-
PDF
- Split View
-
Views
-
Cite
Cite
Hao Shen, Lan Hong, Wanhui Ye, Honglin Cao, Zhangming Wang, The influence of the holoparasitic plant Cuscuta campestris on the growth and photosynthesis of its host Mikania micrantha, Journal of Experimental Botany, Volume 58, Issue 11, August 2007, Pages 2929–2937, https://doi.org/10.1093/jxb/erm168
- Share Icon Share
Abstract
The influence of the holoparasite Cuscuta campestris Yuncker on the growth and photosynthesis of Mikania micrantha H.B.K. was studied. The results indicate that C. campestris infection significantly reduced the light use efficiency and light saturation point of the host. It significantly reduced the net photosynthetic rate (Pn) of the 1st and 8th mature leaves of M. micrantha at light saturation point, the apparent quantum yield of the 1st mature leaves, the carboxylation efficiency and CO2 saturated Pn of the 8th mature leaves, but increased the light compensation point of the 1st mature leaves. Diurnally, it significantly reduced Pn between 08.00 h and 16.00 h and stomatal conductance and transpiration from 10.00 h to 16.00 h for the 8th mature leaves. Moreover, the significantly adverse effects of C. campestris infection on Pn were observed 18 d after parasitization (DAP) for the 4th, 8th and 12th, and 25 DAP for the 1st mature leaves of M. micrantha, and they became greater with infection time. The infection also significantly reduced the number of leaves, leaf area, stem length, and biomass, and prevented flowering of M. micrantha in the growing season, and caused almost complete death of the aerial parts of the host about 70 DAP, but the uninfected plants grew and developed normally. Furthermore, the total biomass of the infected host and the parasite was significantly less than that of the uninfected plants. Therefore, besides resource capture by C. campestris, the reduced growth of the infected plants must also be due to the negative effects of the parasite on host photosynthesis.
Introduction
Mikania micrantha H.B.K. is a fast-growing climbing perennial weed of the family Asteraceae native to Central and South America (Wirjahar, 1976; Holm et al., 1977). At present, the weed has been listed as one of the 100 worst invasive alien species in the world (Lowe et al., 2001), and it is one of the worst exotic weeds in south-east and south Asia (Zhang et al., 2004). In recent years, M. micrantha has caused severe damage to many ecosystems and has led to great economic loss in Guangdong Province of China. It has been listed as one of the 16 most invasive species in China by the State Environmental Protection Administration of China (Zhang et al., 2004). Accordingly, there is an urgent need to develop effective methods to control this notorious weed.
With the aim of biologically controlling M. micrantha in South China, the influence of the holoparasite Cuscuta campestris Yuncker on its growth was examined (Shen et al., 2005). In this study it was found that C. campestris significantly reduced the total biomass, changed the biomass allocation patterns, and completely inhibited the flowering of the infected M. micrantha plants. The result indicated that using C. campestris to control M. micrantha is effective. However, how C. campestris influences the growth of M. micrantha is unknown.
Cuscuta (Convolvulaceae) is an obligate angiosperm parasite which attaches itself to the above-ground parts of a wide range of hosts (Parker and Riches, 1993; Dawson et al., 1994). Cuscuta campestris is the most widespread species in this genus in the world (Dawson et al., 1994). It is holoparasitic and therefore obtains its resources entirely from its host plants in order to grow and complete its life cycle, thus severely suppressing them and even resulting in their death (Dinelli et al., 1993; Dawson et al., 1994).
Among the species in Cuscuta, the impact of C. reflexa on host biomass, photosynthesis, and source–sink relations has been studied extensively (Press et al., 1999). In the presence of C. reflexa, the growth and increase in dry matter of the host plants was severely inhibited, but the total biomass of host plus parasite was almost the same as that of uninfected plants (Jeschke and Hilpert, 1997; Jeschke et al., 1997). This appeared to be caused by the powerful sink of C. reflexa leading to a sink-dependent stimulation of photosynthesis in host plants. However, it has been observed that total dry mass of C. campestris plus host was less than that of uninfected M. micrantha (Shen et al., 2005). Thus, in addition to direct resource capture from M. micrantha for its growth and reproduction, C. campestris might suppress the photosynthesis of the infected M. micrantha plants. Inhibition of host photosynthesis is well known to occur in Striga (Hibberd et al., 1996b; Frost et al., 1997) and Rhinanthus–host associations (Cameron et al., 2005). Therefore, this project was initiated to study if and how C. campestris might influence the photosynthesis of M. micrantha. In addition, growth data were also collected to examine the relationship between photosynthesis and growth of M. micrantha in response to C. campestris infection.
