-
PDF
- Split View
-
Views
-
Cite
Cite
Fedor A. Brovko, Victoria S. Vasil'eva, Anna O. Shepelyakovskaya, Svetlana Yu. Selivankina, Guzel R. Kudoyarova, Alexander V. Nosov, Dmitry A. Moshkov, Alexander G. Laman, Khanafy M. Boziev, Victor V. Kusnetsov, Olga N. Kulaeva, Cytokinin-binding protein (70 kDa): localization in tissues and cells of etiolated maize seedlings and its putative function, Journal of Experimental Botany, Volume 58, Issue 10, July 2007, Pages 2479–2490, https://doi.org/10.1093/jxb/erm108
- Share Icon Share
Abstract
The distribution pattern of a 70 kDa cytokinin-binding protein (CBP70) was studied in 4-d-old etiolated maize seedlings (Zea mays L., cv. Elbrus). CBP70 was detected in crude protein extracts of all root zones and shoot parts by western blotting and by the sandwich ELISA (enzyme-linked immunosorbent assay) technique, using a pair of monoclonal anti-CBP70 antibodies cross-reacting with non-overlapping protein epitopes. The highest amount of CBP70 was found in the root meristem, which corresponds to the concentration in the meristem of zeatin, its riboside, nucleotide, and 9N-glucoside. CBP70 accumulation was also detected in other zones of cell division: in the root cap, shoot apex, and vascular tissues, suggesting involvement of the protein in the processes related to cell proliferation. This suggestion was also supported by CBP70 distribution in the root meristem: mitotically inactive cells of the quiescent centre did not contain a detectable amount of the protein. Stem cells adjoining the quiescent centre contained less CBP70 than their daughter cells. Using monoclonal antibodies against CBP70 for immunocytochemistry, the presence of the protein in the cytoplasm and its accumulation in nuclei and especially in nucleoli was demonstrated; such a pattern was observed in all cell types of seedlings. The subcellular distribution pattern of CBP70 was analysed by immunogold electron microscopy of the meristem and leaf cells; CBP70 was localized in the cytoplasm and nucleoplasm, and its highest concentration was detected in nucleoli. CBP70 was not detected in the vacuole and cell wall. In the RNA polymerase I model system, purified CBP70 mediated a trans-zeatin-dependent activation of transcription in vitro, and anti-CBP70 monoclonal antibodies blocked this activation. Other natural and synthetic physiologically active cytokinins also activated transcript elongation in the model system in the presence of CBP70. Adenine and inactive analogues of cytokinins had no such effects. These data suggest that CBP70 is a transcript elongation factor or a modulator of elongation factor activity specifically mediating a cytokinin-dependent regulation of transcription.
Introduction
Cytokinin was discovered as a factor inducing, together with auxin, cell division in cell culture (Miller et al., 1955). Later, many other cytokinin activities in the regulation of plant growth and development were demonstrated (Mok and Mok, 2001). Recently, cytokinin involvement in the regulation of shoot and root meristem activity was shown (Werner et al., 2003; Higuchi et al., 2004; Nishimura et al., 2004). Considerable progress in understanding the cytokinin mode of action was achieved due to the discovery of membrane-bound cytokinin receptors (CRE1/AHK4/WOL, AHK2, and AHK3), which belong to the class of sensor histidine kinases of the two-component regulatory system (Inoue et al., 2001; Ueguchi at al., 2001), identification of the genes of the primary response to cytokinin (ARR type-A genes) (Brandstatter and Kieber, 1998; Sakakibara et al., 1998; D'Agostino et al., 2000), and transfactors of ARR type-B controlling expression of the ARR type-A genes (Sakai et al., 2001). These discoveries led to the concept of a phosphate cascade in cytokinin signalling (Schmülling, 2001; Ferreira and Kieber, 2005; Sakakibara, 2006). However, more recently, analysis of triple Arabidopsis thaliana mutants displaying damage to all three receptor histidine kinases suggested that cytokinin signalling might be more complex and involve other perception and transduction systems (Higuchi et al., 2004; Nishimura et al., 2004).
To this end, a search for other players in cytokinin signal transduction in plant cells is of interest. Sensor histidine kinases are known to recognize cytokinin by its extracellular CHASE domain and, therefore, are likely to be involved in the perception of extracellular cytokinin. However, cytokinins can also penetrate into plant cells using purine transporters (Bürkle et al., 2003) and probably nucleoside transporters (Hirose et al., 2005). In fact, cytokinins have been detected in different compartments of the plant cell, including the cytoplasm, nucleus, and chloroplasts (Hare and Van Staden, 1997; Benkova et al., 1999; Dewitte et al., 1999). This implies the occurrence of intracellular cytokinin targets or receptors. The presence of both membrane and intracellular (nuclear) receptors was found for auxin (Badescu and Napier, 2006). A number of receptors have now been discovered for abscisic acid (Razem et al., 2006; Shen et al., 2006). Therefore, it is possible that cytokinins, along with membrane receptors, also interact with receptors of other types. Cytokinin-binding proteins (CBPs), if linked with the control of transcription, are likely to be intracellular cytokinin targets. Such proteins with a molecular mass of 67 kDa (CBP67) were isolated from the cytosol and nuclei of mature barley leaves (Kulaeva et al., 2000) and senescent leaves of A. thaliana (Selivankina et al., 2004). The complex of these proteins with trans-zeatin (tZ) activated transcript elongation in test systems containing chromatin and RNA polymerase I, or nuclei isolated from the leaves served as a source for CBP isolation (Selivankina et al., 2001, 2004). The correlation between cytokinin binding to CBP67 and their activities, in complex with the protein, in transcription regulation (Kulaeva et al., 1998; Selivankina et al., 2001) suggested that CBP67s could be related to cytokinin intracellular perception and its signal transduction to the processes participating in control of transcription (Selivankina et al., 2004).
A protein with a molecular mass of 70 kDa (CBP70) was also isolated from etiolated maize seedlings. This protein binds cytokinin with a high specificity and affinity (Brovko et al., 1996; Shepelyakovskaya et al., 2002) and shares immunodeterminants with CBP67 from barley leaves (Kulaeva et al., 1998). Up to now, there is no evidence of the localization of such types of CBPs in plant tissues and plant cells. A set of monoclonal mouse antibodies (mAbs) raised against maize CBP70 (Zagranichnaya et al., 1997) and the selection of a pair of them cross-reacting with non-overlapping epitopes of CBP70 (Shepelyakovskaya et al., 2002) made it possible to analyse the protein in plant material by a very sensitive sandwich ELISA (enzyme-linked immunosorbent assay) technique using streptavidin–biotin detection. Immunocytochemistry and immunogold electron microscopy make it possible to analyse CBP70 localization in seedlings.
The aims of the present study were: (i) to elucidate the distribution of CBP70 in etiolated maize seedlings and to compare the distribution pattern of CBP70 with that of endogenous cytokinins; (ii) to analyse CBP70 localization in seedling cells; and (iii) to examine CBP70 activity in the cytokinin-dependent regulation of transcription in vitro to extend the studies on CBP function.
Materials and methods
Plant materials and treatments
Maize (Z. mays L., cv. Elbrus) seeds were surface-sterilized and germinated on moistened filter paper at 27 °C and 95% humidity in darkness. Four-day-old seedlings were sampled for immunocytology and CBP70 and cytokinin analyses. Barley (Hordeum vulgare L., cv. Viner) plants were grown in boxes with soil in a growth chamber at a 16 h photoperiod, 22/18 °C day/night temperature, 222 μmol m−2 s−1 light intensity, and 70% relative humidity. The fully expanded first leaves of 10-d-old plants were used for chromatin isolation.
