-
PDF
- Split View
-
Views
-
Cite
Cite
Guo-hua Xu, Veronique Chague, Cathy Melamed-Bessudo, Yoram Kapulnik, Ajay Jain, Kashchandra G. Raghothama, Avraham A. Levy, Avner Silber, Functional characterization of LePT4: a phosphate transporter in tomato with mycorrhiza-enhanced expression, Journal of Experimental Botany, Volume 58, Issue 10, July 2007, Pages 2491–2501, https://doi.org/10.1093/jxb/erm096
- Share Icon Share
Abstract
Many plant roots acquire inorganic phosphate (Pi) from soils directly through the root–soil interface via high-affinity Pi transporters and/or through symbiotic associations between the cortical cells and arbuscular mycorrhizal fungi. In tomato, three phosphate transporters (LePT3, LePT4, and LePT5) are up-regulated upon colonization by arbuscular mycorrhizal fungi. In this study, the role of LePT4 in tomato is elucidated by molecular and physiological characterizations of a loss-of-function mutant lept4. In the absence of mycorrhizal infection and under solution-Pi concentrations (Cp) of 0.05 mM and 0.5 mM, the mutant exhibited severe Pi-deficiency symptoms which were associated with significantly lower Pi uptake as compared with that of the wild type. However, at a Cp of 5 mM, lept4 grew better than the wild type. Mycorrhizal infection at a Cp of 0.05 mM resulted in a significant increase in the transcripts of LePT4 in the wild type and a concomitant 2-fold increase in Pi uptake. Although upon mycorrhizal infection, lept4 also exhibited an increased Pi uptake, it was significantly lower than that of the wild type. Under a Cp of 1 mM and in the absence of mycorrhizal infection, LePT4 expression was suppressed in the wild type and a mutation in this gene resulted in a slight reduction in total Pi uptake. These data highlight the pivotal role of LePT4 in mycorrhizal-mediated Pi uptake in tomato, and show that this function may not be fully compensated by other members of the family. Characterization of the mycorrhiza-associated Pi transporter lept4 mutant, along with expression analysis of LePT3, provides evidence for the different routes of mycorrhiza-mediated Pi uptake in plants.
Introduction
Acquisition of inorganic phosphate (Pi) by plants is regulated by two key soil and plant factors: Pi availability in the rhizosphere and the ability of plants to absorb it. The majority of terrestrial vascular plants are able to form arbuscular mycorrhizal (AM) associations which enhance the availability of nutrients, especially phosphorus (P), by forming far-reaching extraradical mycelia that operate as functional extensions of the plant root system (Harrison, 1999; Smith and Barker, 2002; Smith et al., 2004; Karandashov and Bucher, 2005; Bucher, 2007).
The Pi concentration in soil solution rarely exceeds 10 μM (Schachtman et al., 1998). In the absence of AM symbiosis, Pi from the rhizosphere is taken up directly by plant roots mainly via high-affinity Pi transporters expressed in epidermis and root hairs. When roots are colonized by AM fungi (AMF), Pi is acquired via a process that begins with uptake across membranes in the external hyphae, from sources distant from the root itself. This process continues with subsequent translocation of Pi along the fungal hyphae and is finally released across the arbuscular membrane which is then acquired by plant transporters located on the peri-arbuscular membrane (Smith and Smith, 1997; Harrison et al., 2002). In support of evidence suggesting the localization of Pi transporters at the peri-arbuscular membrane is the presence of two genes encoding for H+-ATPase, which provide the H+ ions required for co-transportation of P across the membrane (Gianinazzi-Pearson et al., 2000; Krajinski et al., 2002). Expression of two H+-ATPase genes (pma2, pma4) in tobacco (Gianinazzi-Pearson et al., 2000) and the plasma membrane H+-ATPase gene (Mtha1) from Medicago truncatula (Krajinski et al., 2002) is strongly induced in arbuscule-containing mycorrhizal tissues.
Several AM symbiosis-induced Pi transporter genes in roots have been isolated from different plant species (Bucher, 2007). They are StPT4 and StPT5 in potato and LePT4 and LePT5 in tomato (Nagy et al., 2005) and their orthologue genes in pepper, tobacco, and eggplant (Chen et al., 2007), OsPT11 in rice (Paszkowski et al., 2002), MtPT4 in M. truncatula (Harrison et al., 2002), HORvu;Pht1;8 in barley, TRIae;Pht1;myc in wheat, ZmPT6 in maize (Glassop et al., 2005), and LjPT3 in Lotus japonicus (Maeda et al., 2006). It is interesting to note that these genes have diverged sequences despite similarities in their putative physiological functions and mycorrhiza-regulated gene expression (Chen et al., 2007).
Knockout mutants of the genes encoding Pi transporters involved in Pi acquisition and/or translocation in the plant could greatly facilitate their functional characterization. In Arabidopsis thaliana, a non-mycorrhizal plant, eight of a total of nine Pht1 genes are expressed in roots and AtPht1;1 and AtPht1;4 show the highest transcription levels under a low Pi supply condition (Mudge et al., 2002). Mutants of either AtPht1;1 or AtPht1;4 showed significant defects in P uptake in a growth medium containing low P (Misson et al., 2004; Shin et al., 2004). A double mutation of AtPht1;1 and AtPht1;4 resulted in a 75% reduction in total P uptake when P is supplied at 0.5 mM, suggesting that some Pht1 proteins play a significant role in P acquisition from both low (0.002 mM) and high (0.5 mM) P environments (Shin et al., 2004). In L. japonicus the knockdown of LjPT3, a non-orthologous gene to MtPT4 of M. truncatula (Harrison et al., 2002), exhibited a decrease in Glomus mosseae arbuscules, leading to a significant reduction in Pi uptake via AM and reduced growth under Pi-limiting conditions (Maeda et al., 2006). Similarly, it has also been shown that complete silencing of MtPT4 expression results in premature death of arbuscules and no improvement in Pi acquisition by inoculation with mycorrhizal fungi (Javot et al., 2007).
Isolation of a tomato lept4 mutant (Meissner et al., 2000) has provided a unique opportunity to test the role of the LePT4 transporter in Pi acquisition under mycorrhizal and non-mycorrhizal conditions. Identification of two orthologous mycorrhiza-inducible Pi transporters, LePT4 and its paralogue LePT5 in tomato, has been reported (Nagy et al., 2005). Furthermore, detection by RNA hybridization in tomato using the StPT3 probe has shown that LePT3 might be the orthologue of StPT3, the mycorrhizal-inducible gene first characterized in potato (Rausch et al., 2001), which is expressed, highly in mycorrhizal roots, moderately in non-mycorrhizal roots, and at low levels in the leaves (Karandashov et al., 2004; Nagy et al., 2005). By characterizing a lept4-1 mutant containing two Ds insertions in its genome, Nagy et al. (2005) concluded that a high degree of functional redundancy occurs among the mycorrhiza-induced Pi transporters in solanaceous species. However, lept4-1 exhibited altered root morphology possibly due to a second Ds insertion in its genome (Nagy et al., 2005). In addition, the mycorrhizal-inducible LjPT3 in L. japonicus, appears to have a non-redundant role in Pi acquisition and colonization by mycorrhizal fungi (Maeda et al., 2006). These studies highlight the need for evaluating mycorrhiza-induced Pi-transporters using an appropriate knockout mutant. A homozygous lept4 mutant has been obtained with a normal root phenotype which was derived from the lept4-1 mutant that may have segregated for additional untagged mutations. Detailed functional analysis of the lept4 mutant provided direct evidence for the dual role of LePT4 in Pi acquisition, with and without mycorrhizal association, and in Pi toxicity.
