-
PDF
- Split View
-
Views
-
Cite
Cite
Fedor A. Brovko, Victoria S. Vasil'eva, Antonina L. Lushnikova, Svetlana Yu. Selivankina, Natalia N. Karavaiko, Khanafy M. Boziev, Anna O. Shepelyakovskaya, Dmitry A. Moshkov, Liubov L. Pavlik, Victor V. Kusnetsov, Olga N. Kulaeva, Cytokinin-binding protein (70 kDa) from etioplasts and amyloplasts of etiolated maize seedlings and chloroplasts of green plants and its putative function, Journal of Experimental Botany, Volume 61, Issue 12, July 2010, Pages 3461–3474, https://doi.org/10.1093/jxb/erq170
- Share Icon Share
Abstract
Cytokinins regulate chloroplast differentiation and functioning, but their targets in plastids are not known. In this connection, the plastid localization of the 70 kDa cytokinin-binding protein (CBP70) was studied immunocytochemically in 4-d-old etiolated maize seedlings (Zea mays L., cv. Elbrus) using monoclonal antibodies (mAbs) against CBP70 recognizing this protein not only in nuclei and cytoplasm, but also in plastids. CBP70 was detected in the amyloplasts of the root cap and etioplasts of the mesocotyl, stem apex, and leaves encircling the stem axis in the node. Immunogold electron microscopy demonstrated CBP70 localization in amyloplasts outside starch grains and revealed a dependence of CBP70 content in etioplasts on the degree of their inner membrane differentiation: the low CBP70 amount in etioplasts at the early stages of membrane development, the high content in etioplasts with actively developing membranes, and a considerable decrease in plastids with the formed prolamellar body. This suggests that CBP70 is involved in etioplast structure development. CBP70 was also observed in chloroplasts of the bundle sheath of green maize leaves. CBP70 purified from etioplasts mediated trans-zeatin-dependent activation of transcription elongation in vitro in the transcription systems of maize etioplasts and barley chloroplasts, suggesting that CBP70 is a plastid transcription elongation factor or a modulator of plastid elongation factor activity. CBP70 involvement in the cytokinin-dependent regulation of plastid transcription elongation could be essential for the cytokinin control of the biogenesis of this organelle.
Introduction
The phytohormone cytokinin plays an important role in the regulation of plant growth and development (Mok and Mok, 2001; Argueso et al., 2009). Chloroplasts are among the main targets of cytokinin action in the plant cell. Cytokinins participate in the control of chloroplast biogenesis (Stetler and Laetsch, 1965; Kusnetsov et al., 1994; Zavaleta-Mancera et al., 1999a), activate chloroplast differentiation from proplastids (Khokhlova, 1977; Longo et al., 1979), stimulate chloroplast protein and pigment biosyntheses (Parthier, 1979; Kusnetsov et al., 1994; Zavaleta-Mancera et al., 1999b), and activate the expression of nuclear (Abdelghani et al., 1991; Kusnetsov et al., 1994; Chory et al., 1994) and plastid (Zubo et al., 2005, 2008; Brenner et al., 2005) genes encoding chloroplast proteins. Chloroplasts harbour enzymes, participating in cytokinin biosynthesis, and contain a set of natural cytokinins, including free bases, ribosides, ribotides, and N-glucosides (Benková et al., 1999; Kasahara et al., 2004; Hirose et al., 2008). Transformation of tobacco plants with genes involved in cytokinin metabolism demonstrated that chloroplasts are rather independent in the regulation of their cytokinin metabolism (Polanska et al., 2007). However, in spite of chloroplast significance for cytokinin biosynthesis and an important role of cytokinins in the control of chloroplast biogenesis, the molecular mechanism of cytokinin action in chloroplasts remains unknown. In recent years, a great advance was achieved in the understanding of the mechanism of cytokinin action in the plant cell, including the elucidation of cytokinin membrane receptors and the pathway of cytokinin signal transduction via the multistep phosphate relay into the nucleus, culminated by the induction of cytokinin-responsive genes (Ferreira and Kieber, 2005; Muller and Shen, 2007; Santner and Estelle, 2009). At the same time, the pathways of cytokinin perception and signal transduction in chloroplasts remain to be elucidated. It is not clear whether cytokinin exerts its regulatory action in chloroplasts via the nucleus-cytoplasm compartment or via the regulatory systems in chloroplasts per se. In this connection, the investigation of barley chloroplast cytokinin-binding protein (chlCBP) inducing cytokinin-dependent activation of transcription elongation in chloroplast lysates (Kulaeva et al., 2000) is of interest. ChlCBP activated chloroplast transcription in vitro in combination with trans- but not cis-zeatin (Lyukevich et al., 2002), which indicates its specificity toward trans-zeatin. The data suggest that chlCBP could be a chloroplast cytokinin-dependent transcription elongation factor or the protein affecting the activity of these factors. So far, there is no evidence about the presence of this protein in etioplasts and amyloplasts. The elucidation of this question is of interest because it is known that not only chloroplasts but also etioplasts and amyloplasts could be targets for cytokinin action. Thus, cytokinins activated proplastid differentiation into etioplasts in detached pumpkin cotyledons in darkness (Khokhlova, 1977) and induced amyloplast formation from proplastids in tobacco cell culture (Sakai et al., 1992; Miyazawa et al., 2002).
The availability of the set of monoclonal mouse antibodies (mAbs) against maize CBP with a molecular mass of 70 kDa (CBP70) (Zagranichnaya et al., 1997) made it possible to use immunocytochemistry and immunogold electron microscopy to study CBP70 localization in plant cells (Brovko et al., 2007).
The aims of this work were (i) to study CBP70 localization in the etioplasts of shoots and the amyloplasts of roots of 4-d-old etiolated maize seedlings and in the chloroplasts of 3-week-old green maize plants; and (ii) to isolate CBP70 from etioplasts and to analyse its activity in cytokinin-dependent regulation of transcription elongation in vitro in etioplast and chloroplast transcription systems.
Materials and methods
Plant materials and culture
Maize (Zea mays L., cv. Elbrus) seeds were surface-sterilized and germinated on moistened filter paper at 27 °C and 95% humidity in darkness (Brovko et al., 2007). Four-day-old seedlings were sampled for immunocytochemistry, nuclei and etioplast isolation, and CBP70 analyses. Maize plants were grown in a growth chamber with a 16 h photoperiod, 22/18 °C day/night temperatures, at 222 μmol m−2 s−1 light intensity, and 70% relative humidity. Third leaves of 3-week-old plants were used for immunocytochemistry. Barley (Hordeum vulgare L., cv. Viner) plants were grown under the same conditions as maize plants. The fully expanded first leaves of 10-d-old plants were used for chloroplast isolation.
Immunocytochemistry
Monoclonal antibodies, used in the present study, were raised by Zagranichnaya et al. (1977). In brief, adult BALB/c mice were immunized with purified CBP70 from etiolated maize seedlings; fusions were performed by the method of Köhler and Milstein (1975) with some modification, using SP2/0 myeloma cells and 50% polyethylene glycol. Fusions were screened for CBP70 specific antibody-producing cells by the enzyme immunoassay (EIA). Each mAb was purified by precipitation of the IgG from ascites fluid by the addition of 50% (NH4)2SO4 and subsequent chromatography on a MonoQ column using FPLC. Specificity of each antibody for the CBP70 was determined by reaction with intracellular proteins blotted from SDS-PAGE and detected by the EIA. MAbs raised against 70 kDa CBP cross-reacted with barley 67 kDa CBP. The subclass, to which each antibody belongs, was determined by EIA using commercially prepared subclass-specific antisera (Bio-Rad, USA). An estimation of the affinity constant for each antibody was calculated by the method described by Beaty et al. (1987).
Root tips, mesocotyls, and nodes with the stem axis encircled by leaves of 4-d-old etiolated maize seedlings were cut off and treated according to the procedure described by Brovko et al. (2007). The middle parts of the third leaf (10 cm from leaf base) of 3-week-old green maize plants were also treated in the same way. Plant material was immediately fixed in 4% (w/v) formaldehyde, 0.1% (w/v) glutaraldehyde, and 0.01% (w/v) picric acid in 20 mM sodium phosphate buffer (pH 7.4) for 8–12 h at 4 °C. After washing in distilled water, the samples were dehydrated in graded ethanol (to 100%) and embedded in LR White (Sigma, USA). Polymerization was carried out under UV light for 12 h. Sections from embedded samples were cut with the Ultracut E ultramicrotome (Reichert-Jung, Austria). For light microscopy, semi-thin sections (0.7–1.0 μm) were collected on glass slides coated with γ-aminopropyltriethoxysilane. For electron microscopic observations, ultrathin sections were mounted onto mesh Au grids.