Materials and methods
Experimental materials and design
The experiment was carried out during the May–December 2004 growing season at the same site as in the previous study by Shen et al. (2005). The field station (23°8′N, 113°17′E, elevation 8 m a.s.l.) is in Guangzhou, Guangdong Province, China. The region is characterized by a typical south subtropical monsoon climate. On 31 May 2004, whole M. micrantha plants from a M. micrantha population were collected in the suburb of Dongguan, Guangdong Province, China. Two-node segments, similar in size, were selected from the middle of the stems. Pots (50 cm in height, 50 cm in upper diameter, and 45 cm in lower diameter) were filled with a mixture of pool mud and paddy field clay (1:2 in volume), and three segments were planted in each pot with the lower node buried below and the upper one about 5 cm above the soil surface. The upper nodes began to sprout 3 d later. When the plants were about 350 cm high on 23 August, they were thinned to one per pot and 300 individuals, similar in height and stem diameter, were selected and placed at random in an open field uniform in environmental conditions. Half of them were randomly chosen to be inoculated with C. campestris (infected group), leaving the rest uninfected (control group). To prevent M. micrantha from climbing from one pot to another, pots were separated at least 1 m from each other and a bamboo cane about 5 m long was placed vertically in each pot for M. micrantha to climb on.
Cuscuta campestris infection was carried out using seeds that were collected from the same M. micrantha population as in Shen et al. (2005) and stored at room temperature. On 12 August, the seeds were sown in pots with sand at a depth of ∼1 cm. Three days later, the seeds began to germinate and completed germination on 20 August. On 26 August, when M. micrantha plants were ∼370 cm in height and the mean number of leaves was 450, while C. campestris seedlings were ∼5 cm in height, one C. campestris seedling was placed, together with wet sand, on the soil surface of each M. micrantha pot in the infected group. By 29 August, all the M. micrantha plants in this group had become infected with C. campestris stems. The experiment ended on 27 December, 120 d after parasitization (DAP) or 210 d after planting, when the uninfected M. micrantha plants had started to wither. During the experiment, the pots were weeded when necessary and watered twice daily with tap water at 06.00 h and 18.00 h, except on rainy days.
Growth measurements
The dates of initial flowering of M. micrantha and C. campestris, and the dates of almost complete death of the aerial parts of the infected plants were recorded. On days 0, 10, 20, 30, 40, 50, 60, 70, 80, 100, and 120 after parasitization, eight uninfected and eight infected M. micrantha plants were selected at random and harvested. At each harvest, all the dead materials of both M. micrantha and C. campestris were carefully removed and weighed, while living parts were separated and handled as follows. Mikania micrantha plants were separated into stems, leaves, reproductive organs when there were any, and underground roots. Roots were soaked in tap water, washed, and separated carefully in running water over a 2-mm-mesh sieve. Stems, tendrils, and reproductive organs of C. campestris were carefully dissected from M. micrantha stems and leaves. Mikania micrantha stem length was measured, the number of leaves was counted, and leaf area was measured using a CI-203 portable laser area meter. The dry leaves which were removed from the plants were also counted, but only the number of living leaves was used to address the growth status of the infected and control M. micrantha plants. Plant materials were then oven dried at 70 °C until constant weight was achieved.
Measurements of photosynthesis
In situ measurements of photosynthesis were made on leaves of M. micrantha plants using an LI-6400 portable photosynthesis system with a standard 6 cm2 leaf chamber (LI-COR Inc., Lincoln, NE, USA) on clear days. Net photosynthetic rate (Pn), stomatal conductance (gs), rate of transpiration (E, mmol H2O m−2 s−1), intercellular CO2 concentration (Ci), air temperature (Ta), leaf temperature (Tl), ambient CO2 concentration (Ca), air relative humidity (RH), and photosynthetic photon flux density (PPFD) parameters were recorded, and photosynthetic parameters were calculated as described by von Caemmerer and Farquhar (1981). Except for diurnal gas exchange measurements for which natural conditions were allowed, conditions inside the leaf chamber during gas exchange measurements were controlled as follows. Irradiance was provided by an integrated red-blue light-emitting diode source (model 6400-02B, LI-COR, Inc.) at 1000 μmol m−2 s−1 except for the light response study. Chamber CO2 concentration (Ca) was controlled with a CO2 mixer at 360 μmol mol−1 except for the Pn/Ci study. The leaf chamber had a flow rate of 500 mol s−1, and leaf temperature was controlled at 30 °C. Photosynthesis data were recorded after equilibration to a steady state (coefficient of variation ≤1%) at each measurement. To ensure that the leaves measured were of similar age and developmental stage, only fully expanded mature sun leaves at the same order positions on the main stems were sampled. At each measurement, one leaf per plant and 6–10 randomly selected M. micrantha plants per treatment were sampled.
Light response curves
To construct the light response curves, on 46–47 DAP (14–15 October), photosynthesis measurements were made between 08.00 h and 11.00 h on the 1st and 8th fully expanded leaves of each plant. Light was provided by the light-emitting diode. To avoid photoinhibition, each leaf in the leaf chamber was acclimated to a PPFD of 600 μmol photons m−2 s−1 for 1–3 min and then to 1000 μmol photons m−2 s−1 for 1–3 min prior to gas exchange measurements. Then, photosynthesis measurements were taken at PPFD in the following order: 2000, 1800, 1500, 1200, 1000, 800, 600, 400, 200, 100, 50, 20, 0 μmol photons m−2 s−1.

