-
PDF
- Split View
-
Views
-
Cite
Cite
Emily B. Merewitz, Hongmei Du, Wenjuan Yu, Yimin Liu, Thomas Gianfagna, Bingru Huang, Elevated cytokinin content in ipt transgenic creeping bentgrass promotes drought tolerance through regulating metabolite accumulation, Journal of Experimental Botany, Volume 63, Issue 3, February 2012, Pages 1315–1328, https://doi.org/10.1093/jxb/err372
- Share Icon Share
Abstract
Increased endogenous plant cytokinin (CK) content through transformation with an adenine isopentyl transferase (ipt) gene has been associated with improved plant drought tolerance. The objective of this study is to determine metabolic changes associated with elevated CK production in ipt transgenic creeping bentgrass (Agrostis stolonifera L.) with improved drought tolerance. Null transformants (NTs) and plants transformed with ipt controlled by a stress- or senescence-activated promoter (SAG12-ipt) were exposed to well-watered conditions or drought stress by withholding irrigation in an environmental growth chamber. Physiological analysis confirmed that the SAG12-ipt line (S41) had improved drought tolerance compared with the NT plants. Specific metabolite changes over the course of drought stress and differential accumulation of metabolites in SAG12-ipt plants compared with NT plants at the same level of leaf relative water content (47% RWC) were identified using gas chromatography–mass spectroscopy. The metabolite profiling analysis detected 45 metabolites differentially accumulated in response to ipt expression or drought stress, which included amino acids, carbohydrates, organic acids, and organic alcohols. The enhanced drought tolerance of SAG12-ipt plants was associated with the maintenance of accumulation of several metabolites, particularly amino acids (proline, γ-aminobutyric acid, alanine, and glycine) carbohydrates (sucrose, fructose, maltose, and ribose), and organic acids that are mainly involved in the citric acid cycle. The accumulation of these metabolites could contribute to improved drought tolerance due to their roles in the stress response pathways such as stress signalling, osmotic adjustment, and respiration for energy production.
Introduction
Drought stress causes a cascade of physiological responses within plant cells, including changes in hormone metabolism (Davies et al., 2010). The synthesis of cytokinin (CK), a growth-promoting hormone regulating cell division and various other growth and development events, typically declines in response to drought stress (Yang et al., 2002; Kudoyarova et al., 2006). A decline in CK production has been associated with leaf senescence and the growth inhibition of shoots induced by abiotic stresses, including drought stress. Molecular techniques such as transformation with a gene encoding isopentenyl transferase (ipt) to increase endogenous CK content under stress conditions have been found to be effective means to improve stress tolerance in various plant species, including monocot species such as rice (Oryza sativa) (Peleg et al., 2011), tall fescue (Festuca arundinacea) (Hu et al., 2005), and creeping bentgrass (Agrostis stolonifera) (Xu et al., 2010; Merewitz et al., 2010, 2011a, b). In SAG12-ipt creeping bentgrass (transformed with ipt controlled by a stress- or senescence-activated promoter), ipt expression resulted in increases in CK content, suppression of leaf senescence, and promotion of photosynthetic attributes, antioxidant scavenging, osmotic adjustment, and changes in root growth, which contributed to improved drought tolerance (Merewitz et al., 2010, 2011a). These physiological changes characteristic of enhanced drought tolerance in the ipt plants were related to changes in the abundance and activities of various proteins in ipt plants relative to the null transformant (NT) plants during drought stress, particularly those proteins involved in photosynthesis, energy metabolism, and antioxidant metabolism (Merewitz et al., 2011b). Rivero et al. (2007, 2009) reported that improved drought tolerance in ipt transgenic tobacco (Nicotiana tabacum) was associated with increased photorespiration and antioxidant processes. Elevated CK content in ipt transgenic plants may induce various metabolic changes which could contribute to the improved drought tolerance. However, specific metabolites regulated by CK and involved in drought tolerance have not yet been fully evaluated.
Metabolomic analysis of various plant species has been previously used as a way to elucidate plant responses to various abiotic stresses (Dixon et al., 2006). Knowledge of changes in the accumulation of specific metabolites aids in the understanding of metabolic pathways regulating stress tolerance responses, and could be used in future downstream applications such as gene identification and metabolomics-assisted plant breeding (Fernie and Schauer, 2008; Shulaev et al., 2008). A combination of metabolic profiling, proteomic profiling, and physiological analysis is a powerful approach to increase the understanding of metabolic pathways controlling drought tolerance in various species (Bundy et al., 2009). Previous studies have identified physiological processes and proteins regulated by CK under drought stress in SAG12-ipt creeping bentgrass (Merewitz et al., 2010, 2011a, b). It is feasible to anticipate changes in the accumulation of metabolites resulting from ipt expression, which may contribute to CK-regulated drought tolerance. Therefore, the objectives of the study were to evaluate metabolite changes differentially exhibited between ipt and NT transgenic creeping bentgrass under well-watered and drought stress conditions in order to identify potential drought tolerance mechanisms related to maintenance of CK content under drought stress in a perennial grass species. Ultimately, the metabolite changes revealed in this study can be used in conjunction with previous studies on the effects of drought stress on physiological processes and proteomic profiles for the identification of metabolic pathways of CK-regulated drought tolerance.
Materials and methods
Plant material and growth conditions
Transgenic creeping bentgrass plants were produced using the Agrobacterium transformation method as described in Merewitz et al. (2010). Plant materials used for metabolite analysis by gas chromatography–mass spectrometry (GC-MS) were generated from the same experiment as for the proteomic analysis described in Merewitz et al. (2011b). Briefly, a null transformed line of ‘Penncross’ (NT) and the SAG12-ipt transgenic line (S41) were exposed to drought stress in an environmentally controlled growth chamber (Conviron, Winnipeg, Canada). Growth chamber conditions were set to maintain 20/15 °C (day/night) temperatures, 12 h photoperiod, 60% relative humidity, and 500 μmol m−2 s−1 photosynthetic photon flux density at canopy height. Plants were grown in PVC tubes (40 cm in height×10.16 cm in diameter) in a 1:1 fine sand:soil mix (fine-loamy, mixed mesic Typic Hapludult type soil).
Treatments and physiological measurements
Water treatments consisted of well-watered (control) or water completely withheld (drought) imposed on both NT and S41 plant lines (40 plants of each). Soil volumetric water content (SWC) was determined with the time domain reflectometry (TDR) method (Topp et al., 1980) using a Trase TDR instrument (Soil Moisture Equipment Corp., Santa Barbara, CA, USA). Field capacity using this TDR system and a 1:1 soil was determined to be ∼25%. Measurements were taken from 12 probes, four within the watered treatment and eight within the drought stress treatment (four replicates in each line), that were 20 cm long, three-pronged waveguide probes buried in the pot.
