThermoFisher Scientific).
Results
OsTCP19 affects rice lodging resistance by changing the mechanical characteristics of the culm
The creation of hybrid transcription factors (HTF) is regarded as an efficient method for studying functionally redundant transcription factors in plants. To control the expression of target genes, transcription factors are coupled with a repression module, EAR (tetrameric repetitions of EAR) or a transcriptional activation module, VP64 (tetrameric repeats of VP16). Using this technique, we generated a pool of transgenic rice plants that expressed different HTFs in the culm, and screened for two HTFs based on OsTCP19 (LOC Os06g12230) that displayed opposite lodging characteristics, namely OsTCP19-EAR (designated as TE; OsTCP19 dominant-negative chimeric repressor plants) and OsTCP19-VP64 (designated as TV; OsTCP19 chimeric activator plants) (Supplementary Fig. S1). We obtained 35 independent TE lines and 26 independent TV lines, and compared their phenotypes with wild-type (WT) plants. The TE plants showed dwarfism, shorter internodes, droopy leaves, more tillers, and heavier grains, whereas the TV plants exhibited normal plant height, erect leaves and panicles, fewer tillers, and lighter grains (Fig. 1A; Supplementary Fig. S2). The TE plants were more susceptible to lodging than the WT, while the TV plants were more resistant (lodging index lowered by ~50%) (Fig. 1B). Meanwhile, the tensile strength and elongation ratio of the culm was dramatically reduced in TE plants and significantly increased in TV plants (Fig. 1C, D). These results suggested that OsTCP19 regulates culm development and lodging resistance in rice.
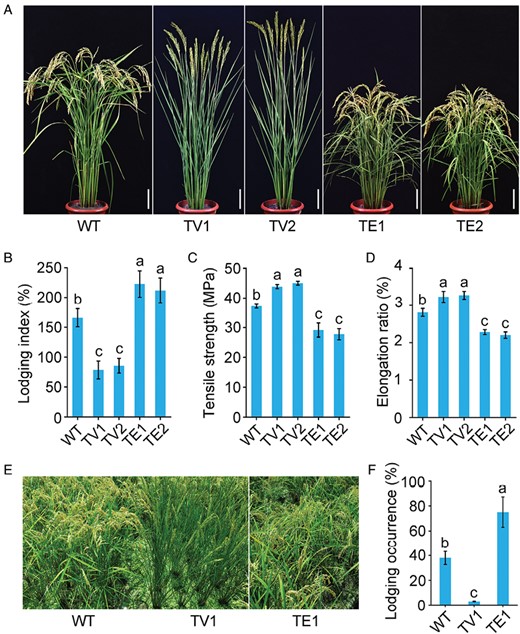
OsTCP19 regulates rice lodging resistance by changing the mechanical characteristics of the culm. (A) Morphology at the grain-filling stage of the wild type (WT), two OsTCP19 chimeric activator lines (TV; OsTCP19-VP64), and two OsTCP19 dominant-negative chimeric repressor lines (TE; OsTCP19-EAR). Scale bars are10 cm. (B) Plant lodging index at the mature stage, determined as the ratio of the bending moment to the breaking strength of the culm. (C) Tensile strength and (D) elongation ratio of the third internodes of the culms. (E) Representative images of mature plants grown in a nitrogen-rich paddy field. (F) Lodging occurrence percentage at the mature stage in the field. Data are means (±SE) for n=6 (B) and n=8 (C, D) replicate plants, and n=10 replicate plots (F). Different letters indicate significant differences among means as determined using ANOVA followed by Tukey’s post-hoc test (P<0.01).
To further evaluate the effect of OsTCP19-overexpression on lodging resistance, we grew TV, TE, and WT plants in a nitrogen-rich paddy field with 10 replicate plots each. The TV plants had a more erect architecture and less lodging at maturity, whereas the TE plants had more severe lodging and brittle culms (Fig. 1E). Compared to the WT, the lodging occurrence in TV plants was reduced by 80–90%, whereas in the TE plants it was increased by 70–85%. (Fig. 1F). These results indicated that increasing the expression of OsTCP19 (TV) enhanced lodging resistance, while suppressing OsTCP19 (TE) impaired lodging resistance in the field.