Ci response curves

Pn of M. micranthaleaves at different leaf positions on different DAP
The Pn of the 1st, 4th, 8th, and 12th fully expanded mature leaves of M. micrantha plants was measured at around 10.00 h on days 7, 18, 25, 45, and 65 after parasitization. After 65 DAP, there were not enough leaves at all these order positions due to withering that started in the infected plants on 70 DAP. Thus, 65 DAP was the last day Pn was measured.
Diurnal variation patterns of gas exchange
The diurnal changes in leaf gas exchange were measured on the 8th fully expanded leaves of M. micrantha on 16 October (48 DAP). Six to ten leaves per infection treatment, one per plant, were measured repeatedly at approximately hourly intervals from 08.00 to 17.00 h. Water use efficiency (WUE, μmol CO2 mmol H2O−1) was calculated as Pn/E for each measurement.
Statistical analysis
All tests were carried out at α=0.05 level using SPSS (version 11.5, SPSS Inc., Chicago, IL, USA). Means of growth and photosynthesis variables between infection treatments were compared using Student's t-test. One-way analysis of variance (ANOVA) was performed for total biomass of the infected plants, controls, and the infected system (host plus parasite). Means of the significant ANOVA effects were compared using Tukey post hoc comparisons.
Results
Growth
During the experiment, the uninfected M. micrantha plants grew vigorously and developed normally, but the infected ones did not. On 16 October, 108 DAP, the uninfected M. micrantha plants started to develop terminal inflorescences, then 10 d later, they were in full bloom, and fruited on 5 November. However, the infected plants did not flower throughout the experiment.
Number of leaves, leaf area, and dry weights of the uninfected M. micrantha plants had the same variation pattern, and those of the infected followed a different variation pattern over time after parasitization (Fig. 1a–c). For the control, they increased from the beginning to the maximum between about 90 DAP and 100 DAP, and then decreased sharply to the end of the experiment due to senescence. For the infected, they decreased from the beginning over time to the minimum between about 70 DAP and 80 DAP, and then increased gradually to the end of the experiment. Compared with the control, C. campestris infection significantly reduced the number of leaves, leaf areas, and dry weights of M. micrantha plants from 20 DAP to the end of the experiment (Fig. 1a–c). Moreover, the sum of the dry mass of the infected system (host plus parasite) was significantly less than that of the uninfected M. micrantha from 20 DAP onwards (P <0.05; data not shown). Stem length of the control M. micrantha plants generally increased, and it varied much less over the experiment period than that of the infected plants (Fig. 1d). For the infected plants, it changed slightly from the beginning to about 60 DAP, then it decreased sharply to a minimum between about 70 DAP and 80 DAP due to the dying off of the upper parts of the host shoots (arrow in Fig. 1d). Compared with the control, C. campestris infection significantly reduced the stem elongation of the infected M. micrantha plants from 30 DAP to the end of the experiment (Fig. 1d). When Pn measurements for light response curves, Ci response curves, and diurnal variation patterns were taken from 46 DAP to 50 DAP, the uninfected and infected M. micrantha plants had approximately 815 leaves and 99 leaves and their mean stem lengths were 537 cm and 432 cm, respectively.
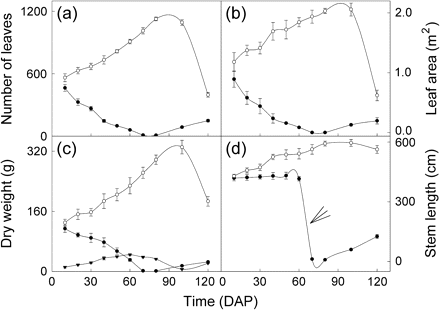
Means (±SE, n=8) of number of leaves (a), leaf area (b), dry weight of living tissues (c), and stem length (d) on different days after parasitization (DAP). Open circles, uninfected M. micrantha plants; closed circles, M. micrantha plants infected by C. campestris; closed triangles, C. campestris. The arrow in (d) indicates the sharp decrease in stem length in the infected from 60 DAP to 70 DAP due to the dying off of the upper parts of the host shoots.
The effect of the parasite on the M. micrantha host could be divided into two phases. In the first phase, from 20 DAP to 80 DAP, the effect enhanced with time. In this period, dry weight of the living tissues of the infected M. micrantha plants decreased from 64% to only 0.4% of the uninfected controls (Fig. 1c). From 10 DAP to 60 DAP, C. campestris grew vigorously with a lot of branching, and its total biomass increased from 11.5 g on 10 DAP to 44.6 g on 60 DAP (Fig. 1c). Then, from 60 DAP to 80 DAP, concomitant with the decrease in the host biomass, the living biomass (including fruit) of the parasite also decreased. More interesting, from 60 DAP to 80 DAP, the dry weight of the parasite was significantly greater than that of the host. Cuscuta campestris started flowering at 20 DAP and was in full bloom 14 d later. From 40 DAP to 80 DAP, C. campestris flowered and fruited vigorously with about 63.7–88.3% of total biomass allocated to its fruit. Fruiting was complete just before the above-ground parts of the host started to die. The aerial parts of the infected M. micrantha plants started to wither 70 DAP and then all died about 15 d later, but the parts up to the 1st node from the base of the stem remained alive.