Prior to immunolabelling, sections were permeabilized with 0.5% (v/v) Triton X-100 and incubated in 0.1% (w/v) NaBH4 to inactivate residual aldehydes. The sections were incubated in the blocking solution of BSA-G [3% (w/v) BSA and 0.5% (w/v) gelatine in PBS] for 1.5 h at 37 °C and then incubated at 4 °C overnight with the primary monoclonal antibodies against CBP70 (17 μg ml−1) diluted in the BSA-T solution [1% (w/v) BSA and 0.01% (v/v) Triton X-100 in PBS]. For control treatments, this step was omitted or substituted as described below. Slides with the sections were washed in the W solution: [0.02% (w/v) NaN3, 0.1% (v/v) Triton X-100, and 0.1 M glycine in PBS] for 10 min and incubated with the BSA-G solution for 20 min. Then the sections were incubated with biotinylated anti-mouse antibodies (Amersham, UK) in BSA-T for 6 h at 4 °C and washed in the W solution for 10 min. The reaction with the streptavidin-alkaline phosphatase conjugate (Amersham) in BSA-T was performed for 1.5 h at 37 °C; slides were washed in the W solution for 10 min, then incubations with biotinylated anti-mouse antibodies and streptavidin-phosphatase conjugate (for 1.5 h at 37 °C each) were repeated. The sections were incubated with the substrate solution (3.8 mM BCIP, 4 mM NBT, 10 mM MgCl2, 150 mM NaCl, and 50 mM TRIS-HCl, pH 9.5) for 15 min. The reaction was stopped by washing in 20 mM TRIS-HCl, pH 7.5. The stained sections were dried and mounted in cedar wood oil, and images were obtained under a Univar microscope (Reichert-Jung, Austria).
Three negative controls were used: (i) mouse anti-CBP70 mAbs were omitted; (ii) mouse anti-CBP70 mAbs were substituted by mouse mAbs raised against a protein, which is absent from plants (anti-Myc antibodies); and (iii) mouse anti-CBP70 antibodies were substituted by mouse non-immune serum (5% mouse serum diluted by PBS). An equally low unspecific staining was detected for all three controls under the chosen conditions of immunolabelling.
Immunolabelling procedure for electron microscopy
Immunolabelling was performed according to Brovko et al. (2007). Ultrathin sections for electron microscopy were mounted onto Au grids. Grids with sections of the root tip and leaf tissues of 4-d-old etiolated maize seedlings as well as ultrathin sections of the middle part (10 cm from the leaf base) of the third leaf of 3-week-old green maize plants were incubated in the moisture chamber. Most of the procedures were carried out at room temperature. Initially, specimens were incubated with a drop of freshly prepared 1% (w/v) aqueous solution of NaBH4 for 30 min, and then they were rinsed with distilled water (three times for 5 min). Unspecific antibody binding was blocked by incubation of specimens in the 5% solution of BSA (w/v) in PBST-500 (16.7 mM Na2HPO4, 3.3 mM KH2PO4, 500 mM NaCl, and 0.1% Tween 20, pH 7.4) for 20 min. Succeeding reactions were carried out using the same buffer. After each incubation, probes were washed in PBST-500 with 50 mM glycine and 0.5% Tween 20. Specimens were incubated with primary anti-CBP70 antibodies for 1 h at 37 °C. Control sections were incubated with non-immune serum. Then the sections were incubated with the solution of biotinylated anti-mouse antibodies (Amersham) (at a dilution of 1:100) for 1 h at room temperature, followed by incubation in the solution of streptavidin gold (15 nm) (Amersham) (at a dilution of 1:100) under the same conditions. The labelled sections were post-stained in uranyl acetate and Reynold`s lead citrate before examination with a Tesla TS-500 electron microscope (Czech Republic) (Vandenbosch, 1991).
Plastid isolation
Plastids were isolated from the first leaf of 5-d-old etiolated maize seedlings. All procedures were performed at 4 °C. The leaf was cut into 1–2 mm pieces, put into 4 vols of buffer A cooled to 4 °C (50 mM TRIS-HCl, pH 7.6, 10 mM MgCl2, and 5 mM KCl) containing 0.3 M sucrose; 10 mM 2-mercaptoethanol; 0.2 mM phenylmethylsulphonyl fluoride (PMSF), and 0.01% polyvinylpyrrolidone (PVP), and kept for 30 min in darkness. Plant material was homogenized using Ultra Turax (IKA, Germany) for 5–6 s at 28 000 rpm; the homogenate was filtered through several layers of nylon mesh and centrifuged at 200 g for 5 min. The supernatant was centrifuged at 800 g for 15 min; the pellet was resuspended in buffer A, loaded onto a discontinuous sucrose gradient (30/45/65%) in buffer B containing 50 mM TRIS-HCl, pH 7.6, 10 mM MgCl2, 5 mM KCl, and 0.01% PVP, and centrifuged at 25 000 g in a JA 20 rotor (Beckman, USA) for 45 min. Plastids were concentrated at the interface between 45% and 65% sucrose. They were collected, and the purity of the fraction obtained was checked by the analysis of plastid pigment fluorescence under an Opton 16 HAL fluorescent microscope (Opton, Germany). The preparation obtained was frozen in liquid nitrogen and stored at –86 °C.
CBP isolation from plastids
Preparations of plastids isolated from 100 g of leaves were diluted twice with buffer C (25 mM TRIS-HCl, pH 7.4, 25 mM KCl, 2 mM MgCl2, and 2 mM Na2EDTA), 5 mM PMSF, and 2 mM 2-mercaptoethanol were added; and incubation was carried out for 10 min at continuous stirring. The suspension was centrifuged at 100 000 g for 1 h in the Ti45 rotor (Beckman). The supernatant was collected; the pellet was resuspended in three volumes of buffer C containing 250 mM KCl, 5 mM PMSF, and 2 mM 2-mercaptoethanol were added; and the suspension was incubated for 1 h at stirring and centrifuged at 100 000 g for 30 min in the Ti45 rotor (Beckman). The supernatants from the first and second centrifugations were combined and loaded onto the Sephadex G25 Fine column (2.6×40 cm) equilibrated with buffer C; the protein fraction was loaded on DEAE Sepharose Fast Flow column (1.5×2 cm) equilibrated with buffer C. The column was washed with three volumes of buffer C. CBP was eluted with buffer C supplemented with 200 mM KCl. CBP-containing fractions were combined and used for CBP isolation by tandem affinity chromatography using adenosine Toyopearl and trans-zeatin riboside Toyopearl according to the procedure described by Brovko et al. (2007). Cytokinin-binding activity in the eluate was determined at all steps of protein purification by precipitation of tZ-CBP complex by ammonium sulphate (Brovko et al., 1996).
Isolation of nuclei and nuclear CBP
Nuclei were isolated from 4-d-old etiolated maize seedlings by the procedure described by Hamilton et al. (1972). CBP was isolated from nuclei according to Brovko et al. (2007).
CBP refolding
CBP-containing fractions were collected and concentrated to the volume of 0.5 ml in the 8MC cell, using the YM10 membrane (Millipore, USA). Then the buffer containing 6 M guanidine hydrochloride was replaced by the buffer for refolding (10 mM TRIS-HCl, pH 7.4, 25 mM KCl, 2 mM MgCl2, 2 mM Na2EDTA, 2 mM 2-mercaptoethanol, and 100 nM ZnCl2 (Colvard and Wilson, 1984). During this procedure, at least 50 vols of refolding buffer (25 ml) were pumped through the cell for concentrating the protein. The obtained preparation of CBP was analysed by SDS-PAGE, and immunoblotting and used for functional studies.