Materials and methods
Isolation of lept4-1 and screening of lept4 mutant
The LePT4 knockout mutant and its nucleotide sequence originated from a Ac/Ds transposable element-tagged Micro-Tom population (Meissner et al., 2000). Identification of the new Pi transporter gene has already been described and the corresponding mutant allele in the background of F3 lines was called lept4-1 (Nagy et al., 2005). F4 plants derived from the lept4-1 F3 line were grown and screened for homozygous mutant alleles by PCR and later confirmed by Southern blot analysis. The homozygote mutant described by Nagy et al. (2005) is a sibling of homozygote F4 plants used in this study. Both siblings have the same mutant allele and the same Southern blot hybridization pattern (data not shown), but may have segregated for additional untagged mutations, such as transposon footprints. F5 seeds obtained from the homozygous F4 lines were called lept4 and were used in subsequent analyses.
Cloning of LePT3 from tomato
Gene-specific primers corresponding to potato Pi transporter StPT3 were designed. These primers (5′-GTATCTCAGCTGCAGTCAATGG-3′ and 5′-CCATGTACTAGTTGTTCCAAGC-3′) also correspond to the conserved sequences among the coding regions of LePT1, LePT2, and LePT4. Amplification of Micro-Tom genomic DNA with these primers yielded a PCR product of 700 bp. Sequencing of the PCR product confirmed that it has a high degree of sequence similarity with StPT3 and so is called LePT3. The full-length cDNA was obtained by rapid amplification of cDNA ends (RACE). The first-strand cDNA synthesis reaction for 3′-RACE was performed with a modified oligo(dt) primer (AP primer): 5′-GGCCACGCGTCGACTAGTAC(T)17-3′. The pair of primers used for PCR amplification of the 3′ ends of the LePT3 cDNA consisted of the AUAP primer 5′-GGCCACGCGTCGACTAGTAC-3′ and the LePT3 gene-specific primer 5′-TTGAGACACAGGGGAATA-3′. The pair of primers used for PCR amplification of the 5′-ends of the LePT3 cDNA were the StPT3 gene-specific primer from 42 bp upstream of the start codon, 5′-TAGTAGTTTGAGAAATATTACTAG-3′, and the LePT3 gene-specific primer, 5′-TGCAGATATAAAAGCTCCACGAG-3′. The nucleotide sequence of the entire coding region (accession no. AY804011) revealed 94% identity with StPT3.
Responses to different Pi supplies of Micro-Tom wild-type and lept4 mutant plants grown without soil
The Micro-Tom wild type (wt) and two bulked populations of homozygous lept4 mutants, each from a different pool of propagated seeds, were sown in compartmented nursery trays filled with perlite as culture medium. Each compartment provided a capacity of 0.2 dm3 for each plant with a maximum water-holding capacity of 0.55 dm3 dm−3 per compartment. The trays were then immersed in the nutrient solutions once a day in order to keep the perlite medium fully charged with nutrients. The nutrients were supplemented with three different Pi concentrations, i.e. 0.05, 0.5, and 5 mM with five replicates for each. The concentrations of the other nutrients supplied (expressed in mg dm−3) were: N, 140; K, 160; and the micro-elements (all EDTA-based) Zn, 0.5; Mn, 0.65; Fe, 0.8; Cu, 0.04; B, 0.4; and Mo, 0.03. The tap water used for irrigation contained (mg dm−3): Ca, 76; Mg, 34; Na, 80; CO3, 150; and Cl, 140. The whole shoot was harvested on the 35th day after sowing. The roots were gently washed with tap water to remove perlite and two sieves with 1 mm and 0.5 mm mesh sizes were used during washing to prevent the loss of fine roots. Harvested samples were blot-dried and weighed immediately to record fresh weight. These samples were then dried in an air-forced oven to determine dry weight. Finally, samples were ground to pass a 20 mesh sieve for subsequent chemical analysis. Samples (100 mg) were wet-ashed with H2SO4–H2O2 and analysed for Na, K, organic-N, and P. HClO4–HNO3 ashing was used in the analysis of Ca, Mg, and micronutrients. Nutrient element concentrations were determined with an injector Lachat Autoanalyzer (N and P), a flame photometer (K), and an atomic absorption spectrophotometer (Ca, Mg, Fe, Zn, and Mn). The data were subjected to statistical analysis by using Tukey's HSD test with a threshold of P0.05.
The effect of solution-Pi concentrations on P uptake and root morphology of the wild type and the mutant
A hydroponics experiment was conducted to evaluate the effects of different Pi concentrations on growth response and Pi uptake kinetics of the wild type and the mutant. Micro-Tom wt and the homozygote lept4 mutant seeds were germinated in Petri dishes and the plantlets were transferred to black plastic pots containing 10 dm3 of the nutrient solution. The nutrient solution contained the following macro-nutrients (mM): KNO3, 2; NH4NO3, 1; Ca(NO3)2, 0.5; H3PO4, 0.4; CaCl2, 0.25; MgSO4, 0.4. The concentrations of micro-nutrients were (μM): MnCl2, 9; H3BO3, 4.6; ZnSO4, 0.75; CuSO4, 0.3; (NH4)4Mo7O24, 0.04; Fe-EDTA, 12. The pH was adjusted with H2SO4 to 5.9. Six plants were grown in each pot and the hydroponic set-up was maintained in a greenhouse with a 14 h light period at 28–35 °C and a 10 h dark period at 20–24 °C. Modest aeration was provided to minimize disturbance to the roots. During the first 2 weeks, one-quarter of the nutrient solution in the hydroponic set-up was replaced with fresh solution after every 4 d. In the third week, one-half of the nutrient solution was replenished after every third day. During the fourth week, the solution was replaced completely with fresh solution on alternate days. During the fifth week the plants were supplied with the nutrient solution deficient in phosphate to produce uniformly Pi-starved plants. Finally, during the last 8 d of growth in hydroponics, sets of plants were supplied with nutrient solution containing different concentrations of Pi (0, 0.025, 0.05, 0.1, 0.2, 0.4, 0.6, and 1.0 mM) which were replaced daily. Each of the Pi concentration treatments had eight replicates. The root systems were scanned with a digital scanner and subsequently analysed for various morphological parameters using a WinRHIZO software package (Regent Instrument Inc., Canada).