In the second, recovery phase, from 80 DAP to 120 DAP, new sprouts emerged from the first node at the base of the stem of the infected M. micrantha plants and grew. By 100 DAP, the biomass of the infected host increased from 1.3 g at 80 DAP to 15.0 g. Furthermore, with the re-growth of the infected plants during this time, the remnant C. campestris also re-grew from 5.8 g at 100 DAP to 21.8 g at 120 DAP (Fig. 1c).
Photosynthesis in response to light
Light response curves of photosynthesis of the 1st and 8th fully expanded mature leaves of M. micrantha plants are given in Fig. 2, and some of the photosynthesis parameter estimates are summarized in Table 1. Pn of leaves from both infection treatments and position order increased with the increase of PPFD until light saturation and decreased with increasing PPFD above the light saturation point (Fig. 2). At PPFD <600 μmol photons m−2 s−1, Pn of the 8th fully expanded leaves was not significantly different between uninfected and infected M. micrantha plants, but it was at PPFD ≥600 μmol photons m−2 s−1; such a critical PPFD was 200 μmol photons m−2 s−1 for the 1st fully expanded leaves (Fig. 2).
Mean photosynthesis parameter estimates from the light response curves for the 1st and the 8th fully expanded mature leaves of uninfected and infected Mikania micranthaby Cuscuta campestris
Parameter estimates of photosynthesis | First fully expanded mature leaves | Eighth fully expanded mature leaves | ||
Uninfected | Infected | Uninfected | Infected | |
LSP (μmol photons m−2 s−1) | 985 | 575***a | 939 | 589*** |
Pmax (μmol CO2 m−2 s−1) | 18.1 | 9.42*** | 12.37 | 8.95*** |
LCP (μmol photons m−2 s−1) | 22.1 | 28.3* | 28.2 | 30.2 |
Φ (mol CO2 mol−1 photons) | 0.061 | 0.048** | 0.050 | 0.046 |
Rd (μmol m−2 s−1) | 1.35 | 1.36 | 1.41 | 1.39 |
Parameter estimates of photosynthesis | First fully expanded mature leaves | Eighth fully expanded mature leaves | ||
Uninfected | Infected | Uninfected | Infected | |
LSP (μmol photons m−2 s−1) | 985 | 575***a | 939 | 589*** |
Pmax (μmol CO2 m−2 s−1) | 18.1 | 9.42*** | 12.37 | 8.95*** |
LCP (μmol photons m−2 s−1) | 22.1 | 28.3* | 28.2 | 30.2 |
Φ (mol CO2 mol−1 photons) | 0.061 | 0.048** | 0.050 | 0.046 |
Rd (μmol m−2 s−1) | 1.35 | 1.36 | 1.41 | 1.39 |
Means were compared between treatments within each leaf order position. LSP, light saturation point; Pmax, net photosynthesis at LSP; LCP, light compensation point; Φ, apparent quantum yield; Rd, dark respiration rate.
*P <0.05;**P <0.01;***P <0.001.
Mean photosynthesis parameter estimates from the light response curves for the 1st and the 8th fully expanded mature leaves of uninfected and infected Mikania micranthaby Cuscuta campestris
Parameter estimates of photosynthesis | First fully expanded mature leaves | Eighth fully expanded mature leaves | ||
Uninfected | Infected | Uninfected | Infected | |
LSP (μmol photons m−2 s−1) | 985 | 575***a | 939 | 589*** |
Pmax (μmol CO2 m−2 s−1) | 18.1 | 9.42*** | 12.37 | 8.95*** |
LCP (μmol photons m−2 s−1) | 22.1 | 28.3* | 28.2 | 30.2 |
Φ (mol CO2 mol−1 photons) | 0.061 | 0.048** | 0.050 | 0.046 |
Rd (μmol m−2 s−1) | 1.35 | 1.36 | 1.41 | 1.39 |
Parameter estimates of photosynthesis | First fully expanded mature leaves | Eighth fully expanded mature leaves | ||
Uninfected | Infected | Uninfected | Infected | |
LSP (μmol photons m−2 s−1) | 985 | 575***a | 939 | 589*** |
Pmax (μmol CO2 m−2 s−1) | 18.1 | 9.42*** | 12.37 | 8.95*** |
LCP (μmol photons m−2 s−1) | 22.1 | 28.3* | 28.2 | 30.2 |
Φ (mol CO2 mol−1 photons) | 0.061 | 0.048** | 0.050 | 0.046 |
Rd (μmol m−2 s−1) | 1.35 | 1.36 | 1.41 | 1.39 |
Means were compared between treatments within each leaf order position. LSP, light saturation point; Pmax, net photosynthesis at LSP; LCP, light compensation point; Φ, apparent quantum yield; Rd, dark respiration rate.
