-
PDF
- Split View
-
Views
-
Cite
Cite
David L. Parrott, Eric P. Downs, Andreas M. Fischer, Control of barley (Hordeum vulgare L.) development and senescence by the interaction between a chromosome six grain protein content locus, day length, and vernalization, Journal of Experimental Botany, Volume 63, Issue 3, February 2012, Pages 1329–1339, https://doi.org/10.1093/jxb/err360
- Share Icon Share
Abstract
Regulatory processes controlling traits such as anthesis timing and whole-plant senescence are of primary importance for reproductive success and for crop quality and yield. It has previously been demonstrated that the presence of alleles associated with high grain protein content (GPC) at a locus on barley chromosome six leads to accelerated leaf senescence, and to strong (>10-fold) up-regulation of several genes which may be involved in senescence control. One of these genes (coding for a glycine-rich RNA-binding protein termed HvGR-RBP1) exhibits a high degree of similarity to Arabidopsis glycine-rich RNA-binding protein 7 (AtGRP7), which has been demonstrated to accelerate flowering under both long-day (LD) and short-day (SD) conditions, but not after vernalization. Development of near-isogenic barley lines, differing in the allelic state of the GPC locus, was compared from the seedling stage to maturity under both SD and LD and after vernalization under LD. Intriguingly, pre-anthesis plant development [measured by leaf emergence timing and pre-anthesis (sequential) leaf senescence] was enhanced in high-GPC germplasm. Differences were more pronounced under SD than under LD, but were eliminated by vernalization, associating observed effects with floral induction pathways. By contrast, differences in post-anthesis flag leaf and whole-plant senescence between low- and high-GPC germplasm persisted under all tested conditions, indicating that the GPC locus, possibly through HvGR-RBP1, impacts on both developmental stages. Detailed molecular characterization of this experimental system may allow the dissection of cross-talk between signalling pathways controlling early plant and floral development on one side, and leaf/whole-plant senescence on the other side.
Introduction
From germination to death, at least three developmental phases can be distinguished in a plant's life, namely a juvenile vegetative, an adult vegetative, and a reproductive phase (Poethig, 2009, 2010; Kaufmann et al., 2010). In monocarpic plants, reproduction is associated with whole-plant senescence leading to death and processes occurring during this last developmental phase are of particular importance to a plant's reproductive success and to crop yield (Guo and Gan, 2005; Lim et al., 2007; Guiboileau et al., 2010). Vegetative phase change appears to be regulated independently of floral induction as maize mutations that prolong the vegetative phase do not have a (large) effect on anthesis timing (Poethig, 1988; Bassiri et al., 1992). It has recently become clear that miR156 and its targets control vegetative phase change in maize and Arabidopsis. This miRNA is very highly expressed during the juvenile phase and decreases during vegetative phase change. Furthermore, its manipulation can extend or eliminate the juvenile phase (Wang et al., 2009; Poethig, 2010).
Molecular mechanisms controlling floral induction have been intensely studied over the past decade, both in Arabidopsis (Amasino, 2010; Fornara et al., 2010) and in important crops including cereals (Colasanti and Coneva, 2009; Distelfeld et al., 2009; Greenup et al., 2009; Trevaskis, 2010). These efforts have led to the identification of circadian clock/photoperiod-dependent, vernalization-dependent, and ‘autonomous pathway’-dependent inputs into flowering control, and to the discovery of a series of genes involved in each pathway and in pathway integration [e.g. CONSTANS (CO), SUPPRESSOR OF OVEREXPRESSION OF CONSTANS 1 (SOC1), FLOWERING LOCUS T (FT); see Amasino, 2010; Fornara et al., 2010, for recent overviews]. Detailed comparisons between Arabidopsis and temperate cereals have identified both similarities and differences between these species. The most important differences between the two systems may be the absence of homologues of the floral repressor FLOWERING LOCUS C (FLC) in cereals, and the absence of VRN (VERNALIZATION)2 homologues in the Brassicaceae (Yan et al., 2004; Hecht et al., 2005; Lee et al., 2005).