OsTCP19 influences both lignin and cellulose biosynthesis
Lignin and cellulose are two key components of the secondary cell wall (SCW) that provide mechanical strength to plants. We examined the sclerenchyma fiber cells and found that they had different levels of lignification in the TE and TV plants compared to the WT, with the TE plants showing increased lignification, while the TV plants showed a decrease (Fig. 2A). Lignin abundance in the SCW is generally associated with cell wall mechanical strength and culm lodging resistance, and therefore inhibiting lignin deposition frequently leads to a decline in mechanical strength. Surprisingly, we found that the TE plants had higher lignification but lower mechanical strength. We further investigated the SCW composition and discovered that the TE plants had 15% more lignin content but 10% less crystalline cellulose content than the WT plants (Fig. 2B, C). In contrast, the TV plants had 20% less lignin but 12% more crystalline cellulose than the WT. These results suggested that modification of OsTCP19 influenced both lignin and cellulose deposition, and in an opposite manner.
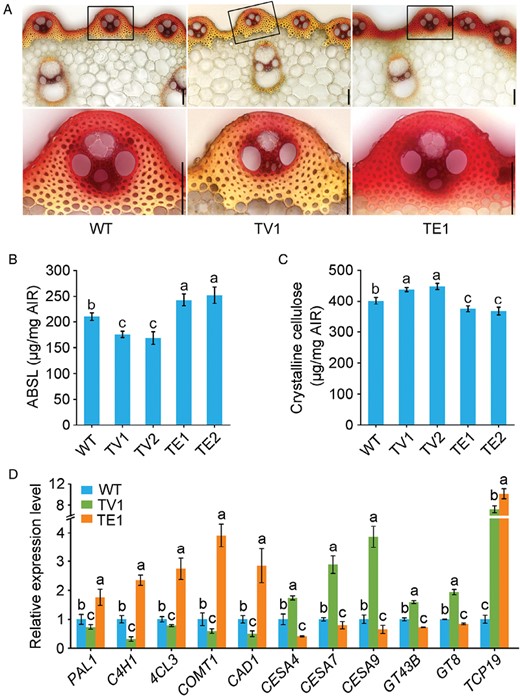
OsTCP19 expression affects both lignin and cellulose biosynthesis in rice. Measurements were conducted on wild-type (WT) rice and transgenic OsTCP19-VP64 (TV) and OsTCP19-EAR (TE) plants. (A) Cross-sections of the third internode stained with phloroglucinol-HCl for lignin detection (upper panels). Scale bars are 500 μm. Magnified images of the vascular cells indicated with rectangles are shown in the lower panels; scale bars 50 μm. (B) Lignin and (C) crystalline cellulose contents in stems of mature plants. ABSL, acetyl bromide-soluble lignin; AIR, alcohol-insoluble cell-wall residue. (D) Expression of genes involved in secondary cell wall biosynthesis in the third basal internode tissues of 2-month-old WT, TV, and TE plants, as determined by qRT-PCR. Expression is relative to that in the WT, the value of which was set as 1. UBIQUITIN1 was used as the reference gene; for results using ACTIN1 as the reference see Supplementary Fig. S3. All data are means (±SE) of three biological replicates. Different letters indicate significant differences among means as determined using ANOVA followed by Tukey’s post-hoc test (P<0.01).
To confirm its role in lignin and cellulose biosynthesis, we examined the abundance of transcripts of OsTCP19 together with those of SCW biosynthesis genes in WT, TV, and TE plants, and found that OsTCP19 was significantly up-regulated in the TV and TE plants (Fig. 2D; Supplementary Fig. S3). We also found that the lignin biosynthesis genes PAL1, C4H1, C3H1, 4CL3, F5H1, COMT1, and CAD1 were significantly up-regulated in TE plants, whereas the cellulose biosynthesis genes CESA4, CESA7, CESA9 and the hemicellulose biosynthesis genes GT43B and GT8 were down-regulated in TE plants. The opposite pattern was observed in the TV plants with lignin biosynthesis genes being markedly down-regulated, and cellulose and hemicellulose biosynthesis genes being up-regulated. Hence, our results indicated that OsTCP19 has a dual function of promoting cellulose biosynthesis and inhibiting lignin biosynthesis in sclerenchyma fibers.