*P <0.05;**P <0.01;***P <0.001.
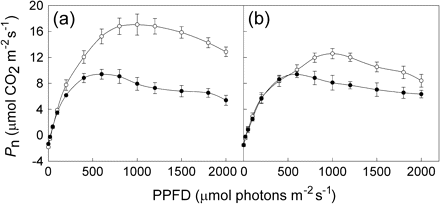
Mean net photosynthetic rates (Pn, mean ±SE, n=6) at different photosynthetic photon flux densities (PPFD) for the 1st (a) and 8th (b) fully expanded mature leaves of M. micrantha plants. Open circles, uninfected plants; closed circles, M. micrantha plants infected by C. campestris.
Dark respiration rates (Rd) were not significantly different between the two infection treatments for each leaf order position (Table 1). However, the 1st fully expanded mature leaves of the uninfected M. micrantha plants had significantly higher LSP, Pmax, and Φ, and lower LCP than those of the infected. This relationship was also true for LSP and Pmax for the 8th fully expanded mature leaves.
Photosynthesis in response to Ci
Infection with C. campestris had a significant effect on the Pn/Ci response of the 8th fully expanded mature leaves of M. micrantha plants (Fig. 3). Leaves of uninfected M. micrantha plants had remarkably higher CE and CO2 saturated rate of photosynthesis (Psat) than those of the infected plants. Leaves of uninfected and infected M. micrantha with C. campestris had the same CO2 saturation point at about 800 μmol mol−1.
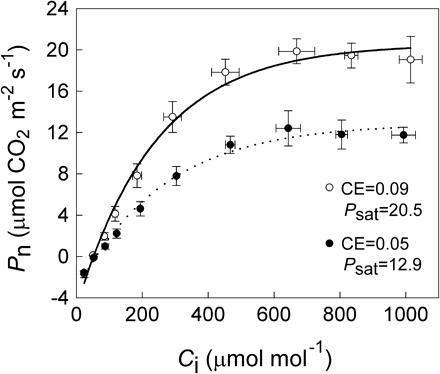
Response of net photosynthetic CO2 assimilation (Pn) to intercellular CO2 concentration (Ci) in the 8th fully expanded mature leaves of the uninfected (open circles) and infected (closed circles) M. micrantha plants by C. campestris. Data points are means ±SE, n=6.
Pn of M. micrantha leaves at different leaf order positions on different DAP
Fully expanded mature leaves with the same order position on the stems of uninfected control M. micrantha plants did not vary significantly in Pn on different measurement days after parasitization, and Pn of leaves of the infected plants decreased with DAP (Fig. 4). The mean Pn of different measurement days for the 1st, 4th, 8th, and 12th leaf order positions were 18.16, 16.38, 13.83, and 11.00 μmol CO2 m−2 s−1 for the uninfected and 11.23, 9.52, 8.44, and 6.23 μmol CO2 m−2 s−1 for the infected M. micrantha plants, respectively. The Pn of leaves at each leaf order position were not significantly different between the control and the infected M. micrantha plants at 7 DAP. Significant differences were observed from 18 DAP for the 4th, 8th, and 12th leaf order position leaves, and from 25 DAP for the 1st order position leaves. Differences of Pn between the infection treatments increased with infection time, and the largest differences occurred at 65 DAP (Fig. 4, Table 2). Generally, the effects of leaf order on Pn varied with DAP. For each leaf order position, the adverse effects of infection on Pn increased with DAP (Table 2). The Pn rates of the 1st, 4th, 8th, and 12th fully expanded mature leaves in the infected plants at 65 DAP were only 15.4%, 10.9%, 9.7%, and 3.1% of those at 7 DAP, respectively.
Mean net photosynthetic rate (Pn) ratios of the 1st, 4th, 8th, and 12th fully expanded mature leaves of infected Mikania micrantha plants by Cuscuta campestris between 7 d and 65 d after parasitization (DAP)
Time (DAP) | Pn ratios (infected/control) at different leaf order positions | |||
1st | 4th | 8th | 12th | |
7 | 94.2 | 96.9 | 91.4 | 102.3 |
18 | 87.8 | 70.5 | 77.8 | 62.7 |
25 | 51.8 | 57.2 | 56.2 | 65.0 |
45 | 50.6 | 49.1 | 58.7 | 39.4 |
65 | 17.3 | 11.7 | 10.9 | 3.7 |
Time (DAP) | Pn ratios (infected/control) at different leaf order positions | |||
1st | 4th | 8th | 12th | |
7 | 94.2 | 96.9 | 91.4 | 102.3 |
18 | 87.8 | 70.5 | 77.8 | 62.7 |
25 | 51.8 | 57.2 | 56.2 | 65.0 |
45 | 50.6 | 49.1 | 58.7 | 39.4 |
65 | 17.3 | 11.7 | 10.9 | 3.7 |
Mean Pn ratios of infected and control plants are presented.