Electrophoresis and immunoblotting
Proteins from the crude extracts of etioplasts and CBP70 isolated from nuclei and etioplasts were analysed by SDS-PAGE (12% w/v) according to the method of Laemmli (1970). Separated proteins were transferred to Hybond-C extra membrane (Amersham, UK) in a semi-dry electroblotter (Biometra, UK) according to the manufacturer's recommendations using the Towbin buffer system (Towbin et al., 1979). Protein bands were visualized with 0.5% Ponceau S solution in 1 M acetic acid. The membrane was rinsed three times with water and blocked with 1% BSA in PBS-T [phosphate-buffered saline containing 0.1% (v/v) Tween 20] overnight at 4 °C. Anti-CBP70 mouse mAbs (100 ng ml−1) in PBS-T was added for 1 h at room temperature. Then the anti-CBP70 mouse antibody was detected using secondary antibody conjugated to alkaline phosphatase (Bio-Rad Laboratories, USA) according to the supplier's recommendations. The enzyme colour reaction was developed with 3-bromo-4-chloro-5-indolyl phosphate (BCIP) and nitroblue tetrazolium (NBT).
Transcription assay systems
The effects of CBP70 isolated from etioplasts of 5-d-old etiolated maize seedlings on the etioplast and chloroplast transcription systems were assayed in the reaction medium (100 μl) containing etioplast or chloroplast lysate (10–50 μg of DNA), 50 mM TRIS-HCl pH 8.0, 10 mM MgCl2, 10 mM 2-mercaptoethanol, 0.2 mM ATP, GTP, CTP, 0.01 mM UTP, and [3H]UTP (1.11 TBq mmol−1) (Amersham Biosciences, UK) and 30–60 ng of CBP70. The reaction was carried out at 30 °C for 20 min and stopped by the addition of an equal volume of cold 0.9% sodium pyrophosphate in 10% trichloracetic acid.
Protein assay
Protein content was determined according to the method of Esen (Esen, 1978), using Comassie brilliant blue R-250 for staining.
Statistics
All experiments were performed three times independently with three replications each. Tables present the mean values and their standard errors.
Results
Selection of anti-CBP70 antibodies recognizing CBP70 in plastids with immunogold microscopy and immunocytochemical analysis
In the present work it was established that in the panel of mouse mAbs raised against CBP70 from etiolated maize seedlings, there are two groups of mAbs differing in their reaction with CBP70 in immunogold electron microscopy and the immunocytochemical analysis of etiolated maize seedlings and green leaf tissues. mAbs of the first group detected CBP70 in the nuclei and cytoplasm, whereas mAbs of the second group, in addition to their interaction with CBP70 in the nuclei and cytoplasm, effectively detected this protein in plastids. The difference in properties of the two mAb groups is demonstrated in Table 1 and Fig. 1. Table 1 presents the results of immunogold electron microscopy obtained with the use of the representatives of both mAb groups: mAb 14A4 belonging to the second group of mAbs and mAb 11B1 belonging to the first group. mAb 14A4 detected CBP70 in leaf etioplasts and root-cap amyloplasts. Control samples with plant material treated with non-immune serum contained practically negligible amounts of gold particles in plastids. mAb 11B1 detected no gold particles in leaf etioplasts and root amyloplasts, indicating the absence of its interaction with plastid CBP70. At the same time, mAbs of both groups detected practically similar amounts of CBP70 in the nucleoplasm, nucleolus, and cytoplasm of leaf and root-cap cells (Table 2).
Quantification of gold particles in leaf etioplasts and in root-cap amyloplasts of 4-d-old etiolated maize seedlings analysed by immunogold electron microscopy using anti-CBP70 mAbs of first (11B1) and second (14A4) groups
Plastid type | Antibody | No. gold particles μm−2 |
Leaf etioplast | 14A4 | 19.57±11.82 |
– | 0.21±0.07 | |
11B1 | 0.34±0.04 | |
– | 0.35±0.06 | |
Root-cap amyloplast | 14A4 | 26.92±6.21 |
– | 0.29±0.09 | |
11B1 | 0.56±0.09 | |
– | 0.42±0.07 |
Plastid type | Antibody | No. gold particles μm−2 |
Leaf etioplast | 14A4 | 19.57±11.82 |
– | 0.21±0.07 | |
11B1 | 0.34±0.04 | |
– | 0.35±0.06 | |
Root-cap amyloplast | 14A4 | 26.92±6.21 |
– | 0.29±0.09 | |
11B1 | 0.56±0.09 | |
– | 0.42±0.07 |
Quantification of gold particles in leaf etioplasts and in root-cap amyloplasts of 4-d-old etiolated maize seedlings analysed by immunogold electron microscopy using anti-CBP70 mAbs of first (11B1) and second (14A4) groups
Plastid type | Antibody | No. gold particles μm−2 |
Leaf etioplast | 14A4 | 19.57±11.82 |
– | 0.21±0.07 | |
11B1 | 0.34±0.04 | |
– | 0.35±0.06 | |
Root-cap amyloplast | 14A4 | 26.92±6.21 |
– | 0.29±0.09 | |
11B1 | 0.56±0.09 | |
– | 0.42±0.07 |
Plastid type | Antibody | No. gold particles μm−2 |
Leaf etioplast | 14A4 | 19.57±11.82 |
– | 0.21±0.07 | |
11B1 | 0.34±0.04 | |
– | 0.35±0.06 | |
Root-cap amyloplast | 14A4 | 26.92±6.21 |
– | 0.29±0.09 | |
11B1 | 0.56±0.09 | |
– | 0.42±0.07 |
Gold particle distribution in various compartments of the leaf and root-cap cells of 4-d-old etiolated maize seedlings analysed by immunogold electron microscopy using anti-CBP70 mAbs of first (11B1) and second (14A4) groups, no. gold particles μm−2
Plant organ | Antibody | Nucleoplasm | Nucleolus | Cytoplasm | Plastids |
Leaf | 14A4 | 9.26±3.79 | 18.92±0.83 | 5.14±0.33 | 21.60±5.83 |
– | 0.37±0.06 | 0.20±0.07 | 0.32±0.09 | 0.22±0.06 | |
11B1 | 9.61±3.98 | 20.89±0.97 | 5.95±0.40 | 0.35±0.03 | |
– | 0.39±0.08 | 0.20±0.06 | 0.55±0.10 | 0.33±0.06 | |
Root cap | 14A4 | 9.14±3.93 | 19.14±0.91 | 4.20±0.53 | 27.30±6.52 |
– | 0.28±0.08 | 0.37±0.06 | 0.30±0.07 | 0.28±0.08 | |
11B1 | 10.96±4.53 | 21.45±0.84 | 4.99±0.48 | 0.51±0.09 | |
– | 0.51±0.09 | 0.35±0.06 | 0.50±0.08 | 0.39±0.08 |
Plant organ | Antibody | Nucleoplasm | Nucleolus | Cytoplasm | Plastids |
Leaf | 14A4 | 9.26±3.79 | 18.92±0.83 | 5.14±0.33 | 21.60±5.83 |
– | 0.37±0.06 | 0.20±0.07 | 0.32±0.09 | 0.22±0.06 | |
11B1 | 9.61±3.98 | 20.89±0.97 | 5.95±0.40 | 0.35±0.03 | |
– | 0.39±0.08 | 0.20±0.06 | 0.55±0.10 | 0.33±0.06 | |
Root cap | 14A4 | 9.14±3.93 | 19.14±0.91 | 4.20±0.53 | 27.30±6.52 |
– | 0.28±0.08 | 0.37±0.06 | 0.30±0.07 | 0.28±0.08 | |
11B1 | 10.96±4.53 | 21.45±0.84 | 4.99±0.48 | 0.51±0.09 | |
– | 0.51±0.09 | 0.35±0.06 | 0.50±0.08 | 0.39±0.08 |
Gold particle distribution in various compartments of the leaf and root-cap cells of 4-d-old etiolated maize seedlings analysed by immunogold electron microscopy using anti-CBP70 mAbs of first (11B1) and second (14A4) groups, no. gold particles μm−2
Plant organ | Antibody | Nucleoplasm | Nucleolus | Cytoplasm | Plastids |
Leaf | 14A4 | 9.26±3.79 | 18.92±0.83 | 5.14±0.33 | 21.60±5.83 |