Molecular mechanisms controlling leaf and whole-plant senescence of monocarpic plants are less-well understood than those governing floral induction (Breeze et al., 2011). This may be unsurprising, as the timing of this last developmental stage is influenced by the complex mechanisms governing all previous developmental phases. Of particular importance to the present study, both flowering time-dependent and -independent inputs into the control of leaf senescence have been identified (Hensel et al., 1993; Kim et al., 2004; Wu et al., 2008; Wingler et al., 2010). Data obtained by Wingler et al. (2010) in Arabidopsis, based on a quantitative trait locus (QTL) mapping approach, demonstrated that flowering and whole-rosette senescence were genetically linked by the vernalization pathway. Specifically, these traits were controlled by a major QTL on chromosome 4 (co-localizing with FRIGIDA) which epistatically interacted with a QTL on chromosome 5 (co-localizing with the floral repressor FLC). In wheat, Bogard et al. (2011) found that variability of leaf senescence during grain-filling was largely controlled by anthesis timing, as demonstrated by the co-location of stable QTLs for these traits on chromosomes 2D and 7D.
Over the last few years, the authors’ laboratory has characterized the control of barley senescence by a QTL on chromosome six, delineated by molecular markers HVM74 and ABG458 and originally discovered in a grain protein content (GPC) mapping population (See et al., 2002; Mickelson et al., 2003). Identification of the gene responsible for a homologous GPC QTL in tetraploid wheat by map-based cloning, and extension of the analysis to barley, has identified a NAC transcription factor (TtNAM-B1, DQ869673 in wheat and HvNAM-1, DQ869678 in barley; Uauy et al., 2006; Distelfeld et al., 2008). Analysis of TtNAM-B1 indicated that the presence of a functional gene copy (as compared to germplasm with loss-of-function mutations or gene deletion) leads to higher GPC and accelerated leaf and whole-plant senescence. Comparison of near-isogenic barley germplasm varying in the allelic state of the GPC locus and HvNAM-1 also indicated that accelerated senescence is causally related to high GPC, as based on extensive physiological and transcriptomic analyses (Heidlebaugh et al., 2008; Jukanti and Fischer, 2008; Jukanti et al., 2008). Furthermore, a detailed developmental study performed under long-day (LD, 16 h) conditions indicated that the barley GPC locus not only influences GPC and (post-anthesis) senescence, but modifies plant development well before anthesis. Leaf emergence of high-GPC germplasm was accelerated starting at ∼45 d after planting, while anthesis occurred at 73 d in high- and 78 d in low-GPC germplasm. In addition, sequential (pre-anthesis) leaf senescence was also accelerated in the investigated high-GPC line, compared with the near-isogenic low-GPC parental variety (Lacerenza et al., 2010), starting at ∼35 d after planting. It was hypothesized that floral transition at the shoot apical meristem (SAM) might occur earlier in high-GPC, early-senescence germplasm; however, this transition occurred simultaneously in both low- and high-GPC barley between 15 d and 21 d after planting. By contrast, inflorescence development, occurring after floral transition, was clearly accelerated in high-GPC germplasm (Lacerenza et al., 2010).
Transcriptomic comparison of flag leaves of near-isogenic early- and late-senescence barley lines at 14 d and 21 d post-anthesis identified numerous genes, which were strongly up-regulated in the early-senescence line and which could therefore be involved in senescence regulation (Jukanti et al., 2008). These included several leucine-rich repeat receptor protein kinase genes and a gene coding for a glycine-rich RNA-binding protein (termed HvGR-RBP1) with ∼65% sequence identity (at the amino acid level) with Arabidopsis glycine-rich RNA-binding protein 7 (AtGRP7). HvGR-RBP1 was also strongly up-regulated (by two to three orders of magnitude) in several leaves of pre-anthesis barley plants at 21, 35, and 42 d after planting (Lacerenza et al., 2010). Intriguingly, Streitner et al. (2008) demonstrated a role for AtGRP7 in anthesis timing. Arabidopsis germplasm without AtGRP7 functionality (T-DNA insertion and RNAi lines) flowered late under both short- (SD) and long-day (LD) conditions, but differences were larger under SD. Furthermore, these differences were abolished in vernalized plants. These and other data pointed to AtGRP7 as a new member of the autonomous floral induction pathway. Due to the obvious parallels in the development of barley germplasm with high versus very low HvGR-RBP1 expression and Arabidopsis with and without AtGRP7 functionality, these genes and their products may have similar functions. Furthermore, while no differences in senescence behaviour have so far been observed in Arabidopsis, the detailed developmental analysis of the described barley germplasm may allow the dissection of cross-talk between signalling pathways controlling early plant and floral development on one side, and leaf/whole-plant senescence on the other side. To approach that goal and extend the comparison of barley and Arabidopsis, the present study analyses the influence of day length and vernalization on development and senescence of near-isogenic low-GPC (with very low HvGR-RBP1 expression) and high-GPC barley germplasm.