OsTCP19 controls the transcriptional network that governs SCW biosynthesis
RNA-seq analysis was performed on stem tissues from WT, TE, and TV plants to explore how OsTCP19 affected SCW biosynthesis. Compared with the WT, we identified 1637 differentially expressed genes (DEGs) in the TE plants and 1925 in the TV plants (Supplementary Dataset S1). GO enrichment analysis showed that the DEGs were significantly enriched in secondary metabolism in both the TE and TV plants (Fig. 3A). KEGG pathway enrichment analysis revealed that the DEGs were primarily concentrated in the phenylpropanoid biosynthesis pathway (Fig. 3B), which is a metabolic process leading to lignin biosynthesis. Among them, lignin biosynthesis genes including PAL1, 4CL2, 4CL5, CCoAOMT1, and CAD1 were up-regulated in the TE plants and down-regulated in the TV plants (Fig. 3C). In contrast, genes for the biosynthesis of cellulose (CESA4, CESA7, CESA9) and hemicellulose (GT47A and XAT3) were down-regulated in the TE plants and up-regulated in the TV plants. qRT-PCR analysis confirmed that lignin and cellulose biosynthesis genes were oppositely regulated in the OsTCP19-modified plants (Figs 2D, 3D), suggesting that OsTCP19 might have an antagonistic function.
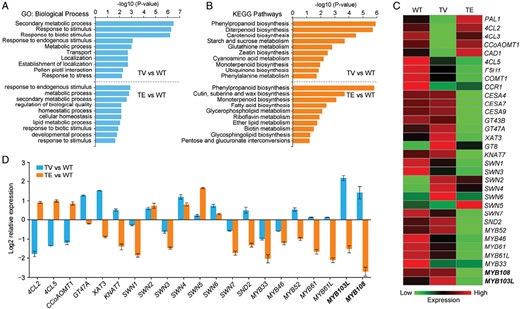
OsTCP19 regulates the expression of genes related to secondary cell wall (SCW) formation in rice. Gene expression was determined in wild-type (WT) rice and transgenic OsTCP19-VP64 (TV) and OsTCP19-EAR (TE) plants. (A) GO and (B) KEGG enrichment analysis of the differentially expressed genes (DEGs) in the culms of TV and TE compared to the WT. The top 10 enriched GO biological process and KEGG pathway terms are shown. A full list of DEGs is given in Supplementary Dataset S1. Three biological replicates were used for each genotype. (C) Heatmap of the expression of genes involved in SCW formation in WT, TV, and TE plants. (D) qRT-PCR validation of the effect of OsTCP19 on the expression of genes related to SCW formation. Data are means (±SE) of three biological replicates.
To understand how OsTCP19 mediated the regulation of lignin and cellulose synthesis in the transgenic plants, we examined the differential expression of transcription factors involved in SCW formation. We found that several genes encoding SECONDARY WALL NAC DOMAIN PROTEIN transcription factors were differently expressed (Fig. 3C, D). The expression of MYB108 and MYB103L, which are both R2R3-MYB transcription factors, was lower in the TE plants and higher in the TV plants compared to the WT. MYB103L has been reported to affect cell-wall mechanical strength by regulating cellulose biosynthesis (Yang et al., 2014; Ye et al., 2015; Wu et al., 2021), whilst MYB108 is involved in the biosynthesis of grass-specific p-coumaroylated and tricin lignins (Miyamoto et al., 2019).
OsTCP19 activates MYB108 and MYB103L by binding to their promoters
Given the alterations in their expression in the transgenic plants, we examined whether OsTCP19 could directly activate MYB108 and MYB103L by binding to their promoters. We first checked the subcellular localization of OsTCP19, and observed that OsTCP19-mCherry and DAPI were co-localized in the nucleus of tobacco leaf cells, indicating that OsTCP19 is a nuclear protein (Fig. 4A). We then generated and purified recombinant OsTCP19 protein from E. coli and performed EMSAs with 226 bp and 167 bp promoter fragments of MYB108 and MYB103L as probes, respectively (Supplementary Fig. S4). We found that OsTCP19 bound to both the MYB108 and MYB103L promoters (Fig. 4B). The protein–DNA binding was abolished when an excess of the unlabeled probe was used or the binding sites were mutated. To verify the binding in vivo, we generated 35S::OsTCP19-3Flag transgenic rice and used anti-Flag antibodies for ChIP assays, which showed that DNA fragments from the MYB108 and MYB103L promoters were significantly enriched (Fig. 4C). These results suggested that OsTCP19 binds to both the MYB108 and MYB103L promoters.