CBP70 isolation
All steps of protein isolation were performed at 0–4 °C. A 100 g aliquot of etiolated 5-d-old maize seedling shoots was homogenized for 10 s at 20 000 rpm with the Ultraturax T25 homogenizer (Ika, Germany) in buffer A (50 mM TRIS-HCl, pH 7.5, 2 mM MgCl2, 2 mM EDTA-Na2, 50 mM KCl), containing 0.5 mM phenylmethylsulphonyl fluoride (PMSF) and 5 mM 2-mercaptoethanol (2-ME). In preliminary experiments, it was shown that these conditions for homogenization resulted in the highest CBP70 yield. The homogenate was filtered through Miracloth and centrifuged at 10 000 g for 15 min. The protein fraction of the supernatant was purified by gel filtration chromatography on a column (5×60 cm) of Sephadex G-25 Fine (Pharmacia, Sweden) equilibrated with buffer A. The eluted proteins were loaded on the DEAE-Sepharose column (1.6×20 cm, Pharmacia, Sweden) equilibrated with buffer A. The column was washed with five bed volumes of buffer A, and CBP70 was eluted with buffer A containing 200 mM KCl. Thereafter, the protein was loaded on an adenosine Toyopearl (A-Toyopearl) column (1.6×10 cm) attached to a trans-zeatin riboside Toyopearl (tZR-Toyopearl) column (2.6×20 cm). Both columns were equilibrated with buffer A. Adenosine and tZR were immobilized on aminopropyl Toyopearl using a modified procedure of Erlanger and Beiser (1964). The A-Toyopearl column was used for removing the adenine-binding proteins and other unspecifically binding proteins. After sample application, the columns were washed with two bed volumes of buffer A, the A-Toyopearl column was detached, the tZR-Toyopearl column was additionally washed with three bed volumes of buffer A containing 500 mM KCl, and CBP70 was eluted with 6 M guanidine-HCl. Protein fractions were collected, and concentrated using an 8MC Concentrator Cell with 30YM membrane (Amicon, USA). Finally, the protein solution was desalted on a PD10 column equilibrated with buffer A and stored at –70 °C.
Preparation of crude protein extracts from plant tissues
Primary roots were sectioned as follows: division zone plus root cap (from 0 mm to 2 mm from the root tip), the elongation zone (from 2 mm to 7 mm), the segment of the differentiation zone (from 7 mm to 17 mm), and a 10 mm segment from the middle part of the lateral root formation zone. The mesocotyl, node, coleoptile, and the first leaf were excised from the shoot. Plant material (50–250 mg) was placed in the centrifuge tube, five volumes of A buffer containing 1 mM PMSF and 8 mM 2-ME were added, and plant tissue was homogenized by Ultraturax T25 at 20 000 rpm. In preliminary experiments, the optimum time for homogenization of every tissue was determined: 2–3 s for root tips, 4 s for the coleoptiles, mesocotyls, and leaves, and 6 s for the lateral root formation zones. The homogenate was centrifuged at 48 000 g and 4 °C for 10 min. The supernatant was separated from low-molecular-weight compounds using Sephadex G-25 Fine mini-columns equilibrated with buffer A. Protein solutions were used for CBP70 analysis by immunoblotting and sandwich ELISA.
Electrophoresis and immunoblotting
Proteins from the crude extracts of plant tissues were analysed by SDS-PAGE (12% w/v) according to the method of Laemmli (1970). The samples of crude extracts loaded onto the gel were equalized in the amounts of CBP70 (estimated by sandwich ELISA).
Separated proteins were transferred to Hybond-C extra (Amersham, UK) in a semi-dry electro blotter (Biometra, UK) according to the manufacturer's recommendations using the Towbin buffer system (Towbin et al., 1979). Protein bands were visualized with 0.5% Ponceau S solution in 1 M acetic acid. The membrane was rinsed three times with water and blocked with 1% bovine serum albumin (BSA) in PBS-T [phosphate-buffered saline containing 0.1% (v/v) Tween-20] overnight at 4 °C. Anti-CBP70 mouse mAb (100 ng ml−1) in PBS-T was added for 1 h at room temperature. Anti-CBP70 mouse antibody was detected using secondary antibody conjugated to alkaline phosphatase (Bio-Rad Laboratories, USA) according to the supplier's recommendations. The enzyme colour reaction was developed with 3-bromo-4-chloro-5-indolyl phosphate (BCIP) and nitro blue tetrazolium (NBT).
Sandwich ELISA
The content of CBP70 in the crude extracts from the plant material was determined by sandwich ELISA using the mAbs Z-5 and Z-8 (Shepelyakovskaya et al., 2002). The mAb Z-8 was biotinylated using biotin N-hydroxysuccinimide ester (Harlow and Lane, 1988). The mAb Z-5 was dissolved in carbonate–bicarbonate buffer (100 mM NaHCO3/Na2CO3, pH 9.6, 10 μg ml−1) and incubated in microtitre 96-well IFA Multiwell plates overnight at 4 °C. Wells were washed twice with PBS [20 mM Na2HPO4/NaH2PO4 and 0.15 mM NaCl, pH 7.2, containing 1% (w/v) BSA] and incubated at 37 °C for 1 h with the same solution. Serial 2-fold dilutions of the crude extracts were added to the corresponding wells and incubated overnight at 4 °C. The medium was then removed, and the wells were washed five times with PBS-T. The biotinylated mAb Z-8 (10 μg ml−1) was dissolved in PBS-T, added to each well, incubated for 2 h at room temperature, and wells were washed five times with PBS-T. The streptavidin–peroxidase conjugate (Amersham, UK) (diluted 1:1000 in PBS-T) was then added and left to react for 1 h at 37 °C, then the wells were washed four times with PBS-T and twice with PBS. A 0.1 ml aliquot of the substrate solution [0.43 mg ml−1o-phenylenediamine, 0.01% (v/v) H2O2, 0.02 M citric acid, and 0.05 mM Na2HPO4, pH 5.0] was added to each well and incubated for 20 min at 24 °C; the reaction was stopped with 0.1 M H2SO4, and the plates were read with Titertek Multiscan (Flow Lab, UK) at 492 nm.
Immunocytochemistry
Root tips 2 mm long, nodes with 1 mm of adjacent organs (mesocotyl and leaves with coleoptile), and the parts of hypocotyl and coleoptile 1 mm long of 4-d-old etiolated maize seedlings were cut off and fixed immediately in 4% (w/v) formaldehyde, 0.1% (w/v) glutaraldehyde, and 0.01% (w/v) picric acid in 20 mM sodium phosphate buffer (pH 7.4) for 8–12 h at 4 °C. After washing in distilled water, the samples were dehydrated in graded ethanol (to 100%) and embedded in LR White (Sigma, USA). Polymerization was carried out under UV light for 12 h. Sections from embedded samples were cut with an Ultracut E ultramicrotome (Reichert-Jung, Austria). For light microscopy, semi-thin sections (1–0.7 μm) were placed on glass slides coated with γ-aminopropyltriethoxysilane. For electron microscopic observations, ultrathin sections (gold) were mounted onto Au grids.