Leaf samples for metabolite analysis were taken from eight plants, four of each line, sampled at an SWC of 25, 10, and 5% in pots of both plant lines, which occurred over a period of 14 d. The treatment with 25, 10, and 5% SWC was designated as control, moderate drought stress (MD), and severe drought stress (SD), respectively. Leaf samples with a relative water content (RWC) of 47% were taken at 10% SWC for NT plants and 5% SWC for SAG12-ipt plants. Leaf RWC was evaluated to determine leaf hydration status for comparison of leaf metabolite changes at a given level of leaf RWC by the method of Barrs and Weatherley (1962). Leaf RWC was calculated based on fresh (FW), turgid (TW), and dry weights (DW) of ∼0.1 g leaf samples. Leaf FW was determined on a mass balance immediately after being excised from the plants. Turgid weights were determined after soaking the leaves in de-ionized water for 12 h in a closed Petri dish and weighed immediately after they were blotted dry. Leaves were then dried in an 80 °C oven for at least 72 h prior to being weighed for DW. RWC was calculated using the formula: (FW–DW)/(TW–DW)×100. Other physiological parameters were measured to evaluate drought tolerance characteristics and were presented previously in Merewitz et al. (2010, 2011a, b).
Extraction of polar metabolites
Plant leaf tissue samples from NT and SAG12-ipt plants with a leaf RWC of ∼47% were used for metabolic profiling. For extraction of metabolites, plant tissue was ground with a mortar and pestle in liquid N and frozen immediately at –80 °C until further analysis. The extraction protocol was modified from Roessner et al. (2000) and Rizhsky et al. (2004). For each sample, frozen leaves were ground to a fine powder with liquid nitrogen, and 25 mg of leaf tissue powders were transferred into 10 ml microcentrifuge tubes and extracted in 1.4 ml of 80% (v/v) aqueous methanol for 2 h at 200 rpm at ambient temperature. Ribitol solution (8 μl, 2 mg ml−1) was added to each sample as an internal standard prior to incubation. Then, the tubes were placed in a water bath at 70 °C for 15 min, centrifuged for 30 min at 12 000 rpm, the supernatant was decanted to new culture tubes, and 1.4 ml of water and 0.75 ml of chloroform were added. The mixture was vortexed thoroughly and centrifuged for 5 min at 5000 rpm. A 2 ml aliquot of the polar phase (methanol/water) was decanted into 1.5 ml HPLC vials and dried in a Centrivap benchtop centrifugal concentrator. The dried polar phase was methoximated with 80 μl of 20 mg ml−1 methoxyamine hydrochloride at 30 °C for 90 min and was trimethylsilylated with 80 μl of MSTFA (with 1% TMCS) for 60 min at 70 °C. Ribitol (99%), methoxyamine hydrochloride, and N,O-bis(trimethylsilyl) trifluoroacetamide (BSTFA) with 1% trimethylchlorosilane (TMCS) were obtained from Sigma (St Louis, MO, USA). All other chemicals were analytical grade from the China National Pharmaceutical Group Corporation (Shanghai, China).
Gas chromatography–mass spectrometry
The procedure for GC-MS analysis was modified from Qiu et al. (2007). The derivatized extracts were analysed with a PerkinElmer gas chromatograph coupled with a TurboMass-Autosystem XL mass spectrometer (PerkinElmer Inc., USA). A 1 μl aliquot of the extracts was injected into a DB-5MS capillary column (30 m×0.25 mm×0.25 μm, Agilent J&W Scientific, Folsom, CA, USA). The inlet temperature was set at 260 °C. After a 6.5 min solvent delay, the initial GC oven temperature was set at 60 °C; 1 min after injection, the GC oven temperature was raised to 280 °C at 5 °C min−1, and finally held at 280 °C for 15 min. The injection temperature was set to 280 °C and the ion source temperature was adjusted to 200 °C. Helium was used as the carrier gas with a constant flow rate set at 1 ml min−1. The measurements were made with electron impact ionization (70 eV) in the full scan mode (m/z 30–550). The metabolites detected were identified by Turbomass 4.1.1 software (PerkinElmer Inc., USA) coupled with commercially available compound libraries: NIST 2005, Wiley 7.0. For GC-MS results, compounds were identified based on retention time and comparison with reference spectra in mass spectral libraries. Response ratios were peak areas compared with the internal standard ribitol, and the peak areas were integrated with the Genesis algorithm. Relative content utilized throughout the manuscript refers to the ratio of peak area of the compouds to the total area of all other peaks within a given treatment.
Experimental design and statistical analysis
Data were treated as appropriate for a split-plot design, with irrigation treatment as the main plots and plant materials as the subplots, with four replicates for each irrigation treatment and plant material. Effects of watering treatment, plant materials, and corresponding interactions were determined by analysis of variance according to the general linear model procedure of SAS using Fisher’s protected LSD test at the 0.05 probability level (version 9.0; SAS Institute, Cary, NC, USA).
Results
The effect of decreasing SWC on leaf RWC and drought damage to NT and SAG12-ipt creeping bentgrass plants has been reported previously (Merewitz et al., 2011b). Briefly, decreasing SWC caused a decrease in leaf RWC to SD levels (47%) earlier in NT plants than in SAG12-ipt plants (10% SWC for NT and 5% SWC for SAG12-ipt). It was found that the maintenance of cellular membrane stability, increases in antioxidant capacity, and other physiological changes could be related to the better maintenance of RWC under drought stress by SAG12-ipt plants. Here, the results of metabolic analysis in both plant types are reported by comparing the content of a metabolite or group of metabolites over the course of drought stress (Figs 1– 6) and at an equivalent level of leaf water deficit (47% RWC; Fig. 7).
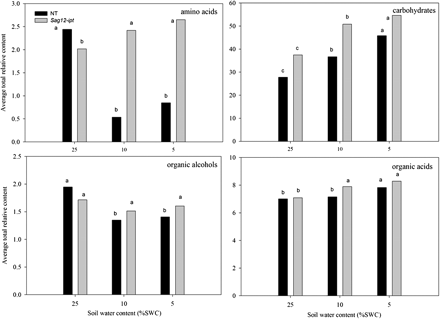
Total relative content of the major metabolite groups based on comparison with ribitol as an internal standard and total area of peaks within a given treatment for null transformant (NT) and SAG12-ipt transgenic creeping bentgrass leaves in response to drought stress. LSD bars and different letters indicate significant differences at each level of soil water content (% SWC) within a plant type (P ≤ 0.05).
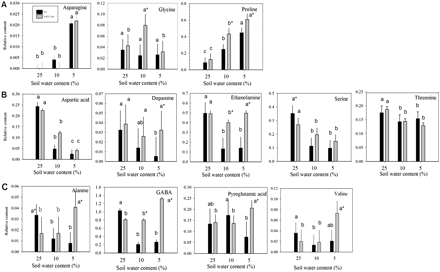
Relative amino acid and amino acid derivative content based on comparison with ribitol as an internal standard and total area of peaks within a given treatment generally (A) increased (B), reduced, or (C) oppositely accumulated in leaves of null transformant (NT) and SAG12-ipt transgenic creeping bentgrass in response to drought stress. Vertical bars indicate the SEM. Different letters indicate significant differences due to soil water content (% SWC) within a plant type, and asterisks indicate significant differences at a given level of SWC between plant types (P ≤ 0.05). Note the y-axis scaling differences for ease of comparison among treatments of a given metabolite.