Materials and methods
Plant material and growth conditions
Germplasm used in this study was derived from a grain protein content (GPC) mapping population (See et al., 2002; Mickelson et al., 2003) as described in detail by Jukanti et al. (2008). The original mapping population was based on a cross between varieties ‘Karl’ (characterized by stable, low GPC) and ‘Lewis’ (exhibiting higher GPC). Utilizing four backcrosses (followed by marker-assisted selection) and selfing, several near-isogenic lines including ‘10_11’ (BC4F3 and F4) were derived, which are near-isogenic to ‘Karl’, but contain high-GPC allele(s) from variety ‘Lewis’ at a quantitative trait locus on chromosome six, delineated by markers ABG458 and HVM74. Data published by Distelfeld et al. (2008) indicate that they also differ in the allelic state of HvNAM-1. Plants were cultivated essentially as described by Lacerenza et al. (2010), but with two additional [short-day (SD) and vernalization (vern)] treatments.
Plants were grown in potting soil in 1 gallon (3.8 l) pots (three plants per pot) under controlled conditions in growth chambers. For line ‘10_11’, BC4F3 was used for LD experiments, and BC4F4 for SD and vern treatments. SD-grown plants (10/14 h light/dark) were kept under 25/20 °C day/night cycles and light intensities of ∼200 μE m−2 s−1 at leaf level. Plants were fertilized with 250 ml of Peter's Professional General Purpose Fertilizer (4 g l−1; Scotts-Sierra Horticultural Products Company, Marysville, OH, USA) per 1 gallon pot at 33, 54, and 75 d after planting. Long-day (LD) grown plants (16/8 h light/dark) were grown using the same day/night temperatures and light intensities and fertilized at 14, 28, and 42 d after planting (due to overall faster development than SD plants). For vernalization, plants were first grown in small soil plugs (2–3 plants per plug) for 10 d under standard LD conditions. Plants were then transferred to a vernalization room (10/14 h light/dark; 4/2 °C; light intensity ∼30 μE m−2 s−1 at leaf level) for 7 weeks, developing 3–4 leaves by the end of the vernalization treatment. They were then re-grown under standard LD conditions for a few days, transferred (three plants per pot) into 1 gallon pots and grown to maturity under standard LD conditions, with fertilizer treatments at 9, 23, and 37 d after removal from the vernalization chamber.
Field experiments were performed at the Arthur Post Research Farm near Bozeman, Montana, USA (45°41′ N, 111°00′ W, 1455 m above sea level) during spring/summer 2007, using BC4F3 plants for line ‘10_11’. Plants were cultivated in small 4-row plots (c. 3×1.3 m) using standard agricultural practice, starting on 18 May (three separate plots per genotype). If necessary, natural precipitation was complemented by irrigation to prevent (severe) drought stress. Temperature data for the relevant period (May–June 2007) are shown as Supplementary Fig. S1 at JXB online and were obtained from an on-site automatic recording station.
Analysis of plant development
For all growth chamber experiments, leaves along the first (primary) shoot of growing plants were tagged with the date on which full leaf expansion occurred, from leaf 1 (the first, oldest leaf) to flag leaves. Numbers of developed leaves differed between treatments (see Results). Full leaf expansion was defined as the day when auricles were developed. At the end of each experiment, tags were collected from mature plants, and leaf development was plotted in days after planting (SD and LD treatments), and in days after transfer from the vernalization room (vern).
Anthesis dates were defined as the day when awns first appeared above the top (flag) leaf lamina, for both primary shoots and tillers of each plant. For field experiments, anthesis was determined as the day on which 50% of the shoots in a research plot reached the described stage.
Chlorophyll assays
Relative chlorophyll content in consecutive main shoot leaves was determined non-destructively using a Minolta SPAD-502 (Konica Minolta Sensing, Osaka, Japan) chlorophyll meter. As described by Lacerenza et al. (2010), three separate measurements were taken from an area ∼3 cm in length in the middle of the leaf blade, averaged, and noted for each data point.