Responses of the wild type and the lept4 mutant to infection with mycorrhizal fungus
Plant growth conditions
The pot experiment was conducted in a screen house (10% shade). During the entire growing period the air temperature varied between 17 °C and 32 °C during the day and between 12 °C and 20 °C at night. Inside the screen house, daily mean values of total irradiance ranged from 204 to 254 MJ m−2 d−1. A single germinated seed was placed in a 3 dm3 plastic pot (15 cm height×16 cm diameter) filled with newly sterilized quartz-sand. The density and pot water-holding capacity of the sand were 1.58 kg dm−3 and 0.136 dm3 dm−3, respectively. One set of wt and the lept4 mutant plants, maintained under two different Pi concentrations (0.05 mM and 1.0 mM), were inoculated with Glomus intraradices. As controls, one set of wt and the mutant plants was maintained without inoculation. The experimental design comprised four randomized blocks with five replicates each. The plants were individually irrigated with nutrient solution via two drippers (2 dm3 h−1) on the surface of the sand medium. The concentrations of other nutrients in the medium (mg dm−3) were: N, 140; K, 160; Zn, 0.5; Mn, 0.65; Fe, 0.8; Cu, 0.04; B, 0.4; Mo, 0.03. All the micro-elements were EDTA based. The initial pH values of the irrigating solutions with high and low P concentrations were 6.55±0.1 and 7.05±0.1, respectively. Plants were irrigated three times a day via a pressure-compensated dripper (2.0 dm3 h−1) (Netafim Inc., Israel).
Preparation and application of Glomus intraradices
The AMF, Glomus intraradices Schenck & Smith (BEG141) was maintained in association with leek plants, in sand, for 4 months. At harvest, the rooting matrix (sand) containing spores, hyphae, and infected roots was air-dried and used as a sand-based mycorrhizal inoculum (SBMI). Prior to the experiment the infectivity potential of the SBMI was evaluated by the most probable number analysis as described by Haas and Krikun (1985). The SBMI was used by placing 0.075 dm3 of it 7.5 cm below the sand surface (middle part of the 3 dm3 pot) in a 0.5-cm-thick layer 1 d before sowing.
Root and shoot sampling at harvest
Fifty days after sowing, five plants from each of the treatments were harvested and pooled. For each treatment, the total shoot fresh weight was recorded and immediately 2–3 g of leaves were frozen in liquid nitrogen and stored at –80 °C till further use in molecular analysis. As the AMF inoculum was placed at a fixed depth of 7 cm as a single band in the pots, roots were sampled 3 cm above and below the inoculum placement band and used for further analysis. Roots were washed in bulk, blot-dried, and weighed before being separated into three random groups: (i) 2–3 g of the root section was harvested, stored at –80 °C, and used subsequently for RT-PCR and northern blot analyses; (ii) 2 g was used for the evaluation of the AMF colonization rate; and (iii) the reminder of the samples was dried in a forced-draught oven at 60 °C and the plant water content computed from the differences between fresh and dried weights. The dried materials were then ground and used for chemical analysis, as described earlier.
Detection of mycorrhizal colonization
Root segments were treated with 10% (w/v) KOH heated to 70 °C for 3 h and then treated further with 1% HCl solution for 10 min. The roots were then stained with trypan blue and examined under a binocular microscope (WILD M8; Heerbrugg, Switzerland) and the percentage of root length colonized by AMF calculated using the line-intersect method (Giovannetti and Mosse, 1980).
Reverse transcription–polymerase chain reaction (RT-PCR)
RT-PCR was performed in a total volume of 21 mm3 using SuperScript™ II RNase H reverse transcriptase as described by the manufacturer (Invitrogen® Corporation, USA). A 2 mm3 aliquot from the 21 mm3 RT-PCR reaction was used for further PCR amplification. Positive and negative controls comprised wt genomic DNA and RT samples with no reverse transcriptase added, respectively. An actin gene in tomato (accession no. U60481) was used as a quantitative control for the RT-PCR (Chen et al., 2007). The primers used were: 5′-ATGGCGAACGATTTGCAAGTGC-3′ and 5′-GTGTATACTAACTGATAACTTGTC-3′ for LePT1; 5′-ATGGCTGTGGGGGATAATGA-3′ and 5′-CATGCTTCTCCAGTTATAGTTT-3′ for LePT2; 5′-AGTCAATGGAGTCGCGTTTG-3′ and 5′-GACGATGAACAAACTCCTTAG-3′ for LePT3; and 5′-CATGGCCTCAGACAACCTTG-3′ and 5′-CCTTCAATAATCGCAGTGTAAC-3′ for LePT4. The expected lengths of amplified LePT1, LePT2, LePT3, and LePT4 transcripts were 1.65, 1.59, 0.67, and 0.72 kb, respectively. PCR was conducted in a thermal cycler with heated lid (Biometra®, Germany) at 94 °C for 3 min, followed by 1 min at 94 °C, 1 min at 55 °C, 1 min at 72 °C (for LePT3 and LePT4), or 2 min (for LePT1 and LePT2) for 35 cycles with a final extension of 5 min at 72 °C. Ten microlitres of the amplification products were analysed by electrophoresis on a 1.5% agarose gel, stained with ethidium bromide, and examined with a UV transilluminator. Images of the gels were captured with an ‘NIH Image 1.61’ program.
The expression of the four Pi transporters in the tomato roots was analysed by semi-quantitative RT-PCR as described by Burleigh (2001). The linear amplification phase was checked according to the actin signal intensity and was found at 15 PCR cycles under the conditions tested. The PCR products were then separated on 1.5% agarose gel, blotted onto Hybond N+ membrane and hybridized with their respective 32P-labelled cDNA probes, amplified with the primers as described above.
Results
Responses to different Pi supplies of Micro-Tom wild-type and lept4 mutant plants grown without soil
Under a Cp of 0.05 mM the lept4 mutant showed severe Pi-deficiency symptoms as indicated by an early accumulation of anthocyanin in the leaves (data not shown). In addition, there was a slight but not significant reduction in the shoot dry weight (DW), shoot-P concentration, Pi uptake, and root DW in the mutant as compared with the wild type (Fig. 1A–D). However, as the Cp increased to 0.5 mM, the Pi uptake and DW of the shoot and the root of the wt were significantly higher as compared with the lept4 mutant (Fig. 1A, C, D). The differences in the ability of the wt and the mutant to acquire and mobilize Pi became more apparent when they were grown under a higher Cp (5 mM). For instance, the wild-type plants exhibited a significantly higher shoot-P concentration as compared with the mutant (Fig. 1B). The effect of a higher accumulation of P by the wt (>10 g kg−1 DW), a critical Pi toxicity level for tomato plants (Parker et al., 1992; Jones, 1998), was reflected in its lower shoot and root DW as compared with the mutant (Fig. 1A, D). The higher shoot DW of the mutant, an indicator of better growth, could thus be attributed to its inability to accumulate toxic levels of Pi due to functional knockout of LePT4. The concentrations of nutritional elements (N, K, Ca, Mg, Fe, Zn, and Mn) in the leaves and the roots of the mutant and wt grown under different Pi levels were within the optimal range (data not presented) for tomato (Jones et al., 1991). This suggests that the difference observed in the biomass yield between the wt and the lept4 mutant under Pi starvation (0.05 mM), sufficient (0.5 mM), and toxic supply (5 mM) conditions were mainly due to their differing abilities to acquire external Pi and its mobilization to growing tissues.
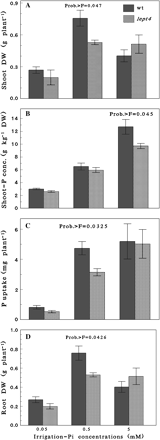
Effects of different irrigation-Pi concentrations on growth and Pi nutrition of Micro-Tom wt and the lept4 mutant grown in soil-less media. The wild type and the mutant were grown in soil-less media supplemented with 0.05, 0.5, and 5 mM Pi concentrations. The data are presented for (A) shoot dry weight (DW), (B) shoot-P concentration, (C) P uptake, and (D) root DW. Probability >F is given on top of the bar when the differences between wt and the mutant were significantly different. Values are mean ±SE, n=5.