– | 0.37±0.06 | 0.20±0.07 | 0.32±0.09 | 0.22±0.06 | |
11B1 | 9.61±3.98 | 20.89±0.97 | 5.95±0.40 | 0.35±0.03 | |
– | 0.39±0.08 | 0.20±0.06 | 0.55±0.10 | 0.33±0.06 | |
Root cap | 14A4 | 9.14±3.93 | 19.14±0.91 | 4.20±0.53 | 27.30±6.52 |
– | 0.28±0.08 | 0.37±0.06 | 0.30±0.07 | 0.28±0.08 | |
11B1 | 10.96±4.53 | 21.45±0.84 | 4.99±0.48 | 0.51±0.09 | |
– | 0.51±0.09 | 0.35±0.06 | 0.50±0.08 | 0.39±0.08 |
Plant organ | Antibody | Nucleoplasm | Nucleolus | Cytoplasm | Plastids |
Leaf | 14A4 | 9.26±3.79 | 18.92±0.83 | 5.14±0.33 | 21.60±5.83 |
– | 0.37±0.06 | 0.20±0.07 | 0.32±0.09 | 0.22±0.06 | |
11B1 | 9.61±3.98 | 20.89±0.97 | 5.95±0.40 | 0.35±0.03 | |
– | 0.39±0.08 | 0.20±0.06 | 0.55±0.10 | 0.33±0.06 | |
Root cap | 14A4 | 9.14±3.93 | 19.14±0.91 | 4.20±0.53 | 27.30±6.52 |
– | 0.28±0.08 | 0.37±0.06 | 0.30±0.07 | 0.28±0.08 | |
11B1 | 10.96±4.53 | 21.45±0.84 | 4.99±0.48 | 0.51±0.09 | |
– | 0.51±0.09 | 0.35±0.06 | 0.50±0.08 | 0.39±0.08 |
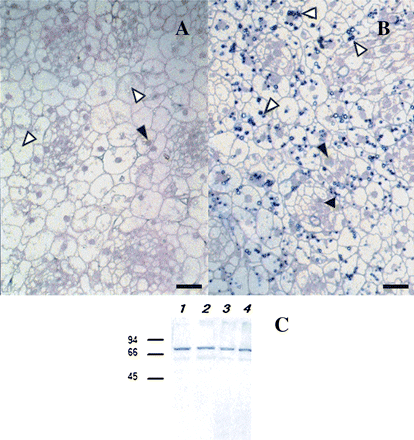
Comparison of the reaction of two groups of anti-CBP70 mAbs with CBP70 as examined immunocytochemically and with Western immunoblot analysis. LR White-embedded cross-sections of the stem apex of dark-grown 4-d-old maize seedlings were used. (A) Sections were incubated with mAbs against CBP70 of the first group (11B3) or (B) the second group (14A4), biotinylated anti-mouse antibody, and streptavidin-alkaline phosphatase conjugate. (A) mAb 11B3 detected CBP70 in nuclei (black arrowhead), but not in plastids (white arrowheads). (B) mAb 14A4 also detected CBP70 in nuclei (black arrowhead), but more effectively reacted with it in plastids (white arrowheads). Scale bars are 25 μm (A, B). (C) Western immunoblot detection of CBP70 isolated from nuclei and etioplasts of etiolated maize seedlings. Blots were probed with mouse anti-CBP70 mAbs of the first (11B3) or second (14A4) group, and the antibody was detected using the secondary anti-mouse antibody conjugated with alkaline phosphatase. Lanes 1, 2, CBP70 from nuclei was detected with mAb 11B3 (lane 1) or with mAb 14A4 (lane 2); lanes 3, 4, CBP70 from etioplasts was detected with mAb 11B3 (lane 3) or with mAb 14A4 (lane 4). Molecular weight standards in kDa are indicated on the left.
A difference between the two groups of mAbs in their cross-reactivity with plastid CBP70 is also clearly seen in Fig. 1, representing the results of the immunocytochemical analysis of CBP70 on cross-sections of the stem apex of 4-d-old dark-grown maize seedling. As evident from Fig. 1A, mAb of the first group (11B3) detected CBP70 in the nuclei (black arrowhead) but not in plastids (white arrowhead); by contrast, mAb of the second group (14A4) recognized CBP70 in the nuclei and cytoplasm (black arrowheads), but also, and with a better efficiency, in plastids (Fig. 1B, white arrowheads).
Figure 1C presents a Western immunoblot of CBP70 isolated from nuclei and etioplasts and detected by anti-CBP mAb of the first (mAb 11B3) or the second (mAb 14A4) groups. Under conditions of immunoblotting, when the protein was completely denatured and all epitopes were available for interaction with mAbs, both groups of mAbs cross-reacted with CBP70 from plastids and nuclei. Thus, epitope availability for mAbs determined the differences in the interaction of the two mAb groups with plastid-localized CBP70 on the sections of plant tissues. The detection of anti-CBP mAbs, which recognized the protein in plastids, allowed the investigation of CBP70 localization in amyloplasts and etioplasts of etiolated seedlings and in chloroplasts of green leaves.
CBP70 localization in amyloplasts of the root-tips
Localization of CBP70 in amyloplasts of the root tips of 4-d-old etiolated maize seedlings was studied immunocytochemically, using two mAbs of the second group (14A4 and 523). These antibodies belong to different subclasses and differ in affinity to CBP70. mAb 523 belongs to IgG2b subclass, whereas mAb 14A4 is IgG1. Affinity constants of these mAbs are 1×107 M−1 and 1×106 M−1, respectively. Both mAbs recognized CBP70 in plastids. On longitudinal sections of the root cap, CBP70 was recognized in the amyloplasts of the columella (Fig. 2A, C; black arrows), using mAb 523. In contrast to columella, no plastids containing CBP70 were detected in the cells of the calyptrogen (Fig. 2A). No staining was seen on control sections of the root tip treated with non-immune serum (Fig. 2B), demonstrating a high specificity of CBP70 recognition by mAbs (cf. Fig. 2A, C with Fig. 2B). Only nuclei coloured with safranin were distinguished in the control samples (Fig. 2B). Figure 2C presents the pattern of CBP70 localization in amyloplasts at a greater magnification (black arrows). Thus, due to the use of anti-CBP70 mAbs of the second group in cytochemical investigations, the high content of CBP70 in amyloplasts of the root-cap columella was demonstrated for the first time.
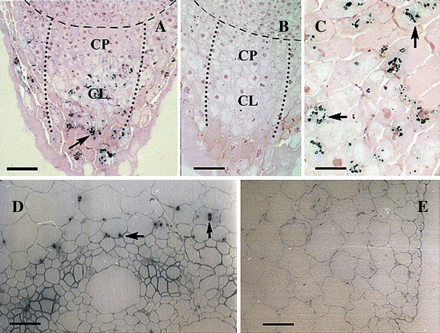
Immunolocalization of CBP70 in longitudinal sections of the root tip and on cross-sections of the mesocotyl of etiolated maize seedlings. LR White-embedded sections of the root tip (A–C) and mesocotyl (D, E) of dark-grown 4-d-old maize seedlings were analysed. (A, C, D, E) Sections were incubated with mouse anti-CBP70 mAb of the second group (523), biotinylated anti-mouse antibody, and streptavidin-alkaline phosphatase conjugate. (B) A negative control: sections incubated with non-immune serum were not stained. (A–C) Sections were post-stained with safranin. (A) CBP70 was detected in amyloplasts of the columella of the root cap (black arrow). Broken line designates a boundary between the root apex and root cap. Dotted lines enclose the zones of columella (CL) and calyptrogen (CP). Amyloplasts with stained CBP70 are well distinguished in the columella cells (black arrow). (C) A 2.5-fold magnified view of (A). (D) CBP70 was detected in plastids of parenchymal cells surrounding vascular bundles (black arrow). (E) No CBP70 was detected in the mesocotyl large parenchymal and epidermal cells. Scale bars are 75 μm (A), 60 μm (B, D), 40 μm (E), and 30 μm (C).
CBP70 localization in etioplasts
Plastid localization of CBP70 was recognized also on the cross-sections of mesocotyl and node of 4-d-old etiolated maize seedlings using anti-CBP70 mAbs of the second group, namely, mAb 14A4. CBP70 was detected in the plastids of mesocotyl cells surrounding vascular bundles (Fig. 2D, black arrows). No plastids containing CBP70 were detected in large parenchymal cells and epidermis (Fig. 2E).