Statistical analysis
For each growth chamber experiment, 36 (12 pots, each containing three plants) ‘Karl’ and 36 ‘10_11’ plants were grown and analysed as described. Data from three experiments were averaged for LD treatments (see Lacerenza et al., 2010), while one experiment each was used for SD and vern treatments. To compare ‘Karl’ and ‘10_11’ development, means and standard deviations were calculated using the corresponding functions in Microsoft Excel for Windows (Microsoft Corporation, Redmond, WA, USA). Student's t tests (two-sided; two independent samples assuming equal variance) were performed with the same software package. Obtained P values are indicated in all tables and figures.
Results
Plant development
To compare barley germplasm with very low (‘Karl’) versus high HvGR-RBP1 expression (line ‘10_11’; see Introduction), several developmental parameters were followed. Table 1 indicates that different environments (day length, vernalization) had a considerable influence on the number of leaves developed by each main shoot. In contrast to Arabidopsis with and without AtGRP7 functionality (Streitner et al., 2008), leaf numbers between ‘Karl’ and ‘10_11’ were not different under SD and LD, while a small difference was observed in vernalized plants.
Number of leaves on main stems of plants grown under short-day (10 h), long-day (16 h), and vernalized/long-day conditions
Short days | Long days | Vernalized/long days | |
Karl | 14.89±1.45 | 12.27±1.01 | 10.67±1.19 |
10_11 | 15.17±1.65 | 11.97±0.89 | 9.85±0.82 |
P-value | 0.60 | 0.22 | 0.0092 |
Short days | Long days | Vernalized/long days | |
Karl | 14.89±1.45 | 12.27±1.01 | 10.67±1.19 |
10_11 | 15.17±1.65 | 11.97±0.89 | 9.85±0.82 |
P-value | 0.60 | 0.22 | 0.0092 |
Number of leaves on main stems of plants grown under short-day (10 h), long-day (16 h), and vernalized/long-day conditions
Short days | Long days | Vernalized/long days | |
Karl | 14.89±1.45 | 12.27±1.01 | 10.67±1.19 |
10_11 | 15.17±1.65 | 11.97±0.89 | 9.85±0.82 |
P-value | 0.60 | 0.22 | 0.0092 |
Short days | Long days | Vernalized/long days | |
Karl | 14.89±1.45 | 12.27±1.01 | 10.67±1.19 |
10_11 | 15.17±1.65 | 11.97±0.89 | 9.85±0.82 |
P-value | 0.60 | 0.22 | 0.0092 |
Unlike leaf numbers, differences in the timing of leaf emergence between ‘Karl’ and ‘10_11’ were easily observed under SD and LD, with leaves along the main stems of ‘10_11’ plants consistently developing earlier (Fig. 1). The trend towards significant differences in lower leaves was more pronounced under SD than under LD, and differences in emergence dates of higher leaves (from leaf 10 on) were considerably larger under SD. By contrast, vernalization completely eliminated these differences (Fig. 1C).
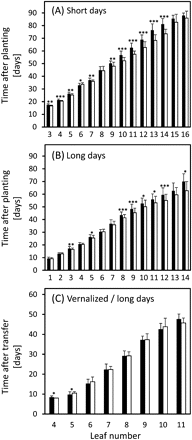
Leaf emergence under short-day (A, 10 h), long-day (B, 16 h), and vernalized/long-day conditions (C). Time points of full leaf emergence (see Materials and methods) are shown for ‘Karl’ (filled columns) and ‘10_11’ (open columns) in days after planting (A, B) and in days after transfer from the vernalization chamber (C). Means and standard deviations are shown. Student's t tests were performed to determine significant differences between ‘Karl’ and ‘10_11’. *P <0.05; **P <0.01; ***P <0.001. Data in (B) are from Lacerenza et al. (2010) modified.
Significant differences of between 3 d and 5 d were found for both main shoot and tiller anthesis under SD and LD, with ‘10_11’ always flowering earlier (Table 2). By contrast, no significant difference was detected for main shoot anthesis of vernalized plants, while tillers of ‘Karl’ actually reached anthesis faster than those of ‘10_11’ in this treatment. In the field experiment, anthesis occurred at 185.7±0.5 (Julian) days for ‘Karl’, and at 185.3±0.5 d for near-isogenic high-GPC lines (P=0.094). As plants were grown starting in mid-May, they experienced (mild) vernalization during the seedling stage (see Supplementary Fig. S1 at JXB online); these data therefore also suggest that vernalization cancels differences in anthesis timing. Overall, our developmental data indicate that vernalization has a major impact on barley development as controlled by the chromosome six GPC locus.
Anthesis dates of plants grown under short-day (10 h), long-day (16 h), and vernalized/long-day conditions
Data for long-day-grown plants are from Lacerenza et al. (2010), modified.