Prior to immunolabelling, sections were permeabilized with 0.5% (v/v) Triton X-100 and incubated in 0.1% (w/v) NaBH4 to inactivate residual aldehydes. The sections were incubated in the blocking solution of BSA-G [3% (w/v) BSA and 0.5% (w/v) gelatine in PBS] for 1.5 h at 37 °C and then incubated at 4 °C overnight with the primary mAbs against CBP70 (17 μg ml−1) diluted in the BSA-T solution [1% (w/v) BSA and 0.01% (v/v) Triton X-100 in PBS]. Slides were washed in the W solution [0.02% (w/v) NaN3, 0.1% (v/v) Triton X-100, and 0.1 M glycine in PBS] for 10 min and incubated with the BSA-G solution for 20 min. Then the sections were incubated with biotinylated anti-mouse antibodies (Amersham, UK) in BSA-T for 6 h at 4 °C and washed in the W solution for 10 min. The reaction with the streptavidin–alkaline phosphatase conjugate (Amersham) in BSA-T was performed for 1.5 h at 37 °C, slides were washed in the W solution for 10 min, then incubations with biotinylated anti-mouse antibodies and streptavidin–phosphatase conjugate (for 1.5 h at 37 °C each) were repeated. Sections were incubated with the substrate solution (3.8 mM BCIP, 4 mM NBT, 10 mM MgCl2, 150 mM NaCl, 50 mM TRIS-HCl, pH 9.5) for 15 min. The reaction was stopped by washing in 20 mM TRIS-HCl, pH 7.5. The stained dry sections were mounted in cedar wood oil, and images were obtained under a Univar microscope (Reichert-Jung, Austria).
Three negative controls were used: (i) mouse anti-CBP70 mAbs were omitted from material treatment; (ii) mouse anti-CBP70 mAbs were substituted by mouse mAbs raised against a protein which is absent in plants (anti-Myc antibodies); and (iii) mouse anti-CBP70 mAbs were substituted by mouse non-immune serum (5% mouse serum diluted by PBS). An equal low unspecific staining level was detected for all three controls under the chosen conditions of immunolabelling.
Immunolabelling procedure for electron microscopy
As mentioned above, ultrathin sections (gold) for electron microscopy were mounted onto Au grids. Grids with sections of the root meristem and leaf tissues of 4-d-old etiolated maize seedlings were incubated in a moisture chamber; most of the procedures were carried out at room temperature. Initially, specimens were incubated with a drop of freshly prepared 1% (w/v) aqueous solution of NaBH4 for 30 min, and then they were rinsed with distilled water (three times 5 min each). Unspecific antibody binding was blocked by incubation of specimens in the 5% solution of BSA (w/v) in PBST-500 (16.7 mM Na2HPO4, 3.3 mM KH2PO4, 500 mM NaCl, and 0.1% Tween-20, pH 7.4) for 20 min. The following reactions were carried out using the same buffer; after each incubation, specimens were washed in PBST-500 with 50 mM glycine and 0.5% Tween-20. Specimens were incubated with primary anti-CBP70 antibodies for 1 h at 37 °C. Control sections were incubated with non-immune serum. Then sections were incubated with the solution of biotinylated anti-mouse antibodies (Amersham) (at a dilution of 1:100) for 1 h at room temperature, followed by incubation in the solution of streptavidin–gold (15 nm) (Amersham) (at a dilution of 1:100) under the same conditions. The labelled sections were post-stained in uranyl acetate and Reynold's lead citrate before examination with a Tesla TS-500 electron microscope (Czech Republic) (Vandenbosch, 1991).
Cytokinin determination
Cytokinins were extracted from plant tissues, purified, and separated by thin-layer chromatography (TLC) as described earlier (Vysotskaya et al., 2001). Cytokinin-containing zones (based on standard positions) were eluted with 0.1 M phosphate buffer, pH 7.4, for 12 h, and the eluates were added directly to microtitre plate wells in serial dilutions. They were assayed using antibodies against zeatin riboside, which has been shown to be highly specific for several zeatin derivatives (Kudoyarova et al., 1998). By this technique, zeatin nucleotide (RF 0–0.1), zeatin riboside (RF 0.4–0.5), and zeatin (RF 0.6–0.7) were successfully separated and assayed. More than 90% recovery was obtained for zeatin, and its riboside and glucoside standards using the described elution procedure. Recovery of zeatin nucleotide was not measured directly. However, the sum of immunoreactivity of the eluted fractions was not more than 20% lower than that of samples applied to the TLC plate, suggesting that most of the immunoreactive material was successfully recovered.
Chromatin isolation and measuring the RNA polymerase I activity
Chromatin was isolated from barley leaves according to the protocol described previously (Selivankina et al., 2004). Transcription elongation directed by chromatin-associated RNA polymerase I was measured in the reaction medium (100 μl) containing 50 mM TRIS-HCl, pH 8.0, 10 mM MgCl2, 10 mM 2-ME, 0.2 mM CTP, GTP, ATP, 0.01 mM UTP, and [3H]UTP (1.11 TBq mmol−1) (Amersham Biosciences, UK), and 10–50 μg of chromatin DNA. The reaction was carried out at 32 °C for 20 min and stopped by the addition of an equal volume of cold 0.9% sodium pyrophosphate in 10% trichloroacetic acid.
Protein assay
Protein content was estimated by the method of Esen (1978).
Determination of the number of cells
Plant material was macerated in 10% H2Cr2O7 at 60 °C for 20 min. The number of cells was counted in a Fuchs–Rosenthal haemocytometer as described by Brown and Rickless (1949).
Statistics
All experiments were performed three times with three replications each. Figures and tables present the mean values and their standard errors (SE).
Results
CBP70 localization in 4-d-old maize seedlings
The distribution of CBP70 in 4-d-old etiolated maize seedlings was analysed. For this purpose, crude protein extracts were prepared from various root zones and shoot organs, and analysed by western blotting using an mAb against CBP70. The loaded samples were equalized in the amounts of CBP70 on the basis of sandwich ELISA results. The CBP70 was detected in all root zones studied: the meristem, elongation, differentiation, and lateral root formation zones (Fig. 1). In shoots, CBP70 was detected in the mesocotyl, the node containing the shoot apex, coleoptile, and first leaf (Fig. 1). Crude protein extracts from all these tissues and organs were analysed to quantify the CBP70 by using a sandwich ELISA. The results obtained showed that the highest content of CBP70 was in the root meristem (Fig. 2A, B, D) where it exceeded the content in other tissues and organs by 10–250 times (Fig. 2A). The root elongation zone contained 10 times less CBP70 than the meristem, but significantly more than the zones of root cell differentiation and lateral root formation (Fig. 2A). The lowest level of CBP70 was in mesocotyl. In shoots, the highest amount of CBP70 was in the node containing dividing cells of shoot meristem and leaflets. Thus, the highest amount of CBP70 in roots and shoots was in the zones of cell division.
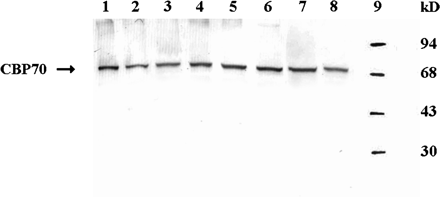
Western immunoblot detection of CBP70 in crude protein extracts from various parts of 4-d-old etiolated maize seedlings. Crude protein extracts were analysed by SDS-PAGE. The samples loaded were equalized in the amounts of CBP70 on the basis of sandwich ELISA data. Blots were probed with mouse anti-CBP70 mAb, and the antibody was detected using the secondary anti-mouse antibody conjugated with alkaline phosphatase. Lane 1, root meristem; lane 2, root elongation zone; lane 3, root differentiation zone; lane 4, zone of lateral root formation; lane 5, first leaf; lane 6, node; lane 7, coleoptile; lane 8, mesocotyl; lane 9, molecular weight standards.