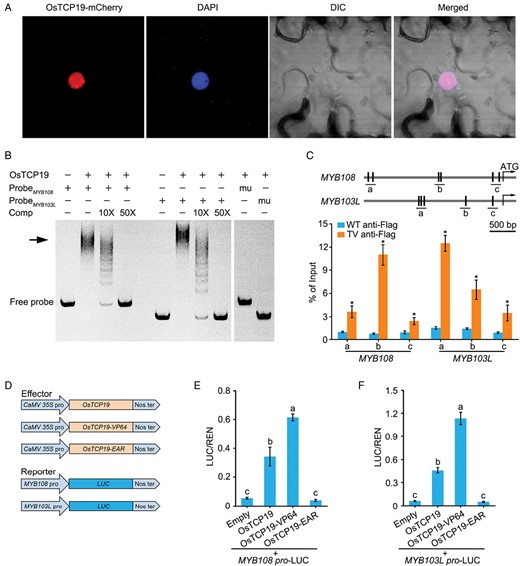
OsTCP19 binds to the promoters of MYB108 and MYB103L. (A) OsTCP19 is localized in nuclei. OsTCP19 was fused with mCherry and transformed into Nicotiana benthamiana leaf epidermal cells, where it co-localized with the DAPI-stained nucleus. (B) EMSAs showing OsTCP19 binding to the promoters of MYB108 and MYB103L. The arrow indicates the promoter–OsTCP19 complex. mu, mutated promoter; Comp, unlabeled cold probe. (C) ChIP-PCR analysis of OsTCP19 binding to the promoters of MYB108 and MYB103L. the promoter regions of MYB108 and MYB103L are shown in the diagram at the top. The vertical lines represent the OsTCP19 binding sites, and the letters indicate the DNA fragment that was used for ChIP-PCR. The rice wild-type (WT) and the T3 generation of the transgenic OsTCP19-VP64 (TV) line were used for immunoprecipitation with the ant-Flag antibody. qRT-PCR assays of the immunoprecipitated DNA were used to show the association of OsTCP19 with the promoters of MYB108 and MYB103L. Data are means (±SE) of three biological replicates. Student’s t-test was used to determine significant differences compared with the WT: *P<0.01. (D–F) Dual-luciferase reporter assays for OsTCP19, OsTCP19-VP64, and OsTCP19-EAR activating MYB108 and MYB103L expression in rice protoplasts. (D) Diagrams of the effector and reporter vectors. The relative luciferase activity for the promoter of (E) MYB108 and (F) MYB103L, calculated as the firefly luciferase/Renilla luciferase ratio. Data are means (±SE) of five replicates. Different letters indicate significant differences among means as determined using ANOVA followed by Tukey’s post-hoc test (P<0.01).
We next used dual-luciferase reporter assays to examine whether OsTCP19 could activate the expression of MYB108 and MYB103L. We constructed reporter vectors with the 2.4 kb and 3.5 kb promoters of MYB103L and MYB108, respectively, driving LUC expression, and effector vectors with the 35S promoter driving the expression of OsTCP19, OsTCP19-VP64, or OsTCP19-EAR (Fig. 4D). We co-transformed these vectors into rice protoplasts and found that OsTCP19 significantly increased LUC activity, and this effect was relatively stronger in OsTCP19-VP64 and weaker in OsTCP19-EAR (Fig. 4E, F). These results suggested that OsTCP19 directly activates both MYB108 and MYB103L by binding to their promoters.