Responses of hydroponically grown Micro-Tom wild type and lept4 mutant to Pi supply
Root growth and Pi uptake were monitored in hydroponically grown wt and lept4 mutant supplied with different concentrations of Pi. No visible differences could be observed in shoot phenotype or root morphology (Fig. 2), shoot and root DW, and primary root length of the wt and the lept4 mutant plants grown hydroponically under different Pi concentrations (Table 1). The significant interaction of genotype×solution-Pi for root DW (P<0.0046), as revealed by the bi-factorial analysis of variance (Table 1), showed that the effect of solution-Pi depends on the plant genotype for the LePT4 locus. The differences in shoot-P between the wt and the lept4 mutant at each single concentration of solution-Pi tested were small and not statistically significant (except at 0.025 mM and 0.4 mM; Fig. 3A). However, taking into account the whole range of solution-Pi concentrations (0–1 mM), the shoot-P concentration of the wt was significantly higher than that of the mutant (P <0.0001). The mutant roots are more sensitive to low P treatments than the high P treatments (Fig. 3A). In addition, the daily Pi uptake by the wt and the mutant as a function of solution-Pi concentrations was evaluated (Fig. 3B). The Michaelis–Menten equation was used to calculate the predicted affinity values for the wt and lept4 mutant. On a dry weight basis, the values were 0.019 mM and 0.026 mM for the wt and the lept4 mutant, respectively. Corresponding values calculated on the basis of root unit length were 0.024 mM and 0.026 mM for the wt and lept4 mutant, respectively.
The effect of solution-P concentrations on dry weights of shoot and root and on root length of lept4 mutant and wt plants
Solution-P (mM) | Shoot DW (g plant−1) | Root DW (g plant−1) | Root length (cm plant−1) | |||
wt | lept4 | wt | lept4 | wt | lept4 | |
0 | 0.12 d | 0.11 d | 0.033 d | 0.025 c | 264 b | 251 d |
0.025 | 0.19 c | 0.18 c | 0.038 cd | 0.044 bc | 380 ab | 296 cd |
0.05 | 0.25 bc | 0.24 b | 0.044 bcd | 0.045 abc | 389 ab | 360 abc |
0.1 | 0.26 bc | 0.32 a | 0.050 bc | 0.053 ab | 409 a | 345 bcd |
0.2 | 0.30 ab | 0.35 a | 0.048 bcd | 0.060 ab | 487 a | 458 a |
0.4 | 0.36 a | 0.33 a | 0.058 ab | 0.060 ab | 403 a | 402 abc |
0.6 | 0.34 a | 0.34 a | 0.058 ab | 0.063 ab | 398 a | 427 ab |
1.0 | 0.36 a | 0.34 a | 0.073 a | 0.068 a | 418 a | 421 ab |
LSD0.05 | 0.068 | 0.052 | 0.0160 | 0.0234 | 132.9 | 106.7 |
Probability >F | <0.0001 | <0.0001 | <0.0001 | <0.0001 | 0.0019 | <0.0001 |
Genotype | 0.279 | 0.508 | 0.065 | |||
Solution-Pi | <0.0001 | <0.0001 | <0.0001 | |||
Genotype×soil-Pi | 0.393 | 0.0046 | 0.430 |
Solution-P (mM) | Shoot DW (g plant−1) | Root DW (g plant−1) | Root length (cm plant−1) | |||
wt | lept4 | wt | lept4 | wt | lept4 | |
0 | 0.12 d | 0.11 d | 0.033 d | 0.025 c | 264 b | 251 d |
0.025 | 0.19 c | 0.18 c | 0.038 cd | 0.044 bc | 380 ab | 296 cd |
0.05 | 0.25 bc | 0.24 b | 0.044 bcd | 0.045 abc | 389 ab | 360 abc |
0.1 | 0.26 bc | 0.32 a | 0.050 bc | 0.053 ab | 409 a | 345 bcd |
0.2 | 0.30 ab | 0.35 a | 0.048 bcd | 0.060 ab | 487 a | 458 a |
0.4 | 0.36 a | 0.33 a | 0.058 ab | 0.060 ab | 403 a | 402 abc |
0.6 | 0.34 a | 0.34 a | 0.058 ab | 0.063 ab | 398 a | 427 ab |
1.0 | 0.36 a | 0.34 a | 0.073 a | 0.068 a | 418 a | 421 ab |
LSD0.05 | 0.068 | 0.052 | 0.0160 | 0.0234 | 132.9 | 106.7 |
Probability >F | <0.0001 | <0.0001 | <0.0001 | <0.0001 | 0.0019 | <0.0001 |
Genotype | 0.279 | 0.508 | 0.065 | |||
Solution-Pi | <0.0001 | <0.0001 | <0.0001 | |||
Genotype×soil-Pi | 0.393 | 0.0046 | 0.430 |
Different letters indicate a significant difference at the P >0.005 level.
The effect of solution-P concentrations on dry weights of shoot and root and on root length of lept4 mutant and wt plants
Solution-P (mM) | Shoot DW (g plant−1) | Root DW (g plant−1) | Root length (cm plant−1) | |||
wt | lept4 | wt | lept4 | wt | lept4 | |
0 | 0.12 d | 0.11 d | 0.033 d | 0.025 c | 264 b | 251 d |
0.025 | 0.19 c | 0.18 c | 0.038 cd | 0.044 bc | 380 ab | 296 cd |
0.05 | 0.25 bc | 0.24 b | 0.044 bcd | 0.045 abc | 389 ab | 360 abc |
0.1 | 0.26 bc | 0.32 a | 0.050 bc | 0.053 ab | 409 a | 345 bcd |
0.2 | 0.30 ab | 0.35 a | 0.048 bcd | 0.060 ab | 487 a | 458 a |
0.4 | 0.36 a | 0.33 a | 0.058 ab | 0.060 ab | 403 a | 402 abc |
0.6 | 0.34 a | 0.34 a | 0.058 ab | 0.063 ab | 398 a | 427 ab |
1.0 | 0.36 a | 0.34 a | 0.073 a | 0.068 a | 418 a | 421 ab |
LSD0.05 | 0.068 | 0.052 | 0.0160 | 0.0234 | 132.9 | 106.7 |
Probability >F | <0.0001 | <0.0001 | <0.0001 | <0.0001 | 0.0019 | <0.0001 |
Genotype | 0.279 | 0.508 | 0.065 | |||
Solution-Pi | <0.0001 | <0.0001 | <0.0001 | |||
Genotype×soil-Pi | 0.393 | 0.0046 | 0.430 |
Solution-P (mM) | Shoot DW (g plant−1) | Root DW (g plant−1) | Root length (cm plant−1) | |||
wt | lept4 | wt | lept4 | wt | lept4 | |
0 | 0.12 d | 0.11 d | 0.033 d | 0.025 c | 264 b | 251 d |
0.025 | 0.19 c | 0.18 c | 0.038 cd | 0.044 bc | 380 ab | 296 cd |
0.05 | 0.25 bc | 0.24 b | 0.044 bcd | 0.045 abc | 389 ab | 360 abc |
0.1 | 0.26 bc | 0.32 a | 0.050 bc | 0.053 ab | 409 a | 345 bcd |
0.2 | 0.30 ab | 0.35 a | 0.048 bcd | 0.060 ab | 487 a | 458 a |
0.4 | 0.36 a | 0.33 a | 0.058 ab | 0.060 ab | 403 a | 402 abc |
0.6 | 0.34 a | 0.34 a | 0.058 ab | 0.063 ab | 398 a | 427 ab |
1.0 | 0.36 a | 0.34 a | 0.073 a | 0.068 a | 418 a | 421 ab |
LSD0.05 | 0.068 | 0.052 | 0.0160 | 0.0234 | 132.9 | 106.7 |
Probability >F | <0.0001 | <0.0001 | <0.0001 | <0.0001 | 0.0019 | <0.0001 |
Genotype | 0.279 | 0.508 | 0.065 | |||
Solution-Pi | <0.0001 | <0.0001 | <0.0001 | |||
Genotype×soil-Pi | 0.393 | 0.0046 | 0.430 |
Different letters indicate a significant difference at the P >0.005 level.