Short days (days after planting) | Long days (days after planting) | Vernalized/long days (days after transfer) | |
Main stems | |||
Karl | 94.1±4.6 | 77.8±6.7 | 53.0±6.1 |
10_11 | 89.2±4.4 | 72.7±4.8 | 50.6±4.1 |
P-value | 0.0086 | <0.001 | 0.24 |
Tillers | |||
Karl | 97.7±4.7 | 81.6±5.8 | 63.6±7.1 |
10_11 | 94.7±6.0 | 77.7±4.8 | 70.2±5.5 |
P-value | 0.0047 | <0.001 | <0.001 |
Short days (days after planting) | Long days (days after planting) | Vernalized/long days (days after transfer) | |
Main stems | |||
Karl | 94.1±4.6 | 77.8±6.7 | 53.0±6.1 |
10_11 | 89.2±4.4 | 72.7±4.8 | 50.6±4.1 |
P-value | 0.0086 | <0.001 | 0.24 |
Tillers | |||
Karl | 97.7±4.7 | 81.6±5.8 | 63.6±7.1 |
10_11 | 94.7±6.0 | 77.7±4.8 | 70.2±5.5 |
P-value | 0.0047 | <0.001 | <0.001 |
Anthesis dates of plants grown under short-day (10 h), long-day (16 h), and vernalized/long-day conditions
Data for long-day-grown plants are from Lacerenza et al. (2010), modified.
Short days (days after planting) | Long days (days after planting) | Vernalized/long days (days after transfer) | |
Main stems | |||
Karl | 94.1±4.6 | 77.8±6.7 | 53.0±6.1 |
10_11 | 89.2±4.4 | 72.7±4.8 | 50.6±4.1 |
P-value | 0.0086 | <0.001 | 0.24 |
Tillers | |||
Karl | 97.7±4.7 | 81.6±5.8 | 63.6±7.1 |
10_11 | 94.7±6.0 | 77.7±4.8 | 70.2±5.5 |
P-value | 0.0047 | <0.001 | <0.001 |
Short days (days after planting) | Long days (days after planting) | Vernalized/long days (days after transfer) | |
Main stems | |||
Karl | 94.1±4.6 | 77.8±6.7 | 53.0±6.1 |
10_11 | 89.2±4.4 | 72.7±4.8 | 50.6±4.1 |
P-value | 0.0086 | <0.001 | 0.24 |
Tillers | |||
Karl | 97.7±4.7 | 81.6±5.8 | 63.6±7.1 |
10_11 | 94.7±6.0 | 77.7±4.8 | 70.2±5.5 |
P-value | 0.0047 | <0.001 | <0.001 |
Leaf- and whole-plant senescence
Previous results obtained with the germplasm used here indicated that chlorophyll levels are a reliable parameter to quantify the progress of leaf senescence (Heidlebaugh et al., 2008; Jukanti and Fischer, 2008; Jukanti et al., 2008; Lacerenza et al., 2010). The results presented here demonstrate that vernalization and day length treatments not only influence developmental differences between ‘Karl’ and ‘10_11’, but have an even larger impact on senescence. Leaves of line ‘10_11’ consistently senesced faster than ‘Karl’ leaves under SD, starting with leaf 3 around 35 d after planting (Fig. 2B). Differences became larger for higher leaf numbers, and with increasing plant age. Senescence of individual ‘Karl’ leaves lagged ∼14 d behind ‘10_11’ when plants reached anthesis (see Fig. 2K, L, leaves 12 and 13, around 90 d after planting). As shown previously (Lacerenza et al., 2010), clear differences in pre-anthesis (sequential) leaf senescence were also observed for LD-treated plants, but started later (with leaf 4) and were smaller than under SD (Fig. 3). Senescence of individual ‘Karl’ leaves at anthesis lagged at most by 7 days (see Fig. 3K, leaf 11, around 75 d). By contrast, and in agreement with developmental data, vernalization completely eliminated differences in leaf senescence between ‘Karl’ and ‘10_11’ in lower leaves and differences remained small when plants reached anthesis around 50–53 d after transfer from the vernalization room (Fig. 4).