MYB108 is a repressor of lignin biosynthesis in sclerenchyma fibers
To investigate the function of MYB108, we used CRISPR/Cas9 genome-editing to generate MYB108-knockout mutants (ME). For detailed functional characterization, two homozygous mutant lines were used, namely ME1 with a one-base deletion and ME2 with a two-base deletion (Supplementary Fig. S5). Both mutants had compact plant architecture, normal height, and erect leaf blades compared to the WT (Fig. 5A). Analysis of mechanical properties revealed that strength was significantly improved in both mutants, as evidenced by an 18% increase in tensile strength without any significant change in the elongation ratio (Fig. 5B, C). Chemical examination of culms showed that the lignin content was increased by ~17% in the mutants while the crystalline cellulose content was unchanged (Fig. 5D, E), suggesting that MYB108 regulates lignin synthesis but not cellulose synthesis in rice. In addition, histochemical staining showed that the ME mutants had more lignin deposition in sclerenchyma fibers than the WT (Fig. 5F).
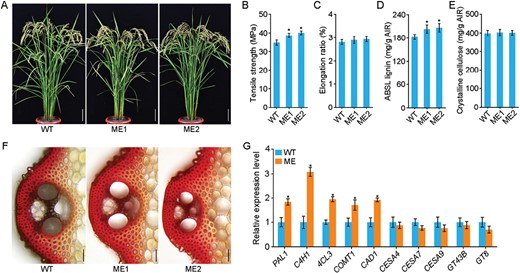
MYB108 suppresses lignin biosynthesis in rice sclerenchyma cells. (A) Morphology of MYB108 mutants (ME) at the grain-filling stage. The mutants were generated by CRISPR/Cas9 genome editing; the wild type (WT) is the transgenic line without edited mutation. Scale bars are 10 cm. (B) Tensile strength and (C) elongation ratio of the third internodes of the culms. Data are means (±SE) of six biological replicates. (D) Lignin and (E) crystalline cellulose contents in stems of mature plants. Data are means (±SE) of three biological replicates. ABSL, acetyl bromide-soluble lignin; AIR, alcohol-insoluble cell-wall residue. (F) Cross-sections of the third internodes of the WT and ME lines stained with phloroglucinol-HCl for lignin detection. Scale bars are 50 μm. (G) Expression of genes involved in secondary cell wall biosynthesis in the third basal internode tissues of 2-month-old WT and ME plants, as determined by qRT-PCR. Expression is relative to that in the WT, the value of which was set as 1. UBIQUITIN1 was used as the reference gene; for results using ACTIN1 as the reference see Supplementary Fig. S6. Data are means (±SE) of three biological replicates. Student’s t-test was used to determine significant differences compared with the WT in each case: *P<0.01.
We also examined the expression of SCW biosynthesis genes and found that those associated with lignin (PAL1, C4H1, 4CL3, COMT1, and CAD1) were up-regulated in the ME lines, while those associated with cellulose (CESA4, CESA7, CESA9) and hemicellulose (GT43B and GT8) genes were not significantly changed (Fig. 5G; Supplementary Fig. S6). Overall, these results indicated that MYB108 is a negative regulator of lignin biosynthesis in sclerenchyma fiber cells.
Fiber-specific expression of OsTCP19 improves lodging resistance and grain yield
We expressed OsTCP19 under the fiber-specific promoter PdDUF579-9P-35Smini to avoid the negative effects of constitutive OsTCP19-overexpression (TV) on grain yield. We generated 23 independent transgenic lines expressing OsTCP19, designated as TOE. The cell-type-specific expression of OsTCP19-3Flag in the TOE transgenics was confirmed by immunolocalization with anti-Flag antibodies, which showed that OsTCP19-3Flag was specifically localized in fibre cells (Supplementary Fig. S7). We selected two lines (TOE1 and TOE2) for detailed studies, of which had compact plant architecture, normal height and tillering, erect leaf blades, and large panicles compared to the WT (Fig. 6A, B; Supplementary Fig. S8). Unlike the TV lines, the TOE transgenics did not show reduced grain yield (Fig. 6C). Moreover, the TOE lines showed increased mechanical strength (Fig. 6D, F). Histochemical staining showed that the TOE lines had more cellulose but less lignin deposited in sclerenchyma fiber cells than the WT (Fig. 6E). Further chemical analysis confirmed this, showing a 13% increase in crystalline cellulose and a 20% decrease in lignin content in the TOE lines (Fig. 6G, H). These results suggested that fiber-specific overexpression of OsTCP19 was able modulate lignin and cellulose biosynthesis with the potential to develop rice plants with high lodging resistance but without compromised grain yield.