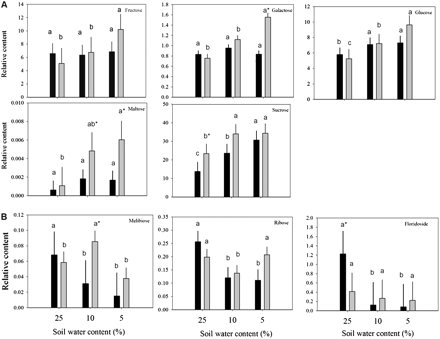
Relative carbohydrate content based on comparison with ribitol as an internal standard and total area of peaks within a given treatment generally (A) increased or (B) either reduced or oppositely accumulated in leaves of null transformant (NT) and SAG12-ipt transgenic creeping bentgrass in response to drought stress. Vertical bars indicate the SEM. Different letters indicate significant differences due to soil water content (% SWC) within a plant type, and asterisks indicate significant differences at a given level of SWC between plant types (P ≤ 0.05). Note the y-axis scaling differences for ease of comparison among treatments of a given metabolite.
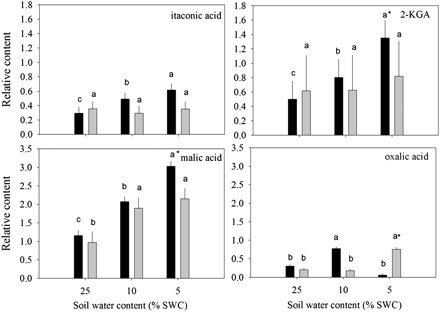
Relative content of organic acids based on comparison with ribitol as an internal standard and total area of peaks within a given treatment generally exhibiting an increase in accumulation in leaves of null transformant (NT) and SAG12-ipt transgenic creeping bentgrass in response to drought stress. Vertical bars indicate the SEM. Different letters indicate significant differences due to soil water content (% SWC) within a plant type, and asterisks indicate significant differences at a given level of SWC between plant types (P ≤ 0.05). Note the y-axis scaling differences for ease of comparison among treatments of a given metabolite.
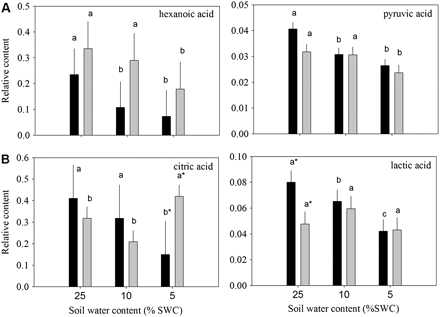
Relative content of organic acids based on comparison with ribitol as an internal standard and total area of peaks within a given treatment generally (A) reduced or (B) oppositely accumulated in leaves of null transformant (NT) and SAG12-ipt transgenic creeping bentgrass leaves in response to drought stress. Vertical bars indicate the SEM. Different letters indicate significant differences due to soil water content (% SWC) within a plant type and asterisks indicate significant differences at a given level of SWC between plant types (P ≤ 0.05). Note the y-axis scaling differences for ease of comparison among treatments of a given metabolite.
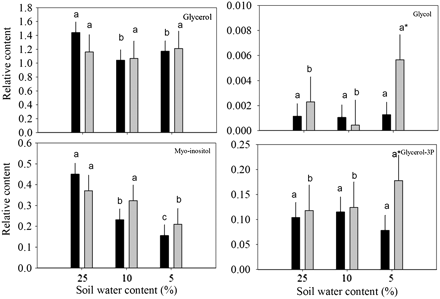
Relative content of organic alcohols based on comparison with ribitol as an internal standard and total area of peaks within a given treatment in response to drought stress in leaves of null transformant (NT) and SAG12-ipt transgenic creeping bentgrass leaves. Vertical bars indicate the SEM. Different letters indicate significant differences due to soil water content (% SWC) within a plant type, and asterisks indicate significant differences at a given level of SWC between plant types (P ≤ 0.05). Note the y-axis scaling differences for ease of comparison among treatments of a given metabolite.
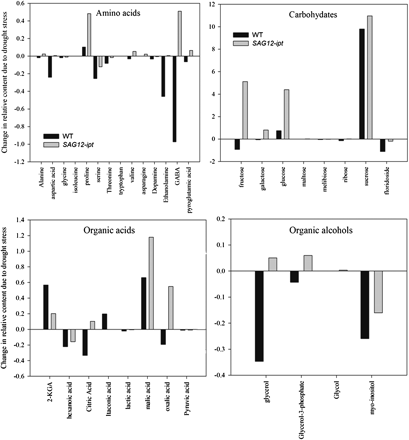
Relative content based on comparison with ribitol as an internal standard and total area of peaks within a given treatment expressed as a change in content from the control condition to 47% leaf relative water content (RWC) of null transformant (NT) and SAG12-ipt transgenic creeping bentgrass leaves in response to drought stress.
Metabolite changes during drought stress
Amino acids and amine derivatives
The total content of amino acids and amine derivatives declined in NT plants by 78% at MD and by 65% at SD, whereas the amino acid content in SAG12-ipt plants significantly increased by 19% under both MD and SD treatments (Fig. 1). The content of individual compounds within this group under well-watered conditions (25% SWC) and during drought stress (at 10% and 5% SWC) had differential patterns of accumulation (Fig. 2). Isoleucine and tryptophan did not have significantly detectable accumulation in response to either drought treatment or the transgene (data not shown). Under watered conditions (25% SWC), the relative contents of the majority of amino acids were not significantly different between plant lines, with the exception of alanine and serine, which were significantly greater in NT plants compared with SAG12-ipt plants.
With increasing drought severity, the content of asparagine, glycine, and proline, increased in either or both transgenic and NT plants (Fig. 2A). Asparagine and proline increased in response to drought stress similarly in both plant lines (Fig. 2A); however, SAG12-ipt had a significantly greater content of proline than NT plants at MD and SD levels (Fig. 2) and at 47% RWC (Fig. 7). Glycine increased in response to drought under MD and declined back to the control level after SD in SAG12-ipt plants, but glycine content was not significantly different in NT plants from the control level (Fig. 2). Aspartic acid, dopamine, ethanolamine, serine, and threonine generally decreased in NT plants, whereas in SAG12-ipt plants a significant decrease was only detected for aspartic acid, threonine, and serine under drought stress (Fig. 2B). Under MD, ethanolamine content decreased by ∼20% in SAG12-ipt leaves whereas it declined by ∼80% in NT leaves. Alanine, γ-aminobutyric acid (GABA), pyroglutamic acid, and valine had an opposite pattern of accumulation in the plant lines (Fig. 2C). Alanine and GABA content both declined in NT plants but were increased in SAG12-ipt plants. For example, the initial contents of alanine were significantly different under control conditions (Fig. 2); however, drought stress caused a decrease in alanine (67%) and GABA (80%) in NT plants, whereas there were increases in GABA (38.5%) and alanine (58%) in SAG12-ipt leaves (Fig. 7).
Carbohydrates and other modified sugars
A total of eight major carbohydrates were identified in both plant lines at both levels of drought stress. Under watered conditions, a significantly greater amount of sucrose and a lower amount of floridoside was detected in SAG12-ipt leaves compared with NT leaves (Table 1). The carbohydrate content generally increased in response to drought stress in both plant lines (Fig. 1). In response to drought stress, the carbohydrates exhibiting a significant increase in both plant lines included glucose, fructose, and sucrose (Fig. 3A). Under SD, fructose and glucose content were ∼50% greater in SAG12-ipt leaves than in the control condition, whereas there was no significant increase in NT leaves for fructose and not such a significant increase in glucose content for NT plants compared with the increase in SAG12-ipt plants. The difference in relative content of these metabolites relative to their respective controls was greater in SAG12-ipt plants than in NT plants at the same level of leaf water deficit (Fig. 7).