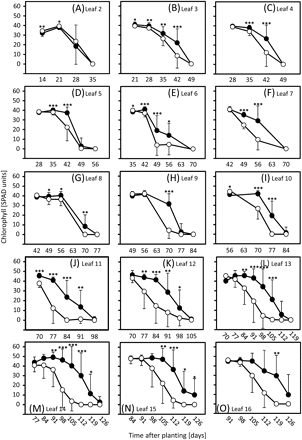
Chlorophyll levels in leaves of short-day-grown plants. Chlorophylls were compared in leaves 2 (A, oldest measured leaf) to 16 (O) of ‘Karl’ (filled circles) and ‘10_11’ (open circles) as outlined in the Materials and methods. Means and standard deviations (on one side only for clarity) are shown. Student's t tests were performed to determine significant differences between ‘Karl’ and ‘10_11’. *P <0.05; **P <0.01; ***P <0.001.
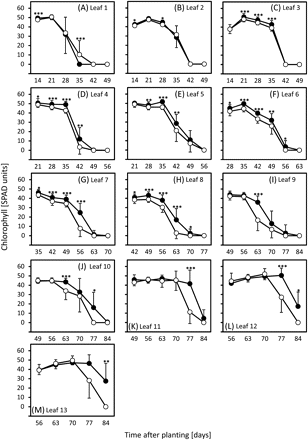
Chlorophyll levels in leaves of long-day-grown plants. Chlorophylls were compared in leaves 1 (A, oldest leaf) to 13 (M) of ‘Karl’ (filled circles) and ‘10_11’ (open circles) as outlined in the Materials and methods. Means and standard deviations (on one side only for clarity) are shown. Student's t tests were performed to determine significant differences between ‘Karl’ and ‘10_11’. *P <0.05; **P <0.01; ***P <0.001. Data are from Lacerenza et al. (2010) modified.
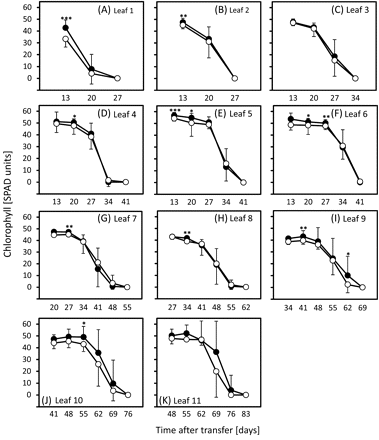
Chlorophyll levels in leaves of vernalized/long-day-grown plants. Chlorophylls were compared in leaves 1 (A, oldest leaf) to 11 (K) of ‘Karl’ (filled circles) and ‘10_11’ (open circles) as outlined in the Materials and methods. Means and standard deviations (on one side only for clarity) are shown. Student's t tests were performed to determine significant differences between ‘Karl’ and ‘10_11’. *P <0.05; **P <0.01; ***P <0.001.
Visual differences between ‘Karl’ and ‘10_11’ have been documented for LD-grown plants post-anthesis (e.g.,Jukanti and Fischer, 2008; Jukanti et al., 2008, see Supplementary data). Visual differences in SD-grown plants were larger, especially at earlier time points, and are documented here at 46 d after planting (Fig. 5A).
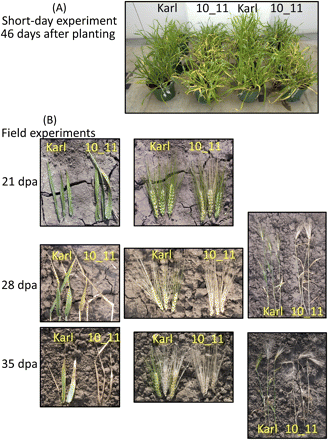
Visual developmental differences between ‘Karl’ and ‘10_11’. (A) Short-day-grown plants are compared at 46 d after planting. (B) Field-grown plants are compared at 21 d post-anthesis (dpa; flag leaves and ears), 28 and 35 dpa (flag leaves, ears, and entire shoots).
As may be expected, the number of leaves developed per shoot was subject to some variation within each treatment (Table 1). Therefore, our data were also analysed by comparing flag leaf (topmost leaf, directly below the ear) senescence for all main shoots (Fig. 6). This analysis also allowed a comparison of LD treatments with previous experiments focusing on flag leaves (Heidlebaugh et al., 2008; Jukanti and Fischer, 2008; Jukanti et al., 2008). Interestingly, this analysis indicated that post-anthesis flag leaf senescence was significantly accelerated in ‘10_11’ for all treatments, including vernalized plants (Fig. 6C). Visual evaluation of field-grown material which experienced (mild) vernalization also indicated clear differences in post-anthesis leaf- and whole-plant senescence (Fig. 5B).