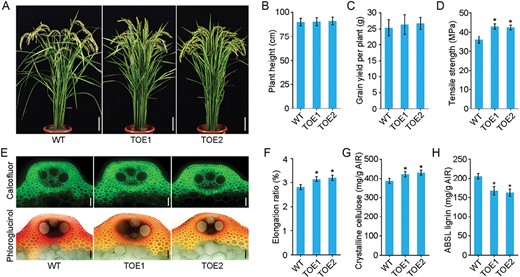
Fiber-specific overexpression of OsTCP19 increases rice lodging resistance without affecting grain yield. The fiber-specific promoter PdDUF579-9P-35Smini was used to produce targeted overexpression of OsTCP19 (TOE). (A) Morphology the rice wild-type (WT) and two TOE lines at the grain-filling stage. Scale bars are 10 cm. (B) Plant height and (C) grain yield per plant in the WT and TOE plants at the maturity. Data are means (±SE) of 20 plants. (D) Tensile strength of the third internodes of the culms. Data are means (±SE) of six biological replicates. (E) Cross-sections of the third basal internode of the WT and TOE plants stained with calcofluor for cellulose (top) and with phloroglucinol-HCl for lignin (bottom). Scale bars are 50 μm. (F) Elongation ratio of the third basal internodes of rice culms. Data are means (±SE) of six biological replicates. (G) Crystalline cellulose and (H) lignin contents in stems of mature plants. ABSL, acetyl bromide-soluble lignin; AIR, alcohol-insoluble cell-wall residue. Data are means (±SE) of three biological replicates. Student’s t-test was used to determine significant differences compared with the WT in each case: *P<0.01).
Discussion
Lignin and cellulose are the main SCW components that provide mechanical strength for lodging resistance. The synthesis of lignin and cellulose frequently exhibits a pattern of reciprocal compensation (Hu et al., 1999; L. Li et al., 2003; Y. Li et al., 2003; Ambavaram et al., 2011); however, to date it is unknown how this reciprocal synthesis is controlled.
OsTCP19 plays a dual role in inhibiting lignin biosynthesis and promoting cellulose biosynthesis
A complex transcriptional regulatory network controls lignin and cellulose synthesis during SCW formation in plants (Zhong and Ye, 2007; Zhang et al., 2021; Zhu and Li, 2021). In this hierarchical network, the top-layer transcription factors, such as SND1 and NST1, and the second-layer transcription factors, such as MYB46 and MYB83, regulate secondary-wall polymer synthesis (Mitsuda et al., 2007; Zhong et al., 2007, 2008). The third-layer transcription factors divergently regulate SCW formation by controlling either lignin or cellulose biosynthesis. For instance, MYB58, MYB63, and MYB85 specifically increase lignin biosynthesis, while MYB4 and LTF1 repress lignin biosynthesis (Zhou et al., 2009; Gui et al., 2019; Geng et al., 2020). On the other hand, MYB103L primarily regulates cellulose biosynthesis in rice, whereas MYB103 is essential for syringyl lignin biosynthesis in Arabidopsis (Ohman et al., 2013; Yang et al., 2014; Ye et al., 2015; Wu et al., 2021). Thus, SCW formation is controlled by a complex transcriptional regulatory network to finely coordinate multiple secondary-wall polymer syntheses.
In this study, we identified OsTCP19 as a transcription factor that regulates both lignin and cellulose synthesis by screening HTF transgenic rice. Overexpression of OsTCP19 decreased lignin but increased cellulose deposition (Fig. 2A–C). In contrast, suppressing its transcriptional activity resulted in an increase in lignin and a decrease in cellulose. The changes in lignin and cellulose deposition in the transgenics were well correlated with the expression levels of lignin and cellulose biosynthesis genes (Fig. 2D). This suggests that OsTCP19 has a dual function of inhibiting lignin biosynthesis genes and promoting cellulose biosynthesis genes. A genome-wide association study has recently identified OsTCP19 as a regulator of the tillering response to nitrogen (Liu et al., 2021), with a 29 bp mutation in the OsTCP19 promoter causing a distinct nitrogen response. Compared with a 29 bp insertion promoter (OsTCP19-L), a 29 bp deletion promoter (OsTCP19-H) exhibited a stronger negative response to nitrogen. Thus, OsTCP19 expression in response to nitrogen is regulated by variations in the promoter. Since nitrogen influences the culm mechanical strength in rice (Zhang et al., 2017), it would be interesting to further investigate whether such OsTCP19 promoter variation also regulates cell wall biosynthesis.