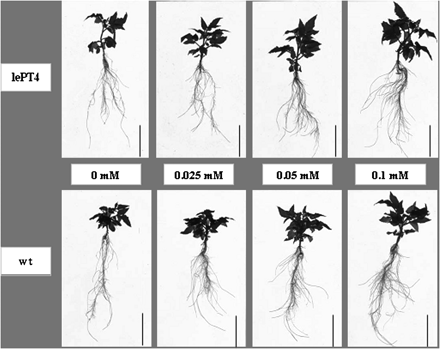
Effects of different solution-Pi concentrations on shoot and root growth of hydroponically grown Micro-Tom wt and lept4 mutant. Photographs are representatives of the wt and the mutant for each of the treatments. Scale bar=6 cm.
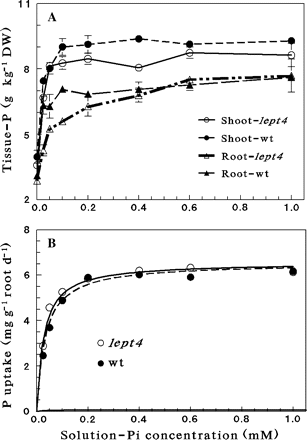
Effects of solution-Pi concentrations on shoot- and root-P concentrations and on P uptake in hydroponically grown Micro-Tom wt and the lept4 mutant. Data are presented for (A) shoot- and root-P concentrations of the two genotypes as a function of Pi concentration; (B) total P uptake as a function of Pi concentration. The predicted Fmax and Km values of the Michaelis–Menten equation (SE of each parameter is indicated in parenthesis): 6.5 (0.13) mg g−1 root d−1 and 0.027 (0.0039) mM Pi for lept4, and 6.5 (0.15) mg g−1 root d−1 and 0.033 (0.0042) mM Pi for the wt, respectively. Values are mean ±SE, n=5.
Responses of the lept4 mutant and the wild type to infection by Glomus intraradices
Under a Cp of 0.05 mM in sand culture, mycorrhizal infection in the form of arbuscules and vesicles was observed on the roots of both lept4 mutant and the wt ,and the percentage of root length colonized by the AMF was 65% and 60%, respectively. However, under a Cp of 1 mM the percentage of root length colonized by the AMF was negligible (∼3%) in both the wt and the mutant. Tomato growth under a Cp of 0.05 mM and with no mycorrhizal infection showed that the lept4 mutant was more sensitive to P deficiency than the wt (Fig. 4; Table 2). Mycorrhizal infection of the wt roots supplied with a Cp of 0.05 mM resulted in an increase in shoot and root DW by 60% and 81%, respectively. The corresponding values for the lept4 mutant were only 47% and 45%, respectively (Table 2). The effects of mycorrhiza were statistically significant, as well as the interaction of genotype×mycorrhiza (Table 2). The results suggest that LePT4 contributes significantly to the beneficial effects of mycorrhizal fungi by enhancing Pi acquisition under low-Pi conditions (Table 2). P concentrations in both the shoots and roots of the lept4 mutant under a Cp of 0.05 mM were slightly but not significantly lower than those in the wt. However, this difference was greatly amplified in response to infection by the mycorrhizal fungi (Table 3). The only significant effects of mycorrhizal inoculation on total Pi uptake have been observed for the wt under a Cp of 0.05 mM, and all other combinations (wt under a Cp of 1 mM and mutant under both Pi concentrations) were not statistically significant (Table 3). A significant interaction of genotype×mycorrhiza showed that the effect of mycorrhiza depends on the plant genotype. No significant effects of either the plant genotype or mycorrhizal inoculation were detected at the higher concentration of Pi (1 mM). Significant linear regressions were obtained under a Cp of 0.05 mM between the shoot yield and both the shoot and root P concentrations (r2 of 0.97 and 0.93, respectively), which suggests that the sensitivity of the lept4 mutant to Pi starvation is due to its impaired ability to acquire external Pi, especially via the mycorrhizal roots. The concentrations of other nutritional elements in the leaves and the roots of the mutant were within the optimal range (data not presented) as reported for tomato (Jones et al., 1991).
Mycorrhizal infection effects on DW of shoot, root, and root:shoot ratio in wt and lept4 plants grown under irrigation-Pi concentrations of 0.05 mM and 1.0 mM
Treatments | 0.05 mM Pi | 1.0 mM Pi | ||||
Shoot (g plant−1 DW) | Root (g plant−1 DW) | Root:shoot (g g-1 DW) | Shoot (g plant−1 DW) | Root (g plant−1 DW) | Root:shoot (g g-1 DW) | |
wt –Myc | 0.60 b | 0.16 b | 0.27 | 2.73 ab | 0.66 | 0.24 |
wt +Myc | 0.96 a | 0.29 a | 0.31 | 2.94 a | 0.60 | 0.20 |
lept4 –Myc | 0.38 b | 0.11 b | 0.30 | 2.39 b | 0.58 | 0.24 |
lept4 +Myc | 0.56 b | 0.16 b | 0.28 | 2.60 b | 0.68 | 0.18 |
LSD0.05 | 0.233 | 0.066 | 0.037 | 0.312 | 0.242 | 0.046 |
Probability >F | ||||||
Genotype | 0.0001 | <0.0001 | 0.9969 | 0.0007 | 0.0972 | 0.5211 |
Myc | 0.0004 | 0.0001 | 0.5402 | 0.1454 | 0.1745 | 0.0145 |
Genotype×myc | 0.132 | 0.0151 | 0.1443 | 0.982 | 0.719 | 0.5513 |
Treatments | 0.05 mM Pi | 1.0 mM Pi | ||||
Shoot (g plant−1 DW) | Root (g plant−1 DW) | Root:shoot (g g-1 DW) | Shoot (g plant−1 DW) | Root (g plant−1 DW) | Root:shoot (g g-1 DW) | |
wt –Myc | 0.60 b | 0.16 b | 0.27 | 2.73 ab | 0.66 | 0.24 |
wt +Myc | 0.96 a | 0.29 a | 0.31 | 2.94 a | 0.60 | 0.20 |
lept4 –Myc | 0.38 b | 0.11 b | 0.30 | 2.39 b | 0.58 | 0.24 |
lept4 +Myc | 0.56 b | 0.16 b | 0.28 | 2.60 b | 0.68 | 0.18 |
LSD0.05 | 0.233 | 0.066 | 0.037 | 0.312 | 0.242 | 0.046 |
Probability >F | ||||||
Genotype | 0.0001 | <0.0001 | 0.9969 | 0.0007 | 0.0972 | 0.5211 |
Myc | 0.0004 | 0.0001 | 0.5402 | 0.1454 | 0.1745 | 0.0145 |
Genotype×myc | 0.132 | 0.0151 | 0.1443 | 0.982 | 0.719 | 0.5513 |
–Myc and +Myc, plants without and with the mycorrhizal inoculum, respectively. Different letters indicate a significant difference at the P >0.005 level.