Derivitization, relative retention times, and mass to charge (m/z) ratios used for identification and quantification of the 45 metabolites identified in non-transgenic (NT) and SAG12-ipt creeping bentgrass leaves
Compound | Derivative | Retention time (min) | m/z |
Lactic acid | O,O-TMSa | 7.974 | 219 |
Glycolic acid | O,O-TMS | 8.394 | 205 |
Pyruvic acid | O-TMS, H-TMS | 8.654 | 217 |
Alanine | N,O-TMS | 9.047 | 218 |
Oxalic acid | O,O-TMS | 10.021 | 190 |
PAME | O,O-TMS | 11.127 | 256 |
Malonic acid | O,O-TMS | 11.754 | 233 |
Valine | N,O-TMS | 12.008 | 246 |
Serine | O,O-TMS | 13.181 | 234 |
Ethanolamine | N,N, O-TMS | 13.395 | 262 |
H3PO4 | O,O,O-TMS | 13.582 | 299 |
Glycerol | O,O,O-TMS | 13.682 | 293 |
Isoleucine | N,O-TMS | 14.142 | 260 |
Threonine | O,O-TMS | 14.182 | 248 |
Proline | N,O-TMS | 14.222 | 216 |
Glycine | N,N,O-TMS | 14.448 | 276 |
Succinic acid | O,O-TMS | 14.788 | 262 |
Glyceric acid | O,O,O-TMS | 15.176 | 307 |
Glycol | O,O-TMS | 15.435 | 191 |
Itaconic acid | O,O-TMS | 15.529 | 259 |
Fumaric acid | O,O-TMS | 15.789 | 245 |
Methylmaleic acid | O,O-TMS | 17.096 | 259 |
Malic acid | O,O,O-TMS | 19.330 | 335 |
Pyroglutamic acid | N,O, TMS | 19.990 | 258 |
Aspartic acid | N,O,O-TMS | 20.070 | 334 |
GABA | N,O-TMS | 20.237 | 304 |
Threonic acid | O,O,O-TMS | 21.037 | 409 |
Hexanoic acid | N,O,O-TMS | 22.457 | 363 |
Asparagine | N,N,O-TMS | 23.621 | 348 |
Ribose | MEOXb1, O-4TMS | 23.731 | 307 |
Aconitic acid | O,O,O-TMS | 25.492 | 375 |
Glycerol-3-P | O,O,O,O-TMS | 25.626 | 445 |
2-KGA | O-5TMS | 26.466 | 437 |
Shikimic acid | O-4TMS | 26.740 | 462 |
Citric acid | O-4TMS | 26.834 | 465 |
Fructose | MEOX1, O-5TMS | 27.896 | 364 |
Glucose | MEOX1, O-5TMS | 28.397 | 319 |
Galactose | MEOX1, O-5TMS | 28.731 | 319 |
Dopamine | N,N,O,O-TMS | 31.866 | 426 |
Myo-inositol | O-6TMS | 32.060 | 393 |
Tryptophan | N,N,O-TMS | 34.379 | 405 |
Floridoside | O-6TMS | 36.178 | 361 |
Melibiose | O-8TMS | 39.343 | 361 |
Sucrose | O-8TMS | 41.466 | 451 |
Maltose | O-8TMS | 59.018 | 361 |
Compound | Derivative | Retention time (min) | m/z |
Lactic acid | O,O-TMSa | 7.974 | 219 |
Glycolic acid | O,O-TMS | 8.394 | 205 |
Pyruvic acid | O-TMS, H-TMS | 8.654 | 217 |
Alanine | N,O-TMS | 9.047 | 218 |
Oxalic acid | O,O-TMS | 10.021 | 190 |
PAME | O,O-TMS | 11.127 | 256 |
Malonic acid | O,O-TMS | 11.754 | 233 |
Valine | N,O-TMS | 12.008 | 246 |
Serine | O,O-TMS | 13.181 | 234 |
Ethanolamine | N,N, O-TMS | 13.395 | 262 |
H3PO4 | O,O,O-TMS | 13.582 | 299 |
Glycerol | O,O,O-TMS | 13.682 | 293 |
Isoleucine | N,O-TMS | 14.142 | 260 |
Threonine | O,O-TMS | 14.182 | 248 |
Proline | N,O-TMS | 14.222 | 216 |
Glycine | N,N,O-TMS | 14.448 | 276 |
Succinic acid | O,O-TMS | 14.788 | 262 |
Glyceric acid | O,O,O-TMS | 15.176 | 307 |
Glycol | O,O-TMS | 15.435 | 191 |
Itaconic acid | O,O-TMS | 15.529 | 259 |
Fumaric acid | O,O-TMS | 15.789 | 245 |
Methylmaleic acid | O,O-TMS | 17.096 | 259 |
Malic acid | O,O,O-TMS | 19.330 | 335 |
Pyroglutamic acid | N,O, TMS | 19.990 | 258 |
Aspartic acid | N,O,O-TMS | 20.070 | 334 |
GABA | N,O-TMS | 20.237 | 304 |
Threonic acid | O,O,O-TMS | 21.037 | 409 |
Hexanoic acid | N,O,O-TMS | 22.457 | 363 |
Asparagine | N,N,O-TMS | 23.621 | 348 |
Ribose | MEOXb1, O-4TMS | 23.731 | 307 |
Aconitic acid | O,O,O-TMS | 25.492 | 375 |
Glycerol-3-P | O,O,O,O-TMS | 25.626 | 445 |
2-KGA | O-5TMS | 26.466 | 437 |
Shikimic acid | O-4TMS | 26.740 | 462 |
Citric acid | O-4TMS | 26.834 | 465 |
Fructose | MEOX1, O-5TMS | 27.896 | 364 |
Glucose | MEOX1, O-5TMS | 28.397 | 319 |
Galactose | MEOX1, O-5TMS | 28.731 | 319 |
Dopamine | N,N,O,O-TMS | 31.866 | 426 |
Myo-inositol | O-6TMS | 32.060 | 393 |
Tryptophan | N,N,O-TMS | 34.379 | 405 |
Floridoside | O-6TMS | 36.178 | 361 |
Melibiose | O-8TMS | 39.343 | 361 |
Sucrose | O-8TMS | 41.466 | 451 |
Maltose | O-8TMS | 59.018 | 361 |
Methoxime derivative(s).
Trimethylsilyl derivative(s).