Relative contents of CBP70 and total protein in various parts of 4-d-old-etiolated maize seedling. CBP70 content in crude protein extracts was estimated by sandwich ELISA using a pair of anti-CBP70 mAbs. Biotinylated mAbs were recognized by a streptavidin–peroxidase conjugate. Peroxidase reaction was recorded at 492 nm. Mean values ±SE are presented. 1, Root meristem and root cap (0–2 mm from the root tip); 2, elongation zone (2–7 mm from the root tip); 3, 10 mm segment of the differentiation zone (7–17 mm from the root tip); 4, 10 mm segment from the middle part of the lateral root formation zone; 5, mesocotyl; 6, node; 7, coleoptile; 8, first leaf. (A) Relative content of CBP70 on a fresh weight basis (A492 g−1 FW). (B) Relative content of CBP70 on a cell basis (A492 per 106 cells). (C) Total protein content on a cell basis (mg per 106 cells). (D) Relative content of CBP70 per total protein unit (A492 mg−1 total protein). (E: insert) Enlarged fragment of D.
Since the sizes of cells from various tissues are very different, it was important to calculate the content of CBP70 not only per fresh weight (FW) basis, but also per cell. This was achieved by counting the number of the cells per 1 g FW (Table 1) and calculating the relative amount of CBP70 per cell in various tissues (Fig. 2B). The total protein was also measured in various parts of seedlings, and the accumulation of CBP70 and total protein in cells of these tissues were compared (Fig. 2B, C).
The number of cells in various tissues of 4-d-old etiolated maize seedlings
Root zone and shoot organ | No. of cells×106 g−1 FW |
Root meristem | 129.1±8.4 |
Root elongation zone | 15.9±2.6 |
Root differentiation zone | 9.8±0.9 |
Zone of lateral root formation | 12.0±0.4 |
Mesocotyl | 9.8±1.1 |
Node | 8.0±1.9 |
Coleoptile | 14.2±1.8 |
Leaf | 243.7±5.3 |
Root zone and shoot organ | No. of cells×106 g−1 FW |
Root meristem | 129.1±8.4 |
Root elongation zone | 15.9±2.6 |
Root differentiation zone | 9.8±0.9 |
Zone of lateral root formation | 12.0±0.4 |
Mesocotyl | 9.8±1.1 |
Node | 8.0±1.9 |
Coleoptile | 14.2±1.8 |
Leaf | 243.7±5.3 |
The root meristem, the elongation zone, a 10 mm long segment of the differentiation zone, and a 10 mm segment from the middle part of the lateral root formation zone were excised from primary roots of 4-d-old seedlings. Mesocotyls, nodes, coleoptiles, and first leaves were excised from seedlings. Three independent experiments were carried out with five replications each. Mean values ±SE are presented.
The number of cells in various tissues of 4-d-old etiolated maize seedlings
Root zone and shoot organ | No. of cells×106 g−1 FW |
Root meristem | 129.1±8.4 |
Root elongation zone | 15.9±2.6 |
Root differentiation zone | 9.8±0.9 |
Zone of lateral root formation | 12.0±0.4 |
Mesocotyl | 9.8±1.1 |
Node | 8.0±1.9 |
Coleoptile | 14.2±1.8 |
Leaf | 243.7±5.3 |
Root zone and shoot organ | No. of cells×106 g−1 FW |
Root meristem | 129.1±8.4 |
Root elongation zone | 15.9±2.6 |
Root differentiation zone | 9.8±0.9 |
Zone of lateral root formation | 12.0±0.4 |
Mesocotyl | 9.8±1.1 |
Node | 8.0±1.9 |
Coleoptile | 14.2±1.8 |
Leaf | 243.7±5.3 |
The root meristem, the elongation zone, a 10 mm long segment of the differentiation zone, and a 10 mm segment from the middle part of the lateral root formation zone were excised from primary roots of 4-d-old seedlings. Mesocotyls, nodes, coleoptiles, and first leaves were excised from seedlings. Three independent experiments were carried out with five replications each. Mean values ±SE are presented.
The average cell in the elongation zone was 8-fold larger and contained three times more total protein than an average cell in the meristem, but the content of CPP70 in the cells was half that in the meristem cells (Fig. 2B), indicating partial degradation of CBP70 during cell elongation.
The amount of CBP70 in various parts of seedlings per milligram of protein was also calculated. The data obtained (Fig. 2D, E) suggested that CBP70 constituted a considerably larger fraction of total protein in meristematic cells than in other root tissues. It also revealed a significant CBP70 accumulation in the node, which contained dividing cells of the shoot apex and young leaflets (Fig. 2E). No correlation was observed between the content of total protein and CBP70 in different parts of the seedling (cf. Fig. 2B, C). Thus, CBP70 was predominantly located in the root and shoot zones containing dividing cells, particularly in the root meristem.
Distribution of endogenous cytokinins in seedling root zones
Analysis of endogenous cytokinins in the root zones demonstrated that the meristem contained much more of all cytokinin forms than other root zones (Fig. 3). The content of zeatin, a physiologically active form of cytokinin, decreased in the elongation zone more sharply than that of other cytokinin forms. At the same time, cytokinin content in the elongation zone was much higher than in the zones of differentiation and lateral root formation, i.e. a progressive decrease in all cytokinin forms was observed from the meristem to other root zones. These data correspond fully to a CBP70 distribution pattern in the root zones of etiolated maize seedlings.
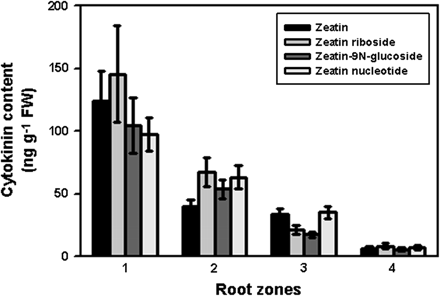
Cytokinin content in roots of 4-d-old etiolated maize seedlings. Root zones: 1, meristem; 2, elongation zone; 3, zone of differentiation; 4, zone of lateral root formation. Mean values ±SE (n = 9).
CBP70 localization within the cells of the etiolated maize seedlings
CBP70 localization within the cells of the 4-d-old etiolated maize seedlings was examined immunocytochemically, using a mouse mAb against CBP70 (Zagranichnaya et al., 1997). Localization of the antibody was detected with anti-mouse biotinylated antibody and streptavidin conjugated with alkaline phosphatase. CBP70 was not detected on control longitudinal sections of the root meristem, which were not treated with mAb against CBP70 (Fig. 4B, F). Root cells treated, instead of with mAb against CBP70, with non-immune serum (Fig. 4G) or with mouse mAbs raised against a protein which is absent in plants (anti-Myc antibody, Fig. 4H), were also not stained. These data indicate a high specificity of CBP70 detection using anti-CBP70 antibodies.