Mycorrhizal infection effects on DW of shoot, root, and root:shoot ratio in wt and lept4 plants grown under irrigation-Pi concentrations of 0.05 mM and 1.0 mM
Treatments | 0.05 mM Pi | 1.0 mM Pi | ||||
Shoot (g plant−1 DW) | Root (g plant−1 DW) | Root:shoot (g g-1 DW) | Shoot (g plant−1 DW) | Root (g plant−1 DW) | Root:shoot (g g-1 DW) | |
wt –Myc | 0.60 b | 0.16 b | 0.27 | 2.73 ab | 0.66 | 0.24 |
wt +Myc | 0.96 a | 0.29 a | 0.31 | 2.94 a | 0.60 | 0.20 |
lept4 –Myc | 0.38 b | 0.11 b | 0.30 | 2.39 b | 0.58 | 0.24 |
lept4 +Myc | 0.56 b | 0.16 b | 0.28 | 2.60 b | 0.68 | 0.18 |
LSD0.05 | 0.233 | 0.066 | 0.037 | 0.312 | 0.242 | 0.046 |
Probability >F | ||||||
Genotype | 0.0001 | <0.0001 | 0.9969 | 0.0007 | 0.0972 | 0.5211 |
Myc | 0.0004 | 0.0001 | 0.5402 | 0.1454 | 0.1745 | 0.0145 |
Genotype×myc | 0.132 | 0.0151 | 0.1443 | 0.982 | 0.719 | 0.5513 |
Treatments | 0.05 mM Pi | 1.0 mM Pi | ||||
Shoot (g plant−1 DW) | Root (g plant−1 DW) | Root:shoot (g g-1 DW) | Shoot (g plant−1 DW) | Root (g plant−1 DW) | Root:shoot (g g-1 DW) | |
wt –Myc | 0.60 b | 0.16 b | 0.27 | 2.73 ab | 0.66 | 0.24 |
wt +Myc | 0.96 a | 0.29 a | 0.31 | 2.94 a | 0.60 | 0.20 |
lept4 –Myc | 0.38 b | 0.11 b | 0.30 | 2.39 b | 0.58 | 0.24 |
lept4 +Myc | 0.56 b | 0.16 b | 0.28 | 2.60 b | 0.68 | 0.18 |
LSD0.05 | 0.233 | 0.066 | 0.037 | 0.312 | 0.242 | 0.046 |
Probability >F | ||||||
Genotype | 0.0001 | <0.0001 | 0.9969 | 0.0007 | 0.0972 | 0.5211 |
Myc | 0.0004 | 0.0001 | 0.5402 | 0.1454 | 0.1745 | 0.0145 |
Genotype×myc | 0.132 | 0.0151 | 0.1443 | 0.982 | 0.719 | 0.5513 |
–Myc and +Myc, plants without and with the mycorrhizal inoculum, respectively. Different letters indicate a significant difference at the P >0.005 level.
Mycorrhizal fungi infection effects on shoot- and root-P concentrations and on total P uptake in wt and lept4 mutant grown under irrigation-P concentrations of 0.05 mM or 1.0 mM
Treatments | 0.05 mM Pi | 1.0 mM Pi | ||||
Tissue-P concentration | Total P (mg plant−1 DW) | Tissue-P concentration | Total P (mg plant−1 DW) | |||
Shoot (g kg−1 DW) | Root (g kg−1 DW) | Shoot (g kg−1 DW) | Root (g kg−1 DW) | |||
wt –Myc | 3.8 b | 2.6 | 2.7 b | 8.1 | 9.6 | 28.5 |
wt +Myc | 4.4 a | 2.9 | 5.1 a | 8.1 | 8.9 | 29.3 |
lept4 –Myc | 3.7 b | 2.1 | 1.6 c | 8.1 | 9.4 | 25.0 |
lept4 +Myc | 3.9 b | 2.3 | 2.5 bc | 8.2 | 9.0 | 25.6 |
LSD0.05 | 0.49 | 0.52 | 1.07 | 1.04 | 1.18 | 5.7 |
Probability >F | ||||||
Genotype | 0.012 | 0.0009 | <0.0001 | 0.9124 | 0.9374 | 0.0210 |
Myc | 0.0059 | 0.0485 | <0.0001 | 0.9178 | 0.0691 | 0. 5955 |
Genotype×myc | 0.1611 | 0.5523 | 0.0145 | 0.9961 | 0.5543 | 0.9527 |
Treatments | 0.05 mM Pi | 1.0 mM Pi | ||||
Tissue-P concentration | Total P (mg plant−1 DW) | Tissue-P concentration | Total P (mg plant−1 DW) | |||
Shoot (g kg−1 DW) | Root (g kg−1 DW) | Shoot (g kg−1 DW) | Root (g kg−1 DW) | |||
wt –Myc | 3.8 b | 2.6 | 2.7 b | 8.1 | 9.6 | 28.5 |
wt +Myc | 4.4 a | 2.9 | 5.1 a | 8.1 | 8.9 | 29.3 |
lept4 –Myc | 3.7 b | 2.1 | 1.6 c | 8.1 | 9.4 | 25.0 |
lept4 +Myc | 3.9 b | 2.3 | 2.5 bc | 8.2 | 9.0 | 25.6 |
LSD0.05 | 0.49 | 0.52 | 1.07 | 1.04 | 1.18 | 5.7 |
Probability >F | ||||||
Genotype | 0.012 | 0.0009 | <0.0001 | 0.9124 | 0.9374 | 0.0210 |
Myc | 0.0059 | 0.0485 | <0.0001 | 0.9178 | 0.0691 | 0. 5955 |
Genotype×myc | 0.1611 | 0.5523 | 0.0145 | 0.9961 | 0.5543 | 0.9527 |
wt, Wild type; lept4, lept4 mutant; –Myc and +Myc, without and with the mycorrhiza inoculum, respectively Different letters indicate significant difference at the P >0.005 level.