OsTCP19 coordinates lignin and cellulose biosynthesis by controlling the expression of MYB108 and MYB103L
Transcriptome analysis of transgenic OsTCP19-modified rice revealed two differentially expressed transcription factor genes, MYB108 and MYB103L, whose expression was up- or down-regulated in a manner that corresponded well with the specific modification of OsTCP19 (Fig. 3C), implying that they could be targets of the OsTCP19 protein. The detailed analysis resulted in binding sites in the MYB108 and MYB103L promoters being discovered. We confirmed that OsTCP19 directly activated the expression of MYB108 and MYB103L using EMSAs, ChIP-PCR, and dual-luciferase reporter assays (Fig. 34), and thus it was simultaneously able to regulate the transcription of both genes. MYB108 has been reported to regulate p-coumaroylated and tricin lignin production in rice (Miyamoto et al., 2019). We found that MYB108-knockout increased lignin deposition (Fig. 5D, F), indicating that MYB108 acts as a repressor of lignin synthesis in rice. MYB103L, on the other hand, is well known for its role in stimulating cellulose synthesis in rice (Yang et al., 2014; Ye et al., 2015; Wu et al., 2021).On the basis of our results, we therefore propose that OsTCP19 and MYB108/MYB103L form a molecular module that regulates the reciprocal synthesis of lignin and cellulose in rice (Fig. 7). Alternatively, OsTCP19 might share this function with other TCP family members, but their roles have not yet been uncovered.
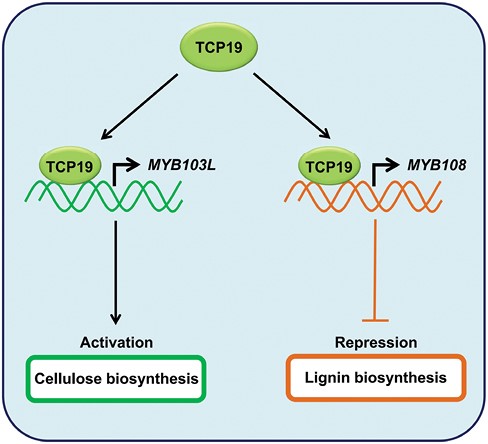
A proposed model of the function of OsTCP19 in rice lignin and cellulose biosynthesis. OsTCP19 activates cellulose biosynthesis and inhibits lignin biosynthesis by directly binding to the promoters of MYB103L and MYB108, respectively, and inducing their expression. MYB103L is essential for cellulose biosynthesis, while MYB108 is required for the repression of lignin biosynthesis. This TCP19-MYB108/MYB103L module regulates lignin and cellulose synthesis in rice, thereby influencing the structure of the secondary cell wall and the culm mechanical properties for lodging resistance.
Up-regulation of OsTCP19 in fibers enhances lodging resistance without affecting yield
High lodging resistance and grain yield are desired traits in breeding for rice production. Coordination of cellulose and lignin biosynthesis is required for proper mechanical strength, because mutants with defects in cellulose biosynthesis usually have brittle culms due to insufficient tensile strength, while mutants defective in lignin biosynthesis have pliable culms due to insufficient compressive strength (Y. Li et al., 2003; Li et al., 2009; Zhang et al., 2009, 2011, 2021; Wu et al., 2012, 2017; Song et al., 2013). We found that modification of OsTCP19 altered the amount and composition of cellulose and lignin, potentially improving the mechanical properties of the cell walls for lodging resistance. Interestingly, constitutive overexpression of OsTCP19 confers strong lodging resistance in rice even under high nitrogen conditions, which are usually detrimental to lodging resistance (Sun et al., 2018). However, constitutive overexpression of OsTCP19 also leads to short panicles and small grain size (Fig. 1A; Supplementary Fig. S2D), resulting in loss of yield. OsTCP19 might be involved in various aspects of plant development, including grain yield formation, and therefore we up-regulated it in a fiber-specific manner. The results showed that fiber-specific expression resulted in large panicles with normal plant height and tiller number (Fig. 6A, B; Supplementary Fig. S8) whilst also conferring greater lodging resistance by increasing cellulose and decreasing lignin (Fig. 6D–H). Although higher cellulose deposition in fibers confers stronger mechanical strength, it remains unclear how higher cellulose together with lower lignin affects the nanostructures and mechanical properties of the cell wall.