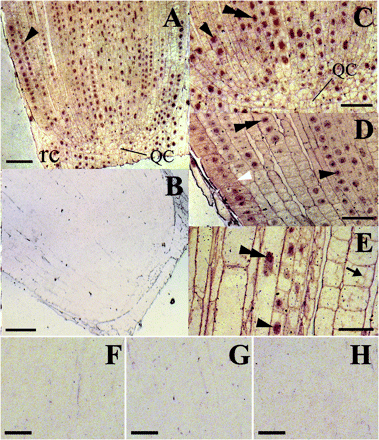
Immunolocalization of CBP70 on longitudinal sections of the root tip of etiolated maize seedlings. LR White-embedded tips (0–2 mm) of the main root from dark-grown 4-d-old maize seedlings were used. (A, C–E) Sections were incubated with mouse anti-CBP70 mAbs, biotinylated anti-mouse antibodies, and streptavidin–alkaline phosphatase conjugate without counterstaining. (B, F) A negative control: sections incubated without primary antibody. Root cells treated with non-immune serum (G) or with mouse monoclonal antibodies raised against a protein which is absent in plants (anti-Myc antibody; H), instead of with mAb against CBP70, were also not stained. (A) CBP70 was detected in the nuclei (black arrowhead) and the cytoplasm of meristematic cells and in nuclei of the root cap (rc) cells. (C) A 3-fold magnified view of the lower part of (A). Nuclei and cytoplasm of the quiescent centre (QC) cells contain much less CBP70, as compared with meristematic cells. CBP70 accumulated in nuclei (black arrowhead), with the highest concentration in nucleoli (black double arrowhead). (D) The root cortex in the region of provascular tissue development. The highest accumulation of CBP70 was detected in nuclei (black arrowhead), mainly in nucleoli (black double arrowhead). The CBP70 concentration in the cytoplasm is especially high in proepidermal and epidermal cells (white arrowhead). (E) Root central cylinder, elongation zone. CBP70 is localized in nuclei, mainly in nucleoli (black double arrowhead) and in cytoplasmic strands (black arrow) of elongating cells. Scale bars are 75 mm (A, B) and 25 mm (C–H).
The highest CBP70 concentration was observed in the nuclei of the meristem cells (Fig. 4A, C, D, black arrowheads) and especially in their nucleoli (Fig. 4C, D, black double arrowhead). CBP70 was also present in the cytoplasm of meristematic cells (Fig. 4C, D, white arrowhead). CBP70 was unevenly distributed within the meristem. The cells of the quiescent centre (QC) contained much less CBP70 than meristematic cells (Fig. 4A, C). Stem cells adjoining the QC, which are characterized by a slow division rate (Laux, 2003), contained less CBP70 in both the cytoplasm and nuclei than their rapidly proliferating daughter cells (Fig. 4A, C). A high content of CBP70 was found in the nuclei of root cap cells (Fig. 4A, rc). A longitudinal section of the root primary cortex in the region of provascular tissue development demonstrates a high CBP70 concentration in the cytoplasm of the protoderm and epidermis cells (Fig. 4D, white arrowhead). In the cells of the elongation zone, CBP70 was detected in nuclei (Fig. 4E, black arrowhead) and especially in nucleoli (Fig. 4E, black double arrowhead). CBP70 was also discerned in the cytoplasmic strands in elongating cells (Fig. 4E, black arrow). However, the total content of CBP70 in the cells of the elongation zone was considerably lower than in the meristem cells (Fig. 4E). These data confirmed the results of biochemical experiments (Fig. 2) and emphasized that the CBP70 concentration was significantly higher in zones of cell division.
Transverse sections of mesocotyl, node, and coleoptile of 4-d-old etiolated maize seedlings demonstrated CBP70 localized in shoot cells (Fig. 5). Control sections, which were not treated with mAb against CBP70, were not stained (Fig. 5A, F, G). Also not stained were control sections treated, instead of with mAb against CBP70, with mouse non-immune serum or with mouse antibodies against a protein which is absent from plants (anti-Myc antibody) (data not shown). These three negative controls demonstrated that CBP70 detection in shoot cells was specific. In the mesocotyl, characterized by the lowest content of CBP70 (Fig. 2), this protein was detected mainly in the cells of the bundle sheath (Fig. 5B). CBP70 was also present in large parenchymal cells (Fig. 5C). In these tissues, as well as in the cells of the coleoptile parenchyma (Fig. 5H) and vascular tissue (Fig. 5I), cell walls/peripheral cytoplasm were strongly stained and it was impossible to discriminate whether CBP70 was present in the cell walls or in the peripheral cytoplasm. However, immunogold electron microscopy clearly demonstrated that CBP70 was absent in the cell wall but abundant in the cytoplasm adjoining the cell wall. This suggests a similar CBP70 distribution in the cells presented in Fig. 5. CBP70 was much more abundant in the node (Fig. 5D, E) than in the mesocotyl and coleoptile (Fig. 5B, C, H, I). The highest CBP70 concentration was observed in the shoot apex and actively dividing leaf cells (Fig. 5D, white double arrowheads). An enlarged fragment of the stem apex section demonstrates a predominant CBP70 localization in the nuclei (Fig. 5E, black arrowheads) and especially in the nucleoli (Fig. 5E, black double arrowhead). The protein was also present in the cytoplasmic strands of growing cells (Fig. 5E, black arrow). Again, the highest CBP70 content was observed in the sites of cell division, where it occurred in both the nucleus and cytoplasm (Fig. 5E, white arrowheads). In the large parenchymal cells of the mesocotyl and coleoptile, CBP70 was detected in the nuclei pressed by the vacuole against the cell wall (Fig. 5B, C, H, I, black arrowheads). Because there was insufficient resolution, it was difficult to be sure that stained structures are really nuclei. However, when tissue sections were treated with safranin O according the method described by Sylvester and Ruzin (1994), these CBP70-containing structures were stained bright red, which is characteristic of nuclei (data not shown). This evidence proves that these structures are really nuclei. The coleoptile vascular tissue contained much more CBP70 than the parenchyma (Fig. 5I).
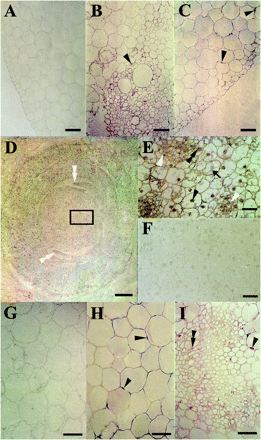
Immunolocalization of CBP70 on cross-sections of mesocotyl (A–C), node (D–F), and coleoptile (G–I) of etiolated maize seedlings. LR White-embedded tissues from dark-grown 4-d-old maize seedlings. (B–E, H, I) Sections were incubated with mouse anti-CBP70 mAbs, biotinylated anti-mouse antibodies, and streptavidin–alkaline phosphatase conjugate. (A, F, G) A negative control: sections incubated without primary antibody. (B) Vascular bundle of the mesocotyl. CBP70 is seen in nuclei (black arrowhead) of phloem cells. (C) Parenchyma of the mesocotyl. CBP70 is localized in the nuclei (black arrowheads), mainly in nucleoli of parenchymal cells. (D) Stem apex region of the node. The highest accumulation of CBP70 is detected in actively dividing cells of the leaf blade edges (white double arrowheads). (E) Detail of (D): stem apex. The cytoplasm and nuclei of small cells at the sites of cell division are well stained (white arrowheads). In largely vacuolated parenchyma cells, nuclei (black arrowhead), especially nucleoli, and cytoplasmic strands (black arrow) are stained. (H) Cross-section of the coleoptile parenchyma. CBP70 is localized in nuclei adjacent to the cell wall (black arrowheads) and in nucleoli. (I) Cross-section of the coleoptile vascular tissue. CBP70 is localized in nuclei (black arrowheads), mainly in nucleoli (black double arrowhead). Scale bars are 50 mm (A–C, G–I), 200 mm (D), and 25 mm (E, F).