Mean net photosynthetic rate (Pn) ratios of the 1st, 4th, 8th, and 12th fully expanded mature leaves of infected Mikania micrantha plants by Cuscuta campestris between 7 d and 65 d after parasitization (DAP)
Time (DAP) | Pn ratios (infected/control) at different leaf order positions | |||
1st | 4th | 8th | 12th | |
7 | 94.2 | 96.9 | 91.4 | 102.3 |
18 | 87.8 | 70.5 | 77.8 | 62.7 |
25 | 51.8 | 57.2 | 56.2 | 65.0 |
45 | 50.6 | 49.1 | 58.7 | 39.4 |
65 | 17.3 | 11.7 | 10.9 | 3.7 |
Time (DAP) | Pn ratios (infected/control) at different leaf order positions | |||
1st | 4th | 8th | 12th | |
7 | 94.2 | 96.9 | 91.4 | 102.3 |
18 | 87.8 | 70.5 | 77.8 | 62.7 |
25 | 51.8 | 57.2 | 56.2 | 65.0 |
45 | 50.6 | 49.1 | 58.7 | 39.4 |
65 | 17.3 | 11.7 | 10.9 | 3.7 |
Mean Pn ratios of infected and control plants are presented.
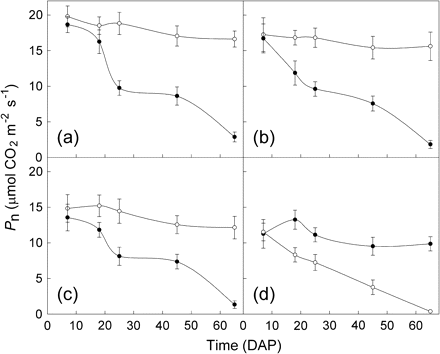
Mean (±SE, n=8) of the net photosynthetic rates (Pn) of leaves from different leaf order positions of uninfected (open circles) and infected (closed circles) M. micrantha plants by C. campestris between 7 d and 65 d after parasitization (DAP). (a), (b), (c), and (d) are for the 1st, 4th, 8th, and 12th fully expanded mature leaves, respectively.
Diurnal variation patterns of microclimate and leaf gas exchange parameters
The variations in ambient air temperature (Ta), PPFD, and RH in the experimental site were recorded on 16 October (Fig. 5a). PPFD increased from 08.00 h to the maximum at midday (about 12.00 h), while it decreased to the minimum at 17.00 h. Ta and RH changed faster from 08.00 h to 11.00 h and from 16.00 h to 17.00 h than between 11.00 h and 16.00 h. Ca changed between 370.7 and 378.9 μmol mol−1 during the day.
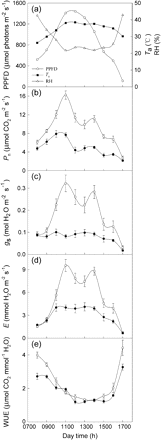
Diurnal changes in photosynthetic photon flux density (PPFD), air temperature (Ta), and relative humidity (RH) at the experimental site (a) and diurnal variations of net photosynthetic rate (Pn) (b), stomatal conductance (gs) (c), transpiration rate (E) (d), and water use efficiency (WUE) (e) of leaves of uninfected (open circles) and infected (closed circles) M. micrantha by C. campestris.
The diurnal variations of Pn, gs, E, and WUE of the 8th fully expanded mature M. micrantha leaves measured on 16 October are given in Fig. 5b–e. Pn, gs, and E of M. micrantha leaves had a similar bimodal diurnal pattern regardless of the infection treatments, although the pattern was weak for gs and E of the infected plants (Fig. 5c, d). The two peaks of Pn, gs, and E of the leaves of the uninfected were at 11.00 h and 14.00 h, while those of the infected were at 10.00 h and 14.00 h for Pn and 13.00 h for gs and E. Pn of the control was significantly higher than that of the infected plant at each measurement time, except at 17.00 h, and similar results were observed for gs and E from 10.00 h to 16.00 h. The variation coefficients of Pn, gs, and E of the uninfected leaves over the measurement times were 41.1%, 52.2%, and 59.1%, respectively. They were significantly (P <0.05) higher than the corresponding values of 37.3%, 31.1%, and 42.7%, respectively, for the infected leaves, except for Pn. WUE of the control was significantly greater than that of the infected at measurement times before 10.00 h and after 16.00 h and showed no significant difference between 10.00 h and 16.00 h (Fig. 5e).