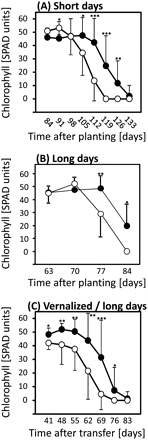
Chlorophyll levels in flag leaves of short-day (A,; 10 h), long-day (B; 16 h), and vernalized/long-day grown plants (C) of ‘Karl’ (filled circles) and ‘10_11’ (open circles). Means and standard deviations (on one side only for clarity) are shown. Student's t tests were performed to determine significant differences between ‘Karl’ and ‘10_11’. *P <0.05; **P <0.01; ***P <0.001. Data in (B) are from Lacerenza et al. (2010) modified.
Discussion
Control and timing of life history traits such as vegetative phase change, floral induction, and whole-plant senescence are of primary importance for plant reproductive success and for crop yield and quality (Laurie et al., 2004; Lim et al., 2007; Zhang et al., 2008). The past decade has seen much progress in our understanding of the molecular mechanisms controlling these traits. The largest advance has been achieved in the area of flowering time control, while our understanding of leaf and whole-plant senescence is still fragmentary (Lim et al., 2007; Breeze et al., 2011). Unsurprisingly, available molecular data indicate connections and cross-talk between signalling pathways controlling different life history traits, and unravelling such connections will substantially enhance our knowledge of plant developmental biology.
From previous work performed in the authors’ laboratory, it became clear that a GPC locus on barley chromosome six which is defined by allelic variation in a NAC transcription factor (HvNAM-1; see Introduction) not only influences post-anthesis senescence and grain protein accumulation (Jukanti et al., 2008), but has additional effects on pre-anthesis plant development and anthesis timing (Lacerenza et al., 2010). Furthermore, the allelic state of that locus controls expression (among other genes) of HvGR-RBP1, coding for a glycine-rich RNA-binding protein with high sequence homology to Arabidopsis AtGRP7 (Jukanti et al., 2008). In our studies, high GPC was always associated with early senescence, and with higher (by one to three orders of magnitude, depending on plant and leaf developmental stage) HvGR-RBP1 transcript levels, both pre- and post-anthesis. In Arabidopsis, the presence of functional AtGRP7 was associated with earlier flowering (compared with T-DNA insertion and RNAi lines), but vernalization abolished these differences (Streitner et al., 2008). It wase therefore hypothesized that our barley germplasm might allow the dissection of cross-talk between signalling pathways controlling the rate of pre-anthesis development and flowering time on one side, and leaf/whole-plant senescence on the other side.
Temperate cereals, including barley, generally flower faster under LD- than under SD-conditions (Greenup et al., 2009), and data shown in Table 2 confirm this for germplasm used in the present study. Plant development under LD was moderately influenced by the allelic state of the GPC locus, as described by Lacerenza et al. (2010) and shown in Figs 1 and 3 and Table 2. Faster development in the high-GPC line may necessitate slightly faster nutrient recycling from lower (older) to younger leaves; this could explain the small differences observed in sequential (pre-anthesis) leaf senescence. Under SD conditions, overall plant development was delayed, as indicated by the production of about three additional leaves (Table 1) and later flowering (Table 2). Under these conditions, the influence of the GPC locus on leaf emergence (Fig. 1) and especially on pre-anthesis (sequential) leaf senescence (Fig. 2) was more pronounced, but differences in anthesis timing per se were not enhanced compared with LD (Table 2). AtGRP7 appears to act through the ‘autonomous’ (i.e. not dependent on environmental cues) pathway, and the GPC locus, possibly through its control of HvGR-RBP1 expression, may do the same in barley (Fig. 7). In that situation, its influence could be more noticeable during the extended pre-anthesis development observed under SD, leading to larger differences in leaf emergence and (sequential) leaf senescence. A detailed analysis of the vrn1, 2, and 3 as well as ppd1 genotypes in our germplasm will allow refined hypotheses about GPC locus, vernalization, and day length interactions in the control of barley development and senescence. This type of approach may also indicate if day length influences the system primarily through signalling involving ppd1, or (also) through differences in photoassimilate production and availability.