Thus, the results of the immunocytochemical analysis of CBP70 localization in shoot organs presented in Fig. 5 are completely consistent with the results of biochemical analyses considered in the previous sections.
Immunogold electron microscopic detection of CBP70 in cells of the root meristem and leaves of 4-d-old etiolated maize seedlings
Subcellular localization of CBP70 and the ultrastructure of cells of the root meristem and leaves of 4-d-old etiolated maize seedlings were studied by immunogold electron microscopy. Ultrathin sections prepared from the root meristem and leaves were successively treated with mouse mAb against CBP70 (or with non-immune serum as control), biotinylated anti-mouse antibody, and streptavidin–gold. The labelled sections were post-stained in uranyl acetate and Reynold's lead citrate. Figure 6 illustrates a representative image of the root meristem (Fig. 6A, C) and leaf cells (Fig. 6B, D). Figure 6C and D demonstrates control sections treated with mouse non-immune serum instead of anti-CBP70 antibody. Gold particles indicate CBP70 localization in the cells (Fig. 6A, B). The highest CBP70 concentration was found in the nucleolus (Fig. 6A, B, black arrowhead). CBP70 was also present in the nucleoplasm (Fig. 6A, B, white arrowhead). The protein concentration in the nucleoplasm was one half of that in the nucleolus (Table 2). The protein distribution in the nucleoplasm was not uniform, demonstrating the regions with predominant CBP70 localization. CBP70 was also well defined in the cytoplasm (Fig. 6A, B). Its concentration in the cytoplasm was substantially lower than in the nucleoplasm and nucleoli (Fig. 6A, B; Table 2). The protein was practically absent from the vacuole and cell wall (Fig. 6A, B). Thus, electron microscopy using the immunogold technique revealed CBP70 localization in the cytoplasm, nucleoplasm, and nucleolus, with predominant accumulation of the protein in the nucleolus, which corresponds to the cytochemistry data (Figs 4, 5).
Gold particle distribution in the root meristem and leaf cells of 4-d-old etiolated maize seedlings (per μm2)
Part of seedling | mAb against CBP70 | Cytoplasm | Nucleoplasm | Nucleolus |
Root meristem | + | 5.0±0.5 | 11.0±5.0 | 21.5±0.8 |
Root meristem | – | 0.50±0.08 | 0.51±0.09 | 0.35±0.06 |
Leaf | + | 6.0±0.4 | 10.0±4.0 | 21.0±1.0 |
Leaf | – | 0.53±0.10 | 0.39±0.08 | 0.20±0.06 |
Part of seedling | mAb against CBP70 | Cytoplasm | Nucleoplasm | Nucleolus |
Root meristem | + | 5.0±0.5 | 11.0±5.0 | 21.5±0.8 |
Root meristem | – | 0.50±0.08 | 0.51±0.09 | 0.35±0.06 |
Leaf | + | 6.0±0.4 | 10.0±4.0 | 21.0±1.0 |
Leaf | – | 0.53±0.10 | 0.39±0.08 | 0.20±0.06 |
Gold particle distribution in the root meristem and leaf cells of 4-d-old etiolated maize seedlings (per μm2)
Part of seedling | mAb against CBP70 | Cytoplasm | Nucleoplasm | Nucleolus |
Root meristem | + | 5.0±0.5 | 11.0±5.0 | 21.5±0.8 |
Root meristem | – | 0.50±0.08 | 0.51±0.09 | 0.35±0.06 |
Leaf | + | 6.0±0.4 | 10.0±4.0 | 21.0±1.0 |
Leaf | – | 0.53±0.10 | 0.39±0.08 | 0.20±0.06 |
Part of seedling | mAb against CBP70 | Cytoplasm | Nucleoplasm | Nucleolus |
Root meristem | + | 5.0±0.5 | 11.0±5.0 | 21.5±0.8 |
Root meristem | – | 0.50±0.08 | 0.51±0.09 | 0.35±0.06 |
Leaf | + | 6.0±0.4 | 10.0±4.0 | 21.0±1.0 |
Leaf | – | 0.53±0.10 | 0.39±0.08 | 0.20±0.06 |
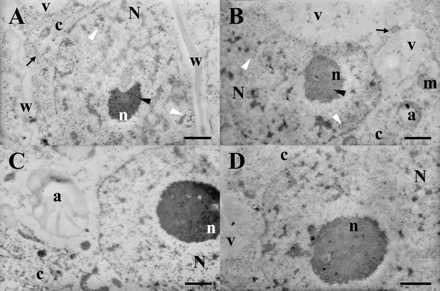
Subcellular localization of CBP70. Ultrathin sections obtained from the root meristem (A, C) and leaf cells (B, D) of 4-d-old etiolated maize seedlings were treated with mouse anti-CBP mAbs (A, B) or with non-immune serum (C, D), biotinylated anti-mouse antibodies, and streptavidin–gold (15 nm). CBP70 is localized in the cytoplasm (black arrows), nucleoplasm (white arrowheads), and nucleolus (black arrowheads). N, nucleus; n, nucleolus; m, mitochondria; c, cytoplasm; a, amyloplast; v, vacuole; w, cell wall. Scale bars are 1 μm.
CBP70 from etiolated maize seedlings mediates cytokinin-dependent activation of transcript elongation in the model system
The high concentration of CBP70 in nuclei and especially in nucleoli of all seedling cells suggested that CBP70 could be involved in the control of transcription. To verify this assumption, a system of transcript elongation in vitro directed by RNA polymerase I, which is tightly associated with the chromatin isolated from barley leaves, was used. RNA polymerase II was lost during chromatin purification (Selivankina et al., 2004). Previously, this system was used to demonstrate CBP67 (isolated from barley leaves) involvement in the cytokinin-dependent transcription activation. CBP70 was purified from etiolated maize seedlings and used for the transcription assay (Fig. 7). In the presence of tZ, CBP70 activated elongation of transcripts in vitro by RNA polymerase I (Fig. 7). CBP70 or tZ alone had no effects on transcription, indicating that CBP70 mediated a cytokinin-dependent regulation of transcript elongation. A specific inhibitor of RNA polymerase II, α-amanitin, had no significant effect on transcription (Fig. 7); thus, under the experimental conditions used, transcript elongation was mediated by RNA polymerase I only. The mAb raised against CBP70 blocked the protein activity in transcription regulation (Fig. 7). Taken together, the data suggest that the presence of functionally active CBP70 is essential for the cytokinin control of rRNA synthesis. CBP70 activated transcription elongation not only in complex with tZ, but also in complex with other physiologically active natural and synthetic cytokinins: isopentenyladenine, 6-benzylaminopurine, and kinetin (Table 3). In contrast to this, inactive analogues of cytokinin and its glucoside had no such effects (Table 3). Therefore, CBP70 interaction with cytokinins is very specific and results in effective complex formation only with functionally active cytokinins.