Cell wall remodeling in a cell type-specific manner has attracted a lot of attention. In Populus, fiber-specific lignin modification results in low lignin wood with no growth penalty (Cao et al., 2020; Gui et al., 2020). In Arabidopsis, fiber-specific expression of ERF035-VP16 and ERF041-VP16 increases biomass yield without affecting plant development (Nakata et al., 2021). Moreover, fiber-specific expression of ERF035 in the nst1 nst3 double-mutant results in a distinct cell wall structure, whereas constitutive expression of ERF035 causes semi-dwarf phenotypes (Sakamoto et al., 2018). In our study, we found that up-regulation of OsTCP19 in culm fibers improved lodging resistance without reducing grain yield, which could be exploited to improve agronomic traits in rice breeding.
Supplementary data
The following supplementary data are available at JXB online.
Fig. S1. Genomic PCR confirmation of TV and TE transgenic rice plants.
Fig. S2. Plant height, internode length, tiller number, and 1000-grain weight of the wild-type and transgenic TV and TE plants.
Fig. S3. Expression analysis of OsTCP19 and secondary cell wall biosynthesis genes in the wild-type and transgenic TV and TE plants by qRT-PCR using ACTIN1 as the reference gene.
Fig. S4. SDS-PAGE showing the purified recombinant protein of OsTCP19.
Fig. S5. Confirmation of the CRISPR/Cas9-edited MYB108 mutants by gene sequencing.
Fig. S6. Expression analysis of secondary cell wall biosynthesis genes in the wild type and MYB108-knockout mutants by qRT-PCR using ACTIN1 as the reference gene.
Fig. S7. Immunolocalization of OsTCP19 in the wild-type and PdDUF579-9P-35Smini-OsTCP19-3Flag (TOE) plants using anti-Flag antibodies.
Fig. S8. Tiller number, panicle length, spikelet number, and 1000-grain weight in the wild-type and PdDUF579-9P-35Smini-OsTCP19-3Flag (TOE) plants.
Table S1. List of primer sequences used in this study.
Dataset S1. Lists of differentially expressed genes detected in the TV and TE transgenic plants compared with the wild type.
Abbreviations
- cDNA
complementary DNA
- DEG
differentially expressed gene
- GO
gene ontology
- HTF
hybrid transcription factor
- KEGG
Kyoto Encyclopedia of Genes and Genomes
- qRT-PCR
quantitative reverse-transcription PCR
- SCW
secondary cell wall
- TCP
teosinte branched1/cycloidea/proliferating cell factor
- WT
wild type
Acknowledgements
We thank Sunil Kumar Sahu (BGI-Shenzhen) for critical reading of the manuscript, and Ziwei Xiang (CEMPS) for OsTCP19 cloning.
Author contributions
JG, LgL, and QX designed the research; JG, SL, ZL, JS, and LfL performed the research; JG, LgL, SL, and ZL analysed the data; JG, SL, and LgL wrote the manuscript.
Conflict of interest
The authors declare that they have no conflicts of interest in relation to this work.
Funding
This work was supported by the National Natural Science Foundation of China (31971617, 32022055), the Strategic Priority Research Program of the Chinese Academy of Sciences (XDB27020104), the Zhejiang A&F University Starting Funding (2021FR026), and the State Key Laboratory of Subtropical Silviculture (KF202002).
Data availability
The sequencing data from this study are openly available under reference no. CRA011499 in the Genome Sequencing Archive. https://ngdc.cncb.ac.cn/gsa.
References
Author notes
Siwei Lv, Zengshun Lin and Junhui Shen These authors contributed equally to this work.
Comments