Discussion
The present study shows that leaves of uninfected M. micrantha plants had a significantly higher capacity for photosynthesis than those infected by C. campestris. Mature leaves of the infected M. micrantha plants had significantly lower Pn than the uninfected plants from the 1st to the 12th leaf order positions on the stems up to 65 d after infection, except at the beginning. Diurnal photosynthesis measurements indicate that C. campestris infection significantly reduced photosynthesis of the 8th fully expanded mature M. micrantha leaves at all the measurement times except at 17.00 h on the day when light was low. The significant effect of C. campestris infection on the photosynthesis of M. micrantha leaves may be partly caused by the leaves of the uninfected plants using light more efficiently than the infected ones as it was observed that the infection significantly reduced LSP and Pmax of the 1st and 8th fully expanded leaves, and reduced the Φ and increased the LCP of the 1st fully expanded leaves. It might also be due to the fact that the infection significantly reduced CE in the present study.
Cuscuta campestris infection negatively influenced photosynthesis more on young M. micrantha leaves than on the old in response to light. The infection reduced Pmax by 48% in the 1st fully expanded mature leaves and 28% in the 8th. Moreover, the uninfected 1st fully expanded mature leaves had significantly higher Φ and lower LCP than the infected, and it did not happen in the 8th. Photosynthesis of the 1st fully expanded mature leaves of the uninfected plants became significantly higher than that of the infected plants at PPFD ≥200 μmol photons m−2 s−1, and this occurred at PPFD ≥600 μmol photons m−2 s−1 for the 8th leaves. It is possible that the photosynthesis of the young mature M. micrantha leaves is more sensitive to infection than the old in response to light conditions. Results of this study also indicate that for each leaf order position, the negative effects of infection on photosynthesis increased as infection time increased. Reduced host photosynthesis has also been reported in root parasite associations of the hemiparasitic Striga hermonthica (Cechin and Press, 1993; Frost et al., 1997) and S. gesnerioides (Hibberd et al., 1996b) and the holoparasitic Orobanche aegyptiaca and O. cernua (Barker et al., 1995; Hibberd et al., 1996a).
Opposite effects of Cuscuta infection on host photosynthesis have been reported in the associations of C. reflexa and its leguminous or non-leguminous hosts under light-saturating conditions (Jeschke et al., 1994a, 1995, 1997; Jeschke and Hilpert, 1997). In these cases, the enhanced photosynthesis of the host seemed to compensate for the resource loss to the parasites. Parasite-related stimulation of photosynthesis has also been found in the dwarf mistletoe Arceuthobium (Clark and Bonga, 1970), uninfected leaves of rust-infected plants (Murray and Walters, 1992), the association Striga–Sorghum during early stages of parasitism (Cechin and Press, 1993), and in a field study for the Striga–Sorghum association (Clark et al., 1994). However, in the Orobanche cernua–tobacco parasitic association, the parasite had no effect on photosynthesis of the youngest fully expanded leaves (Hibberd et al., 1998). It seems there is not a common mode of how a parasite affects host photosynthesis.
A difference in CO2 saturation point between infected and uninfected M. micrantha leaves was not detected in this study. However, C. campestris infection had significant negative effects on CE and CO2 saturated rate of photosynthesis on the host plants. The response is similar to that of Oryza sativa infected by Striga hermonthica in the late stages of the association (Watling and Press, 2000). Additionally, infection with C. campestris decreased gs in the M. micrantha host plants, and Pn and gs followed the same diurnal variation pattern in this study. Thus, C. campestris appears to affect M. micrantha photosynthesis through both the adverse impact on gs and direct effects on photosynthetic metabolism of M. micrantha. This is different from that reported for sorghum in which the parasite appears to affect host photosynthesis largely through its impact on gs, rather than through direct effects on the photosynthetic metabolism of the host (Frost et al., 1997; Watling and Press, 1997).
It has been suggested that plant growth regulators, such as abscisic acid (ABA), may be involved in the regulation of parasitic associations (Drennan and El Hiweris, 1979). ABA has been shown to increase the root:shoot ratio, reduce stem growth (Quarrie, 1991; Trewavas and Jones, 1991) and gs (Quarrie, 1991; Taylor et al., 1996), and inhibit leaf expansion (Van Voikenburgfa and Davies, 1983). Its concentration was higher in Striga hermonthica-infected maize leaves (Taylor et al., 1996) and in the xylem sap of S. hermonthica-infected sorghum (Frost et al., 1997) in comparison to the corresponding uninfected controls. Increased ABA concentration resulted in reduced gs in host plants and hence more host solutes being diverted to the parasite (Taylor et al., 1996), thus leading to an inhibition in photosynthesis of the host. In the present study, the reduced gs in infected M. micrantha plants might also be caused by an increase in host ABA concentration, and then the reduced gs resulted in the suppression of photosynthesis in the host. Musselman (1980) also postulated that there may be a toxin or toxic compound secreted by the parasite into the host's vasculature that suppresses photosynthesis. However, the role of ABA and other plant growth regulators, and the probable toxic effects through involvement of toxins in this parasitic association, need further investigation.