Effects of CBP70 from etiolated maize seedlings, natural and synthetic cytokinins, and their inactive analogues on RNA synthesis in vitro in the transcription elongation system containing chromatin-bound RNA polymerase I from mature barley leaves
Compound | Concentration (M) | [3H]UMP incorporation into RNA | |
cpm per 20 μg of DNA | % | ||
Control (water) | – | 10 731±397 | 100 |
Trans-zeatin | 10−7 | 31 920±1309 | 297 |
Isopentenyladenine | 10−7 | 25 142±1055 | 234 |
6-Benzylaminopurine | 10−7 | 28 931±1128 | 270 |
Kinetin | 10−7 | 22 261± 957 | 207 |
Adenine | 10−7–10−5 | No effect | – |
Trans-zeatin-O-glucoside | 10−7–10−5 | No effect | – |
6-Benzylthiopurine | 10−7–10−5 | No effect | – |
Compound | Concentration (M) | [3H]UMP incorporation into RNA | |
cpm per 20 μg of DNA | % | ||
Control (water) | – | 10 731±397 | 100 |
Trans-zeatin | 10−7 | 31 920±1309 | 297 |
Isopentenyladenine | 10−7 | 25 142±1055 | 234 |
6-Benzylaminopurine | 10−7 | 28 931±1128 | 270 |
Kinetin | 10−7 | 22 261± 957 | 207 |
Adenine | 10−7–10−5 | No effect | – |
Trans-zeatin-O-glucoside | 10−7–10−5 | No effect | – |
6-Benzylthiopurine | 10−7–10−5 | No effect | – |
Effects of CBP70 from etiolated maize seedlings, natural and synthetic cytokinins, and their inactive analogues on RNA synthesis in vitro in the transcription elongation system containing chromatin-bound RNA polymerase I from mature barley leaves
Compound | Concentration (M) | [3H]UMP incorporation into RNA | |
cpm per 20 μg of DNA | % | ||
Control (water) | – | 10 731±397 | 100 |
Trans-zeatin | 10−7 | 31 920±1309 | 297 |
Isopentenyladenine | 10−7 | 25 142±1055 | 234 |
6-Benzylaminopurine | 10−7 | 28 931±1128 | 270 |
Kinetin | 10−7 | 22 261± 957 | 207 |
Adenine | 10−7–10−5 | No effect | – |
Trans-zeatin-O-glucoside | 10−7–10−5 | No effect | – |
6-Benzylthiopurine | 10−7–10−5 | No effect | – |
Compound | Concentration (M) | [3H]UMP incorporation into RNA | |
cpm per 20 μg of DNA | % | ||
Control (water) | – | 10 731±397 | 100 |
Trans-zeatin | 10−7 | 31 920±1309 | 297 |
Isopentenyladenine | 10−7 | 25 142±1055 | 234 |
6-Benzylaminopurine | 10−7 | 28 931±1128 | 270 |
Kinetin | 10−7 | 22 261± 957 | 207 |
Adenine | 10−7–10−5 | No effect | – |
Trans-zeatin-O-glucoside | 10−7–10−5 | No effect | – |
6-Benzylthiopurine | 10−7–10−5 | No effect | – |
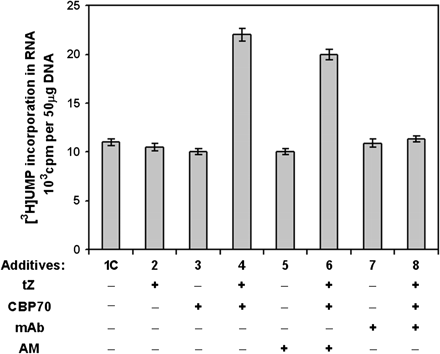
Cytokinin-dependent activation of transcript elongation in vitro by CBP70 from etiolated maize seedlings. The effects of CBP and trans-zeatin (tZ) on RNA synthesis were studied in the transcription elongation system containing RNA polymerase I associated with chromatin isolated from barley leaves. The effects of the following substances added to the reaction medium (100 μl) were studied: CBP70 (20 μg), mAb (20 μg) raised against CBP70, AM, α-amanitin (4 μg ml−1), and tZ (10−7 M),
Discussion
Predominant localization of CBP70 in the zones of cell division of etiolated maize seedlings, its accumulation in nuclei with enrichment in nucleoli
The use of ELISA and western blotting (Shepelyakovskaya et al., 2002) allowed demonstration of the CBP70 distribution pattern in etiolated maize seedlings. CBP70 was detected in all seedling parts and tissues (Figs 1, 2), suggesting its vital importance in plant life.
It is the first information on localization in the whole plant of the protein that belongs to the family of CBPs mediating a cytokinin-dependent regulation of transcript elongation. It was done for CBP70 as the first example of this type of proteins.
Predominant CBP70 localization was established in the zones of cell division of etiolated maize seedlings, especially in the root meristem, where the CBP70 concentration was significantly higher than in other plant organs and tissues (Fig. 2A, B, D). Predominant localization of CBP70 in the zones of active cell proliferation suggested its involvement in the processes related to cell division. CBP70 is distributed in the root meristem non-uniformly. Cells in different regions of a meristem have distinct fates (Laux, 2003). Mitotically inactive cells of the QC did not contain a detectable amount of CBP70 (Fig. 4A, C). Divisions of stem cells adjoining the QC are less frequent than divisions of their daughter cells (Laux, 2003). Corresponding to their lower mitotic activity, stem cells contained less CBP70 than daughter cells (Fig. 4A, C). The data on CBP70 distribution in the root meristem give an additional and a very strong argument for the relationship of the protein to cell division. Actively dividing cells of the root cap (Esau, 1977) were also characterized by a high content of CBP70 in their nuclei (Fig. 4A). It is especially interesting in the context of the data published by Aloni et al. (2005) demonstrating the highest content of active endogenous cytokinins in the root cap of A. thaliana. This mysterious accumulation of CBP70 and cytokinin in the root cap remains to be understood. The present data on cytokinin localization in root zones (Fig. 3) were also correlated with the distribution pattern of CBP70 (Fig. 2), supporting the idea that CBP70 is involved in the cytokinin-dependent regulation of cell division.
In the shoot, as well as in the root, CBP70 was localized mainly in the zone of cell divisions. In the mesocotyl and coleoptile, the zones of cell division are represented by developing vascular tissues (Fig. 5B, I), whose proportion is low relative to extended parenchymal cells (Fig. 5C, H). This explains why a low amount of CBP70 was found in these parts of seedlings by biochemical methods (Fig. 2). A high content of CBP70 in the node detected by biochemical methods (Fig. 2) is consistent with cytological data indicating CBP70 accumulation in the sites of cell division in the shoot apex and leaflets (Fig. 5D). Thus cytochemical data (Figs 4, 5) are in full agreement with biochemical results (Fig. 2) revealing CBP70 accumulation in the zones of cell proliferation. CBP70 was also present in the root elongation zone, although in considerably lower amounts than in the meristem (Figs 2A, B, D, 4E). At the same time, the content of CBP70 in this zone was higher than in many other analysed tissues, and it was decreased further in the zones of differentiation and lateral root formation (Fig. 2A). These results correspond to the pattern of active cytokinin distribution in the root zones of A. thaliana (Aloni et al., 2005) and to the data on isopentenyltransferase gene expression in the roots of A. thaliana (Takei et al., 2004).