Mycorrhizal fungi infection effects on shoot- and root-P concentrations and on total P uptake in wt and lept4 mutant grown under irrigation-P concentrations of 0.05 mM or 1.0 mM
Treatments | 0.05 mM Pi | 1.0 mM Pi | ||||
Tissue-P concentration | Total P (mg plant−1 DW) | Tissue-P concentration | Total P (mg plant−1 DW) | |||
Shoot (g kg−1 DW) | Root (g kg−1 DW) | Shoot (g kg−1 DW) | Root (g kg−1 DW) | |||
wt –Myc | 3.8 b | 2.6 | 2.7 b | 8.1 | 9.6 | 28.5 |
wt +Myc | 4.4 a | 2.9 | 5.1 a | 8.1 | 8.9 | 29.3 |
lept4 –Myc | 3.7 b | 2.1 | 1.6 c | 8.1 | 9.4 | 25.0 |
lept4 +Myc | 3.9 b | 2.3 | 2.5 bc | 8.2 | 9.0 | 25.6 |
LSD0.05 | 0.49 | 0.52 | 1.07 | 1.04 | 1.18 | 5.7 |
Probability >F | ||||||
Genotype | 0.012 | 0.0009 | <0.0001 | 0.9124 | 0.9374 | 0.0210 |
Myc | 0.0059 | 0.0485 | <0.0001 | 0.9178 | 0.0691 | 0. 5955 |
Genotype×myc | 0.1611 | 0.5523 | 0.0145 | 0.9961 | 0.5543 | 0.9527 |
Treatments | 0.05 mM Pi | 1.0 mM Pi | ||||
Tissue-P concentration | Total P (mg plant−1 DW) | Tissue-P concentration | Total P (mg plant−1 DW) | |||
Shoot (g kg−1 DW) | Root (g kg−1 DW) | Shoot (g kg−1 DW) | Root (g kg−1 DW) | |||
wt –Myc | 3.8 b | 2.6 | 2.7 b | 8.1 | 9.6 | 28.5 |
wt +Myc | 4.4 a | 2.9 | 5.1 a | 8.1 | 8.9 | 29.3 |
lept4 –Myc | 3.7 b | 2.1 | 1.6 c | 8.1 | 9.4 | 25.0 |
lept4 +Myc | 3.9 b | 2.3 | 2.5 bc | 8.2 | 9.0 | 25.6 |
LSD0.05 | 0.49 | 0.52 | 1.07 | 1.04 | 1.18 | 5.7 |
Probability >F | ||||||
Genotype | 0.012 | 0.0009 | <0.0001 | 0.9124 | 0.9374 | 0.0210 |
Myc | 0.0059 | 0.0485 | <0.0001 | 0.9178 | 0.0691 | 0. 5955 |
Genotype×myc | 0.1611 | 0.5523 | 0.0145 | 0.9961 | 0.5543 | 0.9527 |
wt, Wild type; lept4, lept4 mutant; –Myc and +Myc, without and with the mycorrhiza inoculum, respectively Different letters indicate significant difference at the P >0.005 level.
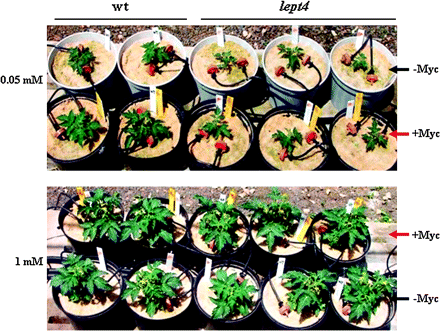
Responses of Micro-Tom wt and lept4 mutant to mycorrhizal infection during growth under irrigation-Pi concentrations of 0.05 mM and 1 mM. +Myc represents sand-based mycorrhizal inoculum containing Glomus intraradices, whereas –Myc indicates the control plants which were not infected with mycorrhizal fungi.
The effect of Pi concentrations and infection with mycorrhizal fungi on the expression of Pi transporters
Transcripts of LePT1 were higher in the low P treatment (Cp of 0.05 mM) than the high P treatment (Cp of 1 mM) with no differences between genotypes and AM fungal colonization (Fig. 5). LePT2 has similar transcript patterns to LePT1; the wt may have higher transcript number than the mutant at low P treatment, but this would need to be confirmed with quantitative real-time PCR. LePT3 transcripts are detected under all conditions with an increase seen due to low P treatment and AM fungal colonization but not genotype. LePT4 transcripts are only detected in the wt and are significantly increased in the wt when grown in the low-P treatment and colonized by AM fungi. No LePT4 transcripts were detected in the mutant under these conditions which indicated the functional inactivation of this gene.
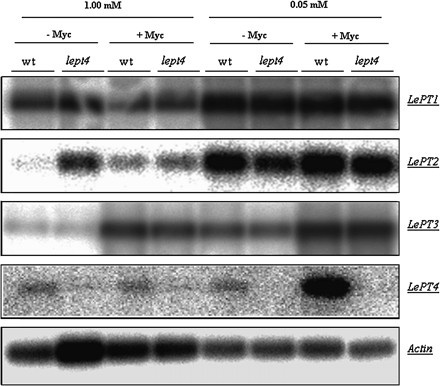
Semi-quantitative RT-PCR coupled with Southern blot analysis (referring to Burleigh, 2001) to evaluate the effects of varying Pi concentrations on the expression of tomato Pi transporters. Fifteen cycles of RT-PCR were performed to amplify the Pi transporter transcripts from tomato (LePT1, LePT2, LePT3, and LePT4), which were subsequently hybridized with their respective cDNA probes for Southern blot analysis. Intensity of actin signal shows the relative amount of initial total mRNA which was amplified by PCR in the linear phases.