On the cross-section of the node, the stem apex surrounded by leaves is presented (Fig. 3). The marked differences in CBP70 level in the plastids of leaves surrounding the stem apex were observed (Fig. 3A–C). Plastids containing CBP70 were abundant in the second leaf. This is clearly seen on the section without staining with safranin (Fig. 3B, black arrow). Localization of CBP70-containing plastids around the nucleus is shown (Fig. 3B). As evident from Fig. 3C, etioplasts of the second leaf cells are very heterogeneous in the CBP70 level. This is seen even in the case of neighbouring plastids (Fig. 3D). Practically no plastids with CBP70 were detected in the first, third, and fourth leaves, differing in their age from the second leaf. These data suggest that different CBP70 amounts in plastids of different leaves could depend on the stage of plastid development.
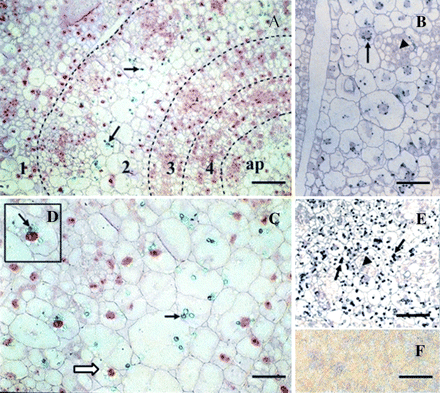
Immunolocalization of CBP70 on cross-sections of the node of etiolated maize seedlings. LR White-embedded sections of the node from dark-grown 4-d-old maize seedlings were analysed. Sections were incubated with mouse anti-CBP70 mAb of the second group (14A4), biotinylated anti-mouse antibody, and streptavidin-alkaline phosphatase conjugate. (F) A negative control was the same as in Fig. 2: sections were treated with non-immune serum. (A, C, D) Post-staining with safranin. (A) Immunochemically stained cross-section of the node. Broken lines designate the boundaries of leaves encircling the stem axis; figures indicate leaf numbers in the order of their formation; ap, stem apex. CBP70 was detected in plastids of the second leaf (black arrows). (C) A 2-fold magnification of the fragment of the second leaf. (D) Enlarged fragment of (C). (C, D) Plastid heterogeneity in CBP70 content is seen: plastids containing CBP70 (black arrow); plastids not containing CBP70 (white arrow). (B) Cross-section of the second leaf without post-staining with safranin. The arrangement of CBP70-containing plastids around the nucleus is seen (black arrow). (E) Stem apex without post-staining with safranin. A great amount of plastids containing CBP70 is seen (black arrows). (B, E) Plastids with CBP70 are absent from the zones of active cell division. CBP70 was recognized in these zones in the nuclei and cytoplasm (black triangles). Scale bars are 50 μm (A), 25 μm (B, C, E).
Application of mAb 14A4 demonstrated the presence of a great number of CBP70-containing plastids in the apex (Fig. 3E, black arrows). Such plastids were absent from the sites of cell division within the apex, where mAb 14A4 revealed the accumulation of CBP70 in the nuclei and its presence in the cytoplasm (Fig. 3E, black triangles).
Hence, use of anti-CBP70 mAbs of the second group permitted CBP70 detection in plastids of the mesocotyl, stem apex, and leaves surrounding the stem apex, as well as in nuclei and cytoplasm at sites of cell division in the apex.
The distribution pattern of CBP70 in leaf and root cells of etiolated maize seedlings studied by immunogold electron microscopy
CBP70 localization in the second leaf and root-cap cells of 4-d-old etiolated maize seedlings was studied with immunogold electron microscopy.
Table 2 demonstrates the distribution pattern of CBP70 in leaf and root-cap cells, as analysed with immunogold electron microscopy using anti-CBP mAb of the first group 11B1 recognizing the protein in nuclei and cytoplasm and mAb 14A4 cross-reacting with CBP70 not only in nuclei and cytoplasm, but also in plastids. Both groups of mAbs detected CBP70 in nuclei with predominant accumulation in the nucleolus. mAbs of the second group also detected CBP70 accumulation in plastids: in amyloplasts of the root cap and in etioplasts of leaf cells. CBP70 was not detected by mAbs of both groups in vacuoles and cell walls.
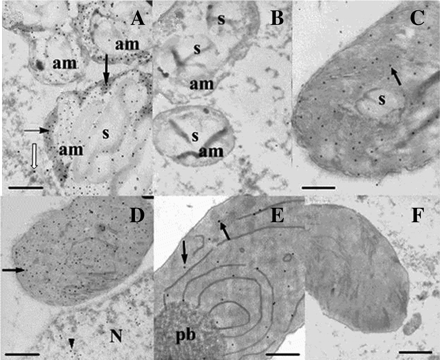
Immunogold electron microscopic detection of CBP70 localization in amyloplasts of the root cap and in etioplasts of the second leaf of the node of etiolated maize seedlings. Ultrathin sections obtained from the root cap (A) and leaf cells (C–E) of 4-d-old etiolated maize seedlings were treated with mouse anti CBP70 mAb of the second group (14A4) or (B, F) with non-immune serum as a negative control, biotinylated anti-mouse antibody, and streptavidin-gold (15 nm). (A) High amount of CBP70 was detected in amyloplasts of the root cap outside starch grains (black arrows). Low amount of CBP70 was in the cytoplasm (white arrow). (B) Negative control for amyloplast: no gold particles are seen. (C) Low amount of CBP70 in etioplasts with poorly developed membrane system (black arrow). (D) High amount of CBP70 in etioplasts with actively developing inner membrane system (black arrow). CBP70 was also detected in the nucleoplasm (black arrowhead). (E) Extremely low content of CBP70 in the etioplast with developed prolamellar body. (F) A negative control for etioplasts. am, amyloplast; s, starch grain; pb, prolamellar body. Scale bars are 1 μm.
Figure 4A demonstrates the high concentration of gold particles in amyloplasts of the root cap. CBP70 was seen in amyloplasts outside the starch grains. Thus, the immunogold technique confirmed the immunocytochemical data on the localization of the CBP70 in amyloplasts of the root cap. Control sections were treated with non-immune serum instead of mAbs against CBP70 (Fig. 4B, F). No gold particles were detected in plastids of the control sections.
The immunogold technique also detected CBP70 in leaf etioplasts (Fig. 4C–E). The concentration of gold particles differed drastically in etioplasts with different development of the plastid inner membrane system (Table 3). The level of CBP70 in etioplasts at the initial stages of membrane differentiation was lower than in plastids with advanced membrane development (Fig. 4C, D). The amount of CBP70 dropped sharply in etioplasts with completely developed prolamellar bodies (Fig. 4E, Table 3). Thus, the CBP70 level in etioplasts changed during the development of their inner membrane system.
Quantification of gold particles in the amyloplasts and etioplasts of 4-d-old etiolated maize seedlings and chloroplasts of green leaf analysed with immunogold electron microscopy using anti-CBP70 mAbs of the second group (14A4)
Plastid | No. gold particles per 1 μm2 |
Amyloplast | 40.0±2.0 |
Etioplasta | |
I | 3.4±0.2 |
II | 26.6±1.3 |
III | 1.8±0.1 |
Chloroplast | 14.1±1.4 |
Plastid | No. gold particles per 1 μm2 |
Amyloplast | 40.0±2.0 |
Etioplasta | |
I | 3.4±0.2 |
II | 26.6±1.3 |
III | 1.8±0.1 |
Chloroplast | 14.1±1.4 |
I, Etioplasts at the beginning of inner membrane development; II, etioplasts with actively developing inner membranes; III, etioplasts with differentiated prolamellar bodies.
Quantification of gold particles in the amyloplasts and etioplasts of 4-d-old etiolated maize seedlings and chloroplasts of green leaf analysed with immunogold electron microscopy using anti-CBP70 mAbs of the second group (14A4)
Plastid | No. gold particles per 1 μm2 |
Amyloplast | 40.0±2.0 |
Etioplasta | |
I | 3.4±0.2 |
II | 26.6±1.3 |
III | 1.8±0.1 |
Chloroplast | 14.1±1.4 |
Plastid | No. gold particles per 1 μm2 |
Amyloplast | 40.0±2.0 |
Etioplasta | |
I | 3.4±0.2 |
II | 26.6±1.3 |
III | 1.8±0.1 |
Chloroplast | 14.1±1.4 |
I, Etioplasts at the beginning of inner membrane development; II, etioplasts with actively developing inner membranes; III, etioplasts with differentiated prolamellar bodies.