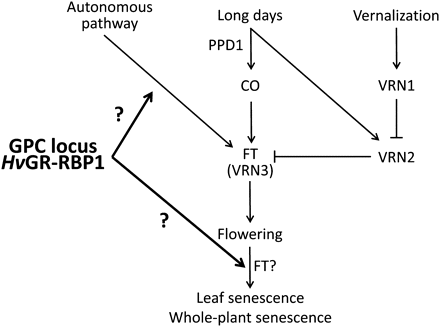
Interaction of anthesis timing and senescence control pathways. The barley GPC locus, possibly through its control of HvGR-RBP1 expression, influences two different plant developmental stages. In this model, vernalization bypasses GPC locus influence on flowering time control, but does not influence GPC locus control over post-anthesis development. FT gene(s) may also be involved in this last developmental stage, in addition to their well-established role in flowering time control. The influence of additional factors (promotion of flowering by warmth once vernalization is achieved; gibberellins, plant age (Fornara et al., 2010) is not shown.
While germplasm used in the present study is best characterized as spring barley (it will flower and complete its development without vernalization, as shown by our LD experiments), Saisho et al. (2011) have demonstrated considerable quantitative variation in barley vernalization requirements, suggesting that development of some spring barleys is accelerated by exposure to low non-freezing temperatures. Based on a small difference in the number of leaves developed between LD and vern/LD treatments (Table 1), this is the case for germplasm used here. Vernalization represents an alternative input into the flowering control system, and may influence the expression of ‘integrator’ genes (such as FT/VRN3) independently of photoperiod and autonomous pathway-controlled signals (Fig. 7; Greenup et al., 2009). If this interpretation is correct, vernalization-derived signals bypass pre-anthesis control of plant development, as exerted by the GPC locus (and possibly HvGR-RBP1). The same effect was observed in Arabidopsis, where vernalization eliminated differences in flowering time between germplasm with and without AtGRP7 functionality (Streitner et al., 2008).
Possibly the most interesting finding from this study is the fact that, while vernalization abolishes differences in sequential (pre-anthesis) senescence and main shoot anthesis timing, differences in leaf and whole-plant senescence observed post-anthesis persist (Figs 5B, 6C). The GPC locus, therefore, exerts control on two distinct developmental phases. It appears likely that a detailed molecular comparison of all 12 experimental conditions utilized in our experiments [2 genotypes×2 developmental stages (pre- and post-anthesis)×3 environmental treatments] will lead to new insights into cross-talk between regulatory pathways controlling anthesis timing on one side, and whole-plant senescence on the other side. Interestingly, data obtained by Jukanti et al. (2008, see Supplementary data) have already indicated post-anthesis differences in the expression of genes commonly associated with flowering control including CO and FT, providing a preview of what an extended data set might deliver.
Unlike senescence of leaves in other monocarpic species, senescence of individual Arabidopsis leaves is not subject to ‘correlative control’ by developing sinks (Noodén and Penney, 2001). Visual evaluation of Arabidopsis plants with different levels of AtGRP7 expression has not, so far, indicated differences in leaf senescence patterns (A Fischer and D Staiger, unpublished observations). This observation, therefore, strengthens the idea that differences in senescence rate caused by the allelic state of the barley GPC locus are associated with nutrient recycling to developing sinks, such as younger leaves (pre-anthesis) and seeds (post-anthesis). Furthermore, molecular analysis of barley and Arabidopsis with different levels of GRP expression may allow the dissection of important differences in senescence regulation between the two systems. It must be borne in mind, however, that different (from the one investigated here) signalling pathways involved in Arabidopsis flowering time control can influence leaf senescence (see work discussed in the Introduction).
In summary, data presented in this and previous studies analysing the barley GPC locus point to important mechanisms in barley flowering time and senescence control, and suggest a novel approach to dissecting molecular cross-talk between the regulatory pathways controlling these important life history traits.
Abbreviations
- AtGRP7
Arabidopsis thaliana glycine-rich RNA-binding protein 7
- GPC
grain protein content
- GRP
glycine-rich RNA-binding protein
- HvGR-RBP1
Hordeum vulgare glycine-rich RNA-binding protein 1
- LD
long days (16 h)
- QTL
quantitative trait locus
- SAG
senescence-associated gene
- SAM
shoot apical meristem
- SD
short days (10 h)
- vern
vernalization
The authors would like to thank Dr T Blake and Mr S Bates for help with the field experiments. This work was supported by grants from the US Barley Genome Project, from the Montana Board of Research and Commercialization Technology and from the National Science Foundation to AMF. Additional support from the Montana Agricultural Experiment Station is also gratefully acknowledged.
Comments