Derivitization, relative retention times, and mass to charge (m/z) ratios used for identification and quantification of the 45 metabolites identified in non-transgenic (NT) and SAG12-ipt creeping bentgrass leaves
Compound | Derivative | Retention time (min) | m/z |
Lactic acid | O,O-TMSa | 7.974 | 219 |
Glycolic acid | O,O-TMS | 8.394 | 205 |
Pyruvic acid | O-TMS, H-TMS | 8.654 | 217 |
Alanine | N,O-TMS | 9.047 | 218 |
Oxalic acid | O,O-TMS | 10.021 | 190 |
PAME | O,O-TMS | 11.127 | 256 |
Malonic acid | O,O-TMS | 11.754 | 233 |
Valine | N,O-TMS | 12.008 | 246 |
Serine | O,O-TMS | 13.181 | 234 |
Ethanolamine | N,N, O-TMS | 13.395 | 262 |
H3PO4 | O,O,O-TMS | 13.582 | 299 |
Glycerol | O,O,O-TMS | 13.682 | 293 |
Isoleucine | N,O-TMS | 14.142 | 260 |
Threonine | O,O-TMS | 14.182 | 248 |
Proline | N,O-TMS | 14.222 | 216 |
Glycine | N,N,O-TMS | 14.448 | 276 |
Succinic acid | O,O-TMS | 14.788 | 262 |
Glyceric acid | O,O,O-TMS | 15.176 | 307 |
Glycol | O,O-TMS | 15.435 | 191 |
Itaconic acid | O,O-TMS | 15.529 | 259 |
Fumaric acid | O,O-TMS | 15.789 | 245 |
Methylmaleic acid | O,O-TMS | 17.096 | 259 |
Malic acid | O,O,O-TMS | 19.330 | 335 |
Pyroglutamic acid | N,O, TMS | 19.990 | 258 |
Aspartic acid | N,O,O-TMS | 20.070 | 334 |
GABA | N,O-TMS | 20.237 | 304 |
Threonic acid | O,O,O-TMS | 21.037 | 409 |
Hexanoic acid | N,O,O-TMS | 22.457 | 363 |
Asparagine | N,N,O-TMS | 23.621 | 348 |
Ribose | MEOXb1, O-4TMS | 23.731 | 307 |
Aconitic acid | O,O,O-TMS | 25.492 | 375 |
Glycerol-3-P | O,O,O,O-TMS | 25.626 | 445 |
2-KGA | O-5TMS | 26.466 | 437 |
Shikimic acid | O-4TMS | 26.740 | 462 |
Citric acid | O-4TMS | 26.834 | 465 |
Fructose | MEOX1, O-5TMS | 27.896 | 364 |
Glucose | MEOX1, O-5TMS | 28.397 | 319 |
Galactose | MEOX1, O-5TMS | 28.731 | 319 |
Dopamine | N,N,O,O-TMS | 31.866 | 426 |
Myo-inositol | O-6TMS | 32.060 | 393 |
Tryptophan | N,N,O-TMS | 34.379 | 405 |
Floridoside | O-6TMS | 36.178 | 361 |
Melibiose | O-8TMS | 39.343 | 361 |
Sucrose | O-8TMS | 41.466 | 451 |
Maltose | O-8TMS | 59.018 | 361 |
Compound | Derivative | Retention time (min) | m/z |
Lactic acid | O,O-TMSa | 7.974 | 219 |
Glycolic acid | O,O-TMS | 8.394 | 205 |
Pyruvic acid | O-TMS, H-TMS | 8.654 | 217 |
Alanine | N,O-TMS | 9.047 | 218 |
Oxalic acid | O,O-TMS | 10.021 | 190 |
PAME | O,O-TMS | 11.127 | 256 |
Malonic acid | O,O-TMS | 11.754 | 233 |
Valine | N,O-TMS | 12.008 | 246 |
Serine | O,O-TMS | 13.181 | 234 |
Ethanolamine | N,N, O-TMS | 13.395 | 262 |
H3PO4 | O,O,O-TMS | 13.582 | 299 |
Glycerol | O,O,O-TMS | 13.682 | 293 |
Isoleucine | N,O-TMS | 14.142 | 260 |
Threonine | O,O-TMS | 14.182 | 248 |
Proline | N,O-TMS | 14.222 | 216 |
Glycine | N,N,O-TMS | 14.448 | 276 |
Succinic acid | O,O-TMS | 14.788 | 262 |
Glyceric acid | O,O,O-TMS | 15.176 | 307 |
Glycol | O,O-TMS | 15.435 | 191 |
Itaconic acid | O,O-TMS | 15.529 | 259 |
Fumaric acid | O,O-TMS | 15.789 | 245 |
Methylmaleic acid | O,O-TMS | 17.096 | 259 |
Malic acid | O,O,O-TMS | 19.330 | 335 |
Pyroglutamic acid | N,O, TMS | 19.990 | 258 |
Aspartic acid | N,O,O-TMS | 20.070 | 334 |
GABA | N,O-TMS | 20.237 | 304 |
Threonic acid | O,O,O-TMS | 21.037 | 409 |
Hexanoic acid | N,O,O-TMS | 22.457 | 363 |
Asparagine | N,N,O-TMS | 23.621 | 348 |
Ribose | MEOXb1, O-4TMS | 23.731 | 307 |
Aconitic acid | O,O,O-TMS | 25.492 | 375 |
Glycerol-3-P | O,O,O,O-TMS | 25.626 | 445 |
2-KGA | O-5TMS | 26.466 | 437 |
Shikimic acid | O-4TMS | 26.740 | 462 |
Citric acid | O-4TMS | 26.834 | 465 |
Fructose | MEOX1, O-5TMS | 27.896 | 364 |
Glucose | MEOX1, O-5TMS | 28.397 | 319 |
Galactose | MEOX1, O-5TMS | 28.731 | 319 |
Dopamine | N,N,O,O-TMS | 31.866 | 426 |
Myo-inositol | O-6TMS | 32.060 | 393 |
Tryptophan | N,N,O-TMS | 34.379 | 405 |
Floridoside | O-6TMS | 36.178 | 361 |
Melibiose | O-8TMS | 39.343 | 361 |
Sucrose | O-8TMS | 41.466 | 451 |
Maltose | O-8TMS | 59.018 | 361 |
Methoxime derivative(s).
Trimethylsilyl derivative(s).
Galactose and maltose were less significantly impacted by drought stress or ipt expression; however, their content increased due to SD stress in SAG12-ipt leaves, whereas no significant increase was detected in NT leaves at any level of drought. Carbohydrates exhibiting a decrease in content due to drought stress in NT plants included floridoside (93%), ribose (57%), and melibiose (78%), whereas there was no significant decline in floridoside, only 31% for ribose, and only 56% for melibiose in SAG12-ipt plants. Melibiose exhibited an increase in response to MD followed by a decline under SD in SAG12-ipt plants, whereas only a decrease in melibiose content occurred in response to drought stress in NT plants (Fig. 3B). At the same level of leaf water deficit, fructose, galactose, glucose, and sucrose were increased or maintained better in SAG12-ipt leaves since the difference in relative content relative to their respective controls was greater for SAG12-ipt plants compared with NT plants (Fig. 7).
Organic acids and alcohols
A total of 19 organic acids and four organic alcohols were detected in the leaves of both plant lines. Aconitic acid, malonic acid, glyceric acid, and shikimic acid were detected, but not significantly affected by either ipt expression or drought stress. Fumaric acid, glycolic acid, methylmaleic acid, threonic acid, PAME (phosphoric acid monomethyl ester), and succinic acid increased in response to drought stress but were not significantly different between plant types (data not shown). Total organic acid content exhibited a significant increase in response to drought stress in both plant lines, but under MD for SAG12-ipt plants and under SD for NT plants (Fig. 1). Of the organic acids, 2-keto-gluconic acid (2-KGA) and itaconic acid content increased under drought stress in NT, but not in SAG12-ipt plants. Malic acid content gradually increased with the increase in drought intensity, but the extent of increase was the same at both levels of water deficit for SAG12-ipt plants and lower than in NT plants under SD. Oxalic acid content increased under MD for NT plants and under SD for the SAG12-ipt plants compared with their respective well-watered controls (Fig. 4). Hexanoic acid and pyruvic acid content decreased with drought stress for both plant lines, but occurred later in SAG12-ipt than in NT plants (Fig. 5A). Organic acids oppositly accumulated between the plant lines, including citric acid and lactic acid. The content of citric acid decreased with drought stress in NT but increased in SAG12-ipt plants under SD, and lactic acid declined significantly in NT plants but not significantly in SAG12-ipt plants.