CBP70 in chloroplasts of bundle-sheath cells in green leaves
It was important to elucidate whether CBP70 detected in etioplasts is preserved in the mature chloroplasts of green leaves. In these experiments, the middle part of the third leaf (at a distance of 10 cm from the leaf base) of 3-week-old green maize plants was used. The immunocytochemical analysis and immunogold electron microscopy of CBP70 on the leaf sections were performed similarly to the root and shoot sections of etiolated maize seedlings, using anti-CBP70 mAb of the second group (14A4). The mesophyll and bundle-sheath cells of the leaves of maize, a C4 plant, contain chloroplasts differing in their structure and function. In green maize leaves, large cells of the bundle sheath surround the vessel bundle (Fig. 5A). The bundle-sheath cells comprise large elongated chloroplasts positioned near the cell wall opposite that adjoining the vessel bundle (Fig. 5A). Immunochemical reaction with alkaline phosphatase revealed in every chloroplast discrete brightly coloured regions containing CBP70 and situated in sequence with one another along the longitudinal chloroplast axis (Fig. 5A, black arrow). Such CBP70 accumulation was not detected in the chloroplasts of mesophyll cells (data not shown). Section treatment with non-immune serum did not reveal any staining in the bundle-sheath chloroplasts, indicating a specificity of anti-CBP70 mAb (14A4) interaction with CBP70 (Fig. 5B). Figure 5A also shows the region of the nucleus containing CBP70, as detected with mAb 14A4.
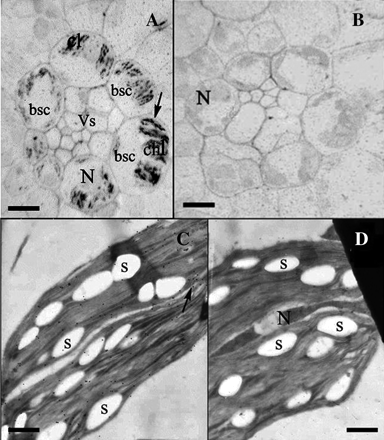
Immunochemical and immunogold electron microscopic detection of CBP70 in chloroplasts of bundle-sheath cells of green maize leaves. (A, B) Immunochemical localization of CBP70 on cross-sections of the middle part (10 cm from the base) of the third leaf of 20-d-old green maize plants. LR White-embedded leaf sections were used. (A) Sections were incubated with mouse anti-CBP70 mAb of the second group (14A4) or (B) with non-immune serum as a negative control, biotinylated anti-mouse antibodies, and streptavidin-alkaline phosphatase conjugate. (A) CBP70 in chloroplasts of bundle-sheath cells surrounding vascular bundle. Large elongated chloroplasts are positioned in bundle-sheath cells near the cell wall opposite to the vascular bundle. CBP70 is localized within chloroplasts in discrete regions arranged along the longitudinal chloroplast axis (black arrow). Plastids in other cells are not stained. (B) Negative control: CBP70 was not detected. (C) Immunogold electron microscopic detection of CBP70 in chloroplasts of bundle-sheath cells of the third leaf of 20-d-old maize green plant. (C) Ultrathin sections were treated with mouse anti-CBP70 mAbs of the second group (14A4) or (D) with non-immune serum (negative control), biotinylated anti-mouse antibodies, and streptavidin-gold (15 nm). CBP70 is localized in the chloroplast (black arrow); it is absent from starch grains bsc-bundle-sheath cells; chl, chloroplast; N, nucleus; S, starch grain; Vs, vascular bundle. Scale bars are 30 μm (A, B) and 1 μm (C, D).
Immunogold electron microscopy confirmed CBP70 localization in mature chloroplasts of the bundle-sheath cells of green leaves (Fig. 5C; Table 3). Gold particles were observed in the chloroplast stroma. No gold particles were present in the control section treated with non-immune serum (Fig. 5D). Thus, plastid CBP70 was detected not only in amyloplasts and etioplasts of etiolated maize seedlings but also in differentiated chloroplasts of green leaves, for example, in the bundle-sheath cells. In the mesophyll chloroplasts, the content of CBP70 was lower than could be detected immunocytochemically. However, the low CBP70 content in mesophyll chloroplasts could be detected by immunogold technique (data not shown). Thus, the content of CBP70 in chloroplasts of mesophyll and bundle-sheath cells differed drastically in accordance with the different structure and function of these plastids.
Activation of transcription elongation in vitro in the etioplast and chloroplast lysates by CBP70 in the presence of trans-zeatin
To study the functional activity of plastid CBP70, the protein was isolated from etioplasts of shoots of 5-d-old etiolated maize seedlings, purified by affinity chromatography, subjected to refolding, and was analysed by Western blotting using anti-CBP70 of the second group (Fig. 6). The same results were obtained with mAb 11B3 of the first group (data not shown). Figure 6 presents CBP70 from the crude protein extract of etioplast (lane 1) and the purified protein (lane 2).
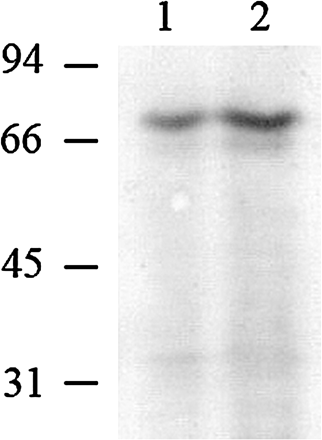
Western immunoblot detection of CBP70 isolated from etioplasts of etiolated maize seedlings. Proteins were analysed by SDS-PAGE. Blots were probed with mouse anti-CBP70 mAbs of the second group (14A4), and the antibody was detected using the secondary anti-mouse antibody conjugated with alkaline phosphatase. Lane 1, 100 μg of plastid proteins. Lane 2, CBP70 (2 μg) isolated by affinity chromatography using tZR-Toyopearl. Molecular weight standards are indicated on the left (kDa).
CBP70 activity in the regulation of plastid transcription was tested in the etioplast lysate containing all components of the transcription system. Such a system carried out elongation of transcripts initiated in vivo. In the presence of tZ, CBP70 activated RNA elongation in the etioplast lysate (Table 4). No activation was exerted by CBP70 alone or tZ alone. Thus, plastid CBP70 mediated cytokinin-dependent activation of etioplast transcription elongation.
Cytokinin-dependent activation of transcript elongation in etioplast lysates by CBP70 from etioplasts of etiolated maize seedlings
CBP70 (ng per 100 μl) | Trans-zeatin 10−7 M | [3H]UMP incorporation into RNA | |
cpm per 10 μg DNA | % | ||
0 | – | 38682±1802 | 100 |
0 | + | 35204±2201 | 91 |
48 | – | 31196±2464 | 81 |
16 | + | 47627±3810 | 123 |
32 | + | 78595±6951 | 203 |
48 | + | 115614±6127 | 299 |
CBP70 (ng per 100 μl) | Trans-zeatin 10−7 M | [3H]UMP incorporation into RNA | |
cpm per 10 μg DNA | % | ||
0 | – | 38682±1802 | 100 |
0 | + | 35204±2201 | 91 |
48 | – | 31196±2464 | 81 |
16 | + | 47627±3810 | 123 |
32 | + | 78595±6951 | 203 |
48 | + | 115614±6127 | 299 |
Cytokinin-dependent activation of transcript elongation in etioplast lysates by CBP70 from etioplasts of etiolated maize seedlings
CBP70 (ng per 100 μl) | Trans-zeatin 10−7 M | [3H]UMP incorporation into RNA | |
cpm per 10 μg DNA | % | ||
0 | – | 38682±1802 | 100 |
0 | + | 35204±2201 | 91 |
48 | – | 31196±2464 | 81 |
16 | + | 47627±3810 | 123 |
32 | + | 78595±6951 | 203 |
48 | + | 115614±6127 | 299 |
CBP70 (ng per 100 μl) | Trans-zeatin 10−7 M | [3H]UMP incorporation into RNA | |
cpm per 10 μg DNA | % | ||
0 | – | 38682±1802 | 100 |
0 | + | 35204±2201 | 91 |
48 | – | 31196±2464 | 81 |
16 | + | 47627±3810 | 123 |
32 | + | 78595±6951 | 203 |
48 | + | 115614±6127 | 299 |
In the presence of tZ, CBP70 from maize etioplasts also activated transcription elongation in lysates of barley leaf chloroplasts (Table 5). Thus, CBP70 detected in etioplasts of etiolated maize seedlings was not only preserved in mature chloroplasts of green maize leaves but manifested activity in the cytokinin-dependent control of transcription elongation in the chloroplast transcription system.