Cuscuta campestris, in this study, significantly reduced the number of leaves, stem length, leaf area, and total biomass starting from 20 d to 30 d after infection and completely inhibited the flowering of the infected M. micrantha plants. It is consistent with the previous study by Shen et al. (2005). Both the studies indicate that C. campestris can effectively control vegetative and reproductive growth of M. micrantha plants after infection. This is attributable to the fact that C. campestris significantly reduces photosynthesis of the host as observed in this study and captures resources from M. micrantha host plants.
The reduced number of leaves is partly explained by C. campestris reducing new leaf initiation and/or accelerating premature leaf senescence and abscission of the infected M. micrantha plants. It would lead to reduced leaf area. Consequently, biomass growth of the infected M. micrantha plants was decreased by the combined influences of reduced number of leaves and leaf area, as well as lowered photosynthesis rates. However, it has been reported that the presence of the parasite C. reflexa caused a delay in leaf senescence in Ricinus communis (Jeschke and Hilpert, 1997) and in Coleus blumei (Jeschke et al., 1997) as C. reflexa induced a sink-dependent stimulation of net photosynthesis in host leaves in these studies.
A source–sink relationship has been proposed to explain the effects of parasites on the growth of their host (Press et al., 1999). Some studies have shown that the obligate parasite Cuscuta can form a strong sink to redirect the flow of host resources to itself (Wolswinkel, 1974; Jeschke et al., 1994a, b; Jeschke and Hilpert, 1997), and Cuscuta species alter host physiology by acting as a stronger sink for photosynthates than any host organs (Ihl et al., 1984; De Bock and Fer, 1992; Parker and Riches, 1993). In this study, the total biomass of C. campestris and the infected M. micrantha host was significantly less than that of the control 30 d after infection. It is consistent with the fact that the reduction in biomass accumulation in infected plants may be an order of magnitude greater than the biomass of the parasitic sink (Parker et al., 1984; Press, 1995; Hibberd et al., 1996b; Watling and Press, 1997). Thus, the source–sink relationship cannot explain the effects of C. campestris on its host M. micrantha. Accordingly, it is suggested that the effect of C. campestris on the growth of the infected M. micrantha is caused more by its influence on photosynthesis than by its capturing resources from the host.
It has been observed that Striga hermonthica reduced growth of its sorghum host largely through its impact on host CO2 assimilation by decreasing leaf areas and photosynthesis rates (Graves et al., 1989). In this study, both resource drain and the negative effects of the parasite on the host photosynthesis must have caused the reduction in host growth. Significant growth reduction might begin before a significant reduction in host photosynthesis. Thus, the reduction in host photosynthesis may result from some other primary effect of the parasite (associated with its drain on host resources) and hence is a secondary effect rather than a primary one. There is, of course, then a strong feed-back effect and the reduced photosynthesis enhances whatever the other primary effects of C. campestris have on the host. It is also probable that Cuscuta contributes <1% to its own photosynthesis product requirements (Jeschke et al., 1994a) and has high respiratory losses (Jeschke and Hilpert, 1997). Therefore, the parasites use resources less efficiently than their hosts so that the reduction in host biomass is greater than the gain in parasite (Matthies, 1995, 1996, 1997; Marvier, 1998; Matthies and Egli, 1999).
In summary, C. campestris infection significantly reduced Pn of the fully expanded mature leaves of the infected M. micrantha plants immediately after infection. The inhibitory effect of the infection on Pn of the leaves at different leaf positions of M. micrantha became greater with infection time. The decrease in Pn of the leaves of the infected M. micrantha plants is at least partly because the infected had lower light use efficiency and CE than the uninfected plants. Such decreased photosynthesis must have resulted in the reduced growth of the infected M. micrantha plants as observed in the number of leaves, stem elongation, leaf area, and total biomass in this study. The results indicate that C. campestris cannot only obtain its resource directly from M. micrantha for its growth and reproduction, but also it has marked effects on the photosynthesis of the infected M. micrantha plants and consequently on the host growth, which to our knowledge has not been reported in the association between Cuscuta species and their hosts. The results also indicate that the use of C. campestris is an effective way to control M. micrantha.
Abbreviations
- ABA
abscisic acid
- Ca
ambient CO2 concentration
- CE
carboxylation efficiency
- Ci
leaf internal CO2 concentration
- DAP
days after parasitization
- E
transpiration
- gs
stomatal conductance
- LCP
light compensation point
- LSP
light saturating point
- Pmax
net photosynthesis at saturating irradiances
- Pn
net photosynthetic rate
- PPFD
photosynthetic photon flux density
- Psat
CO2 saturated rate of photosynthesis
- Rd
dark respiration rate
- RH
relative humidity
- Ta
air temperature
- Tl
leaf temperature
- WUE
water use efficiency
- Φ
apparent quantum yield
We thank Chris Parker for his research guidance and constructive comments on the manuscript. This work was supported by the National Natural Science Foundation of China (30470345) and the Natural Science Foundation of Guangdong Province, China (021536).
Comments