Total organic alcohol content was significantly lower for NT plants under both MD and SD compared with the control, but no significant changes occurred in SAG12-ipt plants (Fig. 1). Changes in organic alcohols in response to drought stress in both plant lines included a decrease in the content of glycerol for NT plants and myo-inositol for both plant lines. Myo-inositol decreased significantly earlier in NT plants than in SAG12-ipt plants; the level of myo-inositol was ∼50% lower in NT plants under MD but was not significantly different from the control condition in SAG12-ipt plants under MD. In response to SD, glycol and glycerol-3-phosphate were significantly increased in SAG12-ipt leaves, but not in NT leaves (Fig. 6).
Discussion
Increased endogenous CK content through ipt transformation has been found to be associated with improved drought tolerance in various plant species (Clark et al. 2004; Zhang et al., 2010; Peleg and Blumwald, 2011), including creeping bentgrass (Merewitz et al., 2010, 2011a, b). The improvements in drought tolerance were observed through evaluation of several physiological parameters at an equivalent level of leaf water deficit. For example, SAG12-ipt plants were better able to maintain chlorophyll content (Merewitz et al., 2010, 2011a) and photochemical efficiency (Merewitz et al., 2010, 2011a) than NT plants under drought stress. Additionally, an elevated CK content was previously found to effect the concentration of other hormones such as abscisic acid (ABA) (Merewitz et al., 2010) and protein content such as antioxidant enzymes (Merewitz et al., 2011b). Thus, the changes in metabolites discussed here could be due to CK content directly or a secondary effect caused by the changes in other signalling agents, hormones, or other biochemical pathways. Despite the well-recognized physiological changes induced by CK, changes in CK-regulated metabolites in relation to drought tolerance are not well documented. Results from the present study suggest that SAG12-ipt creeping bentgrass were able to maintain higher levels of various metabolites or exhibited less decline in the accumulation of some metabolites under drought stress, compared with NT plants, and the differential regulation of major metabolites may reflect the influence of CK on the metabolic pathways associated with improved drought tolerance, as discussed below.
Total carbohydrate content increased under drought stress in both SAG12-ipt and NT creeping bentgrass leaves. In response to drought, inhibition of growth prior to cessation of photosynthesis and the hydrolysis of complex storage carbohydrates can contribute to an increase in the content of simple carbohydrates (Hsiao, 1973; Chaves, 1991; Spollen and Nelson, 1994). The accumulation of sugars in response to drought is frequently cited to be an important mechanism of plant adaptation to drought, particularly due to their role in maintaining cellular hydration by osmotic adjustment (Bray, 1997). Creeping bentgrass leaves that were able to maintain greater levels of carbohydrates under drought stress were found to be more tolerant to dehydration (DaCosta and Huang, 2006). In this study, SAG12-ipt creeping bentgrass was generally able to maintain a greater amount of total carbohydrates than NT plants. This finding could be related to the more active photosynthetic activities and increased level of proteins involved in light harvesting and carbon fixation, as well as other proteins involved in sugar metabolism under drought stress conditions due to elevated CK (Merewitz et al., 2010, 2011a, b). CK deficiency in plants has been shown to cause a decrease in free sugars such as sucrose, glucose, and fructose, with a concomitant increase in starch content in shoot sink tissues of tobacco (Nicotiana tobacum; Werner et al., 2008).
The accumulation of sucrose, galactose, glucose, fructose, and maltose was more apparent in SAG12-ipt than in NT plants in response to drought stress. Sucrose content was significantly greater under well-watered conditions and under drought stress in SAG12-ipt plants compared with NT plants. Peleg et al. (2011) found the sucrose and starch content to be significantly higher in ipt rice (Oryza sativa L.) leaves under water stress, which was related to the finding that several genes for sugar transporters and starch synthesis were differentially regulated in ipt rice relative to the wild type. Rizhsky et al. (2004) found that glucose and fructose were accumulated under drought stress in Arabidopsis. Similar results were found in a metabolomics study of perennial ryegrass (Lolium perenne L.) under water stress, with a greater amount of glucose, fructose, maltose, and other carbohydrates in the more drought-tolerant cultivar (Foito et al., 2009). In potato (Solanum tuberosum), galactose was a primary metabolite accumulated to a greater extent in a drought-tolerant cultivar relative to a sensitive one at similar levels of RWC (Evers et al., 2010). It is known that carbohydrates of primary importance in drought response can be species specific (Gupta and Kaur, 2000). For instance, in ipt transgenic rice, mannitol and trehalose transporters were up-regulated by ipt (Peleg et al., 2011), but these sugars were not detected in the perennial grass species in the present study. In grape (Vitis vinifera ‘Cabernet Sauvignon’), glucose and malate were the primary carbohydrates up-regulated by drought stress (Cramer et al., 2007). Ivic et al. (2001) did not see a significant alteration in sucrose content related to the ipt gene in sugarbeet (Beta vulgaris L.). Nevertheless, changes in CK content may alter accumulation of different forms of carbohydrates, leading to improved drought tolerance, although not all species may exhibit accumulation of the same types of carbohydrates in response to increased CK.
Other carbohydrates differentially regulated between NT and SAG12-ipt plants were melibiose and floridoside. Melibiose is a disaccharide consisting of galactose and glucose moieties and is known to be involved in the promotion of cold tolerance (Sharma et al., 2005). During drought stress, melibiose is often below the detection limit in some species (Evers et al., 2010; Legay et al., 2011), and therefore limited information is available regarding its involvement in drought response or hormonal regulation. In SAG12-ipt leaves an increase in melibiose content was detected under MD and then a significant decrease occurred under SD, whereas melibiose content in NT plants decreased consistently with increasing stress severity. A similar increase in melibiose content followed by a decrease during drought stress was found only in stress-tolerant bermudagrass (Cynodon dactylon) under heat stress, but not in stress-sensitive Kentucky bluegrass (Poa pratensis; Du et al., 2011). Under watered conditions, a significantly lower amount of floridoside was detected in SAG12-ipt leaves compared with NT leaves (Fig. 3B), and the same result was reported by Du et al. (2011) in stress-tolerant bermudagrass. This could be related to a lower amount of natural leaf senescence, since leaf senescence stimulates the breakdown of more complex carbohydrates and starches to cause an increase in low molecular weight sugars for energy availability (Crafts-Brandner et al., 1984). Elevated endogenous CK content and the inhibition of senescence caused a reduction in energy reserve remobilization in ipt tobacco (Cowan et al., 2005). In algae (Rhodophyceae sp.), floridoside is a photoassimilate serving as a ready available energy reserve that can be a quick source of energy since it can be easily cleaved to release glucose (Kremer, 1978). The roles of floridoside in plant stress responses or due to altered CK content, particularly in perennial grasses, are unknown.