Cytokinin-dependent activation of transcript elongation in barley chloroplast lysates by CBP70 from etioplasts of etiolated maize seedlings
Treatment | Incorporation of 3H-UMP into RNA | |
cpm per 10 μg DNA | % of Control | |
Control | 27106±1221 | 100 |
Trans-zeatin | 26292±1314 | 97 |
CBP70 | 33875±2371 | 125 |
Trans-zeatin+CBP70 | 76984±5388 | 284 |
Treatment | Incorporation of 3H-UMP into RNA | |
cpm per 10 μg DNA | % of Control | |
Control | 27106±1221 | 100 |
Trans-zeatin | 26292±1314 | 97 |
CBP70 | 33875±2371 | 125 |
Trans-zeatin+CBP70 | 76984±5388 | 284 |
Concentration of trans-zeatin in reaction medium was 10−7 M, CBP70 was added in the amount of 32 ng per 100 μl.
Cytokinin-dependent activation of transcript elongation in barley chloroplast lysates by CBP70 from etioplasts of etiolated maize seedlings
Treatment | Incorporation of 3H-UMP into RNA | |
cpm per 10 μg DNA | % of Control | |
Control | 27106±1221 | 100 |
Trans-zeatin | 26292±1314 | 97 |
CBP70 | 33875±2371 | 125 |
Trans-zeatin+CBP70 | 76984±5388 | 284 |
Treatment | Incorporation of 3H-UMP into RNA | |
cpm per 10 μg DNA | % of Control | |
Control | 27106±1221 | 100 |
Trans-zeatin | 26292±1314 | 97 |
CBP70 | 33875±2371 | 125 |
Trans-zeatin+CBP70 | 76984±5388 | 284 |
Concentration of trans-zeatin in reaction medium was 10−7 M, CBP70 was added in the amount of 32 ng per 100 μl.
Discussion
Identification among the set of mAbs raised against CBP70 from etiolated maize seedlings, the mAbs recognizing CBP70 in plastids showed CBP70 localization in amyloplasts and etioplasts of etiolated seedlings and in chloroplasts of green maize plants (Figs 1, 2, 3, 5). This is the first information on CBP70 localization in plastids. The presence of CBP70 in all types of plastids studied suggests its important role in these organelles. CBP70 in plastids was recognized only by mAbs against CBP70 of the second group (14A4, 523) in immmunocytochemical analysis and immunogold electron microscopy on leaf and root-tip sections (Table 1; Fig. 1B). The mAb of the first group (11B1) did not interact with CBP70 in plastids (Table 1; Fig. 1A). However, in Western immunoblot analysis, when the protein was completely dissociated and denatured, both groups of mAbs interacted not only with nuclear but also with plastid CBP70 (Fig. 1C). Thus, a difference between the two groups of mAbs depended on the difference in the availability for mAbs corresponding to the CBP70 epitope within the plastids. It seems likely that the molecular surrounding of CBP70 in plastids masked the protein epitopes responsible for the protein recognition by mAbs of the first group.
In the previous work (Brovko et al., 2007), also performed on 4-d-old etiolated maize seedlings, the application of the mAbs of the first group allowed CBP70 detection in nuclei of all cell types in the seedling roots and shoots; the highest amount of protein was detected in the meristem zones where it was also detected in the cytoplasm. In the nuclei and cytoplasm in the zones of active cell divisions within the shoot apex, the presence of CBP70 was also demonstrated in this study with the use of the mAbs of the second group (Fig. 3E). However, the most important and principally novel fact discovered in this study was the presence of CBP70 in all types of plastids.
CBP70 in the leaf and root-cap cells was quantified with immunogold electron microscopy using mAbs of both groups (Table 2). The predominant of CBP70 localization was established in nuclei and plastids. Its low amount was detected in the cytoplasm, and no CBP70 was found in the vacuoles and cell walls. Thus, CBP70 accumulated in places, where its functional activity in transcription regulation was demonstrated.
CBP70 localization in amyloplasts
Using anti-CBP70 mAbs of the second group, the high level of CBP70 was observed in amyloplasts in the cells of the root-cap columella (Fig. 2A, C). Immunogold electron microscopy demonstrated CBP70 accumulation in amyloplasts positioned outside starch grains, where CBP70 was not detected (Fig. 4A). It has long been known that specialized amyloplasts of the root cap, statoliths, are involved in the perception of the gravity signal (Sievers et al., 1989; Volkmann et al., 1991; Braun et al., 2002). However, the involvement of CBP70 in this process seems not very likely because this protein was also detected in non-sedimenting amyloplasts of marginal cells. The involvement of CBP70 in the cytokinin-dependent amyloplast formation from proplastids seems more likely. A correlation between the high content of CBP70 in amyloplasts of the columella cells in maize root cap (Table 3), data on the extremely high content of active cytokinins in the columella of the Arabidopsis root cap (Aloni et al., 2005), and the findings that the high cytokinin concentration in nutrition medium induced amyloplast differentiation from proplastids in tobacco cell culture (Sakai et al., 1992; Miyazawa et al., 2002) argues for this suggestion.
A transcription assay using isolated plastid nucleoids demonstrated that cytokinin-induced conversion of proplastids into amyloplasts was accompanied by changes in the transcriptional activities of plastid genes (Sakai et al., 1992). Since it was demonstrated that plastid CBP70 participated in cytokinin-dependent activation of transcription elongation in vitro in the plastid transcription system (Table 4), CBP70 accumulation in amyloplasts permits the suggestion that it is involved in the control of transcription related to the biogenesis of these organelles.
According to da Costa e Silva et al. (2004), mutation in the gene encoding the chloroplast factor of transcription elongation ET in maize plants could lead to the damage not only of chloroplast but also of amyloplast development. Thus, the factors of transcription elongation could affect plastid biogenesis.
CBP70 localization in etioplasts and chloroplasts
In 4-d-old etiolated maize seedlings, CBP70 was detected in etioplasts of the mesocotyl (Fig. 2D), leaves of the node (Fig. 3A–D), and stem apex (Fig. 3E). Earlier Mackender (1978) demonstrated a great heterogeneity in the structure and degree of differentiation of the etioplast population in etiolated maize leaves. In agreement with the results by Mackender, the population of etioplasts in the leaves encircling the stem axis of etiolated seedlings also differed dramatically in the CBP70 level (Fig. 3A). Great differences were observed not only between etioplasts of different leaves and cells but also within a single cell (Fig. 3D). The second leaf contained the highest amount of etioplast CBP70 (Fig. 3A, B). In the older first leaf and the younger third and fourth leaves, most etioplasts did not contain CBP70 (Fig. 3A), suggesting that the level of CBP70 in etioplasts depended on the degree of their development. This assumption was confirmed with immunogold electron microscopy using anti-CBP70 mAbs of the second group. This technique demonstrated that CBP70 content in etioplasts differed markedly in the dependence on the development of etioplast inner membranes (Table 3): the amount of CBP70 was low in etioplasts at the beginning of membrane differentiation (Fig. 4C), increased dramatically with the enhancement of this process (Fig. 4D), and decreased in etioplasts with the developed prolamellar bodies (Table 3; Fig. 4C, D, E). The data on the dramatic differences in the CBP70 level in etioplasts differing in the degree of their inner membrane differentiation suggest that CBP70 was involved in processes related to etioplast biogenesis.