Discussion
Plants have intricate mechanisms to acquire the essential nutrient Pi via a set of transporters responding to Pi deficiency and mycorrhizal symbiosis. Physiological studies with 33Pi demonstrated that AM fungi could provide the dominant route for Pi uptake in many plant species irrespective of the responses of both growth and total P uptake to AM colonization (Smith et al., 2003, 2004; Li et al., 2006). Mycorrhizal plants preferentially switch over to symbiotic Pi delivery during Pi deficiency by co-ordinated and concurrent suppression and/or induction of genes. Gene knockouts and mutants offer some of the most powerful genetic tools to dissect the function of candidate genes. Suppression of LjPT3, a mycorrhiza-inducible gene in L. japonicus, by RNAi significantly reduced plant growth, Pi uptake via mycorrhiza, and suppression of mutualistic symbioses (Maeda et al., 2006). Mycorrhiza-regulated Pi uptake may involve multiple transporters as suggested by the existence of non-orthologues of LjPT3 such as MtPT4 in Medicago, another model legume. Recently, Javot et al. (2007) found that complete knockdown of MtPT4 in Medicago resulted in loss of the improvement in P uptake by inoculation with an AM fungus (G. versiforme) and inhibition of arbuscular formation in the cortex cells of roots in the mutant. Thus, they suggested that plants would allow continual arbuscular development and existence of mycorrhizal fungal in the roots only when the soil P captured by the hyphae could be efficiently transported into the roots (Javot et al., 2007), although studies on two non-orthologous mycorrhiza-responsive genes encoding Pi transporters in the Solanaceae (Nagy et al., 2005) led to the functional characterization of a lept4 homozygote mutant. The data presented here provide direct evidence that LePT4 is involved with Pi acquisition in both uptake pathways, i.e. direct or mycorrhizal. In addition, lack of Pi toxicity in lept4 mutants grown with a high level of Pi (5 mM) (Table 1) indicates the potential involvement of LePT4 in loading when the Pi supply is in excess, which is one of the characteristic features of a low-affinity Pi transporter. This is in agreement with the yeast complementation data showing that LePT4 could encode for a low-affinity Pi transporter in tomato (Nagy et al., 2005). Regardless of the Pi concentrations in the growth medium, in the absence of mycorrhizal fungi the LePT4 transcript level was very low in the wt plants (Fig. 5). Under a Cp of 1 mM, the overall growth of the lept4 mutant was comparable to that of the wt (Tables 2, 3). However, under a lower Cp level (0.05 mM) the lept4 mutant exhibited severe P-deficiency symptoms, including significant reduction in growth and Pi uptake (∼30% less than in wt). These findings were confirmed in several independent experiments using different siblings of the original mutant heterozygote for the LePT4 mutation (data not shown). In this study, major changes were not observed in the root morphology of the mutant and the wild type as reported by Nagy et al. (2005). This was surprising considering that both the mutants are homozygous for LePT4. The original LePT4 mutation was isolated from a heterozygote plant that contained two Ds insertions. Homozygotes were selected for the LePT4 locus, but another Ds insertion segregated in the background of the homozygote lept4 mutant. This may explain the differences observed between the present study and that of an earlier report (Nagy et al., 2005). It is also very unlikely that the mycorrhiza/Pi uptake phenotypes observed in the mutant could have resulted from a segregating second site mutation, because (i) the phenotype was related to the expected gene function thus the chance that the second mutation was also in a mycorrhiza-related gene is very low; (ii) the phenotype was observed in all the progenies of the homozygote, regardless of the segregation of the second Ds. This suggests that despite the relatively low expression level of LePT4, and strong induction of LePT1 and LePT2 under low-Pi conditions (Fig. 5), LePT4 is involved with the uptake of Pi via the direct plant route. It is noteworthy that in both wt and lept4 mutant there was no significant increase in the transcript levels of any of the other Pi transporters (LePT1, 2, and 3). This suggests that the ‘loss of function’ of LePT4 is not adequately compensated by other members of the Pi transporter family. This observation differs from that of the earlier study of Nagy et al. (2005) which suggested that there is redundancy within mycorrhiza-inducible Pi transporters (LePT3 and LePT5). This discrepancy may be due to the usage of different isolates of the AMF G. intraradices in their study. In the present study, G. intraradices strain BEG141 was used, while Nagy et al. (2005) used strains BEG75 and 87. This assumption is not entirely unfounded since large variations in functional diversity, measured in terms of Pi uptake and transport, and colonization has been observed between various species of AMF (Pearson and Jakobsen, 1993; Ravnskov and Jakobsen, 1995; Smith and Jakobsen, 2000; Smith et al., 2004; Munkvold et al., 2004). Recently, Poulsen et al. (2005) showed that different isolates of the same AMF species interact differently even with a mycorrhizal-defective tomato mutant (rmc). These observations eloquently demonstrate the importance of ‘functional diversity’ in AMF–host symbiosis depending on the strains of G. intraradices used in the experiments. Another reason behind the dissimilarity in results could be the different experimental growth conditions used to establish symbiosis. Nagy et al. (2005) examined a 10-d-old plant in a cuvette system, while in the present study the plants examined were grown in 3 dm3 pots throughout the growth period. The present work was carried out in Israel during the summer months; a period characterized by high sunlight and illumination. Under such conditions, a highly vigorous AMF–host interaction is generally observed. Altering the LePT4 gene expression under these conditions could have triggered major physiological changes, including changes in the host carbon dynamics, resulting in growth retardation. By contrast, the earlier work was carried out under artificial light illumination, a condition which may be sub-optimal for a vigorous AMF establishment. Under such conditions, any subtle physiological difference due to the alteration of LePT4 gene expression could easily get masked, resulting in no perceptible differences. In addition, the mutant lines (lept4-1 and lept4) used in these experiments may also have contributed to these differences. Lack of functional redundancy among the Pi transporters could be further substantiated by the relative resistance of lept4 to very high P levels (5 mM) as compared with the wt which showed inhibited growth (Fig. 1). This suggests that LePT4 may be involved in loading Pi into plants under Pi excess. This response is quite interesting considering that expression of both LePT1 and 2 are usually suppressed in the presence of high Pi (Daram et al., 1998; Liu et al., 1998). A similar lack of functional redundancy among high-affinity Pi transporters Pht1;1 and Pht1;4 in Arabidopsis was demonstrated in earlier studies (Mission et al., 2004; Shin et al., 2004).
Biomass production and Pi uptake capacity by roots of the two genotypes under solution culture were similar (Figs 2, 3B); however, under near realistic growth conditions (pot experiments), the DW production and ability of the wt plant to acquire Pi was significantly higher compared with the lept4 mutant (Fig 1C; Table 3). Note that in a well-stirred solution the Pi-deficient zone around the roots is rarely developed while in the quartz-sand substrate the depletion zone in the vicinity of the root–substrate interface limits Pi uptake (Barber, 1995). The effect of ‘loss of function’ of LePT4 was more pronounced in mycorrhiza-inoculated plants maintained under low Pi conditions. For example, following inoculation of the wt a significant increase was observed in both its growth and Pi uptake (∼90%) compared with the non-infected plants (Fig. 4; Table 3). Although a similar trend was observed for the mutant plants, the magnitude of increase (50–60% above non-infected plants) was significantly lower (Fig. 4; Table 3). The beneficial effect of mycorrhizas on Pi uptake was correlated with the enhanced transcript level of LePT4 in the wt upon infection (Fig. 5). The present study highlights the potential role of LePT4 as a dual-function transporter with a major role in Pi uptake during mycorrhizal colonization of the plant. Further empirical evidence ascertaining their physiological role could be established by their tissue, cellular, and subcellular distribution.
It was interesting to observe that the effect of mycorrhizal inoculation on Pi uptake was still evident despite the functional inactivation of LePT4 (Fig. 5). This suggests that, in addition to LePT4, other Pi transporters could also be potentially involved in mycorrhizal-mediated Pi uptake in tomato. The identification of LePT3, based on its homology with StPT3, and its enhanced expression in the presence of mycorrhizal infection (Fig. 5) suggest the potential role of LePT3 as an alternative route for mycorrhiza-mediated Pi uptake. In addition, LePT5, whose expression is also inducible by mycorrhizal infection (Nagy et al., 2005), could be another candidate involved in mycorrhiza-mediated Pi uptake in tomato. Multiple gene mutants for LePT3, LePT5, and LePT4 are needed to obtain a more comprehensive understanding of molecular regulation of Pi uptake in tomato.
The authors are grateful to the anonymous reviewers for critical readings of the article and their constructive comments. This paper is contribution No. 604/07 of the Agricultural Research Organization of The Volcani Center, Israel. We thank Aiquan Chen and Baozhen Li for conducting the hydroponic experiments, Smadar Wininger for detection of mycorrhizal colonization, and Irit Levkovitch for chemical analysis. This work was supported by the Israel-US Bi-national Agricultural R&D (BARD) Program (grant no. US-3231-01R), China National Nature Science Foundation, China 973 Programme 2005CB120903), and 111 project (No. B07030). GX was a recipient of a Kaplan Postdoctoral Fellowship of the Weizmann Institute of Science.
Comments