The amino acids and amine derivatives asparagine and proline were increased by drought stress in both NT and SAG12-ipt leaves, whereas alanine, GABA, and valine increased only in SAG12-ipt plants. Other compounds, such as aspartic acid and serine, showed decreases due to drought stress in both plant types, or were maintained better by exhibiting less of a decline or no decline in SAG12-ipt leaves, such as ethanolamine and dopamine, respectively. Therefore, the total amino acid content exhibited an increase in SAG12-ipt plants but a decrease in NT plants. Free amino acids are known to accumulate in response to drought stress in various plant species, and drought-tolerant plants generally accumulate more free amino acids than drought-sensitive plants, although a few exceptions have been found (Rai, 2002). Proline accumulation due to drought stress has been studied extensively and is known to exhibit numerous beneficial functions in the stress defence response including osmotic adjustment, stabilization of cellular structures and membranes, and free radical scavenging (Cramer et al., 2007; Szabados and Savoure, 2009). Dobra et al. (2010) found that tobacco plants expressing a transgene to increase endogenous proline content were able to mitigate drought-induced reductions of CK to a greater extent under drought stress than non-transgenic plants with lower levels of proline. In their study, proline content did not significantly alter bioactive CKs under non-stressed conditions. Thomas et al. (1995) found that the act of exogenously applying CK to ipt transgenic tobacco plants may have elicited a slight osmotic stress response which could thereby increase proline content. Therefore, it was unclear whether an increase in proline content was directly related to elevated CK content or was indirectly caused through osmotic adjustment. It has been noted that the CK 6-benzylaminopurine delays leaf senescence, and a concomitant increase in proline content was observed (Alvarez et al., 2008), but conflicting results have been found on the direct effect of benzylaminopurine and other CKs on proline biosynthesis genes (Hu et al., 1992). The interaction between CK and proline content could also be related to the accumulation of carbohydrates such as glucose, fructose, mannitol, and, most notably, sucrose, since these carbohydrates have been found to play a role in increasing proline accumulation (Kishor et al., 2005). In the present study, proline content increased to a greater extent in SAG12-ipt plants than in NT plants during drought stress, which could enhance osmotic adjustment and other stress defence mechanisms for plant tolerance to drought stress. However, whether proline accumulation in SAG12-ipt plants is due to direct effects of elevated CK content or is a result of an increase in carbohydrate content such as of sucrose or an osmotic response is unclear. Thus, the association of elevated CK content with proline accumulation in SAG12-ipt plants may be worth further investigation.
Several other free amino acids accumulated differentially in SAG12-ipt and NT leaves under drought stress. Asparagine exhibited an accumulation in response to drought in both SAG12-ipt and NT plants, but had a more marked increase in SAG12-ipt plants when both plants experienced the same level of leaf water deficit (47% RWC). Asparagine content has been found either to increase or to decrease in response to drought, depending on the plant species and stress severity (Stewart and Larher, 1980; Aranjeulo et al., 2011). Previously it was found by proteomic analysis of the same creeping bentgrass plants that several proteins involved in amino acid metabolism had differential expression patterns between NT and SAG12-ipt plants. For instance, the leaf proteins methionine synthase (MS), S-adenosylmethionine synthetase (SAMS), aspartate and alanine aminotransferases, and the root asparagine-tRNA ligase accumulated more or were degraded less in SAG12-ipt relative to the change in NT in during drought stress conditions (Merewitz et al., 2011b). Changes in MS, SAMS, and aminotransferases could play a role in the altered accumulation pattern of various amino acids due to differential fluxes through the various amino acid synthesis and degrative pathways and play a role in osmotic adjustment (Bohnert and Jensen, 1996). Asparagine-tRNA ligase is an enzyme that catalyses the reaction determining the aminoacyl-tRNA activity state and has been linked to the regulation of expression of asparagine synthetase, and could lead to asparagine synthesis by feedback mechanisms, or function in other non-ribosomal pathways (Arfin et al., 1977; Mocibob et al., 2010). In addition, asparagine accumulation has been linked to a delay in leaf senescence (Downs et al., 1997). Thus, the increased content of asparagine and aminoacyl-tRNA could be related to the increased endogenous CK and to the improved drought tolerance of SAG12-ipt plants. However, this mechanism is still unclear. Valine exhibited a large increase in SAG12-ipt leaves in response to drought, but did not change significantly in NT leaves. Metabolic profiling of pea (Pisum sativum L.) conducted by Charlton et al. (2008) reported that valine along with leucine, isoleucine, and threonine were important metabolites exhibiting a large degree of accumulation in response to drought stress. Pyroglutamic acid (also known as 5-oxoproline) is an uncommon amino acid and is not well documented in drought stress response; however, it may be associated with freezing tolerance in Arabidopsis (Korn et al., 2010). As an intermediate, pyroglutamic acid is a precusor to the synthesis of glutamate (Ohkama-Ohtsu et al., 2008) and proline (Jones, 1985). Glycine is a proteogenic amino acid that functions in the biochemical pathway as a precursor leading to purines such as CKs and other porphyrin structures (Mok and Mok, 1994), and drought-responsive metabolites such as glycine betaine (Weretilnyk et al., 1989) and glycine may act as a signalling molecule (Dubos et al., 2003; Bouche and Fromm, 2004). Glycine is also a predominant precursor of the antioxidant compound glutathione and may result from an increase in photorespiration, which has been attributed to protection of the photosynthetic apparatus during drought stress (Rivero et al., 2009, and references therein). The accumulation of the amino acids such as valine, pyroglutamic acid, and glycine could contribute to improved drought tolerance in SAG12-ipt plants as a source of precursors for key metabolic pathways regulating drought tolerance.
Alanine and GABA were differentially regulated between the plant lines since they increased in response to drought in SAG12-ipt leaves, but decreased in NT leaves. Increased alanine due to drought stress has been detected in other plant species. In rapeseed (Brassica napus), the accumulation of alanine was due to an increase in the precursors pyruvate and glutamate, and not due to enzymes in the alanine biosynthetic pathway (Good and Zaplachinski, 1994). The same mechanism may be occurring in creeping bentgrass because a clear trend in accumulation of alanine aminotransferase was not observed previously by proteomic analysis (Merewitz et al., 2011b). Several studies have shown that GABA accumulates in response to drought stress (Raggi, 1994; Bor et al., 2008). GABA is a non-protein amino acid that may be a signalling molecule in plants involved in regulating numerous stress response mechanisms such as the carbon:nitrogen balance, osmotic potential, free radical scavenging, pH regulation, plant growth, and root development (Kinnersley and Turano, 2000; Bouche and Fromm, 2004). Specific to drought stress, GABA may function primarily as an osmolyte (Shelp et al., 1999). However, studies evaluating GABA content have demonstrated a greater GABA content in drought-sensitive compared with drought-tolerant cultivars (Kinnersley and Turano, 2000), whereas other studies have found GABA receptor genes to be expressed more in drought-tolerant cultivars than in sensitive ones (Guo et al., 2009). In regards to GABA as a signalling molecule, it has long been known that GABA interacts with various hormones in animals but has only recently been indicated to have this function in plants (Bouche and Fromme, 2004); therefore, the role of GABA in the drought response and its relationship to CK remain to be elucidated. This study may provide some evidence that CK signalling may alter the flux through the GABA shunt pathway or may be associated with changes in oxidative status or other signalling pathways. The differences in GABA content in SAG12-ipt plants compared with NT plants could be related to differences in the content of GABA precursors (pyroglutamic acid and proline), differences in CK, growth habits, or osmotic adjustment, but the specific implications of GABA accumulation cannot be directly concluded from this study alone. Future work focused on how CK signalling pathways such as via calmodulin (Brault and Maldiney, 1999; Müller and Sheen, 2007) may relate to the shift in GABA and alanine content may be warranted. In general, the differential abilities of NT and SAG12-ipt plants to maintain content at the control level, or actually to increase levels of various free amino acids under drought stress, could be related to CK accumulation and may be a factor promoting drought-enhanced tolerance in SAG12-ipt plants.