The functional significance of CBP70 in plastids was emphasized by the detection of the protein in chloroplasts of 3-week-old green maize plants. CBP70 accumulation was demonstrated with both immunocytochemistry and electron microscopy (Fig. 5) in chloroplasts of bundle-sheath cells, typical for C4 plants (Romanowska and Drožak, 2006). It is necessary to emphasize that proteomic analysis revealed pronounced differences in the composition of polypeptides of etioplasts and chloroplasts (Zychlinski et al., 2005) and demonstrated that only those etioplast proteins were preserved in chloroplasts, which were involved in chloroplast differentiation and function (Blomqvist et al., 2008). Therefore, the presence of CBP70 protein in mature chloroplasts in green maize leaves suggests a functional importance of this protein in chloroplasts.
Participation of etioplast CBP70 in cytokinin-dependent activation of transcription elongation in etioplasts and chloroplasts
Regulation of transcription by CBP70 isolated from etioplasts of etiolated maize seedlings was studied in the lysates of etioplasts from the same seedlings. In this transcription system, only elongation of transcripts initiated in vivo occurred. In the presence of tZ, etioplast CBP70 activated transcription in etioplast lysates (Table 4). CBP70 alone or tZ alone had no effect. Thus, CBP70 mediated transcription activation by cytokinin in the etioplast transcription elongation system. This allows etioplast CBP70 to be considered as a cytokinin-dependent transcription elongation factor or as a protein inducing a cytokinin-dependent modulation of the elongation factor activity.
The differences in the composition of polypeptides in etioplasts and chloroplasts include some differences in their transcription systems (Zychlinski et al., 2005). Despite the structural similarity, RNA polymerases of bacterial type and sigma-like transcription factors (SLF) of etioplasts and chloroplasts differ functionally, as reflected in the the use of different promoters and in the different strength of core RNA polymerase-SLF associations (Tiller and Link, 1993). Using protein kinase and protein phosphatase, these authors demonstrated that differences in the properties of chloroplast and etioplast transcription systems depended on the degree of RNA polymerase and SLF phosphorylation, although specific features of protein phosphorylation in the etioplast and chloroplast transcription systems were not studied. Keeping in mind the above differences in the transcription systems of etioplasts and chloroplasts, in the present work the functional activity of etioplast CBP70 was studied not only in maize etioplasts but also in barley chloroplast lysates. The results presented in Table 5 demonstrate that etioplast CBP70 induced cytokinin-dependent activation of transcription elongation in the transcription system in barley chloroplasts. Thus, differences in the properties of the transcription systems from chloroplasts and etioplasts did not interfere with the etioplast CBP70 cytokinin-dependent activation of transcription elongation in the chloroplast system. This suggests that CBP70 is involved in the control of transcription in all types of plastids.
Activity of maize etioplast CBP70 in the regulation of transcription elongation in the chloroplast transcription system of barley demonstrated that plastid CBP70 did not manifest species-specificity in the control of transcription, at least in related species, such as maize and barley.
Recent findings indicated that the control of transcript elongation is a very important step for the regulation of gene expression in eukaryotes (Björklund et al., 1999; Lis, 2007; Mavrich et al., 2008). Activation of several hundred nuclear genes involved in the control of development and responses to environmental signals occurs on the level of transcription elongation (Aiyar et al., 2004; Muse et al., 2007; Zeitlinger et al., 2007). Numerous nuclear eukaryotic elongation factors activating or repressing transcript elongation by RNA polymerases II and I were identified (Björklund et al., 1999; Yamaguchi et al., 2002; Narita et al., 2003; Aiyar et al., 2004; Yik et al., 2005; Komori et al., 2009; Zhang et al., 2009).
The regulation of transcription elongation in chloroplasts is poorly studied. Several sigma-like transcription factors regulating chloroplast transcription initiation were identified and studied thoroughly (Lysenko, 2007), but only a single candidate for the elongation factor of chloroplast transcription, the zinc ribbon ET1 protein of maize chloroplasts, was described (da Costa e Silva et al., 2004). ET1 possesses striking structural similarity to the eukaryotic transcription elongation factor TFIIS regulating RNA polymerase II in nuclei. The elongation in vitro of all transcripts initiated in vivo in chloroplasts of the et1 maize mutant was significantly reduced compared with chloroplasts of wild-type plants, suggesting that the ET1 protein is a chloroplast transcription elongation factor similar to the nuclear elongation factor TFIIS (da Costa e Silva et al., 2004). The et1 mutation, affecting both amyloplast and chloroplast development, suggests that ET1 protein, as a regulator of transcript elongation, might be involved in the essential processes common to the development of chloroplasts and amyloplasts (da Costa e Silva et al., 2004).
The precise molecular mechanism of ET1 action has not been elucidated so far. The authors (da Costa e Silva et al., 2004) indicate that it is necessary to study the interaction of this factor with other proteins required for its functional activity. At present, it is known that nuclear transcription factors function in the complex with other regulatory proteins, which activate or inhibit these factors (Yamaguchi et al., 2002; Fath et al., 2004; Yik et al., 2005; Komori et al., 2009). It might be that CBP70 activates transcript elongation in plastids via its interaction with ET1, although it is not excluded that CBP70 itself could be a factor of transcription elongation. From previous work, it was known that CBP70 is involved in cytokinin-dependent activation of nuclear transcription elongation (Brovko et al., 2007). As already mentioned, chloroplast elongation factor ET1 displays a structural homology with nuclear elongation factor TFIIS. It might be that CBP70 fulfils its function via its interaction with ET1 in plastids and with TFIIS in the nucleus. The gene encoding TFIIS was recently found in the Arabidopsis genome, and nuclear localization of TFIIS protein was established (Grasser et al., 2009). It was demonstrated that TFIIS plays a crucial role in Arabidopsis seed development (Grasser et al., 2009). Another Arabidopsis transcript elongation factor FACT affected plant vegetative and reproductive development (Lolas et al., 2009). These data emphasize the important role of transcript elongation factors in the regulation of plant life.
The results of this and previous (Brovko et al., 2007) studies demonstrated that plastid and nuclear CBP70 have similar functions, the same molecular mass (Fig. 1C), and common epitopes recognized by the same mAbs (Fig. 1C). These data suggest that nuclear and plastid CBP70 could be one and the same protein. At the same time, it can not be excluded that these proteins have some differences in their primary structures or modifications.
When discussing CBP70 localization and functional activity in nuclei and plastids, it is essential to consider the recent information on human estrogen receptor (ER) (Yager and Chen, 2007). Since it has long been known that this receptor is a nuclear estrogen-dependent trans-factor, but recently ER was also found in mitochondria, where it is represented by the same polypeptides as in the nucleus and encoded by the same genes (Pedram et al., 2006a, b). Mitochondrial ER was shown to participate in the regulation of mitochondrial gene expression (Psarra and Sekeris, 2009). The presence of ER in various human-cell compartments, for example, in the nucleus and mitochondria, corresponds to the present data about CBP70 presence in the nuclei and plastids of plant cells, where the protein participated in the regulation of transcription. It is especially attractive to study CBP70 localization and function in mitochondria, the third plant cell compartment possessing its own genome, the more so that cytokinin-binding proteins were found in mitochondria (Brinegar et al., 1992).
In conclusion, CBP70 localization in amyloplasts and etioplasts of etiolated maize seedlings and in chloroplasts of green maize plants was demonstrated for the first time. Cytokinin-dependent activation of plastid transcript elongation in vitro by etioplast CBP70 was established. These data suggest that CBP70 isolated from etioplasts is a cytokinin-dependent plastid transcript elongation factor or a protein regulating such factor, and this might be essential for cytokinin control of chloroplast and etioplast biogenesis.
Abbreviations
- CBP
cytokinin-binding protein
- mAb
monoclonal antibody
- NBT
nitroblue tetrazolium
- PBST
phosphate-buffered saline containing Tween 20
- PBS
phosphate-buffered saline
- PMSF
phenylmethylsulphonyl fluoride
- tZ
trans-zeatin
- TBST
TRIS-buffered saline containing Tween 20
We dedicate this work to the 80th anniversary of Professor Igor S Kulaev (Moscow State University, Russia). We thank Professors Nella L Klyachko and Vasily M Studitsky for critical reading and helpful discussion of the manuscript, and Victoria Yu Shtratnikova for technical help with the manuscript preparation.This work was partly supported by the Russian Foundation for Basic Research, projects nos 08-04-00739 and 10-04-00594.
Comments