Cytochemical analysis using the mAb against CBP70 showed the presence of this protein in the cytoplasm of meristematic cells and its accumulation in nuclei and especially in nucleoli (Fig. 4A, C, D). Nuclear localization of CBP70 in meristematic cells corresponded to the presence of zeatin in nuclei of the cells of the vegetative shoot apex detected in tobacco plants by Dewitte et al. (1999). CBP70 was clearly revealed not only in the cells from dividing zones but also in the nuclei and nucleoli of non-dividing cells, for instance cells of the root elongation zone and mature parenchymal cells of the mesocotyl and coleoptile (Figs 4E, 5C, H). Cytochemical data on CBP70 accumulation in the nucleolus were confirmed by electron microscopy with the use of immunogold labelling (Fig. 6; Table 3). The highest concentration of gold particles in the root meristem and leaf cells was found in the nucleoli, where it was 3–4 times higher than in the cytoplasm and twice as high as in the nucleoplasm (Table 3). CBP70 was not detected in the vacuole and cell wall. As far as we know, this is the first evidence of such a type of CBP localization in plant cells with predominant accumulation in nucleoli.
The data on CBP70 accumulation in nuclei and nucleoli of cells in all seedling tissues suggest the involvement of the protein in the control of transcription.
CBP70-mediated cytokinin-dependent activation of transcript elongation in vitro
The suggestion of the involvement of CBP70 in transcription regulation was confirmed in experiments on transcript elongation in vitro in a model system containing RNA polymerase I tightly associated with chromatin isolated from barley leaves (Selivankina et al., 2004). In this system, new transcripts are not initiated; the system only maintains the elongation of transcripts initiated in vivo. Previously, using this system, a cytokinin-dependent activation of transcript elongation in vitro by barley CBP67 was demonstrated (Selivankina et al., 2001). In the present study, it was shown that maize CBP70 also activated transcript elongation by RNA polymerase I in the presence of tZ (Fig. 7).
The inhibition of this effect by mAbs against CBP70 emphasized the requirement for the functionally active protein for the cytokinin-dependent regulation of transcription (Fig. 7). Other natural and synthetic physiologically active cytokinins replaced tZ in formation of a complex with CBP70, which enhanced transcript elongation in the model system (Table 3), whereas adenine, an analogue of cytokinins but lacking their hormonal activity, and other inactive analogues of cytokinin had no such effects (Table 3). Hence, CBP70 interaction with cytokinins in the regulation of transcript elongation is very specific and is achieved only by bioactive cytokinins. As mentioned above, transcript elongation in the model system in the present experiments was directed by RNA polymerase I synthesizing rRNA precursors. This process is localized in nucleoli. Immunocytochemistry (Figs 4, 5) and immunogold electron microscopy (Fig. 6) demonstrated that CBP70 accumulated in seedling cells in nucleoli. Co-localization of CBP70 and RNA polymerase I in nucleoli, together with results of the in vitro experiments are very important new arguments for the protein's involvement in the cytokinin-dependent regulation of rRNA precursor synthesis. This conclusion is in agreement with cytokinin-induced activation of rRNA synthesis in vivo, which was established previously (Gaudino and Pikaard, 1997). Thus, the results obtained in the model system used in the present experiments adequately reflect the effect of the cytokinin in the cell. All these data suggest that maize CBP70 belongs to the class of transcript elongation factors or proteins controlling elongation factor activity.
The present results demonstrated CBP70 activity only in cytokinin-dependent regulation of transcript elongation by RNA polymerase I. There are no data on the effect of CBP70 on RNA polymerase II, which was lost during chromatin purification (Selivankina et al., 2004), but barley CBP67 (related to CBP70) activated transcript elongation directed not only by RNA polymerase I, but also by RNA polymerase II in nuclei isolated from barley leaves (Selivankina et al., 2001). These results are consistent with the discovery of the common mechanism controlling transcript elongation in yeast by RNA polymerase II and RNA polymerase I (Fath et al., 2004). The presence of CBP70 in maize seedling cells not only in nucleoli but also in the nucleoplasm (Figs 4C, D, E, 6) demonstrated the importance of studying further the participation of this protein in the regulation of RNA polymerase II activity.
Possible involvement of CBP70 in the cytokinin control of cell proliferation
The significance of cytokinins for cell division is well known (Miller et al., 1955; Mok and Mok, 2001). Cytokinins participate in the regulation of the expression of D-cyclins and other genes involved in the cell cycle (Frank and Schmülling, 1999; Riou-Khamlichi et al., 1999; Fountain et al., 2003; Hartig and Beck, 2005).
As discussed above, a predominant localization of CBP70 in the root meristem and other zones of cell division suggests that the protein could be involved in cytokinin control of cell proliferation. At the same time, it is known that receptor histidine kinases CRE1/AHK4/WOL, AHK2, and AHK3 participate in the regulation of cell division by cytokinin (Inoue et al., 2001; Ueguchi et al., 2001; Higuchi et al., 2004; Nishimura et al., 2004). The functions of the three AHKs partially overlap, but all of them are necessary for the proper response of cultured cells to cytokinin. All three AHKs are required for the maintenance of cell division in both stem and root meristems (Higuchi et al., 2004; Nishimura et al., 2004). The analysis of β-glucuronidase (GUS) gene expression under the control of AHK promoters in the roots of transformed A. thaliana plants demonstrated that the genes of all three AHKs were expressed in the root meristem (Nishimura et al., 2004), but CBP70 localization corresponded more accurately to the zones of cell division (Fig. 4A, C) than GUS gene expression under the control of AHK promoters. In intact A. thaliana plants, triple mutants displaying damage to all three AHKs had severely reduced meristems of the shoot and root, which indicates a suppression of cell division and the systems maintaining cells in the state of division and retarding their transit to growth and differentiation. Nevertheless, some reduced mitotic activity was maintained in triple mutants. They develop embryos, seedlings, and dwarf plantlets. Based on these facts, it was concluded that the mechanism of cytokinin action may involve, together with the receptor histidine kinases and phosphate cascade, some other unknown system of hormonal signal perception and transduction (Higuchi et al., 2004; Nishimura et al., 2004). In this connection, maize CBP70, studied in this work, might also be involved in transduction of the cytokinin signal. As discussed above, CBP70 belongs to the transcript elongation factors or proteins controlling elongation factor activity. Recently, numerous eukaryotic elongation factors facilitating transcription directed by either RNA polymerase I or II were identified (Bjorklund et al., 1999; Yik et al., 2005). A complex system involved in the control of elongation factor activity was elucidated recently, and some participating proteins were isolated (Fath et al., 2005; Yik et al., 2005). It was found that the control of transcript elongation is a very important step for the regulation of gene expression in eukaryotes (Bjorklund et al., 1999) and that transcript elongation factors are involved in the regulation of cell proliferation not only in animals but also in plants (Grasser, 2005; Nelissen et al., 2005). Further studies of the mechanism of cytokinin-dependent regulation of transcript elongation by CBP70 could be a key to understanding the protein's involvement in the processes related to cytokinin control of cell division.
Abbreviations
- BCIP
3-bromo-4-chloro-5-indolyl phosphate
- BSA
bovine serum albumin
- CBP
cytokinin-binding protein
- ELISA
enzyme-linked immunosorbent assay
- mAb
monoclonal antibody
- 2-ME
2-mercaptoethanol
- NBT
nitro blue tetrazolium
- PMSF
phenylmethylsulphonyl fluoride
- QC
quiescent centre
- tZ
trans-zeatin
We thank Professors Nella L Klyachko, Vasily M Studitsky, and Richard M Napier for critical reading and helpful discussion of this manuscript, and Dr Elena A Burkhanova for counting the cell numbers and protein estimation. This work was partly supported by the Russian Foundation for Basic Research, project nos 05-04-48289 and 05-04-49411, and by a grant from the President of the Russian Federation for the Support of Leading Scientific Schools, no. NSh-3692.2006.4
Comments