Of all the compounds in the amino acid and amino acid derivative category, that exhibited a decrease in content in response to drought, ethanolamine (ETA) and dopamine were less responsive to drought stress in SAG12-ipt plants than in NT plants. ETA is primarily found in plant membranes as the head group of polar lipids. Maintenance of ETA during drought stress could be beneficial to SAG12-ipt plants since ETA-containing lipids inhibit the activity of phospholipase D reactions. Phospholipases break down lipid membrane bilayers and re-organize lipid saturation and structure (Austin-Brown and Chapman, 2002). Additionally, ETA is a precursor to stress-protective compounds such as glycine betaine and has been found to accumulate due to abiotic stress in other plant species, protecting cellular membranes from stress damage (Suzuki et al., 2003). It has been found that SAG12-ipt plants with the ability to maintain ETA content under drought stress exhibited less membrane damage, as demonstrated by reduced electrolyte leakage, compared with NT plants (Merewitz et al., 2010). Dopamine is a catecholamine regulating various growth and development processes and affecting carbohydrate metabolism and the actions of plant hormones such as ethylene (Dai et al., 1993; Świędrych et al., 2004; Kulma and Szopa, 2007). The synthesis of catecholamines is regulated by stress conditions, but the role and actions in stress responses are not well understood (Kulma and Szopa, 2007). Previously CK compounds have been shown to inhibit dopamine-13-hydroxylases (Abdelnour-Esquivel et al., 1992). It is unknown whether the maintenance of dopamine content in SAG12-ipt plants may be due to less drought damage or a reduction in the activity of degrading enzymes in plants with elevated CK content.
The organic acids citric, oxalic, and malic acids may be important metabolites regulated by CK content since these compounds were maintained to a greater extent or were increased by drought stress in SAG12-ipt leaves (Fig. 7). Citric acid is a key intermediate of the citric acid (TCA) cycle where it is created from incoming pyruvate and acetyl Co-A molecules. The maintenance of citric acid content could be related to a differential flux through the TCA pathway in SAG12-ipt plants under drought stress. Oxalic acid is also known to be a source of defence against biotic stress (Punja, 2001). In grass pea (Lathyrus sativus L.), an accumulation of free polyamines due to drought stress was correlated with an increase in the β-N-oxalyl-l-α,β-diaminopropionic acid (ODAP), and the synthesis of ODAP requires oxalic acid (Xiong et al., 2006). Polyamines have been shown play a large role in environmental stress protection by functioning to reduce membrane lipid peroxidation, scavenging free radicals, and other mechanisms (Pang et al., 2007). Thus, in SAG12-ipt plants, oxalic acid could indirectly play a role in drought tolerance. Malic acid accumulated to a greater extent in SAG12-ipt plants. In crested wheatgrass (Agropyron cristatum), malic acid was shown to accumulate due to drought stress and this was attributed to protection of roots from drought via accumulation in root exudates (Henry et al., 2007). Specific differences in the content of some of the organic acids could have an effect on the differential drought tolerance characteristics of SAG12-ipt and NT plants. It should be noted that differential accumulation of organic acids could also be related to respiratory processes such as glycolysis. Rivero et al. (2007) have found photorespiration to be enhanced in ipt transgenic tobacco plants and previously several enzymes involved in respiration and energy production, such as glyceraldehyde phosphate dehydrogenase (GAPDH), leaf aldolases, triose phosphate isomerases, and enolases have been found to be maintained better in SAG12-ipt bentgrass under drought stress (Merewitz et al., 2011b). However, how most of the organic acids relate to the drought response or CK content remains to be determined due to the complexity of the metabolic pathways.
Organic alcohols such as glycerol, myo-inositol, and other organic alcohols are involved in promoting stress tolerance, particularly through osmotic adjustment during salt and drought stresses (Shevleva et al., 1997;,Nelson et al., 1998; Bartels and Sunkar, 2005). Several organic alcohols were maintained at greater levels in SAG12-ipt plants compared with NT plants under drought stress. Organic alcohols are beneficial osmoregulators that are generally non-toxic to cells and have a relatively low energy cost of production (Chen and Jiang, 2009). Glycerol-3-phosphate was also greater at equivalent water deficit in SAG12-ipt plants compared with NT plants, and is primarily used metabolically for rapid generation of NAD+ (Shen et al., 2006). Recently, glycerol and glycerol-3-phosphate, in addition to their essential role in glycolysis and lipid formation, have been found to be important components of signalling the defence response against biotic stress (Venugopal et al., 2009). Partra et al. (2010) found that transgenic tobacco plants with enhanced contents of myo-inositol and other inositol derivatives exhibit better growth and photosynthetic activity, and a reduction of oxidative stress during salt stress. Previously a promotion of photosynthesis and photosynthetic proteins and reduced oxidative damage and increased antioxidant enzyme activity were found in SAG12-ipt plants (Merewitz et al., 2010, 2011a, b). Therefore, it is worth noting that differences in organic alcohols could be related to various stress-protective mechanisms in addition to osmotic adjustment and signalling as found previously. The importance of organic alcohols in the response of creeping bentgrass to CK and drought stress may be worth investigation.
In conclusion, the greater drought tolerance of creeping bentgrass plants with an ipt transgene promoting elevated levels of endogenous CK was associated with changes in the total content of several major metabolites and differential accumulation of some specific metabolites. The most significant metabolites that exhibited greater accumulation in SAG12-ipt than in NT plants under drought include amino acids such as alanine, glycine, valine, proline, GABA, ethanolamine, and pyroglutamic acid, carbohydrates such as fructose, glucose, and galactose, organic acids such as citric acid, malic acid, and oxalic acid, and the organic alcohol myo-inositol and glycerol-3-phosphate. These compounds could be related to stress defence due to their roles in the stress response pathways such as stress signalling, osmotic adjustment, and respiration for energy production. The method of maintenance of these compounds (i.e. due to less degradation or enhanced biosynthesis) and their relationship to CK may deserve further investigation.
Abbreviations
- DW
dry weight
- FW
fresh weight
- GABA
γ-aminobutyric acid
- G3P
glucose3 phosphate
- hexanoic acid
6-hydroxy-2-aminohexanoic acid
- 2-KGA
2-keto-gluconic acid
- PAME
phosphoric acid monomethyl ester
- TW
turgid weight
Comments